Resistant starch (RS) is defined as starch that escapes digestion in the small intestine of healthy individuals. Potential food applications of RS are of interest to product developers and nutritionists for two reasons: (1) fibre-fortification and the potential physiological benefits of RS, which has fibre-like functions; and (2) the unique functional properties of RS, which can yield high-quality products that are not attainable otherwise with traditional insoluble fibre. RS has been classified into four types, termed RS-type 1 to RS-type 4, depending on the cause of digestive resistance( Reference Englyst, Kingman and Cummings 1 , Reference Topping, Morell and King 2 ).
High-amylose starch usually comprises more than 50 % amylose( Reference Li, Jiang and Campbell 3 ) and is classified as RS-type 2. High-amylose starch resists digestion due to its granular structure and molecular architecture. High-amylose maize starch (HAMS) has been reported to provide many health benefits( Reference Anderson 4 ), and is often used as a source of dietary fibre( Reference Morita, Ohhashi and Ikai 5 ).
Starch can be widely modified by chemical methods and is subsequently classified as RS-type 4. Chemically modified starch includes starch esters, starch diethyl ethers and cross-bonded starches. Starch is primarily digested by pancreatic α-amylase and brush border disaccharide hydrolases in the small intestine. Chemically modified starch resists digestion in the small intestine, and starch with lower digestibility increases the amount of digesta in the distal gut( Reference Topping, Gooden and Brown 6 ). A large amount of digesta provides a favourable environment for microbial growth in the distal gut by providing nutrients to gut bacteria. Therefore, having a greater digesta mass may increase the diversity and population of gut bacteria and the production of SCFA.
The glucose and insulin response is strongly related to the rate of starch hydrolysis( Reference Holm, Lundquist and Björck 7 ); however, other factors such as incretins and glucagon-like peptide-1 (GLP-1) may be important( Reference Holst and Gromada 8 ). The incretins are hormones secreted from the gut in response to food and stimulate the secretion of insulin. Fermentation products, particularly SCFA, may be involved in a cascade of events that improve insulin sensitivity( Reference Maki, Pelkman and Finocchiaro 9 ).
It has been reported that HAMS lowers plasma cholesterol and TAG concentrations( Reference Liu, Kishida and Ebihara 10 , Reference Lopez, Levrat-Verny and Coudray 11 ) and influences the glycaemic and insulinaemic responses( Reference Behall and Hallfrisch 12 , Reference Britesa, Trigoa and Carrapiçob 13 ).
Hydroxypropylated tapioca starch retarded the development of insulin resistance in genetically diabetic KKay mice fed a normal fat diet( Reference Kato, Tachibe and Sugano 14 ) and a high-fat diet( Reference Tachibe, Kato and Sugano 15 ). So, hydroxypropylation of HAMS would reduce the digestibility and show many physiological effects through changes of energy, glucose and lipid metabolism and fermentation. However, the physiological effects of hydroxypropylated HAMS (HP-HAMS) have not been researched. Hydroxypropylation is an etherification process that is commonly used to modify food starch. Therefore, we investigated whether the physiological effects of HAMS on fermentation-dependent parameters such as caecal SCFA and caecal bacterial profile, glycaemic control and plasma and hepatic lipid concentrations are influenced by hydroxypropylation.
Materials and methods
Materials
Waxy maize starch (WMS) (about 100 % amylopectin, Matsunorin A60M; Matsutani Chemical Industry Co., Ltd.), HAMS (about 70 % amylose, HS-class 7, J-Oil Mills Inc.) and HP-HAMS were used in this study.
Hydroxypropylation of high-amylose maize starch
HAMS was hydroxypropylated according to a previously described procedure( Reference Tachibe, Kato and Nishibata 16 ). The hydroxypropyl content was determined by the method of Johnson( Reference Johnson 17 ). The degree of substitution for HP-HAMS was 0·21.
Animals and diets
Male Wistar rats (SLC) weighing 450–500 g for experiments 1 and 2 and 200–220 g for experiment 3 were housed individually in screen-bottomed, stainless-steel cages in a room maintained at 23 ± 1°C with a 12 h light:dark cycle (light on, 07.00–19.00 hours). The study was approved by the Laboratory Animal Care Committee of Ehime University. Rats were maintained in accordance with the Guidelines for the Care and Use of Laboratory Animals of Ehime University.
Experiment 1: apparent starch digestibility in the small intestine
After acclimatisation on the American Institute of Nutrition (AIN)-93G-based purified diet without cellulose for 7 d, the rats were subjected to ileorectomy in which the distal ileum was anastomosed to the rectum. The rats were not allowed food for 24 h before and after the operation. They received a daily intramuscular injection of 10 µl of Mycillin sol (containing procaine penicillin G (200 g/l) and dihydrostreptomycin sulphate (250 g/l); Toyo Jozo) for the first 3 d after surgery to prevent infection. They were then freely fed the AIN-93-based purified diet without cellulose for 10 d. A constant growth rate (5–7 g body weight gain/d) was achieved with this diet after 5 d.
After post-operative recovery, the rats were divided into three groups (n 7) and given the WMS, HAMS or HP-HAMS diet (Table 1) and water containing neomycin sulphate (0·1 g/100 ml; Wako Pure Chemical Industries, Co.) for 10 d to inhibit the action of the intestinal bacteria which remain after ileorectomy. The ileorectomy effluent was collected from each rat on the final 3 d of the experimental period. The ileorectomy effluent was freeze-dried, weighed and stored at −50°C until analysis. The amount of protein in the diet and effluent was determined by the Kjeldahl method with an N to protein conversion factor of 6·25. The amount of lipid in the diet and effluent was determined by the Soxhlet method. The amount of ash in the diet and effluent was determined by combustion in a muffle furnace (FM-26Yamato Scientific Co. Ltd.) at 550°C overnight. The amount of starch in the diet and effluent was determined by subtracting the weight of crude protein, lipid, cellulose, moisture and ash from the total weight. The amounts of WMS and HAMS can be determined by an enzymatic assay, but the amounts of HP-HAMS can not. Therefore, the apparent starch digestibility (%) was calculated as (A − B)/A × 100, where A is the starch intake and B is the starch output in the effluent as follows:
Table 1. Composition of experimental diets
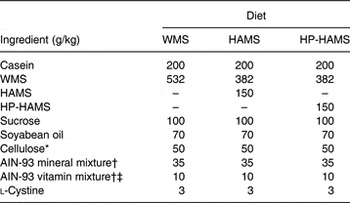
WMS, waxy maize starch; HAMS, high-amylose maize startch; HP-HAMS, hydroxypropylated HAMS.
* Cellulose powder, PC200 (Danisco Japan Ltd).
† Based on AIN-93G( Reference Reeves, Nielsen and Fahey 18 ).
‡ The vitamin mixture contained 20 g choline bitartrate per 100 g.
A = (food intake − cellulose) − sum of dietary protein, lipid, ash and water intake
B = (faecal dry weight − dietary cellulose intake) − sum of dietary protein, lipid and ash in faeces.
Experiment 2: measurement of hydrogen expired in rat breath
After acclimatisation on a commercial solid diet for 7 d, the rats were allowed free access to the AIN-93 diet without cellulose for 8 h per d from 09.00 to 17.00 hours for 2 weeks, and then for 4 h per d from 09.00 to 13.00 hours for 2 weeks. The rats were then divided into three groups (n 7) and given access to one of three kinds of test meal containing 70 g of soyabean oil, 130 g of sucrose and 800 g of test substance (WMS, HAMS or HP-HAMS) for 30 min.
Breath-test system
The system used for monitoring H2 in the air expired from the rats is shown in Fig. 1. In brief, the system was composed of an animal chamber with a volume of 9500 ml, a pump (Masterflex model 7524-50; Cole-Parmer Instrument Co. Ltd.) and a breath-sampling bag of 500 ml (Laboratory for Expiration Biochemistry Nourishment Metabolism Co., Ltd.). The air in the chamber was continuously aspirated during the experimental period. A desiccator was employed as the animal chamber so that the rats could move freely within the chamber and the expired air could be collected effectively in the breath-sampling bag.

Fig. 1. System used for monitoring hydrogen amount in the air expired from rats. The system comprised a desiccator that was used as an animal chamber, a pump and a breath-sampling bag. Aspiration of the expired air through the aspiration tube caused fresh air to be automatically drawn through a hole in the side of the desiccator.
Hydrogen-breath test
The rats were placed in the chamber immediately after access to the test meal for 30 min in the cage described previously. The expired air was collected at 30 min intervals for 12 h after the rats were placed in the chamber. The expired air was obtained by a single-pass (as opposed to re-breathing) method, in which air was passed through the chamber containing the rats at a flow rate of 100 ml/min. Aspiration of the expired air through the aspiration tube caused fresh air to be automatically drawn through a hole (2 cm in diameter), through which the aspiration tube also passed, on the side of the desiccator (Fig. 1). The air in the chamber was continuously aspirated during the experimental period. The aspirated air was discharged outside the breath-test system, except for the period during which the expired air was collected in the breath-sampling bag. The temperature and moisture in the chamber were checked every 30 min, and were maintained at 22 ± 2°C and 50 ± 10 %, respectively. The H2 concentration of each sample was determined by means of a breath gas analyser (BGA-1000D; Laboratory for Expiration Biochemistry Nourishment Metabolism Co., Ltd).
Experiment 3: effects of hydroxypropylation of high-amylose maize starch on digestion and fermentation-dependent parameters
After acclimatisation on a commercial solid diet for 7 d, the rats were randomly divided into three groups (n 6), and allowed free access to one of the three experimental diets (Table 1) for 4 weeks. Body weight and food intake were recorded daily for each rat in the morning before the food was replaced.
Sampling and analytical procedures
Before the rats were killed, faeces were collected from each rat for the final 3 d of the experimental period. The faeces were freeze-dried, weighed and milled.
At the end of the experiment, rats were anaesthetised by intraperitoneal injection of sodium pentobarbital (40 mg/kg body weight), a mid-line laparotomy was performed and the bile-pancreatic duct was exposed and ligated distally. The bile-pancreatic duct was then cannulated with a PE-10 polyethylene tube (Clay Adams), and bile-pancreatic juice was collected into a pre-weighed tube that had been cooled on ice for 30 min. The volume of bile-pancreatic juice was determined gravimetrically (1·0 ml = 1·0 g).
After the bile-pancreatic juice was collected, the rats were killed by decapitation and a blood sample was taken from the carotid artery into a blood collection tube (Vacutainer; Becton Dickinson) containing heparin as an anticoagulant. Plasma was separated by centrifugation at 1400 g at 4°C for 15 min, and stored at −50°C until analysis. After blood collection, the liver was immediately perfused with cold saline, removed, washed with cold saline, blotted dry on filter paper, weighed and stored at −50°C until analysis. After the liver had been removed, the small intestine except for the duodenum portion (the first 15 cm distal from the pylorus) was removed, flushed with ice-cold saline (9 g NaCl/l), everted, washed again and divided into two segments: an upper half (jejunum) and a lower half (ileum). The caecum was then removed and weighed, and the content of the caecum was transferred into a pre-weighed tube. Subsequently, the caecal wall was flushed clean with ice-cold saline, blotted dry on filter paper and weighed. The caecal content was homogenised under CO2.
Plasma and liver lipids
Plasma total cholesterol, TAG and glucose concentrations were assayed spectrophotometrically with commercially available kits (Cholesterol-E test Wako, TAG-E test Wako and Glucose-test Wako; Wako Pure Chemical Industries). The concentration of liver total lipids was determined gravimetrically after extraction by the method of Folch et al. ( Reference Folch, Lees and Sloane Stanley 19 ). Liver TAG and cholesterol concentrations were determined enzymatically, as previously described( Reference Ebihara, Shiraishi and Okuma 20 ).
Plasma glucose, insulin and active glucagon-like peptide-1 concentration
The plasma glucose concentration was measured spectrophotometrically with a commercially available kit (Glucose CII Test Wako; Wako Pure Chemical Industry). The plasma insulin concentration was measured by a sandwich ELISA method (Rat Insulin Kit, Morinaga Institute of Biological Science). The plasma active gut hormone GLP-1 was measured by using a commercially available ELISA kit (LINCO Research).
Amylase assay in bile-pancreatic juice
Amylase activity in the bile-pancreatic juice was determined by using a QuantiChrom α-Amylase Assay Kit (DAMY-100; BioAssay Systems). Crystalline porcine amylase (type I-A; Sigma Chemical) was used as a standard.
Digestive enzymes in the brush border membrane
The mucosa of the upper half (jejunum) and the lower half (ileum) was scraped from each segment with a microscopic glass microscope slide. The intestinal mucosa was homogenised in ten volumes (v/w) of ice-cold 10 mmol/l potassium phosphate buffer (pH 7·0). Maltase, isomaltase and sucrase activities were assayed as described by Dahlqvist( Reference Dahlqvist 21 ). The protein concentration was measured according to the method of Lowry et al. ( Reference Lowry, Rosebrough and Farr 22 ).
Caecal water content, pH and organic acids
The water content, pH and caecal organic acids (acetic, propionic, n-butyric, succinic and lactic acids) were measured by HPLC as described previously( Reference Ebihara, Shiraishi and Okuma 20 ).
IgA in the caecal content
The amount of IgA in the caecal content was determined by ELISA using a Rat IgA ELISA Quantitation Set (Bethyl Laboratories, Inc.).
Profile analysis of caecal microbiota by PCR-denaturing gradient gel electrophoresis
DNA was extracted from the caecal content using a faecal DNA isolation kit (TaKaRa Ex Taq Hot Start Version; Takara Bio Inc.) according to the manufacturer's instructions. DNA samples were used as a template to amplify fragments of the 16S rRNA gene with the universal primers HDA1-GC (5′-CGC CCG GGG CGC GCC CCG GGC GGG GCG GGG GCA CGG GGG GAC TCC TAC GGG AGG CAG CAG T-3′) and HDA2 (5′-GTA TTA CCG CGG CTG CTG GCA C-3′)( Reference Walter, Tannock and Tilsala-Timisjarvi 23 ). PCR was performed as described by Walter et al. ( Reference Walter, Tannock and Tilsala-Timisjarvi 23 ). Denaturing gradient gel electrophoresis (DGGE) was performed with a DCode universal mutation detection system (Bio-Rad) as described by Walter et al. ( Reference Walter, Tannock and Tilsala-Timisjarvi 23 ). DGGE profiles were compared with Quantity One version 4.6.0 software (Bio-Rad Laboratories), and similarities were expressed with Dice's similarity coefficient.
Statistical analysis
Data were analysed by one-way ANOVA, and significant differences among means were discriminated by the Tukey–Kramer test. When variances were not homogeneous, the Bartlett test( Reference Ichihara 24 ) was used and the data were transformed logarithmically before analysis by ANOVA, followed by multiple comparisons, or were analysed by the Steel–Dwass test. Differences were considered significant at P < 0·05. The Tukey–Kramer test and the Bartlett test were performed with StatView 5.0 software (SAS Institute), and the Steel–Dwass test and linear regression analyses were performed with the Excel Statistics program (version 6.0; Esumi).
Results
Experiment 1
The apparent digestibility of starch was significantly lower in the HAMS and HP-HAMS groups than in the WMS group; furthermore, the apparent digestibility of starch in the HP-HAMS group was significantly lower than in rats fed the HAMS diet (Fig. 2).

Fig. 2. Apparent digestibility of starch in rats fed the waxy maize starch (WMS), high-amylose maize starch (HAMS) or hydroxypropylated HAMS (HP-HAMS) diet. Values are means, with standard errors represented by vertical bars. a,b,c Mean values with unlike letters were significantly different (P < 0·05; Tukey-Kramer test).
Experiment 2
Food intake for 30 min was 3·7 (sem 0·1) g for rats fed WMS, 3·6 (sem 0·2) g for rats fed HAMS and 3·7 (sem 0·3) g for rats fed HP-HAMS. The excretion of H2 in breath was markedly increased in rats fed HAMS, reaching a peak at 4 h and then decreasing just a little, although a considerable amount of H2 was also excreted in breath at 12 h (Fig. 3). There was a more moderate increase in the excretion of H2 in breath after the ingestion of HP-HAMS, reaching a peak at 4 h and then decreasing towards the base value, which was reached after 8 h. The amount of H2 excreted in breath changed slightly after WMS feeding as compared with that at fasting. The incremental AUC for breath H2 expiration after HP-HAMS feeding was 75 % lower than that after HAMS feeding.

Fig. 3. Time course of hydrogen in air expired from rats (A) and incremental AUC (iAUC) (B) after ingestion of waxy maize starch (WMS), high-amylose maize starch (HAMS) or hydroxypropylated HAMS (HP-HAMS). Values are means, with standard errors represented by vertical bars. a,b,c Mean values with unlike letters were significantly different (P < 0·05; Tukey–Kramer test). -▲-, HAMS; -•-, HP-HAMS; -■-, WMS; ppm, parts per million.
Experiment 3
Body weight gain in the WMS group was significantly higher than that in the HAMS and HP-HAMS groups (Table 2). Food intake was significantly lower in the HAMS group than in the WMS group, but was not different from that in the HP-HAMS group. Liver weight, liver total lipid, liver and plasma cholesterol and plasma insulin concentrations were not affected by diet. Plasma TAG concentration in the HP-HAMS group was significantly lower than that in the WMS group, but was not different from that in the HAMS group. Plasma GLP-1 concentrations were significantly higher in the HAMS and HP-HAMS groups than in the WMS group. Plasma glucose concentration in the HP-HAMS group was significantly lower than in the HAMS group, but was not different from that in the WMS group.
Table 2. Body weight gain, food intake, liver weight, liver lipids, plasma lipids, plasma glucose, plasma insulin and plasma glucagon-like peptide-1 (GLP-1) in rats fed the waxy maize starch (WMS), high-amylose maize starch (HAMS) and hydroxypropylated high-amylose maize starch (HP-HAMS) diets*
(Mean values and pooled standard errors for six rats per group)

a,b Mean values within a row with unlike superscript letters were significantly different (P < 0·05).
* Data were analysed by one-way ANOVA, followed by the Tukey–Kramer test.
The amount of bile-pancreatic juice secreted in the HAMS and HP-HAMS groups was significantly higher than that in the WMS group (Table 3). The activities of amylase in bile-pancreatic juice, maltase and isomaltase in the jejunum and maltase, isomaltase and sucrase in the ileum were not affected by diet. Sucrase activity in the jejunum was significantly lower in the HAMS group than in the WMS group, but was not different from that in the HP-HAMS group.
Table 3. Secretion of bile-pancreatic juice, amylase activity of pancreatic juice, and activity of membrane digestive enzymes in rats fed the waxy maize starch (WMS), high-amylose maize starch (HAMS) and hydroxypropylated high-amylose maize starch (HP-HAMS) diets*
(Mean values and pooled standard errors for six rats per group)

a,b Mean values within a row with unlike superscript letters were significantly different (P < 0·05).
* Data were analysed by one-way ANOVA followed by the Tukey–Kramer test.
The wet weight of faeces excreted in the HP-HAMS group was significantly higher than that in the WMS and HAMS groups, but the number of faecal pellets was not affected by diet (Table 4). Faecal moisture was significantly higher in the HP-HAMS group than in the WMS group, but was not different from that in the HAMS group. The caecum wall weight of rats in the HAMS and HP-HAMS groups was significantly greater than that in the WMS group, and the caecum wall weight in the HP-HAMS group was significantly greater than that in the HAMS group. The wet weight of the caecal content and the moisture percentage of the caecal content were significantly greater in the HAMS and HP-HAMS groups than in the WMS group, but were not significantly different between the HAMS and HP-HAMS groups.
Table 4. Faecal output, caecal tissue weight and wet weight, pH, IgA and organic acids in the caecal contents of rats fed the waxy maize starch (WMS), high-amylose maize starch (HAMS) and hydroxypropylated high-amylose maize starch (HP-HAMS) diets*
(Mean values and pooled standard errors for six rats per group)
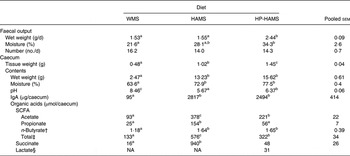
NA, not analysed statistically.
a,b,c Mean values within a row with unlike superscript letters were significantly different (P < 0·05).
* Data were analysed by one-way ANOVA, followed by the Tukey–Kramer test.
† Data for butyrate were transformed logarithmically before analysis. n-Butyrate was expressed as the log concentration.
‡ Total (μmol/caecum) = acetate (μmol/caecum) + propionic (μmol/caecum) + n-butyrate (μmol/caecum).
§ Lactate was detected in three of the six rats fed the WMS diet (3·6–5·3 µmol/caecum) and in one of the six rats fed the HAMS diet (479 µmol/caecum).
The amount of acetic acid and total SCFA in the caecal content were significantly greater in the HAMS and HP-HAMS groups than those in the WMS group, and were significantly greater in the HAMS group than those in the HP-HAMS group. The amount of propionic and succinic acids in the caecal content was significantly greater in the HAMS group than that in the WMS and HP-HAMS groups, but was not significantly different between the WMS and HP-HAMS groups. The amount of n-butyric acid in the caecal content was significantly greater in the HAMS and HP-HAMS groups than in the WMS group, but did not differ significantly between the HAMS and HP-HAMS groups.
The pH of the caecal content was significantly lower in the HAMS and HP-HAMS groups than that in the WMS group, and was significantly lower in the HAMS group than in the HP-HAMS group. The amount of IgA in the caecal content was significantly higher in the HAMS and HP-HAMS groups than that in the WMS group, but did not differ significantly between the HAMS and HP-HAMS groups. Linear regression analysis showed that the caecal amount of IgA positively correlated with the caecal amount of acetic acid (r 0·696), propionic acid (r 0·644), n-butyric acid (r 0·716), SCFA (acetic + propionic + n-butyric acids; r 0·710) and succinic acid (r 0·555).
The DGGE patterns of the three dietary groups (six samples for rats fed the WMS and HP-HAMS diets, and five samples for rats fed the HAMS diet) are shown in Fig. 4(A). Bacterial diversity was divided into three distinct groups. Rats fed the WMS and HP-HAMS diets formed a group with high similarity (Dice's similarity coefficient, 78 %) (Fig. 4(B)). Rats fed the HAMS diet were segregated from those fed the WMS and HP-HAMS diets with low similarity (Dice's similarity coefficient, 31 %).

Fig. 4. PCR-denaturing gradient gel electrophoresis (DGGE) analysis of the caecal microbiota, based on 16S rRNA gene sequences in rats fed the waxy maize starch (WMS), high-amylose maize starch (HAMS) or hydroxypropylated HAMS (HP-HAMS) diet for 4 weeks. (A) DGGE gel image stained with SYBR green. (B) DGGE banding patterns were analysed using FPQuest software (Bio-Rad). Similarity was visualised by dendograms using the unweighted pair group method using the arithmetic averages (UPGMA).
Discussion
The digestibility of HAMS in the small intestine was significantly decreased by hydroxypropylation. Although all of the three hydroxyl groups (at 2-O, 3-O and 6-O) are potentially available for substitution, the hydroxypropyl groups are predominantly located at position 2-O in the glucose units( Reference Xu and Seib 25 ). Hydroxypropyl groups at position 2-O sterically hinder the enzymatic hydrolysis of neighbouring glycoside bonds in hydroxypropylated starch( Reference Richardson, Nilsson and Bergquit 26 ). Therefore, the decreased digestibility of HP-HAMS would be mainly due to enzyme inhibition by hydroxypropyl groups at position 2-O.
The digestibilities of cellulose, hemicellulose and pectin for ileosotmised human subjects were 16, 17 and 15 %, respectively( Reference Holloway and Tasman-Jones 27 – Reference Holloway and Tasman-Jones 29 ). The digestibility of HP-HAMS was 82 %, which shows that the digestibility of HP-HAMS is higher than that of cellulose.
Body weight gain was significantly lower in the HP-HAMS group than in the WMS group, while food intake was not. The structural modification of starch might decrease its digestibility, and hydroxypropylation has been shown to decrease the susceptibility of starch to digestive enzymes( Reference Hoover, Hannouz and Sosulski 30 , Reference Wootton and Chaudhry 31 ). The small intestinal digestibility of starch was significantly lower in the HP-HAMS group than in the HAMS group, as calculated from the recovery of starch from effluent in neomycin-treated ileorectomised rats. Ingesting less energy is the major determinant towards weight loss. Therefore, the decreased body weight gain observed in the HP-HAMS group might be due to the lower digestibility of HP-HAMS.
HAMS reduces plasma TAG concentrations in rats( Reference Liu, Kishida and Ebihara 10 , Reference Goda, Urakawa and Watanabe 32 ). Propionate inhibits fatty acids synthesis( Reference Lin, Vonk and Slooff 33 , Reference Wright, Anderson and Briges 34 ) and decreases the concentration of fatty acids synthesis mRNA in cultured hepatocytes( Reference Cheng and Lai 35 ). Propionate reduces serum and hepatic cholesterol amounts in rats( Reference Chen, Anderson and Jennings 36 ). In the present study, the amount of propionic acid in the caecal content was significantly higher in the HAMS group than in the WMS and HP-HAMS groups. The concentration of TAG in the liver was significantly lower in the HAMS group than in the WMS group. Therefore, feeding a diet rich in HAMS might reduce lipogenesis in the liver. However, the concentration of plasma TAG in the HAMS group was not lower than that in the WMS group. Conversely, the concentration of plasma TAG was significantly lower in the HP-HAMS group than in the WMS group, but the concentration of TAG in the liver was not. The concentration of plasma TAG is also controlled by the amount of TAG absorbed from the small intestine. Hydroxypropyl groups are hydrophilic in nature, and the solubility, viscosity and swelling power of starch are increased after hydroxypropylation( Reference Liu, Ramsden and Corke 37 ). Hydroxypropylation also decreases the temperature at peak viscosity( Reference Takahashi, Suzuki and Yoshida 38 ). Moreover, the addition of sucrose increased in viscosity( Reference Takahashi, Suzuki and Yoshida 38 ). As a result, the digestion of HP-HAMS may be lower than that of WMS. The slower digestion of HP-HAMS might in turn make the digestion of TAG slow, which might result in a lower concentration of plasma TAG in the HP-HAMS group.
Faecal wet weight in the HP-HAMS group was higher than that in the WMS group, but did not differ significantly from that in the HAMS group. Faecal weight was found to be significantly higher in rats fed a diet with over 20 % HAMS than in those fed a normal maize starch diet( Reference Lopez, Levrat-Verny and Coudray 11 , Reference Kishida, Nogami and Ogawa 39 ). The amount of HAMS in the diets used in this study was 150 g per 1 kg diet. The moisture content of the faeces in the HP-HAMS group was greater than that in the HAMS group, suggesting that HP-HAMS might possess water-holding properties. Hydroxypropylated waxy rice and maize starches have a higher water-holding capacity than unmodified starches( Reference Han, Lee and Lim 40 ). The introduction of hydroxypropyl groups has been found to increase the water-holding capacity of all starches( Reference Onofre and Wang 41 ).
An increase in caecal tissue weight in rats ingesting carbohydrates of low digestibility has been reported by various authors. Dietary HAMS and HP-HAMS increase the wet weight of the caecal content. Maldigested materials reaching the caecum are potential sources for intestinal bacteria, resulting in the production of SCFA. Here, an increase in caecal tissue weight was observed in rats fed the HAMS and HP-HAMS diets. This hypertrophic effect is mediated by butyrate, because butyrate has been recognised as the main source of energy for the caecal mucosa( Reference Le Blay, Michel and Blottière 42 ). On the other hand, Oku et al. ( Reference Oku, Konishi and Hosoya 43 ) speculated that caecal enlargement depends on the amount of maldigested materials reaching the caecum. As a result, the increase in the caecal tissue weight in the HAMS and HP-HAMS groups appears to depend on a greater caecal content and a higher concentration of butyrate.
The increased propionate concentration measured in samples from the HAMS group was unexpected because dietary RS is reported to increase butyrate production, although propionate can be produced in preference to butyrate( Reference Topping and Clifton 44 ). Succinic acid is converted to propionic acid by intestinal bacteria ( Reference Johns 45 ). In the caecal content, the amount of propionic acid increased with the amount of succinic acid (r 0·911, P < 0·001). On the basis of PCR-DGGE profiles, the similarity between the HAMS and HP-HAMS groups was low (Dice's similarity coefficient, 31 %). Therefore, the unexpected amount of propionic acid in the caecal content might be due to changes in the microbiota in the caecum.
The amount of caecal SCFA and the pH of the caecal content in the HP-HAMS group were significantly lower and higher, respectively, than those in the HAMS group. When undigested carbohydrates reach the large intestine, the intestinal bacteria that normally live in the large intestine break them down. This breakdown of carbohydrates by intestinal bacteria produces SCFA and H2. H2 is produced in the body only by intestinal bacteria. H2 excretion in breath in the HP-HAMS group was about 25 % of that in the HAMS group, suggesting that HP-HAMS is broken down by intestinal bacteria to a lesser extent than HAMS.
Organic acids may contribute to intestinal immune and barrier functions( Reference Ishizuka, Tanaka and Xu 46 ). Here, large amounts of caecal organic acids and caecal IgA were manifested in rats fed the HAMS and HP-HAMS diets. The amount of caecal IgA positively correlated with the amount of caecal acetic acid, propionic acid, n-butyric acid and total SCFA and positively with caecal pH. The amount of caecal IgA correlates with the caecal amount of lactic acid( Reference Ito, Wada and Ohguchi 47 – Reference Ito, Tanabe and Kawagishi 49 ), propionic acid( Reference Ito, Wada and Ohguchi 47 ), n-butyric acid( Reference Ito, Wada and Ohguchi 47 ) and total SCFA( Reference Ito, Wada and Ohguchi 47 ). Higher production of butyrate in the rat caecum results in more immune cells in the gut epithelial layer cells( Reference Sung, Jeong and Choi 50 ). In this study, the caecal amount of IgA correlated with the amount of succinic acid in the caecal content, in contrast with the findings of Ito et al. ( Reference Ito, Wada and Ohguchi 47 – Reference Ito, Tanabe and Kawagishi 49 ). However, the amount of caecal IgA positively correlated with the pH of the caecal content, which is in agreement with the result of Sung et al. ( Reference Sung, Jeong and Choi 50 ). Therefore, it appears that SCFA production was connected with the IgA production.
HAMS can reduce insulin resistance( Reference Maki, Pelkman and Finocchiaro 9 , Reference Robertson, Bickerton and Dennis 51 ), although the exact mechanism underlying improved insulin sensitivity has not been elucidated. One hypothesis is that SCFA produced by the fermentation of RS in the large intestine may lead to improved insulin sensitivity( Reference Maki, Pelkman and Finocchiaro 9 ). SCFA triggers secretion of GLP-1 from mixed colonic cultures in vitro ( Reference Tolhurst, Heffron and Lam 52 ). GLP-1 improves insulin sensitivity in human subjects and rodents( Reference Parlevliet, de Leeuw van Weenen and Romijn 53 ).
Food intake, amylase and digestive enzymes in brush border membrane activities and plasma insulin and gastric inhibitory peptide concentrations were not significantly different between the HAMS group and the HP-HAMS group. However, the plasma glucose concentration of the HP-HAMS group was significantly lower than that of the HAMS group. HP-HAMS might interfere with the digestion and absorption of starch and sucrose in the diet as the viscosity of starch increases after hydroxypropylation.
The digestibility of carbohydrate in ileorectomised rats fed HAMS and HP-HAMS was about 80 %, which suggests that about 20 % of ingested carbohydrate reach the ileum. Under normal physiological situations, undigested nutrients can reach the ileum and induce activation of the so-called ‘ileal brake’. The ileal brake is a neurohormonal feedback mechanism that delays gastric and intestinal transit time and reduces food intake in response of unabsorbed nutrients in the distal ileum. Carbohydrate is a significant stimulus for the ileal brake mechanism( Reference Spiller, Trotman and Adrian 54 ). Activation of the ileal brake is associated with the secretion of gut peptides, such as peptide YY (PYY) and GLP-1( Reference Strader 55 ). A high intake of amylose-resistant maize starch in rodents has also been found to consistently increase GLP-1 and peptide YY( Reference Keenan, Zhou and McCutcheon 56 , Reference Prigeon, Quddusi and Paty 57 ). GLP-1 is an intestinal hormone that has potent effects on glucose-mediated insulin secretion, insulin gene expression, and β-cell growth and differentiation( Reference Kjems, Holst and Vølund 58 ). The plasma GLP-1 concentration was significantly higher in the HAMS and HP-HAMS groups than in the WMS group; however, the plasma insulin concentration was not affected by diet. GLP-1 secretion is dependent on the presence of nutrients in the distal intestine. HAMS and HP-HAMS might stimulate the secretion of GLP-1 by increasing the delivery of nutrients to the distal intestine. GLP-1 acts to reduce food intake( Reference Zhang and Ritter 59 ). In the present study, food intake decreased as the concentration of GLP-1 increased (r −0·685, P < 0·002).
Species composition of the microbiota and substrate availability largely determine the amount and types of SCFA produced( Reference Macfarlane and Macfarlane 60 ). On the basis of the PCR-DGGE profiles, the similarity in caecal bacteria population of the HAMS group and HP-HAMS group was 31 %, which indicates that the caecal bacteria population was markedly changed by hydroxypropylation of HAMS. On the other hand, the similarity of caecal bacteria population of the WMS group and HP-HAMS group was 78 %, which indicates that the microbial population of the HP-HAMS group was similar to that of the WMS group. In ileorectomised rats, the apparent digestibility of HAMS was significantly decreased by hydroxypropylation. However, the amount of total SCFA and succinic acid in the caecal content of rats fed the HP-HAMS diet decreased to about 1/2 and 1/20, respectively, of that detected in rats fed the HAMS diet. Therefore, the part of HP-HAMS that escaped digestion in the small intestine was hardly utilised by caecal bacteria and did not affect the bacterial population.
In conclusion, digestibility of HAMS in the small intestine and its fermentability in the caecum of rats were significantly decreased by hydroxypropylation, and the microbial population of the caecal content was markedly changed. However, the microbial population of the caecal content of rats fed HP-HAMS was similar to that of rats fed WMS. Food intake, plasma and hepatic cholesterol and TAG concentrations, plasma GLP-1 concentration, amylase activity in bile-pancreatic juice and the activity of membrane digestive enzymes were not affected by hydroxypropylation of HAMS. Thus, hydroxypropylation of HAMS changes digestion and fermentation-dependent parameters, but does not affect glucose and lipid metabolism.
Acknowledgements
This work was supported by a research grant from the Japanese Ministry of Education, Science and Culture (16580101). K. E. designed the study and wrote the manuscript. M. T. and N. K. planned the study, summarised the results of the study and discussed with the other researchers the results of the study. T. K. provided advice on all aspects of the study.
None of the authors has any conflicts of interest.