Introduction
Off-target movement occurrences of synthetic auxin herbicides have been identified as a major concern throughout agriculture in the mid-southern United States (Riar et al. Reference Riar, Norsworthy, Steckel, Stephenson, Eubank and Scott2013). Off-target movement of herbicides can occur primarily by physical drift or vapor drift from the volatilization of an herbicide. Of the causes of herbicide off-target movement, physical drift is believed to be the most preventable. According to a 2019 survey of herbicide applications in Arkansas, more than half (51%) of the applications were aerially applied, with herbicide drift highlighted as a primary concern among survey respondents (Butts et al. Reference Butts, Barber, Norsworthy and Davis2021). Applying florpyrauxifen-benzyl aerially as a spray has been shown to have increased drift potential compared to when the herbicide is sprayed using ground equipment (Butts et al. Reference Butts, Fritz, Kouame, Norsworthy, Barber, Ross, Lorenz, Thrash, Bateman and Adamczyk2022). Herbicide off-target movement can also be influenced by many other factors. For example, temperature, wind, rainfall, and herbicide formulation have all been reported to affect the off-target movement of dicamba (Behrens and Lueschen Reference Behrens and Lueschen1979; Soltani et al. Reference Soltani, Oliveira, Alves, Werle, Norsworthy, Sprague, Young, Reynolds, Brown and Sikkema2020).
Florpyrauxifen-benzyl is a rice herbicide that was commercially launched as Loyant® (Corteva Agriscience, Wilmington, DE, USA) in 2018 in the mid-southern United States. Florpyrauxifen-benzyl is a synthetic auxin, Herbicide Resistance Action Committee (HRAC)/Weed Science Society of America (WSSA) Group 4 herbicide that is labeled for application in rice from early postemergence to 60 d but is recommended for preflood applications at 30 g ai ha−1 (Anonymous 2023). Florpyrauxifen-benzyl offers broad-spectrum control of grass, broadleaf, and sedge species and provides an advantage of a lower use rate compared to other common HRAC/WSSA Groups 1, 3, 4, and 5 rice herbicides (Anonymous 2019; Miller and Norsworthy Reference Miller and Norsworthy2018a). However, recent research indicated that coating florpyrauxifen-benzyl on urea reduced barnyardgrass [Echinochloa crus-galli (L.) P. Beauv.] control efficacy compared to when the herbicide was applied as a foliar spray (Cotter et al. Reference Cotter, Norsworthy, Butts, Roberts and Mauromoustakos2024). Commercialization of florpyrauxifen-benzyl was followed by variable barnyardgrass control, varying rice cultivar tolerance, and off-target movement of the herbicide to soybean, which led to a rapid decrease in use of the herbicide, especially in rice fields near soybean fields (J. Hardke, personal communication, 2020). Soybean and rice represent the top two agronomic crops planted and harvested in Arkansas (USDA-NASS 2023). Rice commonly follows soybean in a cropping rotation, causing the two crops to be grown near each other (Hardke Reference Hardke2021).
Following evaluations of multiple crops—soybean, cotton (Gossypium hirsutum L.), corn (Zea mays L.), grain sorghum [Sorghum bicolor (L.) Moench ssp. bicolor], and sunflower (Helianthus annuus L.)—in response to low rates of florpyrauxifen-benzyl, soybean exhibited the greatest sensitivity to the herbicide (Miller and Norsworthy Reference Miller and Norsworthy2018c). Additionally, soybean expressed slightly greater injury and growth reductions from less-than-labeled rate applications of florpyrauxifen-benzyl compared to dicamba at the same rates (Miller and Norsworthy Reference Miller and Norsworthy2018c). However, florpyrauxifen-benzyl is not the first herbicide to easily injure soybean from off-target movement. Following the first year dicamba could be legally applied as a foliar spray to dicamba-tolerant soybean and cotton in 2017, Arkansas reported the highest number of dicamba off-target movement complaints nationwide, at 986 complaints (Bish et al. Reference Bish, Oseland and Bradley2021). However, off-target movement of dicamba can be characterized by vapor drift via volatilization, whereas florpyrauxifen-benzyl is unlikely to cause serious soybean injury from volatilization, as it is considered a low-volatile compound with a vapor pressure of 4.6 × 10–5 at 25 C (APVMA 2018; Behrens and Lueschen Reference Behrens and Lueschen1979; Bish et al. Reference Bish, Farrell, Lerch and Bradley2019; Egan and Mortensen Reference Egan and Mortensen2012). Furthermore, florpyrauxifen-benzyl is not likely to translocate in plants below the treated area and has limited root uptake (Anonymous 2019; Miller and Norsworthy Reference Miller and Norsworthy2018b). Hence foliar interception of florpyrauxifen-benzyl is the main point of concern following off-target movement of the herbicide.
With the off-target movement of florpyrauxifen-benzyl being a main concern when applying the herbicide, an alternative method was warranted to aid in safer applications of the herbicide and reduce physical drift onto susceptible crops. The evolution of application equipment and its improved utilization have led to alternative herbicide application methods, such as shielded and directed sprayers, to reduce crop injury (Davis and Pradolin Reference Davis and Pradolin2016; Sumner and Culpepper Reference Sumner and Culpepper2017). Additionally, coating herbicides onto fertilizers as an alternative application method has been utilized. Starches have been utilized to entrap herbicides on urea to decrease crop injury and reduce herbicide volatilization (Shasha and Trimnell Reference Shasha and Trimnell1989). Fine water particles are considered to have the highest drift potential, and urea granules can reduce that drift potential due to a greater downward terminal velocity than fine water particles (Hofstee Reference Hofstee1992). Previous research showed that coating herbicides on fertilizers allowed for better crop canopy and residue penetration in conservation cropping systems (Kells and Meggit Reference Kells and Meggit1985). Florpyrauxifen-benzyl is currently labeled and recommended to be coated at 30 g ai ha−1 onto a minimum of 112 kg ha−1 of urea (Anonymous 2021). Therefore urea granules may have the potential to be utilized as a more effective herbicide carrier than water, as granules are larger in diameter and density than spray droplets when utilized at a preflood application timing to help ensure granule-to-soil contact. However, limited research has been conducted to determine the effectiveness of this application method in decreasing off-target florpyrauxifen-benzyl movement and soybean injury.
Because of the broad-spectrum activity of florpyrauxifen-benzyl, an effective alternative application method is warranted to help ensure safe applications of the herbicide in unique cropping scenarios. Coating florpyrauxifen-benzyl on urea can lower the risk of off-target movement by reducing foliar interception while still utilizing the herbicide for rice weed control after floodwater has been established. Hence research is needed to determine whether coating florpyrauxifen-benzyl on urea decreases soybean injury compared to spray applications and if coating the herbicide on urea increases the likelihood of volatilization.
Materials and Methods
General Methodology of Low-Dose Florpyrauxifen-benzyl Exposure to Soybean
Two separate experiments were conducted at the Milo J. Shult Research and Extension Center in Fayetteville, AR (36.094°N, 94.173°W), in 2020 and 2021, to compare soybean sensitivity to low rates of florpyrauxifen-benzyl spray-applied and coated on urea in narrow- and wide-row soybean systems. Soil texture in both years was a Leaf silty clay loam consisting of 17.1% sand, 74.2% silt, and 8.7% clay with 1.8% organic matter and pH 6.6. Plot sizes were 6.1 × 1.8 m with a 1.5-m alley between plots. Seedbeds were prepared using conventional tillage, and soybean was flat-planted using a drill with 15-cm row spacing for narrow-row soybean. For the wide-row soybean treatment, soybean was planted with a tractor-mounted John Deere 7200 MaxEmerge (Deere & Company, Moline, IL, USA) four-row planter on 0.9-m beds. All soybean was planted at 358,000 seeds ha−1 at a 2-cm depth. The previous crop was grain sorghum in both years.
A glufosinate-resistant soybean (LibertyLink®, BASF, Florham Park, NJ, USA) cultivar was planted in both years; ‘4410GTLL’ was planted on June 11, 2020, and ‘4539GTLL’ was planted on June 25, 2021. No more than two applications of glufosinate (Liberty®, BASF) postemergence at 655 g ai ha−1, pyroxasulfone (Zidua® WG) preemergence at 180 g ai ha−1, S-metolachlor (Dual II Magnum®, Syngenta Crop Protection, Greensboro, NC, USA) at 1,070 g ai ha−1 following soybean emergence, cloransulam-methyl (FirstRate®, Corteva Agriscience) at 35 g ai ha−1 after soybean reached V1, and glyphosate (Roundup PowerMAX® 3, Bayer Crop Science, St. Louis, MO, USA) postemergence at 1,260 g ai ha−1 were utilized until the beginning of soybean reproductive stages to maintain weed-free plots, along with occasional hand weeding when necessary. Soybean management practices typical for the region, including planting, tillage, fertilization, irrigation, and pest control, were utilized along with the use of overhead irrigation (UADA-SCC 2014). Previously described plot sizes represented the treated area, and an additional soybean row was established on both sides of each plot to serve as an application buffer within the wide-row system. Approximately 1 m of bare ground was utilized between narrow-row soybean plots as a buffer zone.
The experiment was conducted as a randomized complete-block design, two-factor treatment structure, with four replicates. Factor A included florpyrauxifen-benzyl rates at 0.18, 0.35, 0.70, 1.41, 2.81, and 5.63 g ai ha−1; factor B application method was spray-applied and coated on urea. Florpyrauxifen-benzyl at 30 g ai ha−1 was coated onto 317 kg ha−1 of urea using an electric motor–driven mixer in batches of 23 kg. Florpyrauxifen-benzyl and a blue dye were sprayed onto the urea and mixed continuously for 5 min to ensure an even dispersion of herbicide and to provide sufficient time for adhesion of the herbicide to the urea surface. After mixing, florpyrauxifen-benzyl-coated urea was weighed to equate the previously mentioned rates where each respective treatment received the amount of urea as directed by the respective herbicide rate. Additionally, noncoated urea was applied to spray-applied plots at equivalent rates for the respective herbicide-coated urea rate structure immediately before herbicide applications. Nontreated check plots received no urea fertilizer. All spray applications of florpyrauxifen-benzyl included methylated seed oil at 0.58 L ha−1 and were applied using a CO2-pressurized backpack sprayer calibrated to deliver 140 L ha−1 at 276 kPa with a handheld boom containing four AIXR 110015 nozzles (TeeJet® Technologies, Springfield, IL, USA) spaced 48 cm apart. All florpyrauxifen-benzyl coated on urea treatments were applied using a handheld fertilizer spreader. All florpyrauxifen-benzyl applications occurred at the V3 soybean growth stage on July 20, 2020, and July 23, 2021. If a rainfall event had not occurred within 24 h after application, 1.3 cm of irrigation water was applied using an overhead lateral irrigation system.
Visible estimates of soybean injury were rated at 3 wk after treatment (WAT) on a scale of 0 to 100, where 0 represents no crop injury and 100 represents crop death (Frans and Talbert Reference Frans and Talbert1986). Soybean injury ratings were based on aboveground biomass, stunting, leaf malformations, stem epinasty, and groundcover. Aerial images of all plots were taken on the days that the injury was rated using a DJI Phantom Pro 4 (DJI, Shenzhen, China) in 2020 and a DJI Mavic Pro 2 in 2021. From these aerial images, soybean groundcover was determined for each plot using FieldAnalyzer and made relative to nontreated plots. Additionally, 15 individual plants were collected, at crop maturity, from each plot as subsamples to record yield components: seeds per plant, pods per plant, seeds per pod, and 100-seed weight. Grain yield was determined at maturity by harvesting the two treated rows within each plot using a small-plot combine. Visible estimates of relative groundcover at 3 WAT determined a gamma distribution. All yield components were determined to follow a normal distribution based on corrected Akaike information criterion (AICc) and Bayesian information criterion (BIC) values in the distribution platform of JMP 16 (SAS Institute, Cary, NC, USA). A two-factor analysis of variance (ANOVA) was used in SAS 9.4 utilizing the PROC GLIMMIX procedure. Means were separated using Tukey’s honestly significant difference (HSD) (α = 0.05). Block and year were considered random effects with block nested within year. By considering blocks a random effect, blocks are assumed to be uncorrelated with the individual main effects.
Regression analysis was utilized to identify relationships between soybean injury and herbicide rate and soybean injury and relative yield. To model narrow- and wide-row soybean injury at 3 WAT by florpyrauxifen-benzyl rate, a Weibull growth model (Equation 1) was fit to the data and used to explain the relationship:

where a is asymptote, b is inflection point, c is growth rate, and x is herbicide rate.
Additionally, the relationship between narrow- and wide-row soybean injury at 3 WAT and relative yield was determined by a logistic 3P model (Equation 2) when florpyrauxifen-benzyl was spray-applied:
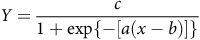
where a is growth rate, b is inflection point, c is asymptote, and x is soybean injury at 3 WAT.
Florpyrauxifen-benzyl Coated on Urea Volatility Potential
A low-tunnel field experiment was conducted in 2020 and 2021 at the Milo J. Shult Research and Extension Center in Fayetteville, AR, to determine if coating florpyrauxifen-benzyl on urea increases the likelihood of volatilization. The soil texture in both years was a Leaf silty clay loam consisting of 22.2% sand, 59.0% silt, and 18.8% clay with 1.8% organic matter and pH 6.4. Glufosinate-resistant soybean (LibertyLink®) cultivars ‘4410GTLL’ and ‘4918LL’ were planted on July 3, 2020, and June 24, 2021, respectively. Soybean was planted similarly to the previously mentioned wide-row soybean experiment and served as a florpyrauxifen-benzyl/dicamba-sensitive bioindicator for qualitative assessments.
The experiment was conducted as a single-factor randomized complete-block design with four replicates. Treatments included florpyrauxifen-benzyl coated on urea at 120 g ai ha−1 and applied to (1) field-dried, (2) saturated, and (3) field-dried soil that was flooded immediately following application. Additional herbicide treatments included spray-applied florpyrauxifen-benzyl at 120 g ai ha−1 onto saturated soil and a mixture of dicamba (Xtendimax®, Bayer Crop Science) at 2,240 g ae ha−1 and glyphosate (Roundup PowerMAX® 3) at 5,040 g ae ha−1 spray-applied to saturated soil to serve as a known volatility standard. Previously mentioned rates represented 4X the respective label rates to represent the total soil surface area within the low tunnels. Prior to application, florpyrauxifen-benzyl at 30 g ai ha−1 was coated onto urea at 317 kg ha−1 using an electric motor–driven mixer to serve as a 1X rate of florpyrauxifen-benzyl and urea mixture. Additionally, the soil was collected from the top 5 cm of the soil profile at the experiment location, sieved through a 10-mm sieve, filled into two plastic flats measuring 38 × 48 × 5.5 cm, and served as the treated area within each plot (Oseland et al. Reference Oseland, Bish, Steckel and Bradley2020). A similar methodology has been used previously to assess the volatility of the auxin herbicide dicamba (Castner et al. Reference Castner, Norsworthy and Roberts2022; Zaccaro-Gruener et al. Reference Zaccaro-Gruener, Norsworthy, Piveta, Barber, Mauromoustakos, Mueller and Roberts2023). Glyphosate was added to the mixture as an herbicide known to increase the volatility potential of dicamba (Mueller and Steckel Reference Mueller and Steckel2019a, Reference Mueller and Steckel2019b). Trays of soil were treated approximately 1 km from the test site, then taken to the test site and immediately placed within the tunnels following treatment. Applications occurred at the soybean V3 growth stage, as soybean are highly sensitive and more likely to elicit auxin symptomology at this stage (Jones et al. Reference Jones, Norsworthy, Barber, Gbur and Kruger2019).
Polyvinyl chloride (PVC) low tunnels were constructed to cover two bioindicator soybean rows in the plot area measuring 1.5 m wide, 6 m long, and 1.2 m tall. Each section of the low tunnel was bent to conform to the specified dimensions, and five bent PVC tube sections were connected using four additional 1.25-cm-diameter PVC tubing sections measuring 1.5 m in length to reach the desired total low-tunnel length of 6 m. Once PVC low-tunnel pieces were assembled and placed over the two soybean bioindicator rows, the PVC frame was covered with 1.5 mil painter’s plastic. Each tunnel end was open, and excess plastic was secured on each frame using plastic clamps. Excess plastic along the edges was secured using soil from outside of the plot area and with metal tent stakes. Tunnels were placed in the field with a minimum of 6 m separating replicates and 2 m between tunnels within the same replication (Castner et al. Reference Castner, Norsworthy and Roberts2022; Zaccaro-Gruener et al. Reference Zaccaro-Gruener, Norsworthy, Piveta, Barber, Mauromoustakos, Mueller and Roberts2023).
Immediately before herbicide-treated flats were placed in the covered tunnels, a single air sampler (Hi-Q Environmental Products Company, San Diego, CA, USA) that filters air at 185 L min−1 was placed in the center of each tunnel between the two bioindicator soybean rows. Air samplers were generator powered (American Honda Motor Company, Torrance, CA, USA) and ran continuously for 48 h after all air samplers and treated flats were placed inside covered tunnels. Each air sampler captured herbicide on a glass fiber filter paper that measured 102 mm in diameter. Following in succession to the glass fiber filter paper, air filtered through cylindric polyurethane foam filters (PUF) measuring 6 × 7.6 cm (Restek Corporation, Bellefonte, PA, USA). After 48 h, all tunnels, flats, and air samplers were removed from the field.
Following termination of the 48-h sampling period, the PUF and filter paper from dicamba-containing treatments were collected, separately labeled, and immediately placed into coolers containing dry ice at −20 C and shipped to the Mississippi State Chemical Laboratory in Starkville for dicamba residue analysis (Riter et al. Reference Riter, Sall, Pai, Beachum and Orr2020; Soltani et al. Reference Soltani, Oliveira, Alves, Werle, Norsworthy, Sprague, Young, Reynolds, Brown and Sikkema2020). The following dicamba acid extraction and analysis methods are pursuant to those of Castner et al. (Reference Castner, Norsworthy and Roberts2022). Dicamba acid (221.04 g mol−1) was extracted from each PUF and filter paper with 30 ml of methanol, which contained 13C6-labeled dicamba (Sigma Aldrich, St. Louis, MO, USA) as an internal standard. All PUF samples were homogenized with a SPEX SamplePrep Geno/Grinder® (OPS-Diagnostics, Lebanon, NJ, USA). The supernatant was concentrated with a TurboVap to 1 ml, then filtered, evaporated, and solvent-exchanged to an appropriate volume of 25% acetonitrile in a water solution, so the samples were at a 50-fold concentration. For quality control, each sample included a blank matrix sample with no dicamba present and a spiked matrix sample that included a known concentration of dicamba. The dicamba-spiked matrix sample determined extraction efficiency for each sample, with a mean accuracy range of 70% to 120%. The limit of dicamba detection for PUFs and filters was 10 ng PUF−1 or filter paper−1.
Dicamba concentrated in PUFs and filter paper was quantitated using an Agilent 1290 liquid chromatograph combined with an Agilent 6460 C triple quadrupole mass spectrometer (Agilent Technologies, Santa Clara, CA, USA) (Monsanto Company Method; Soltani et al. Reference Soltani, Oliveira, Alves, Werle, Norsworthy, Sprague, Young, Reynolds, Brown and Sikkema2020). Chromatographic separation was performed using an Agilent Zorbax Eclipse Plus 100-mm column with a run time of 8 min and a 3-min post-run. The mobile phases utilized 0.1% formic acid in water for the aqueous phase (solvent A) and 0.1% formic acid in acetonitrile for the organic phase (solvent B). The flow rate was 0.3 ml min−1 with the subsequent solvent gradient system: 0 to 0.5 min of 25% B, 0.5 to 1 min of 50% B, and 1 to 4 min of 60% B. Ionization of dicamba was performed using electrospray ionization in negative mode with an auxiliary gas (N2), source temperature of 200 C, and a gas flow rate of 10 L min−1.
Following the same sampling termination period previously mentioned, PUF and filter papers from florpyrauxifen-benzyl treatments were collected, labeled, and immediately placed into coolers containing dry ice at −20 C and shipped to Corteva Agriscience (Indianapolis, IN, USA) for analysis of florpyrauxifen-benzyl residue. For PUF extraction and analysis, each PUF was placed into a 500-ml high-density polyethylene (HDPE) container with a 400-ml mixture of acetonitrile/1 N HCl (90/10) for extraction. Samples were shaken for 60 min on high. The solution was then compressed from the PUF into a 250-ml HDPE container by pushing a 50-ml conical plug into the PUF. An aliquot of 20 ml was then removed, spiked with 25 µl of 0.1 µg ml−1 mixed internal standard, vortexed to mix, and evaporated until dry. The sample was then reconstituted with 1 ml of a methanol/water (50/50) mixture and 0.1% formic acid, vortexed, sonicated, and filtered through a 0.2-µm syringe filter. Florpyrauxifen-benzyl was then quantified using liquid chromatography combined with mass spectrometry. The limit of florpyrauxifen-benzyl detection for PUFs was 10 ng PUF−1.
For filter paper extraction and analysis, each filter paper was placed into a 50-ml HDPE container with 20 ml of a mixture of acetonitrile/1 N HCl (90/10). Samples were shaken for 60 min on high, and a 10-ml aliquot was removed. The aliquot was spiked with 25 µl of 0.1-µg ml−1 mixed internal standard. The sample was vortexed to mix, evaporated to dry, and reconstituted using a 1-ml mixture of methanol/water (50/50) and 0.1% formic acid. The sample was vortexed, sonicated, and filtered through a 0.2-µm syringe filter. Florpyrauxifen-benzyl from filter papers was quantified using liquid chromatography combined with mass spectrometry. The limit of florpyrauxifen-benzyl detection for filter papers was 1 ng filter paper−1.
Visible estimates of soybean injury were rated at 3 WAT on a scale of 0 to 100, where 0 represents no crop injury and 100 represents crop death (Frans and Talbert Reference Frans and Talbert1986). The two bioindicator soybean rows were divided into four 1.5-m row quadrants. Each of the eight individual quadrants per plot was rated independently of the others. Collective ratings in each plot allowed for the assessment of maximum injury and an average estimated injury rating per plot. Additionally, the distance to 5% injury was calculated from the center of each plot toward the greatest amount of injury, most commonly in the downwind direction from the treated area. Distance to 5% injury was used to assess the movement of florpyrauxifen-benzyl and dicamba, as 5% soybean injury was expected to be an insignificant amount of injury most producers would struggle to assess.
The total nanograms of herbicide acid detected, the average and maximum injury 3 WAT, and the distance to 5% injury were all statistically analyzed by treatment. Average and maximum injury were determined to follow a beta distribution based on AICc and BIC values in the distribution platform of JMP Pro 16. Distance to 5% injury was determined to follow a gamma distribution. No statistical analysis was applied to the total ng of herbicide acid detected, which was only reported for qualitative assessment. A single-factor ANOVA was used to assess each respective treatment in SAS 9.4 utilizing the PROC GLIMMIX procedure. Block and year were considered random effects, as they were assumed to be uncorrelated to the individual main effect. Means were separated using Fisher’s protected least significant difference (α = 0.05).
Results and Discussion
Low-Dose Florpyrauxifen-benzyl Exposure to Narrow-Row (15 cm) Soybean
Regression analysis was utilized to model narrow-row soybean injury at 3 WAT (Figure 1). Narrow-row soybean was more susceptible to injury from low-dose exposures of spray-applied florpyrauxifen-benzyl than the herbicide coated on urea combined over 2020 and 2021. For instance, if soybean intercepted florpyrauxifen-benzyl at 2 g ai ha−1, a spray application would be expected to cause >90% injury, whereas the same rate of herbicide coated on urea would be expected to cause <10% injury. Additionally, injury caused by florpyrauxifen-benzyl coated on urea never exceeded 30%, whereas spraying florpyrauxifen-benzyl at 3 g ai ha−1 resulted in greater than 95% injury. Soybean injury caused by spray-applied florpyrauxifen-benzyl increased at a much greater rate as herbicide rate increased compared to coated urea applications. The highest florpyrauxifen-benzyl rate (5.63 g ai ha−1), which is ∼1/20th of the labeled rate, was the most injurious rate coated on urea in both years compared to subsequently lower rates. As in the research by Miller and Norsworthy (Reference Miller and Norsworthy2018c), soybean injury levels numerically increased as the rate increased following low-dose exposure to spray-applied florpyrauxifen-benzyl across both years. The florpyrauxifen-benzyl rates (0.18 to 5.63 g ai ha−1) evaluated in this research are representative of simulated drift rates and are rates that can be obtained from both aerial and ground applications (Butts et al. Reference Butts, Fritz, Kouame, Norsworthy, Barber, Ross, Lorenz, Thrash, Bateman and Adamczyk2022).
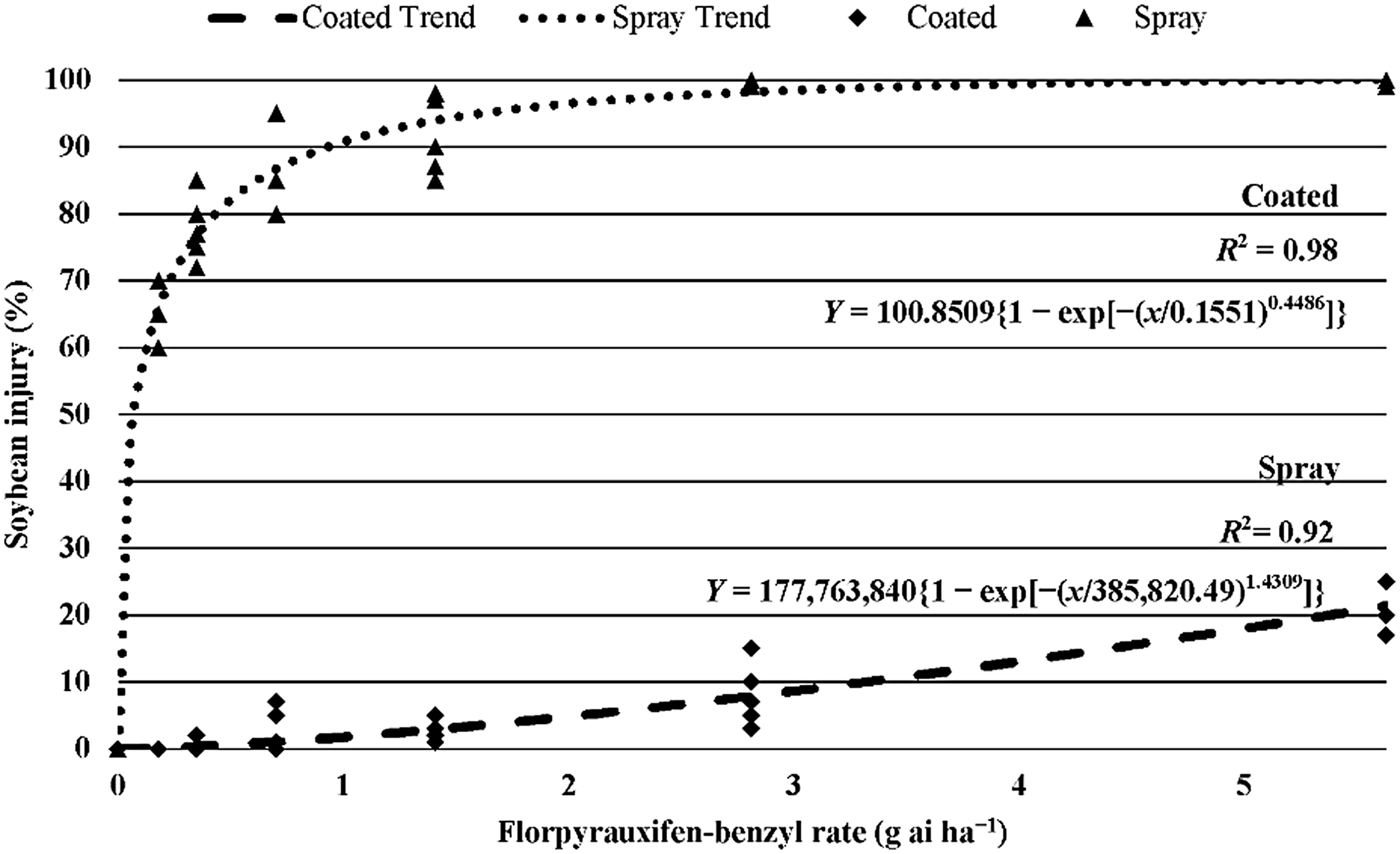
Figure 1. Weibull growth model Y = a * {1 − exp[−(x/b) c ]}, where a is asymptote, b is inflection point, c is growth rate, and x is herbicide rate, fit to florpyrauxifen-benzyl (FPB) rate (coated on urea and spray-applied) and narrow-row soybean injury at 3 wk after treatment (WAT) in 2020 and 2021. The R 2 value displays the percentage of variability explained by the fit of the line for each respective application.
When soybean relative yield was regressed over soybean injury at 3 WAT for spray-applied florpyrauxifen-benzyl, soybean relative yield decreased as soybean injury increased (Figure 2). Figure 2 indicates that soybean began to exhibit yield losses following an application of spray-applied florpyrauxifen-benzyl when the level of soybean injury reached ∼65%. When soybean injury reached 90%, soybean relative yield decreased to <20% of nontreated soybean. However, coating florpyrauxifen-benzyl on urea resulted in no yield loss compared to nontreated controls as the rate increased, even though some soybean injury did persist, as mentioned previously (data not shown). The level of injury caused by spray-applied florpyrauxifen-benzyl was enough to equate to yield losses. Figure 1 illustrates that applying florpyrauxifen-benzyl coated on urea is safer than applying the herbicide as spray with a reduced risk of soybean yield loss, while Figure 2 shows the relative yield loss resulting from spray-applied florpyrauxifen-benzyl on soybean.
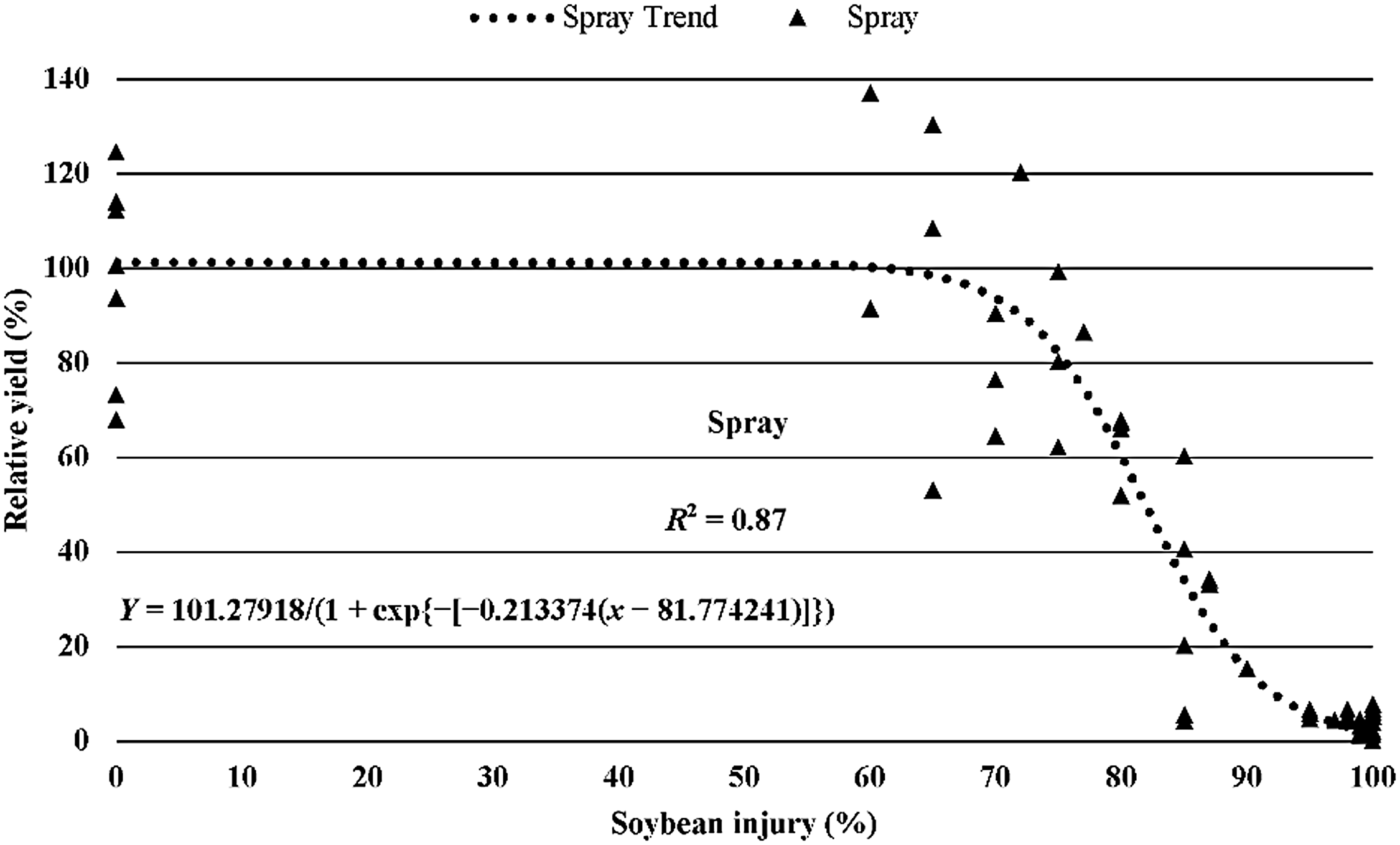
Figure 2. Logistic 3P model Y = c/(1 + exp{−[a(x − b)]}), where a is growth rate, b is inflection point, c is asymptote, and x is soybean injury, fit to the relationship between narrow-row soybean injury at 3 WAT and relative yield caused from an application of spray-applied FPB in 2020 and 2021. The R 2 value displays the percentage of variability explained by the fit of the line for each respective application.
As expected, on the basis of the responses illustrated in Figures 1 and 2, narrow-row soybean groundcover relative to nontreated plots at 3 WAT produced a significant two-way interaction between florpyrauxifen-benzyl rate and application method (Table 1). Generally, the two-way interaction indicated that spraying florpyrauxifen-benzyl caused greater injury to narrow-row soybean compared to when the herbicide was applied coated on urea, which, in return, resulted in less amounts of aboveground foliage. At 3 WAT, coated florpyrauxifen-benzyl on urea at 0.18 to 5.63 g ai ha−1 caused no differences in relative groundcover. However, when florpyrauxifen-benzyl was spray-applied at 0.35 to 5.63 g ai ha−1, all levels of relative groundcover were lower than the respective rate coated on urea. Similar to Figure 1, which shows that greater spray-applied florpyrauxifen-benzyl rates caused greater injury, relative groundcover decreased as spray-applied rates increased (Table 1). Nonetheless, as rates of spray-applied florpyrauxifen-benzyl increased to over 0.35 g ai ha−1, soybean injury increased, leading to noticeable decreases in relative groundcover at 3 WAT and relative yield. Relative soybean groundcover is often utilized as a predictive tool for soybean growth inhibition and potential yield losses (Donald Reference Donald1998). No such observation was made when florpyrauxifen-benzyl was coated on urea.
Table 1. Narrow-row soybean relative groundcover differences caused by low rates of florpyrauxifen-benzyl spray-applied and coated on urea in 2020 and 2021 at Fayetteville, AR. a,b,c
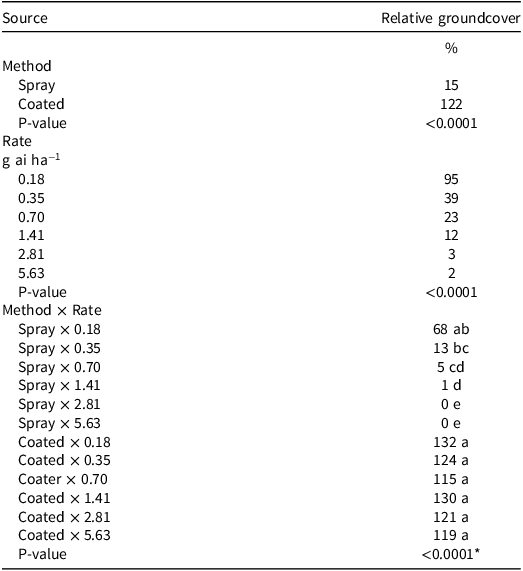
a Applications of florpyrauxifen-benzyl applied to V3–V4 soybean.
b Groundcover reported as relative to nontreated control. Nontreated control = 100%.
c Means within the same column not containing the same letter are different according to Tukey’s HSD (α = 0.05).
* Significant at P < 0.05.
Yield components, including the number of pods per plant, the number of seeds per pod, and 100-seed weight, all produced significant two-way interactions between florpyrauxifen-benzyl rate and application method (Table 2). Generally, many florpyrauxifen-benzyl rates and application method combinations were not different, but spray-applied florpyrauxifen-benzyl at 5.63 g ai ha−1 had the lowest number of pods per plant, especially lower than when the herbicide was spray-applied at 0.7 g ai ha−1 and coated on urea at 2.81 g ai ha−1. Applications of florpyrauxifen-benzyl did not result in differences in seeds per plant likely due to high levels of variability in plant development following exposure to the herbicide. Overall, an application of florpyrauxifen-benzyl coated on urea, across rates, generally caused little to no change in the number of seeds per plant, but spray-applied florpyrauxifen-benzyl at 5.63 g ai ha−1 generally caused a decrease in the number of pods per plant.
Table 2. Effects of low doses of florpyrauxifen-benzyl spray-applied and coated on urea on narrow-row soybean yield parameters in 2020 and 2021 at Fayetteville, AR. a,b,c
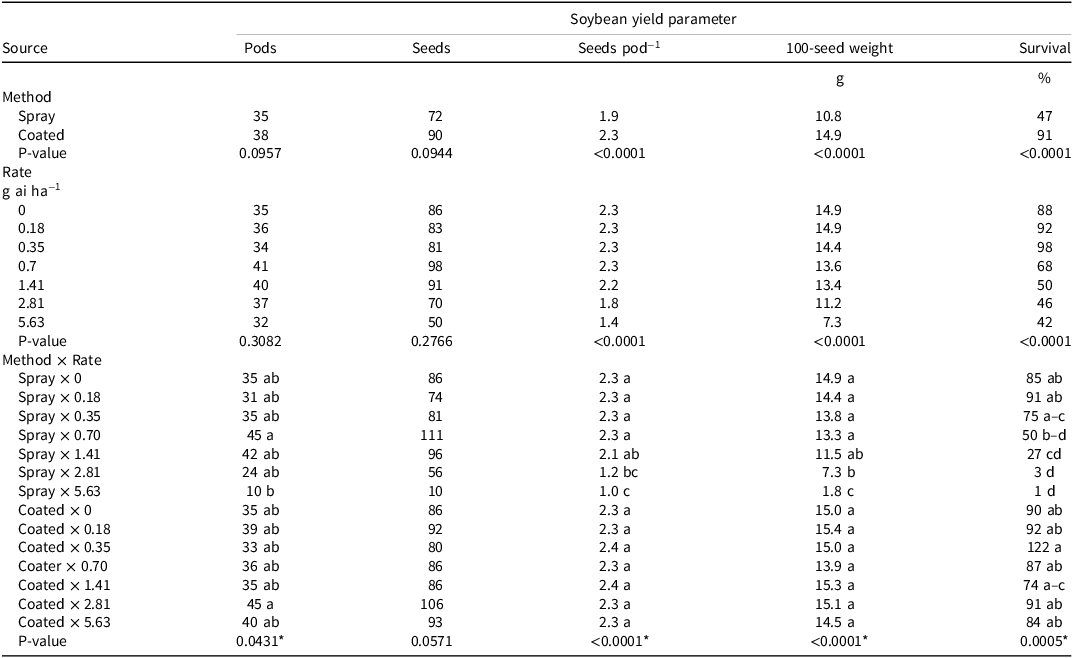
a Applications of florpyrauxifen-benzyl applied to V3–V4 soybean.
b Survival rate recorded only in 2021.
c Means within the same column not containing the same letter are different according to Tukey’s HSD (α = 0.05).
* Significant at P < 0.05.
A similar result was observed in the number of seeds per pod following applications of florpyrauxifen-benzyl as pods per plant, where differences were observed between florpyrauxifen-benzyl rates and application methods (Table 2). Consequently, a lower number of seeds per plant occurred when florpyrauxifen-benzyl was spray-applied at 5.63 g ai ha−1 where soybean plants averaged 1.0 seeds pod−1. Additionally, soybean following spray-applied florpyrauxifen-benzyl at 2.81 g ai ha−1 produced a lower number of seeds per pod (1.2) than any rate of florpyrauxifen-benzyl coated on urea and spray-applied at 0 to 1.41 g ai ha−1. Seeds per pod were expected to follow a similar response as pod and seed counts, as they are directly linked; however, seeds per plant failed to exhibit differences between application methods.
Following similar statistical separations as the seed per pod analysis, 100-seed weights were typically higher where a greater number of seeds per pod were recorded (Table 2). Soybean treated with spray-applied florpyrauxifen-benzyl at 5.63 g ai ha−1 resulted in the lowest recorded 100-seed weight at 1.8 g and was less than all reported rates of florpyrauxifen-benzyl coated on urea and spray-applied at 0 to 1.41 g ai ha−1. Similarly, when florpyrauxifen-benzyl was spray-applied at 2.81 ga ai ha−1, the second lowest 100-seed weight (7.3 g) was recorded and was less than all rates of florpyrauxifen-benzyl coated on urea and spray-applied at 0 to 0.7 g ai ha−1. The results from the 100-seed weight assessment indicate that spray-applied florpyrauxifen-benzyl at 2.81 and 5.63 g ai ha−1 not only caused fewer seeds per pod to be produced but also caused seeds with less weight compared to lower rates of spray-applied herbicide and all rates coated on urea.
A two-way interaction between florpyrauxifen-benzyl rate and application method was observed for soybean survival (Table 2). On the basis of the results reported in Figure 1, modeled soybean injury levels reached nearly 90% as spray-applied florpyrauxifen-benzyl rates exceeded ∼1.5 g ai ha−1. Similarly, the increase in soybean injury helped explain the decrease in soybean survival rate (Table 2). As spray-applied florpyrauxifen-benzyl rates reached and exceeded 1.41 g ai ha−1, soybean survival reached a level (27%) lower than all coated on urea rates, except 1.41 g ai ha−1 and spray-applied at 0 and 0.18 g ai ha−1. No differences in soybean survival occurred among florpyrauxifen-benzyl rates when the herbicide was coated on urea. Generally, if a soybean survived a spray application of florpyrauxifen-benzyl, no major differences in soybean yield parameters were observed until the sprayed herbicide rate exceeded 1.41 g ai ha−1. Likewise, these results indicate that although florpyrauxifen-benzyl coated on urea may produce injury, there is a low likelihood that the injury will be extreme enough to cause soybean mortality.
Low-Dose Florpyrauxifen-benzyl Effect on Wide-Row (0.9 m) Soybean
Regression analysis of wide-row soybean injury data at 3 WAT revealed that soybean was more susceptible to injury following spray-applied florpyrauxifen-benzyl applications than when the herbicide is coated on urea (Figure 3). On the basis of the responses, modeled wide-row soybean injury following applications of florpyrauxifen-benzyl within the selected low doses should not exceed 30% at 3 WAT. Conversely, wide-row soybean injury was modeled to be greater than 90% when exposed to florpyrauxifen-benzyl spray-applied at 2 g ai ha−1. Generally, soybean injury caused by spray-applied florpyrauxifen-benzyl increased at a greater rate compared to when the herbicide was coated on urea. These soybean injury results at 3 WAT correspond with research by Miller and Norsworthy (Reference Miller and Norsworthy2018c) in which soybean injury levels increased as the rate of spray-applied florpyrauxifen-benzyl increased. Previous research outlined that a lack of foliar interception of postemergence herbicides resulted in reduced efficacy in weed control (Norsworthy et al. Reference Norsworthy, Oliver and Purcell1999). Likewise, a similar conclusion can be drawn here, where a lack of foliar interception of florpyrauxifen-benzyl when coated on urea led to less soybean injury. Florpyrauxifen-benzyl has limited soil activity and root uptake (Miller and Norsworthy Reference Miller and Norsworthy2018b). Hence increased soybean injury was explained by the foliar interception of increased spray-applied rates of florpyrauxifen-benzyl.
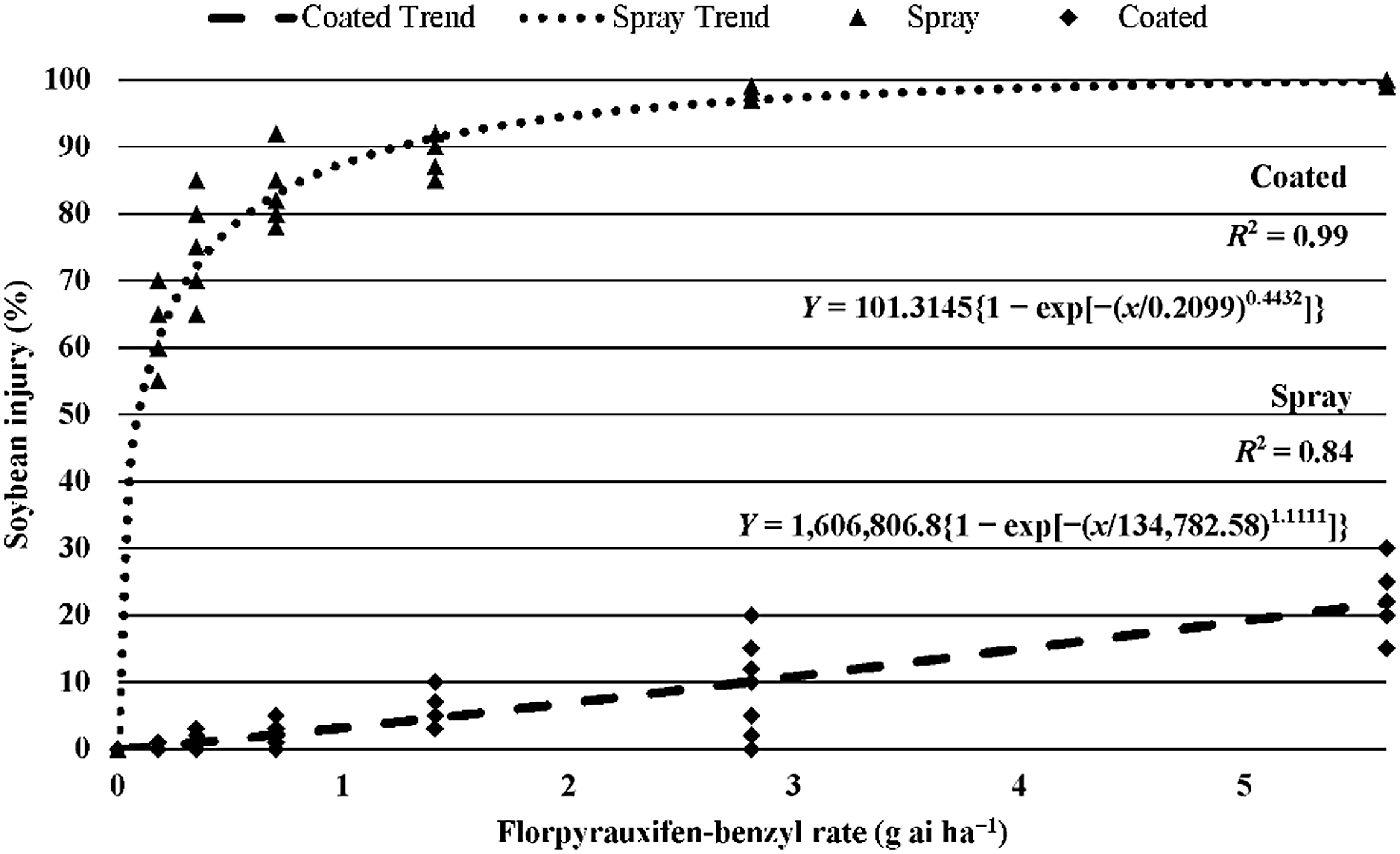
Figure 3. Weibull growth model Y = a * {1 − exp[−(x/b) c ]}, where a is asymptote, b is inflection point, c is growth rate, and x is herbicide rate, fit to FPB rate (coated on urea and spray-applied) and wide-row soybean injury at 3 WAT in 2020 and 2021. The R 2 value displays the percentage of variability explained by the fit of the line for each respective application method.
The relationship between wide-row soybean relative yield and soybean injury at 3 WAT illustrates that spray-applied florpyrauxifen-benzyl causes the relative yield to decrease as soybean injury levels increase (Figure 4). As soybean injury caused by spray-applied florpyrauxifen-benzyl exceeded 60%, soybean relative yield decreased. No significant decrease in soybean relative yield was exhibited until soybean injury exceeded 60% injury. Likewise, as injury levels rose to 90%, soybean relative yield fell below 20%, indicating yield losses compared to the nontreated control. However, no rate of coated florpyrauxifen-benzyl on urea caused any differences in relative yield compared to the nontreated control, even when soybean exhibited injury (Figure 3; data not shown).
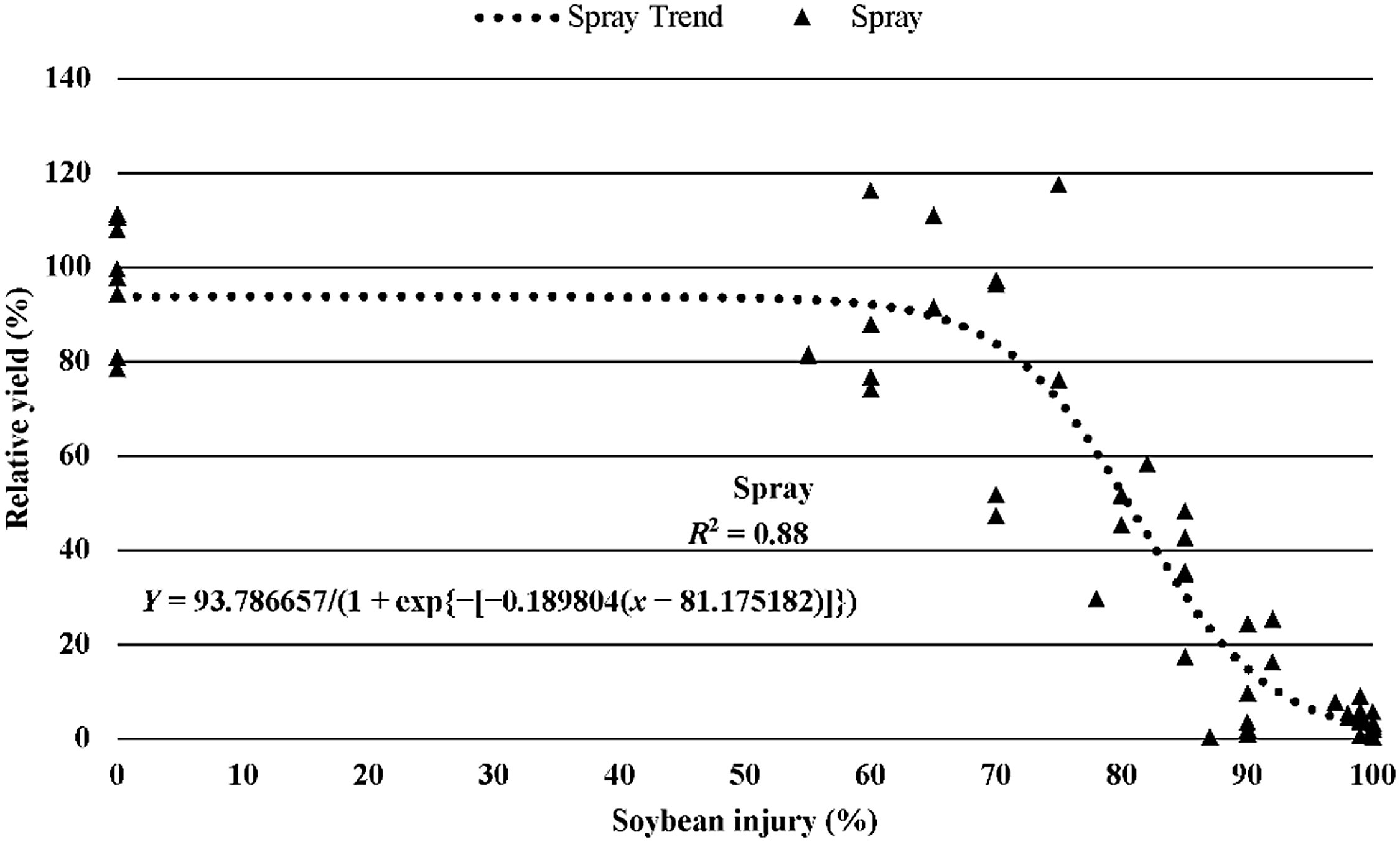
Figure 4. Logistic 3P model Y = c/(1 + exp{−[a(x − b)]}), where a is growth rate, b is inflection point, c is asymptote, and x is soybean injury, fit to the relationship between wide-row soybean injury at 3 WAT and relative yield caused from an application of spray-applied FPB in 2020 and 2021. The R 2 value displays the percentage of variability explained by the fit of the line for each respective application.
Like in the narrow-row soybean experiment, differences in soybean relative groundcover at 3 WAT were explained by an interaction between florpyrauxifen-benzyl rate and application method (Table 3). When evaluating wide-row soybean relative groundcover at 3 WAT, every rate of florpyrauxifen-benzyl that was coated on urea produced a relative groundcover that was not different from each rate. Additionally, spray-applied florpyrauxifen-benzyl at 0.7 to 5.63 g ai ha−1 caused the relative groundcover to be lower than florpyrauxifen-benzyl coated on urea at 0.35 to 5.63 g ai ha−1. Differences in relative groundcover were expected considering the high level of injury caused by spray-applied florpyrauxifen-benzyl and the lack of injury caused by florpyrauxifen-benzyl coated on urea, as illustrated in Figure 3. As previously stated, relative groundcover serves as an indicator of potential yield losses, and yield losses are expected based on the trend of relative groundcover losses in Table 3 (Donald Reference Donald1998). Like the narrow-row soybean experiment, no such observation was observed when florpyrauxifen-benzyl was coated on urea in this experiment.
Table 3. Narrow-row soybean relative groundcover differences caused by low rates of florpyrauxifen-benzyl spray-applied and coated on urea in 2020 and 2021 at Fayetteville, AR. a,b,c
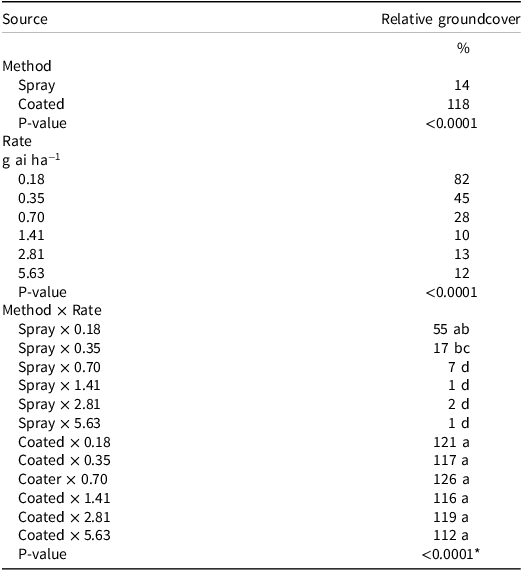
a Applications of florpyrauxifen-benzyl applied to V3–V4 soybean.
b Groundcover reported as relative to nontreated control. Nontreated control = 100%.
c Means within the same column not containing the same letter are different according to Tukey’s HSD (α = 0.05).
* Significant at P < 0.05.
Yield components of seeds per plant, seeds per pod, and 100-seed weight results were best illustrated by the two-way interaction of florpyrauxifen-benzyl rate and application method, while differences in pods per plant were best explained by the single factor of herbicide rate (Table 4). When the florpyrauxifen-benzyl rate increased to 5.63 g ai ha−1, the number of pods per plant decreased to 19, which was fewer than the number of pods produced following an application of florpyrauxifen-benzyl at 1.41 g ai ha−1. When the number of seeds per plant was evaluated, spray-applied florpyrauxifen-benzyl at 5.63 g ai ha−1 decreased from 15 seeds plant−1 to a level lower than spray-applied florpyrauxifen-benzyl at 0 to 2.81 g ai ha−1 and coated on urea at 0 and 1.41 to 5.63 g ai ha−1. Spraying florpyrauxifen-benzyl at the highest rate appeared to generally decrease the number of seeds per plant, whereas coating the herbicide on urea did not.
Table 4. Effects of low doses of florpyrauxifen-benzyl spray-applied and coated on urea on narrow-row soybean yield parameters in 2020 and 2021 at Fayetteville, AR. a,b,c
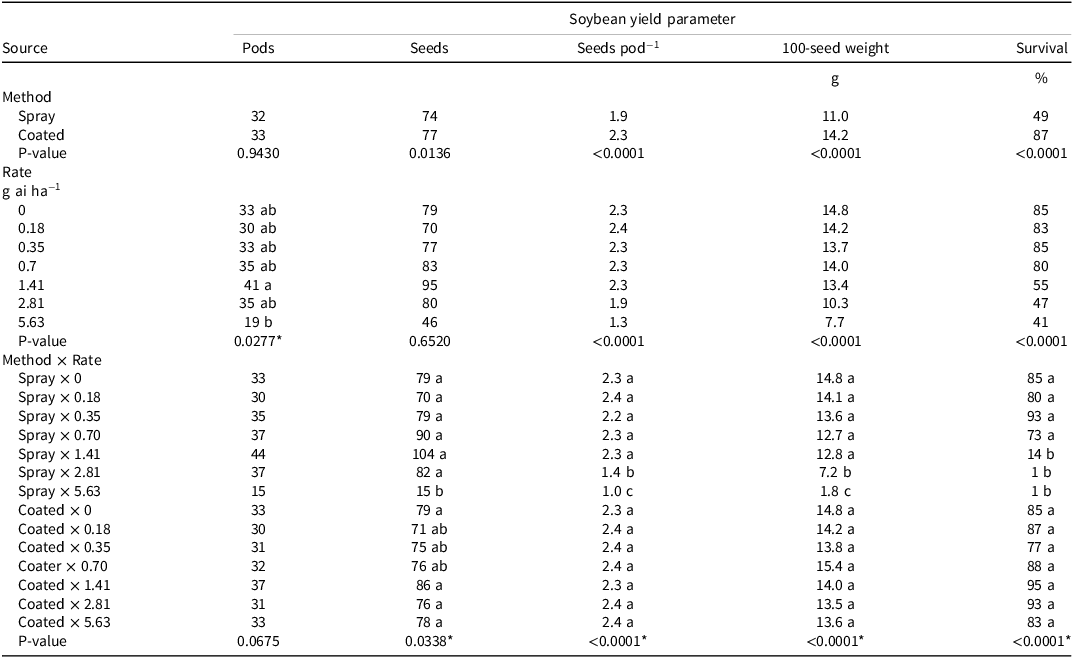
a Applications of florpyrauxifen-benzyl applied to V3–V4 soybean.
b Survival rate recorded only in 2021.
c Means within the same column not containing the same letter are different according to Tukey’s HSD (α = 0.05).
* Significant at P < 0.05.
Differences in the number of seeds per pod were illustrated by an interaction between florpyrauxifen-benzyl rate and application method (Table 4). No rate of florpyrauxifen-benzyl coated on urea was statistically different than the other. Moreover, as the spray-applied florpyrauxifen-benzyl rate increased to 2.81 g ai ha−1, the number of seeds per pod fell to 1.4, which was lower than the number of seeds per pod produced from spray-applied florpyrauxifen-benzyl at 0 to 1.41 g ai ha−1. When florpyrauxifen-benzyl was spray-applied at 5.63 g ai ha−1, only 1.6 seeds pod−1 were recorded, whereas florpyrauxifen-benzyl coated on urea produced 2.4 seeds pod−1. Seeds per pod were expected to follow a similar response as pod and seed counts, as they are directly linked; however, pods per plant failed to exhibit differences between factors. The decreases in pods per plant, seeds per plant, and seeds per pod also correspond to decreases in 100-seed weight.
Following similar statistical separations as seeds per pod, 100-seed weight differences were illustrated by the interaction between florpyrauxifen-benzyl rate and application method (Table 4). No differences in 100-seed weight between florpyrauxifen-benzyl coated on urea rates were observed, and weights ranged from 13.5 to 15.4 g. However, as the spray-applied florpyrauxifen-benzyl rate increased to 2.81 g ai ha−1, the 100-seed weight fell to 7.2 g, which was lower than 100-seed weights produced from spray-applied florpyrauxifen-benzyl at 0 to 1.41 g ai ha−1 and all rates of florpyrauxifen-benzyl on urea. Additionally, spray-applied florpyrauxifen-benzyl at 5.63 g ai ha−1 caused 100-seed weight to decrease to 1.8 g, which was lower than for all other herbicide rates and application method combinations.
Owing to wide-row soybean yield differences in 2020, the soybean survival rate was evaluated in 2021 to better explain those yield losses (Table 4). Differences in wide-row soybean survival rate began to appear when spray-applied florpyrauxifen-benzyl rates reached 1.41 g ai ha−1, where spraying florpyrauxifen-benzyl caused greater mortality compared to coated on urea. Survival rates for soybean treated with florpyrauxifen-benzyl coated on urea was lower than 77%, but spraying florpyrauxifen-benzyl onto soybean decreased the survival rate to 1% at the 2.81 and 5.63 g ai ha−1 rates. Although spray-applied florpyrauxifen-benzyl at 0 to 0.7 g ai ha−1 did not lower soybean survival rate, survival rate fell to 14% when florpyrauxifen-benzyl was spray-applied at 1.41 g ai ha−1. Off-target movement of spray-applied florpyrauxifen-benzyl would likely have detrimental impacts on soybean to the point of mortality, whereas coating the herbicide on urea would not. Following the logic explained in the narrow-row soybean experiment, soybean survival was a key driver for decreases in soybean yield parameters, relative groundcover, and relative yield. These results indicate that soybean mortality is limited following an application of florpyrauxifen-benzyl coated on urea and increases as the rate of spray-applied florpyrauxifen-benzyl increases. Although soybean injury may persist when applying florpyrauxifen-benzyl coated on urea, it is unlikely that the injury will rise to the magnitude of killing plants and reducing yield.
Florpyrauxifen-benzyl Coated on Urea Volatility Potential
All florpyrauxifen-benzyl treatments were less volatile than dicamba mixed with glyphosate (Table 5). Regardless of soil condition at application or application method, florpyrauxifen-benzyl exhibited a low likelihood of volatilization by causing no injury at 3 WAT, along with no detection of florpyrauxifen-benzyl above the limit of detection in air samples. Comparatively, dicamba plus glyphosate caused soybean to elicit 47% maximum injury, 24% average injury, and a distance to 5% injury in the downwind direction of 5 m. Additionally, 3,700 ng of dicamba acid was detected following the 48-h duration of the experiment. The theory behind evaluating the volatility of florpyrauxifen-benzyl coated on urea was derived when considering that ammonia tends to volatilize from hydrolysis of urea fertilizer on the soil surface when in contact with water and not immediately flooded (Norman et al. Reference Norman, Wilson, Slaton, Griggs, Bushong and Gbur2009). On the basis of this experiment, ammonia volatilization from a coated urea appears to have little influence on the volatility of the herbicide. This research validates that florpyrauxifen-benzyl is a nonvolatile herbicide and that any off-target movement that occurs is a direct result of physical spray drift.
Table 5. Maximum and average injury to sensitive soybean, distance to 5% injury, and total dicamba or florpyrauxifen-benzyl detected 3 wk after treatment in 2020 and 2021 at Fayetteville, AR. a,b,c,d,e

a Application timing was V3–V4 soybean.
b Abbreviations: FPB, florpyrauxifen-benzyl applied at 30 g ai ha−1; ND, no detection; WAT, weeks after treatment.
c Total acid detected is a combination of filter paper and polyurethane filter detection.
d The limit of detection of florpyrauxifen-benzyl for filter paper and polyurethane filter analysis was 0.3 and 3 ng sample−1, respectively.
e Means within the same column not containing the same letter are different according to Tukey’s HSD (α = 0.05).
f Sprayed florpyrauxifen-benzyl included methylated seed oil at 584 ml ha−1.
g Dicamba represents dicamba and glyphosate mixed at 560 and 1,260 ga ae ha−1, respectively.
* Significant at P < 0.05.
Practical Implications
Coating florpyrauxifen-benzyl on urea is likely a successful application method to mitigate soybean injury caused by off-target movement when the exposure occurs at the V3 to V4 soybean growth stage. Likewise, any injury that may occur from florpyrauxifen-benzyl coated on urea at 0.18 to 5.63 g ai ha−1 is unlikely to negatively impact overall soybean yield. However, spray-applied florpyrauxifen-benzyl at 0.18 g ai ha−1 or higher will cause significant injury and negative impacts on soybean yield. If soybean is near the off-target movement occurrence where herbicide exposure will be the greatest, soybean mortality should be expected. Additionally, coating florpyrauxifen-benzyl on urea did not increase the likelihood of herbicide volatilization. Overall, the alternative application method of coating florpyrauxifen-benzyl on urea has the potential to replace the traditional method of spraying as a safer application method when applied near soybean fields.
Acknowledgments
This research was conducted in cooperation with Corteva Agriscience. Facilities and equipment were provided by the University of Arkansas System Division of Agriculture.
Funding
Corteva provided partial research funding and florpyrauxifen-benzyl. Additional funding for this research was provided by the Arkansas Rice Checkoff Program administered by the Arkansas Rice Research and Promotion Board and the Arkansas Soybean Promotion Board.
Competing interests
The authors declare no conflicts of interest.