Cholesterol is a lipid that is synthesised in the body. It is also consumed as part of the daily diet. Although cholesterol plays important biochemical roles in the body, high cholesterol levels and abnormal metabolism can lead to the development of chronic obesity-related diseases, such as CVD( Reference Seo and Choi 1 , Reference Shao, Bartley and Yokoyama 2 ). As an alternative to pharmacological medicine, dietary means to control risk factors for obesity-related diseases have recently received considerable attention to reduce CVD risk( Reference Zhang, Zhou and Fang 3 ). Accumulated evidence suggests that the cholesterol-lowering effects could be achieved by the following mechanisms, each with its own cellular markers: inhibition of hepatic synthesis, which has AMP-activated protein kinase α (AMPKα)( Reference Jeon 4 ), 3-hydroxy-3-methylglutaryl coenzyme A reductase (HMG-CoAr)( Reference Chen, Wang and Li 5 ) and sterol regulatory element-binding protein-1c (SREBP-1c)( Reference Ji, Zhao and Leng 6 ) as biomarkers; inhibition of cholesterol accumulation in peripheral tissue, with apoB( Reference Kim, Hong and Jeon 7 ), LDL receptor (LDLR)( Reference Kemper, Srivastava and King 8 , Reference Levinson and Wagner 9 ), liver X receptor (LXR)( Reference He, Wang and Pan 10 ) and PPARα ( Reference Ogata, Tsujita and Hossain 11 ) as biomarkers; acceleration of cholesterol conversion into bile acids, with cholesterol 7α-hydroxylase (CYP7A1)( Reference Ogata, Tsujita and Hossain 11 ) and farnesoid X receptor (FXR)( Reference Moschetta 12 ) as biomarkers; and inhibition of bile acid reabsorption, with apical sodium-dependent bile acid transporter (ASBT), FXR and ileal bile acid-binding protein (IBABP)( Reference Fu and Klaassen 13 ) as biomarkers. The relative activity of each mechanism can be assessed by quantifying relevant biomarkers( Reference Zhang, Zhou and Fang 3 ).
Increasing evidence supports that higher whole-grain consumption is associated with a reduced risk of CVD development( Reference Ross, Bruce and Blondel-Lubrano 14 , Reference Zhou, Hergert and Rompato 15 ). According to previous studies, barley exhibited hypocholesterolaemic effects, which may be attributed to its bioactive components, particularly dietary fibre and β-glucans( Reference Zhou, Hergert and Rompato 15 – Reference De Angelis, Montemurno and Vannini 19 ). In contrast to regular hulled barley, hull-less barley is more advantageous in terms of processing and food applications( Reference Knutsen and Holtekjølen 20 ). Highland hull-less barley, also known as Qingke in Chinese, grows under extreme geographical conditions and contains a higher amount of β-glucans and dietary fibre( Reference Xia, Li and Ding 21 ). However, available information on the cholesterol-lowering effect of whole-grain highland hull-less barley (WHLB) is limited. In particular, the specific mechanism by which WHLB affects cholesterol metabolism is still unknown.
Thus, the present study aims to determine the hypocholesterolaemic effect and mechanism of WHLB. Plasma levels of total cholesterol (TC), TAG, LDL-cholesterol and HDL-cholesterol and TC, TAG and total lipid levels of the liver were investigated after high-fat diet (HFD)-fed rats were given different amounts of WHLB. The degrees of hepatic steatosis were examined through haematoxylin–eosin (H&E) staining of sections of the liver. Bile acid levels in small intestinal contents and faeces were examined simultaneously. To determine the molecular mechanism, real-time PCR (RT-PCR) was conducted to quantify the mRNA levels of abovementioned cellular biomarkers. Protein levels of CYP7A1, HMG-CoAr, LDLR, ASBT and IBABP were analysed to confirm the main results of RT-PCR. Overall, this study may provide important implications for future functional food development of WHLB.
Methods
Sample preparation
WHLB (Tibet Hordeum vulgare L. Zangqing 320) was provided by Jun Pro Food Co., Ltd. WHLB was dried in an oven at 55°C for 24 h and then ground and passed through an 80-mesh sieve (0·5 mm). The moisture (American Association of Cereal Chemists (AACC) method 44-16)( 22 ) content in WHLB was 8·71 (sd 0·03) %. The contents (based on dry weight) of ash (AACC method 08-01)( 22 ), fat (AACC method 30-10)( 22 ), protein (KjelFlex K-360 nitrogen determination system; Buchi Laboratory Equipment Trading, Ltd) and β-glucans (Mixed-linkage β-glucan kit; Megazyme Int. Ireland Ltd) in WHLB were 1·95 (sd 0·08), 1·03 (sd 0·02), 17·00 (sd 0·26) and 5·77 (sd 0·28) g/100 g, respectively. The contents (based on dry weight) of total dietary fibre, insoluble dietary fibre, and soluble dietary fibre contents (AOAC method 991.43)( 23 ) in WHLB were 19·01 (sd 0·54), 11·64 (sd 0·95) and 9·28 (sd 0·01) g/100 g, respectively.
Animals and diets
Four dietary groups were created to investigate the dosage effect of WHLB, as follows: normal control (NC) group, fed with normal AIN-93G( Reference Reeves, Nielsen and Fahey 24 ) diet; blank control (BC) group, fed with an HFD, plus 1 % cholesterol and with additional 10 % lard instead of soyabean oil( Reference He, Wang and Pan 10 ); the low-dose (LD) group, fed with HFD containing low-dose (10 %) of WHLB; and the high-dose (HD) group, fed with HFD containing high dose (48·95 %) of WHLB instead of maize starch( Reference Xia, Li and Ding 21 ) (online Supplementary Table S1). To study the time effect of WHLB, half of the animals in each group were fed with experimental diets for 4 weeks, whereas the other half was fed for 8 weeks. Therefore, a total of eight groups were created. The sample size was calculated by power analysis according to the formula for quantitative data published by Charan & Kantharia( Reference Charan and Kantharia 25 ). The type I error (α/significance level) was set at 0·05 and the power at 0·80 (with β/type II error at 0·02). The effect size was set at 0·50 (plasma TC of rats decreased from 4·00 to 3·50 mmol/l after high diet dose of WHLB) and sd at 0·33 (mmol/l), based on an initial pilot study. A sample size of eight animals for each group was sufficient for consideration of 10 % attrition. We finally used nine rats per group, because three rats were housed in one stainless steel screen-bottomed cage because of the limited space. Therefore, a total of 72 4-week-old specific pathogen-free Sprague–Dawley male rats (151 (sd 12) g weight) were purchased from Chongqing Tengxin Biotechnology Co., Ltd (permitted by SCXK 2012-0005 (Chongqing)). The room was illuminated with a 12 h light–12 h dark cycle at a constant temperature of 23±2°C and a relative humidity of 55 (sd 10) %. The rats were acclimated by feeding with an AIN-93G diet for 1 week and were given free access to food and water. After acclimation, rats were randomly assigned to the four dietary groups (n 18/group). The experiment design was approved by the Animal Care and Use Committee of Southwest University (Permit SYXK2009-0002) and strictly conducted in accordance with the guidelines for animal care of the National Institute of Health( 26 ).
Sampling and analytical procedures
Fresh faeces (approximately 0·2–1·0 g and 0·2–0·4 g) of each rat were collected weekly. The water content and pH of the faeces were determined. Faeces were collected on the last 3 d of the experimental period, freeze-dried, and then milled for bile acid measurement( Reference Zhang, Fang and Zheng 27 ). On the last day of the experimental period, the rats were fasted overnight (12–14 h) and lightly anaesthetised with diethyl ether( Reference Park and Park 28 ). Their body weights were measured. After decapitation, blood was collected from the neck of each rat into a blood collection tube (Vacutainer; Liuyang City Medical Instrument Factory) containing heparin as an anticoagulant. The plasma was centrifuged at 1400 g at 4°C (5810 centrifuge; Eppendorf China Ltd) for 15 min and the obtained plasma was stored at −80°C until analysis.
The stomach, liver, kidney, abdominal fat and caecum (together with the contents) of each rat were immediately removed, washed with ice-cold 0·9 % NaCl solution, blotted dry on filter paper, and weighed. Organ index was evaluated as follows: organ weight (g)/body weight (g)×100( Reference He, Wang and Pan 10 ). The liver was incised to 5×5×5 mm sections and fixed in Bouin solution( Reference Zhang, Zhou and Fang 3 ). Liver tissues (1·0 and 0·1 g) from each rat were dissected, washed, frozen in liquid N2, and stored at −80°C for lipid analysis and RNA extraction, respectively. The small intestine of each rat was excised. The small intestine was douched with ice-cold 0·9 % NaCl solution. Then, a suspension of intestinal content was collect, freeze-dried, weighed, and then milled for bile acid measurement( Reference Liu, Ogawa and Kishida 29 ). A total of 0·1 g of the small intestine (ileum part) in each rat was dissected, washed, frozen in liquid N2, and stored at −80°C for RNA extraction. Liver and ileum tissues (0·2 g) were collected from each rat in the NC, BC and HD groups after 8 weeks of feeding and stored at −80°C for protein extraction.
Lipid analysis
The concentrations of bile acids in freeze-dried small intestinal contents and faeces were determined using a Rat Total Bile Acid Elisa kit (Fengxiang Biotech Co., Ltd) according to the manufacturer’s instructions. Total lipid in the liver was extracted according to the method of Folch et al. ( Reference Folch, Lees and Sloane Stanley 30 ) and subsequently determined gravimetrically( Reference Zhang, Fang and Zheng 27 ). The levels of TAG and TC in the plasma and liver were quantified with enzymatic methods using assay kits purchased from Sichuan Maker Biotechnology Co., Ltd. LDL-cholesterol and HDL-cholesterol in the plasma were also quantified through enzymatic methods using assay kits (Sichuan Maker). Measurements were performed using a 7020 Automatic Analyzer (Hitachi) in accordance with the manufacturer’s instructions.
Histopathological analysis
The liver was stored in 70 % ethanol until histological analysis after storing at Bouin solution for 24 h. A portion of the stored liver was embedded in paraffin and then cut into 5-μm thick semi-serial histological sections using RM2235 microtome (Microsystems GmbH). Then H&E staining was performed( Reference Zhang, Zhou and Fang 3 ). Representative images were acquired at 20× magnification using an Olympus BX43 microscope (Olympus Optical Co.).
RNA extraction and real-time PCR analysis of gene expression
Total RNA was extracted from the frozen liver and ileum samples using RNAiso Plus reagent (Takara Bio, Inc.). RNA concentration and quality were checked using a NanoDrop 2000 spectrophotometer (Thermo Scientific and integrity was examined using a 1·2 % (w/v) agarose gel. Extracted RNA was used for complementary DNA (cDNA) synthesis using PrimeScript RT reagent kit with gDNA Eraser (Takara). The resulting cDNA was amplified using the SYBR Premix Ex Taq II (Takara) and specific primers (Table 1, synthesised by Sangon Biotech Co., Ltd). The gene expressions of AMPKα, ApoB, CYP7A1, FXR, HMG-CoAr, LDLR, LXR, PPARα and SREBP-1c in the liver and ASBT, FXR, and IBABP in the ileum were investigated. RT-PCR was performed using the LightScanner 32 system (BioFire Diagnostics). The amplification programme consisted of one cycle of 95°C for 30 s, 45–55 cycles of 95°C for 5 s and 60°C for 20 s. To examine the quality of amplification products, melting curve analysis was performed under the following conditions: 95°C 0 s, 20°C/s; 65°C 15 s, 20°C/s; 95°C 0 s, 0·1°C/s, continuous measurement of fluorescence; and 50°C cooled 30 s, 20°C/s. Melting peaks were recorded by plotting the absolute value of the first derivative against the temperature( Reference Xia, Ran and Ye 32 ). Gene expression was calculated using 2-ΔΔCt method; the β-actin gene was used as the housekeeping gene for normalisation( Reference Zhang, Zheng and Tian 31 ).
Table 1 Primers for real-time PCR

AMPKα, AMP-activated protein kinase α; CYP7A1, cholesterol 7α-hydroxylase; HMG-CoAr, 3-hydroxy-3-methylglutaryl coenzyme A reductase; LDLR, LDL receptor; LXR, liver X receptor; SREBP-1c, sterol regulatory element-binding protein-1c; ASBT, apical sodium-dependent bile acid transporter; IBABP, ileal bile acid-binding protein; FXR, farnesoid X receptor.
Protein extraction and Western blot assays
Protein was extracted from the frozen liver and ileum samples using a commercial Tissue Total Protein Lysis Buffer (Biotech). The amount of pooled proteins was determined using a commercial Bradford Protein Assay Kit (Beijing Solarbio Science & Technology Co., Ltd) with bovine serum albumin as the standard. SDS-PAGE and Western blot analysis were performed according to the protocol of Zhang et al. ( Reference Zhang, Zhou and Fang 3 ) After blocking in PBS containing 2 % Tween-20 and 3 % bovine serum antigen, the membranes were incubated with anti-CYP7A1, anti-HMG-CoAr, anti-LDLR, anti-ASBT or anti-IBABP experimental antibodies (Abcam, Inc.) overnight at 4°C. The β-actin was used as a loading control. The membrane was washed thrice with PBS-T (0·1 % Tween-20 in PBS) and incubated with horseradish peroxidase-conjugated secondary antibodies at room temperature for 1 h. Reactive bands were visualised by a SuperSignal R West Pico Chemiluminescence substrate (Pierce). The density of each protein immunoblot was determined using Quantity One Analyzer software (Bio-Rad Laboratories).
Statistical analysis
The results are reported as mean values and standard deviations. Statistical analysis was conducted through one-way ANOVA using SPSS 20.0 software (IBM). P<0·05 was regarded as statistically significant.
Results
Organ indexes
The changes in organ indexes among all groups are displayed in Table 2. No significant differences in stomach and kidney indexes were observed among all groups. The indexes of liver and abdominal fat of the BC group were significantly higher than those in the NC group. The abdominal fat indexes in the LD group were significantly lower, whereas the caecum indexes were significantly higher than those in the BC group after 4-week feeding. All organ indexes of the LD group were similar with those of the BC group after 8-week feeding. However, the indexes of liver and abdominal fat of HD groups were significantly lower than those in the BC group. Caecum indexes of HD groups were significantly higher than those in other groups.
Table 2 Effect of diet with different doses of whole-grain highland hull-less barley on organ indexes of high-fat diet Sprague–Dawley ratsFootnote * (Mean values and standard deviations; n 9)

NC, normal control group; BC, blank control group; LD, low-dose group; and HD, high-dose group.
a,b,c Mean values in the same row with unlike letters are significantly different (P<0·05).
* Caecum index was calculated together with the contents.
Plasma and liver lipid profiles
Changes in plasma and liver lipid levels are shown in Fig. 1. The plasma TC, TAG, HDL-cholesterol and LDL-cholesterol levels in the BC group were significantly higher than those in the NC group. The TC and LDL-cholesterol levels in the HD group were significantly lower than those in the BC group. HDL-cholesterol levels in the HD group were significantly higher than those in the BC group after 8-week feeding. The TC, TAG, and total lipid levels in the liver of all HFD groups were significantly higher than those in the NC group. The TC content and lipid concentration in the liver of the HD group were significantly lower than those in the BC group at week 8. TAG levels of the HD group were slightly lower than the BC and LD groups, whereas the difference was not significant. The lipid levels of the LD group showed no significant differences with those of the BC group.
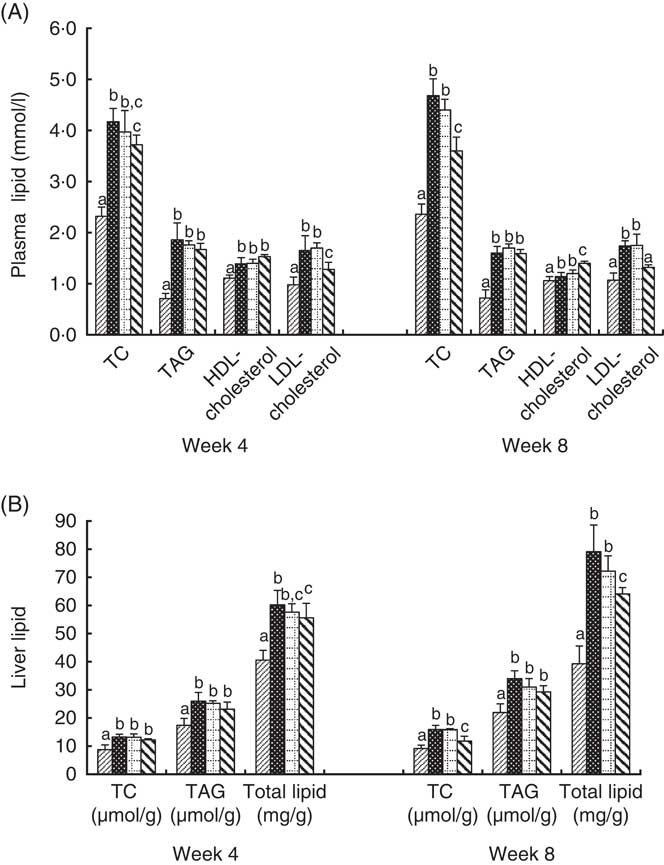
Fig. 1 Effect of diet with different doses of whole-grain highland hull-less barley on plasma (A) and liver (B) lipid levels of high-fat diet Sprague–Dawley rats. Values are means (n 9) and standard deviations. a,b,c Mean values of the same index in the four groups with unlike letters are significantly different (P<0·05). TC, total cholesterol; , normal control group;
, blank control group;
, low-dose group;
, high-dose group.
Haematoxylin–eosin-stained sections of liver
After stained with H&E, the degrees of hepatic steatosis were examined (Fig. 2). In the liver of the NC group, the cells had clear borders with intact cytoplasm and prominent nuclei. Moreover, no inflammatory cell infiltrate was observed. Different degrees of hepatic steatosis were observed in other rat groups. Significant morphological changes were observed in the liver cells of the BC group after 4 weeks of the HFD. Steatosis became significant, excessive lipid accumulation occurred in vesicles, and most lipid droplets surrounded the nucleus or were located at the side of the cells. Meanwhile, some lipids formed large droplets after 8 weeks of the HFD. Compared with the BC group, hepatic steatosis slightly decreased in the LD group, whereas remarkably decreased in the HD group.
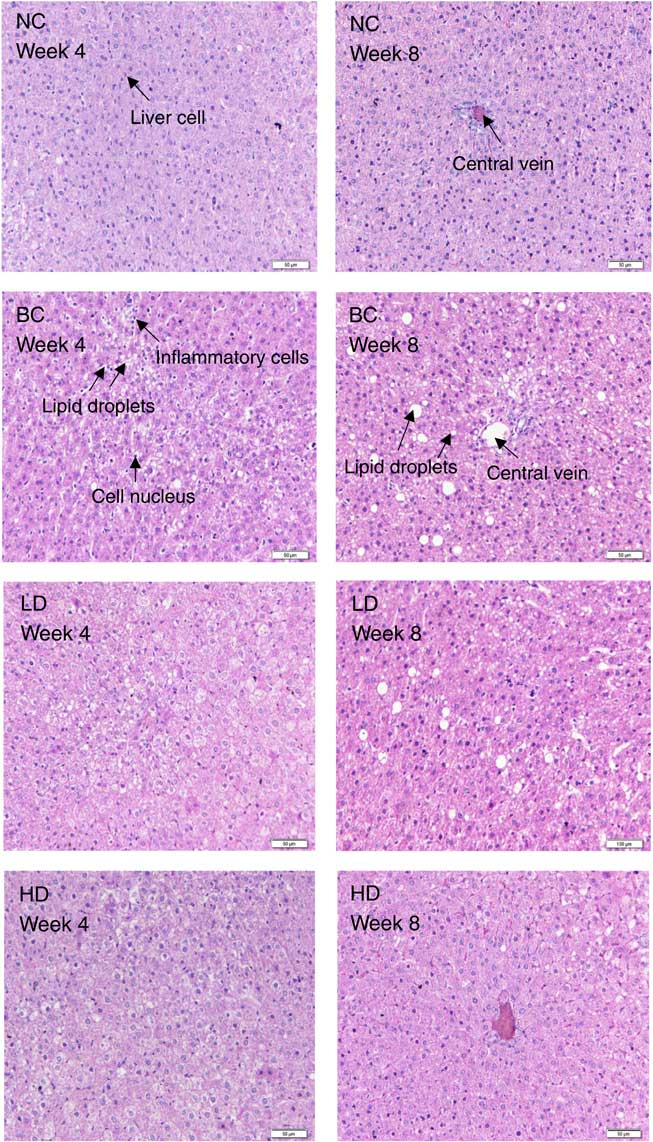
Fig. 2 Effect of diet with different doses of whole-grain highland hull-less barley on haematoxylin–eosin-stained liver sections of high-fat diet Sprague–Dawley rats. NC, normal control group; BC, blank control group; LD, low-dose group; HD, high-dose group.
Bile acid levels in small intestinal contents and faeces
The dry weights of small intestinal contents and faeces were not affected by fat intake (Table 3). The dry weights of faeces in the HD group were significantly higher than other groups. The bile acid levels of small intestinal contents and faeces in the BC and LD groups showed no significant difference after 8-week feeding. However, the bile acid levels in small intestinal contents and faeces of HD groups were significantly higher than those of the other groups. Changes in water content and pH of faeces were also measured (online Supplementary Fig. S1). The faecal water contents of all groups were fluctuating changed. Similarly to water content, the faecal pH of all groups fluctuated. However, the pH of the HD group had the lowest values compared with other groups.
Table 3 Effect of diet with different doses of whole-grain highland hull-less barley on the bile acid levels in small intestinal contents and faeces of high-fat diet Sprague–Dawley rats (Mean values and standard deviations; n 9)
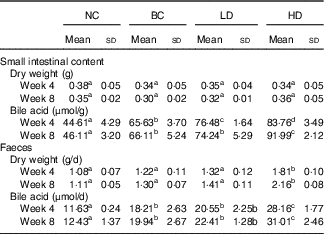
NC, normal control group; BC, blank control group; LD, low-dose group; and HD, high-dose group.
a,b,c,d Mean values in the same row with unlike letters are significantly different (P<0·05).
mRNA levels of key genes in hepatic and ileal lipid metabolism
The integrity of the total RNA extracted from liver was verified by agarose gel electrophoresis images, in which three clear bands (5S, 18S and 28S) were observed for each representative sample (online Supplementary Fig. S2). The specificity of primers was confirmed by melting curves, in which only one dominant peak was observed for each primer (online Supplementary Fig. S3). Changes in mRNA levels of measured genes are shown in Fig. 3. The mRNA levels of ApoB, SREBP-1c and HMG-CoAr in the BC group were significantly higher than those in the NC group. The mRNA levels of measured genes in the LD group showed no significant difference with that in the BC group, except for PPARα levels which were significantly higher than those in the BC group after 4-week feeding. Compared with the BC group, the mRNA levels of AMPKα, LDLR, PPARα and ileal FXR in the HD group were significantly higher, whereas mRNA levels of HMG-CoAr and ASBT were significantly lower. The mRNA levels of CYP7A1 and LXR in the HD group were significantly higher than those in the BC group after 8-week feeding.
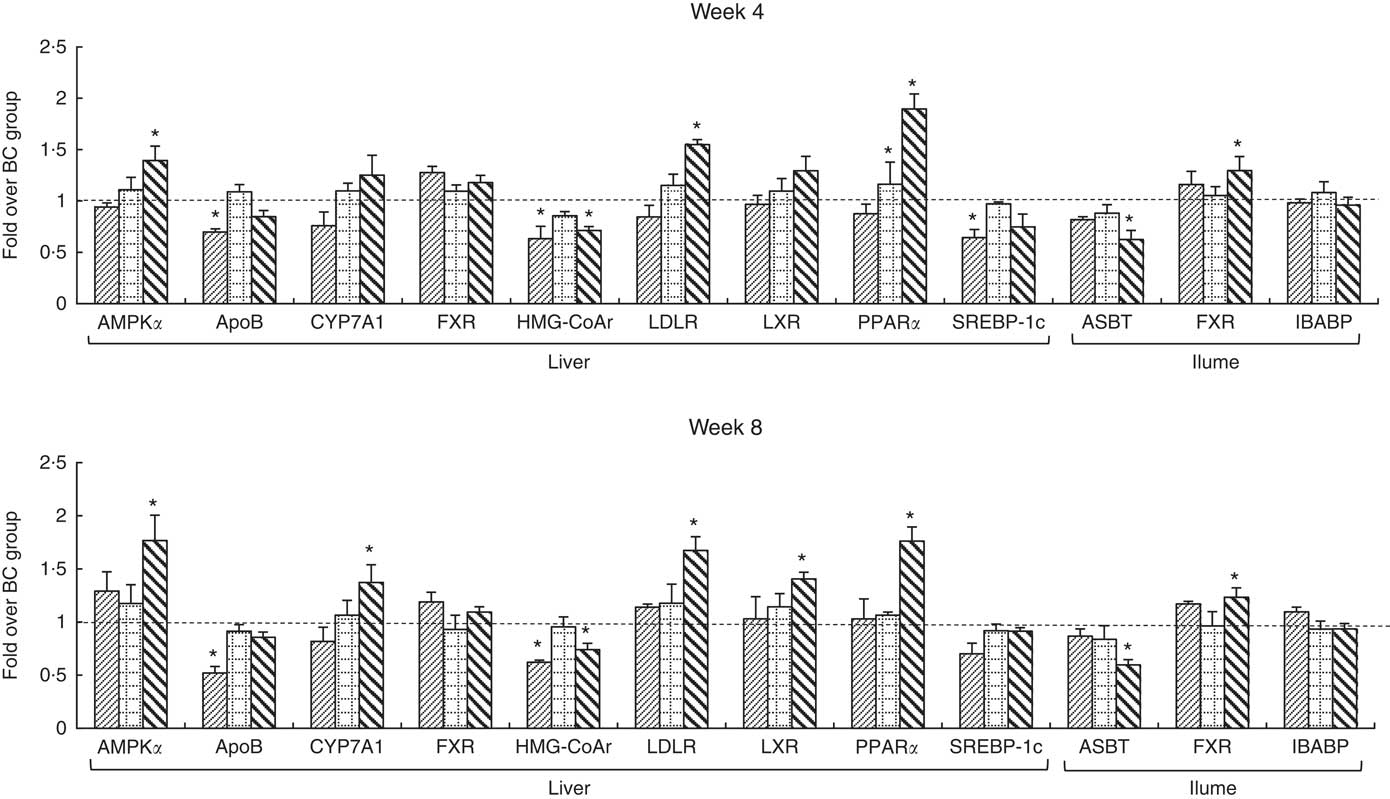
Fig. 3 mRNA levels of hepatic and ileal lipid metabolic regulators. Values are means (n 9) and standard deviations. Each mRNA was normalised to β-actin and is expressed as a relative level to blank control (BC) group. * mRNA levels of regulators were significantly different (P<0·05) compared with the BC group. AMPKα, AMP-activated protein kinase α; CYP7A1, cholesterol 7α-hydroxylase; FXR, farnesoid X receptor; HMG-CoAr, 3-hydroxy-3-methylglutaryl coenzyme A reductase; LDLR, LDL receptor; LXR, liver X receptor; SREBP-1c, sterol regulatory element-binding protein-1c; ASBT, apical sodium-dependent bile acid transporter; IBABP, ileal bile acid-binding protein; , normal control group;
, low-dose group;
, high-dose group.
Protein levels of key genes in hepatic and ileal lipid metabolism
Expressions of hepatic protein (CYP7A1, HMG-CoAr and LDLR) and ileal protein (ASBT and IBABP) in the NC, BC and HD groups after 8-week feeding are shown in Fig. 4. Similar to the RT-PCR results, the expression levels of CYP7A1 and LDLR in the HD group were significantly higher, whereas the levels of HMG-CoAr and ASBT were significantly lower than in the BC group.
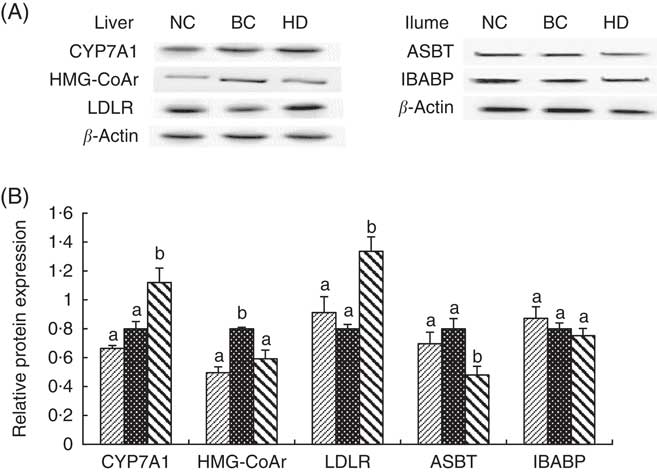
Fig. 4 Effect of whole-grain highland hull-less barley on hepatic protein (cholesterol 7α-hydroxylase (CYP7A1), 3-hydroxy-3-methylglutaryl coenzyme A reductase (HMG-CoAr) and LDL receptor (LDLR)) and ileal protein (apical sodium-dependent bile acid transporter (ASBT) and ileal bile acid-binding protein (IBABP)) expressions after 8 weeks of feeding. Values are means (n 9) and standard deviations. The protein levels in each sample were normalised to the β-actin level. Histograms illustrate the densitometric analysis of protein levels shown in (A) based on β-actin expression (B). NC, normal control group; BC, blank control group; HD, high-dose group. B: , NC;
, BC;
, HD. a,b Mean values of the same index in the three groups with unlike letters are significantly different (P<0·05).
Discussion
To provide insights into the hypocholesterolaemic effect and mechanism of WHLB so as to offer economic significance for its consumption, the hypocholesterolaemic effect of WHLB was investigated systematically in the present study. The 2015–2020 Dietary Guidelines for Americans recommend that at least one-half of all grains consumed should be whole grains( 33 ). A growing body of evidence supports that increased whole-grain consumption is associated with a reduced risk of developing chronic diseases( Reference Zhou, Hergert and Rompato 15 ). Therefore, we replaced all maize starch with WHLB (48·95 %) in the HD group. As a control, the LD group was fed with 10 % WHLB, which was lower than the recommended dose. Our previous study showed that WHLB increased body weight and feed efficiency ratios while decreased the obesity degree of HFD rats( Reference Xia, Li and Ding 21 ). Consistently, results of organ index tests showed that the indexes of liver and abdominal fat in HFD rats decreased significantly after administration of high-dose WHLB. The liver plays a central role in maintaining lipid homoeostasis( Reference Ogata, Tsujita and Hossain 11 ), and increased abdominal fat has been linked to increased CVD risk( Reference Carmina, Bucchieri and Esposito 34 ). These results suggested that WHLB regulated lipid metabolism.
The results of plasma and liver lipid tests showed that administration of high-dose WHLB significantly decreased the TC and LDL-cholesterol levels in the plasma and the TC and the total lipid levels in the liver. Elevated plasma levels of TC and LDL-cholesterol are biomarkers for elevated risk of CVD( Reference Shao, Bartley and Yokoyama 2 ). Ishimwe et al. ( Reference Ishimwe, Daliri and Lee 35 ) reported that 1 % reduction in TC and 1 % reduction in LDL-cholesterol translate to a 2 % and 1 % decrease in heart disease risk. The lipid-lowering activity of WHLB was consistent with previously evaluated barley( Reference Hoang, Houng and Jun 16 , Reference Kim, Turowski and Anderson 17 , Reference Sindhu and Khetarpaul 36 ). The lipid regulation effect of WHLB was further confirmed through hepatocyte morphologic observation. The hepatic fat-droplet accumulation decreased after consuming WHLB diet, particularly at high doses. However, WHLB diet did not significantly affect TAG levels, which was in line with results from barley( Reference Hoang, Houng and Jun 16 ) and oat diet( Reference Zhou, Hergert and Rompato 15 ), whereas the mechanism should be further studied. HDL-cholesterol levels in the HD group were significantly higher than those in the BC group after 8-week feeding. High levels of functional HDL in plasma protect against atherosclerotic coronary disease( Reference Leiva, Verdejo and Benítez 37 ). Kim et al. ( Reference Kim, Turowski and Anderson 17 ) reported that HDL-cholesterol levels in Syrian Golden hamsters with HFD were slightly increased after administrating diets that contain bread of whole-grain barley. Meanwhile, Sindhu & Khetarpaul( Reference Sindhu and Khetarpaul 36 ) reported that barley diet did not significantly affect HDL-cholesterol in mice. Hoang et al. ( Reference Hoang, Houng and Jun 16 ) reported that the HDL-cholesterol levels were slightly decreased in HFD mice after a whole-grain barley diet. These differences may be attributed to the varieties of grains or experimental animal species used. Lipoprotein metabolism differs between different experimental model species. For example, mice show up to 40-fold higher LDL clearance by the liver compared with humans, and mice carry most of their plasma cholesterol in HDL particles( Reference van der Wulp, Verkade and Groen 38 ).
Cholesterol is mainly eliminated from the body via conversion to bile acids( Reference Hoang, Houng and Jun 16 ). Bile acid levels in small intestinal contents and faeces were investigated accordingly. Results showed that diet with high-dose WHLB significantly increased the bile acid levels in small intestinal contents and faeces of HFD rats. These results suggested that WHLB may regulate lipid metabolism by increasing bile acid excretion, which is consistent with the mechanism of barley( Reference Hoang, Houng and Jun 16 , Reference Dongowskia, Hutha and Gebhardta 39 , Reference Zhong, Marungruang and Fåk 40 ). β-Glucan and dietary fibre compounds were reported to delay bile acid reabsorption and facilitate faecal bile acid excretions( Reference Kim, Turowski and Anderson 17 – Reference De Angelis, Montemurno and Vannini 19 ). Martinez-Floresa et al. ( Reference Martinez-Floresa, Chang and Martinez-Bustos 41 ) reported that hypocholesterolaemic effect of dietary fibre attributed primarily to soluble dietary fibre. WHLB used in the current study has relatively high content of β-glucans and dietary fibre, particularly soluble dietary fibre, compared with other grains( Reference Yalçın, Çelik and Akar 42 – Reference Zhang, Wang and Chen 44 ). Therefore, the hypocholesterolaemic effect of WHLB may be partly attributed to its high content of β-glucan and (soluble) dietary fibre. Undigested dietary fibre will be fermented in the caecum, which is a site of vigorous microbial activity in rats, thereby yielding various products( Reference Kishida, Nogami and Ogawa 45 ). The values of caecum indexes and faecal dry weights in HD groups were significantly higher, whereas the faecal pH was markedly lower compared with those in the BC group, thereby corresponding to the changes in bile acid.
To study the molecular mechanism by which WHLB affects the lipid metabolism of the HFD rats, the mRNA levels of key genes in hepatic and ileal lipid metabolic were measured. AMPKα, HMG-CoAr and SREBP-1c were proven to be involved in hepatic cholesterol synthesis. AMPK regulates lipid metabolism and inhibits cholesterol synthesis by inducing the inhibitory phosphorylation of HMG-CoAr. AMPK can also inhibit fatty acid synthesis by inducing the inhibitory phosphorylation of SREBP-1c( Reference Jeon 4 ). HMG-CoAr is the rate-limiting enzyme for cholesterol synthesis( Reference Chen, Wang and Li 5 ). Meanwhile, SREBP-1c is a transcription factor that modulates the expression of a large number of genes involved in the synthesis of cholesterol( Reference Ji, Zhao and Leng 6 ). RT-PCR results showed that the mRNA levels of HMG-CoAr and SREBP-1c were significantly up-regulated in the BC group, which indicated that HFD stimulated cholesterol synthesis. These results were corresponded with the high levels of plasma and liver lipid of the BC group. After administration of high-dose WHLB, the mRNA levels of HMG-CoAr significantly decreased, whereas the AMPKα levels significantly increased. These results suggested that the low TC concentrations in the HD group may be due to the down-regulation of HMG-CoAr, which in turn may be partly caused by the up-regulation of AMPKα. The down-regulation of HMG-CoAr after WHLB intake was also noted with the intake of barley( Reference Hoang, Houng and Jun 16 ).
When the hepatic cholesterol synthesis increased, the liver secretes cholesterol with ApoB and increases the circulating LDL( Reference Kim, Hong and Jeon 7 ). Increased level of LDL, which is the principal atherogenic lipoprotein in the blood, promotes cholesterol accumulation in the artery wall, thereby initiating atherosclerosis( Reference Tall and Yvan-Charvet 46 ). Therefore, the significant up-regulation of ApoB in the BC group may be responsible for the high LDL-cholesterol levels and abdominal fat indexes. Conversely, LDLR mediates endocytosis of LDL and increases the reabsorption of LDL, decreasing synthesis of liver cholesterol( Reference Kemper, Srivastava and King 8 , Reference Levinson and Wagner 9 ). The significant up-regulation of LDLR in the HD group may be responsible for the low LDL-cholesterol levels and abdominal fat indexes. HDL plays a central role in transporting cholesterol from extrahepatic tissues to the liver for catabolism of cholesterol to bile acids. Thus, HDL is thought to contribute to removing cholesterol from peripheral tissues( Reference Ogata, Tsujita and Hossain 11 ). Regulator of HDL biogenesis is up-regulated by the transcription factor LXR, and HDL expression is further enhanced by PPARα activation( Reference Ogata, Tsujita and Hossain 11 ). Compared with the BC group, the significantly higher mRNA levels of PPARα in the LD group may be responsible for the significantly lowered abdominal fat indexes at week 4. The mRNA levels of LXR and PPARα in the HD group were higher than those in the BC group, and the difference became significant after 8-week feeding. Consistent with our results, Ogata et al. ( Reference Ogata, Tsujita and Hossain 11 ) concluded that PPAR and LXR are involved in HDL biogenesis in a cooperative signal transduction pathway. The concurrent up-regulation of LXR and PPARα may be responsible for the high HDL-cholesterol levels in the HD group.
In the liver, CYP7A1 catalyses the rate-limiting step in the bile acid synthetic pathway( Reference Musso, Gambino and Cassader 47 ). The mRNA levels of CYP7A1 in the HD group were significantly higher than those in the BC group after 8-week feeding. Musso et al. ( Reference Musso, Gambino and Cassader 47 ) reported that LXR can increase CYP7A1 transcription; therefore, the CYP7A1 up-regulation may be attributed to LXR up-regulation. Consistent with our results, Choi et al. ( Reference Choi, Kim and Jung 48 ) and Yang et al. ( Reference Yang, Kim and Lee 49 ) reported that barley β-glucan diet significantly increased the mRNA level of CYP7A1. Hoang et al. ( Reference Hoang, Houng and Jun 16 ) reported that hepatic CYP7A1 expression was surprisingly decreased after barley diet, and they partly attributed the effect to the up-regulation of hepatic FXR. FXR, a ligand-activated transcription factor belonging to the adopted orphan receptor, plays an important role in maintaining the health of the liver and the intestines( Reference Zhang, Osaka and Tsuneda 50 ). Hepatic FXR has been proposed to play a central role in the feedback repression of the CYP7A1 gene( Reference Moschetta 12 ). However, the negative correlation between FXR and CYP7A1 was uncertainty( Reference Park and Park 28 ). Conversely, Musso et al. ( Reference Musso, Gambino and Cassader 47 ) reported that FXR activates the bile acid synthesis by inducing CYP7A1. Bile acids are reabsorbed by the ASBT at the end of the small intestine (ileum part) and transferred from the apical to the basolateral membrane by the IBABP( Reference Fu and Klaassen 13 ). FXR was coupled to reduce ASBT expression, thereby resulting in decreased intestinal absorption of bile acids( Reference Hoang, Houng and Jun 16 ). ASBT is almost exclusively expressed in the terminal section of the ileum( Reference Out, Patankar and Doktorova 51 ). Therefore, the mRNA levels of ASBT, FXR and IBABP in the ileum were investigated. The significantly decreased level of ASBT after a diet with a high dose of WHLB, which may be caused by the significantly increased level of ileal FXR, was consistent with the reported effect of barley diet by Hoang et al. ( Reference Hoang, Houng and Jun 16 ). Therefore, the CYP7A1 up-regulation and ASBT down-regulation may be responsible for the high content of bile acid in the small intestine and faeces after WHLB diet.
We only investigated the protein levels of CYP7A1, HMG-CoAr, LDLR, ASBT and IBABP because of the limited mature experimental antibody. The protein levels of these biomarkers were consistent with their mRNA levels, thereby confirming the validity of the RT-PCR analysis. We only studied the mRNA and protein levels of several key genes related to hepatic and ileal lipid metabolism. However, the lipid regulatory mechanism of diet involves numerous proteins( Reference Domínguez-Avila, Alvarez-Parrilla and López-Díaz 52 ). Therefore, proteome analysis should be performed in future studies to comprehensively investigate the lipid regulatory mechanism of WHLB.
In conclusion, high dose of WHLB has a hypocholesterolaemic effect, and the molecular mechanism of this effect may include inhibition of cholesterol synthesis through up-regulation of AMPKα expression and down-regulation of HMG-CoAr expression; stimulation of cholesterol conversion to bile acids through up-regulation of CYP7A1 expression; decrease cholesterol accumulation in peripheral tissues through up-regulation of expression of LDLR, LXR and PPARα; inhibition of bile acid reabsorption through up-regulation of ileal FXR expression and down-regulation of ASBT expression.
Acknowledgements
This work was financially supported by the Science and Technology Support Demonstration Project of Chongqing (CSTC2014JCSF-JCSSX004).
Contributions of authors are as follows: X. X. and J. K. designed the study; X. X., J. S. and G. L. performed the experiments; G. L. and J. Z. analysed the data; X. X. and G. L. wrote and revised the manuscript. All authors reviewed the manuscript.
The authors declare that there are no conflicts of interest.
Supplementary material
For supplementary material/s referred to in this article, please visit https://doi.org/10.1017/S0007114518000831