Introduction
The ice streams draining the West Antarctic ice sheet into the Ross and Ronne Ice Shelves have been studied intensively, but relatively little is known about the ice streams and glaciers draining the ice sheet through the Marie Byrd Land coast. The most conspicuous ice streams in that area arc Pine Island and Thwaites Glaciers, which lack a buttressing ice shelf at their termini. They have been studied by Reference HughesHughes (1981), Reference Crabtree and DoakeCrabtree and Doake (1982), Reference Lindstrom and HughesLindstrom and Hughes (1984), Reference Lindstrom and TylerLindstrom and Tyler (1984) and Reference Ferrigno, Lucchitta, Mullins, Allison, Allen and GouldFerrigno and others (1993), but little is known about the glaciers and ice shelves west of Thwaites Glacier along the Marie Byrd Land coast. The present study concerns velocities on ice tongues and ice shelves in that area (Fig. 1). It is part of a more comprehensive mapping project and inventory of all glaciological features along the Marie Byrd Land coast and of changes that have occurred there within the last 20 years. Here, we present velocities obtained for Smith Glacier, which empties into an ice-shelfless part of the Amundsen Sea immediately west of Thwaites Glacier, and for the Dotson Ice Shelf at the mouth of Kohler and Horrall Glaciers on the Walgreen Coast (Reference Geological SurveyU.S. Geological Survey, 1968).

Fig. 1. Index map of investigated area in Marie Byrd Land, Antarctica.
Method
Large crevasses in the floating part of glaciers or ice shelves commonly retain their identity over many years and, by measuring their displacement on sequential satellite images, their average velocities for a given time interval can be determined. For our measurements, we digitally co-register the images by using a minimum of three well-dispersed fixed points such as nunataks or ice walls. We then use an interactive-display technique to match and align the crevasse patterns displaced with time, and we calculate the velocities from the distances moved. To obtain the distribution of average velocities over the length of the glacier tongues, we also measure the distance from the location of each point on the earlier image of a pair to a base line drawn perpendicular to glacier movement and ideally lying on the grounding line; where the grounding line is complex, the base line may only approximate its position. Because the velocity field may also change across the glacier tongues, we divide them into several segments (identified by upper-case letters on our images), and we subdivide the segments into longitudinal paths (identified by P1, P2, etc.). To obtain an estimate of the spread of measured points, we calculate a regression line and the mean absolute deviation of the points about this line. The measurement results are shown in graphs that display average velocities per given time interval versus the distance from the base line for all points in each field. To give an indication of the general velocity of an ice tongue or ice shelf, we also calculate the mean of all the average velocity values per path and per segment, and we give the results in tables.
Table 1. Landsat images used in this study

Details of our method have been described by Reference Lucchitta, Mullins, Allison and FerrignoLucchitta and others (1993).
In this study, the velocities were measured on four co-registered Landsat images (Table 1). The Multispectral Scanner (MSS) images were obtained from Computer Compatible Tapes (CCTs), and the Thematic Mapper (TM) images from scanned transparencies because of the high cost of Landsat (TM) computer tapes. For the present study, we co-registered three of the images to the scanned 1988 Landsat 4 Thematic Mapper (TM) image. Thus, we were able to obtain average velocities for the time intervals 1972–88 (Fig. 2), 1973–88 (Fig. 3) and 1988–90 (Fig. 4).

Fig. 2. Dotson Ice Shelf and displacement vectors of its crevasse patterns and distances moved during 15.0 year interval (December 1972- January 1988). Landsat 1 AISS image 1160-14554, 30 December 1972.

Fig. 3. Dotson Ice Shelf; Smith Glacier and displacement vectors of their crevasse patterns and distances moved during 15.0 year interval (January 1973 January 1988). Landsat 1 MSS image 1177-14500, 16 January 1973. Orientation, scale and feature names as in Figure 2.
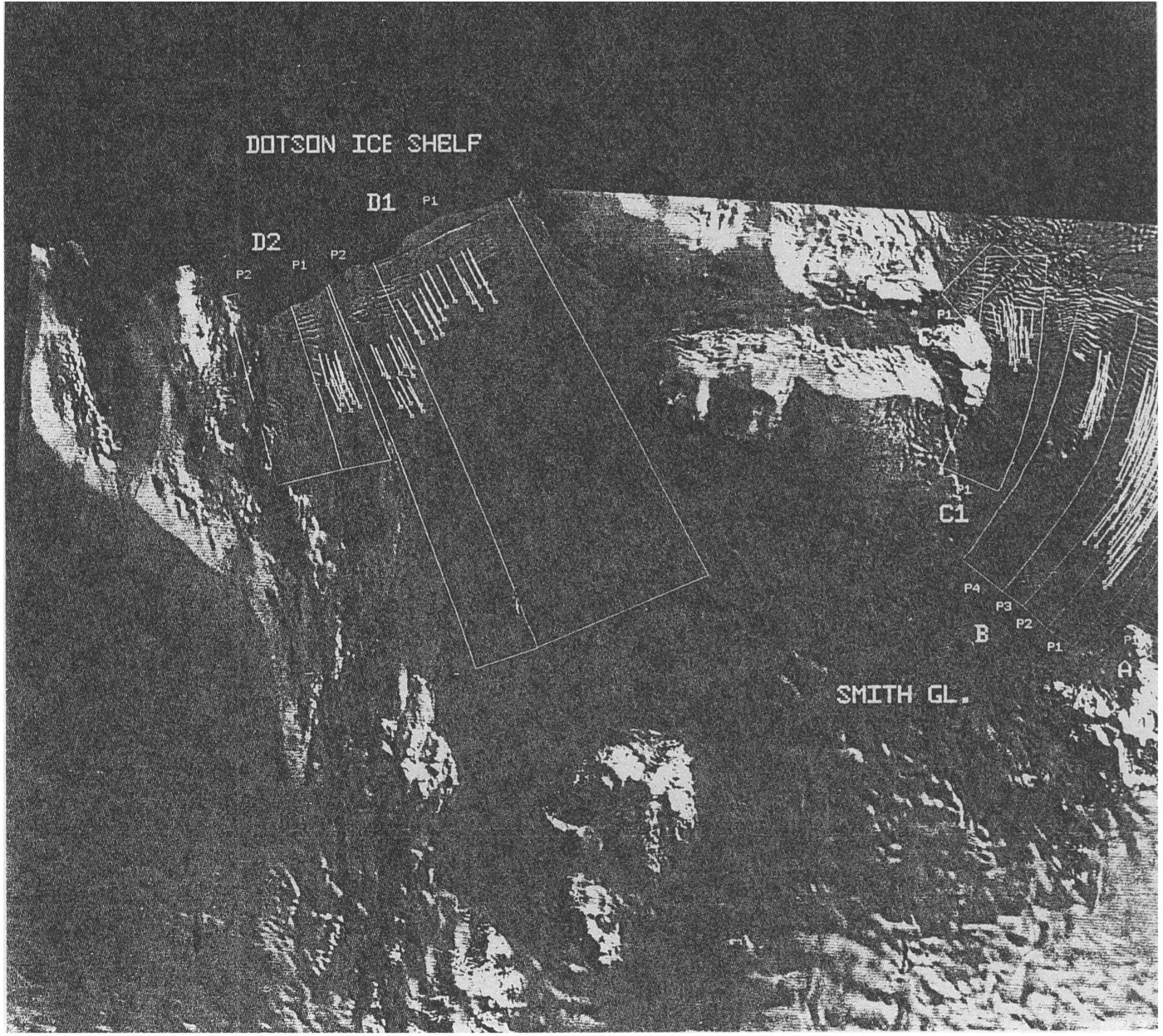
Fig. 4. Dotson Ice Shelf, Smith Glacier and displacement vectors of their crevasse patterns and distances moved during 2.1 year interval (January 1988-January 1990). Landsat 4 TM image 41996-14580, 2 January 1988. Orientation, scale and feature names as in Figure 2.
Our previous investigations (Reference Lucchitta, Mullins, Allison and FerrignoLucchitta and others, 1993) have shown that errors in measured average velocities may be as little as 0.02 km year 1 if (I) the time interval is longer than 10 years, (2) the average velocity is higher than 0.5 km year 1, (3) co-registration points arc well dispersed and surround the ice tongue to be measured, and (4) the image pair includes a Landsat 4 or 5 image. Landsat TM images are accurate to sub-pixel levels, thus meeting National Horizontal Map Accuracy standards for scales as large as 1: 100000 (Reference Borgeson, Batson and KiefferBorgeson and others, 1985; Reference Welch, Jordan and EhlersWelch and others, 1985). For this study, we used the same TM image as a base for co-registration; therefore, all the measurements were made at the same scale. The earlier time intervals (1972–88 and 1973–88) exceed 10 years; most average velocities approach or exceed 0.5 km year-1; at least eight well-dispersed co-registration points were used to match the images and they were co-registered to a Landsat 4 TM image. The time interval of 1988–90 is much shorter but both images are of the higher resolution of Landsat TM. For our situation, we predict the measurements are accurate to about 0.05 km year-1.
We also investigated the residual pixel errors remaining after co-registering the 1973 MSS to the 1988 TM image, because internal distortions causing possibly substantial errors were introduced in the Landsat 1, 2 and 3 MSS images due to variations in scanning-mirror velocity and roll, pitch and yaw of the spacecraft. Generally, to minimize errors, we use only co-registration points with residual errors that are less than two pixels, or 100 m on the ground at the approximate 1 : 1000 000 scale of the images. After co-registering our images, we measured the residual errors of 36 randomly selected points dispersed across the images and found the errors are no more than one pixel or an average of <0.25 pixels (< 15 m on the ground).
Misidentification of crevasse patterns remains another potential source for error. The greater the scatter in our plots of velocities versus distance to the base line, the more likely it is that measurement errors occurred. Also, shorter time intervals generally cause greater scatter, because even slight imprecisions in measurement are proportionately more noticeable over short times. Our calculated mean absolute deviation about the regression line gives an indication of measurement errors; however, locally the spread about the regression line may reflect real velocity variations across the width of the ice tongue or ice shelf rather than errors. We used two mean deviations about the regression line to eliminate points of obvious faulty measurements from our statistics.
Results
Smith Glacier
Smith Glacier empties into the Amundsen Sea immediately west of the Thwaites Glacier ice tongue (Fig. 1). The Smith and Thwaites Glacier ice tongues appear to be remnants of a former ice shelf that extended from Bear Peninsula in the west toward Pine Island Bay in the east (Reference Geological SurveyU.S. Geological Survey, 1968). Smith Glacier drains a major part of the West Antarctic ice sheet (Reference SwithinbankSwithinbank, 1988) between Mount Takahe and the Executive Committee Range. Bedrock in the Smith Glacier and Dotson Ice Shelf region lies near 500 m (Reference DrewryDrewry, 1983).
We subdivided the Smith Glacier ice tongue into four segments with one to four paths per segment (Figs 3 and 4). Displacement of crevasse patterns on the Smith Glacier ice tongue was measured for two time intervals (1973–88 and 1988 90). The velocity of path 1 of segment Smith A (Figs 4 and 5a; Table 2) was slightly higher than velocities on other ice shelves (Reference Lucchitta, Mullins, Allison and FerrignoLucchitta and others, 1993). This path is not part of the main glacier ice tongue and has a very small intake area. Segment Smith B covers the main part of the ice tongue (Figs 3 and 4). Paths 1-3 were relatively fast; path 4 was slowed by several small ice rises in its path (Fig. 5b and c; Table 2). Comparison of the graphs (Fig. 5b and c) and of the general velocity values (Table 2) shows an increase in velocity of about 0.1 km year-1 for the 1988–90 time interval.

Fig. 5. Average annual velocity of Smith Glacier plotted against distance to base line. a. 2.1 year interval, 1988–90; b. 15.0 year interval, 1973–88; c. 2.1 year interval, 1988–90; d. 15.0.year interval, 1973–88; e. 2.1 year interval, 1988–90;f 2.1 year interval, 1988–90 (note change in scale).
Table 2. Average velocities

Path 1 of Smith Cl (Figs 3, 4, 5d and e; Table 2) has a negative slope on the graph representing 1973–88 (Fig. 5d). This apparent decrease in velocities toward the calving front can be readily explained: the proximal measurements were made in a faster part of the ice tongue near the main glacier, and the distal measurements include points in a slower part of the tongue near the shear zone with the adjacent Bear Peninsula (Fig. 3). Smith C1 is slower than Smith B, because the C1 segment is impeded by the base of Bear Peninsula (Figs 3 and 4). The 1988–90 average velocities are about 0.15 km year 1 higher than the 1973–88 velocities, despite a cluster of low velocities measured in a shear zone next to the peninsula and included in the averages (Figs 4 and 5e; Table 2). The 1990 image contains some cloud cover in this area; the partial obscuration could cause errors in our measurements due to misidentification of crevasse features on this image. However, the systematic trend in velocities derived from the 1988–90 image set (Fig. 5e), along with a careful check of the features in our initial data collection, make this possibility less likely. Also, the later measurements were made closer to the calving front, which had receded between 1973 and 1988: the different position of the front may explain the higher velocities of the later time interval.
Path 1 of Smith C2 (Figs 4 and 5f; Table 2) was measured only for the 1988–90 time interval. It is on a short ice tongue between ice rises of Bear Peninsula, and its relatively high velocity probably reflects the break-up of the ice tongue near the terminus; this velocity is similar to those near the terminus of Smith C1 (1988–90). It is possible, though unlikely, that these measurements may also be in error because of the poor viewing conditions.
Dotson Ice Shelf
The Dotson Ice Shelf is partly fed by branches of Smith Glacier (Reference Geological SurveyU.S. Geological Survey, 1968). The feeders cross a low swell to form the eastern part of the ice shelf and they spill through a gap in the Kohler Range to form the central part of the ice shelf Thus, the drainage basin for part of the Dotson Ice Shelf is the same as that of Smith Glacier. The western part of the ice shelf drains the Martin Peninsula ice rises (Figs 2, 3 and 4).
The Dotson Ice Shelf velocities were measured in three time intervals: 1972–88 (Fig. 2), 1973–88 (Fig. 3) and 1988–90 (Fig. 4). We subdivided the ice shelf into two segments and each segment into two paths.
The average velocities of Dotson D1 (Figs 2, 3, 6a and b: Table 2) are for almost identical time intervals and are indeed very similar. The average velocity for the 1988–90 time interval (Figs 4 and 6c; Table 2) also remained the same. The scatter in the Dotson D1, path 1 plots (Fig. 6a, b and c) probably reflects the width of the path, which covers nearly half the ice shelf Therefore, the spread in velocities may represent faster ice movement near the center and slower movement toward the margins of this ice shelf.

Fig. 6. Average annual velocity of the Dotson Ice Shelf plotted against distance to base line. a. 15.0 year interval, 1972–88; b. 15.0 year interval, 1973–88; c. 2.1 year interval, 1988–90; d. 15.0 year interval, 1973–88 (note change in scale); e. 2.1 year interval, 1988–90 (note change in scale).
The Dotson D2 ice-shelf segment has low velocities, in keeping with ice shelves elsewhere that are not fed by major ice streams (Figs 3, 4, 6d and e; Table 2). The somewhat higher speeds in the earlier image set may be real but they may also reflect the fact that relatively few points were sampled. Also, the distances measured on the later set are short (0.5 km), which makes our velocity uncertainties proportionately larger for this data set. The slope on the plotted points is low, indicating little increase in velocity toward the terminus of the ice shelf. This observation may reflect the location of this part of the ice shelf in a small bay within Martin Peninsula (so that it is constrained on both sides), as well as the relatively small size of the intake area for this part of the ice shelf.
Discussion
Our data support the premise that the higher average velocities of the Smith Glacier ice tongue in the 1988–90 time interval reflect a true increase in velocities (Fig. 7a). If the higher velocities were an artifact of our procedure or of the images, similar increases should be noted elsewhere. Yet, even though the same images were used for the Dotson Ice Shelf measurements, no equivalent increases were noted there (Fig. 7b). Furthermore, Figure 7a clearly shows a convergence of the velocities toward the base line. This observation suggests that the velocities were similar near the grounding line in both the earlier and later time intervals, reflecting no change in discharge from the ice sheet. On the floating ice tongue, however, the velocities show an increase toward the calving front for the later interval.

Fig. 7. a. Average annual velocity of paths 1-3, segment B, on Smith Glacier plotted against distance to base line. Comparison of movement of 1973–88 (15.0 year interval) and 1988–90 (2.1 year interval). Note the convergence toward the grounding line of the regression lines of the two data sets. b. Average annual velocity if segment D1 on Dotson Ice Shelf plotted against distance to base line. Comparison of movements of 1988–90 (2.1 year interval), 1972–88 (l5.0 year interval) and 1973–88 (15.0 year interval).
What caused this increase in velocities? Figures 2 and 3, and adjacent Landsat images (Thwaites Glacier area; Reference Ferrigno, Lucchitta, Mullins, Allison, Allen and GouldFerrigno and others, 1993), show that in 1972 and 1973 the Amundsen Sea in front of the Smith Glacier ice-tongue terminus and between the Thwaites and Smith Glacier ice tongues was densely packed with large floating icebergs, whereas in 1990 the area was virtually clear (Fig. 8). By 1984, most of the icebergs were still in the vicinity but a large, rounded iceberg had broken off Smith Glacier. By 1988, most of the icebergs had drifted away, the rounded iceberg had rotated counter-clockwise and drifted out about 15km and a second large iceberg of more rectangular shape had broken off the ice tongue. By 1990, the rounded iceberg had moved 60 km out to sea, away from the 1988 position (Fig. 8). The distance of drift of the rounded iceberg in just 2 years corresponds to that of the Thwaites Glacier iceberg tongue during the same time interval (Reference Ferrigno, Lucchitta, Mullins, Allison, Allen and GouldFerrigno and others, 1993). Perhaps the rotation and drifting were aided by strong winds that were reported for the general area by Reference Kellogg and KelloggKellogg and Kellogg (1987).
The increase in velocity of the Smith Glacier ice tongue is probably related to this clearing of icebergs in front of the ice tongue. Until 1984, the Smith Glacier ice tongue was buttressed by tightly packed icebergs, possibly welded by multi-year sea ice and wedged between the Thwaites Glacier ice tongue (seen in adjacent Landsat images; in Reference Ferrigno, Lucchitta, Mullins, Allison, Allen and GouldFerrigno and others, 1993, fig. 2) and pinning points of Bear Peninsula. After 1984, the area apparently began to clear. In contrast to observations on earlier images, in January 1988, open water locally covered by thin sea ice was present next to the Smith Glacier terminus (Figure 4 and adjacent Landsat images; not shown). Sometime after 1984, the ice tongue apparently began to spread, probably through the opening of crevasses, resulting in the apparent increase of velocities detected in the 1988–90 time interval.

Fig. 8. Sketch map showing position of the Smith Glacier ice-tongue terminus in 1972 (dotted line). The thin solid lines enclose icebergs in front of the terminus in 1972. Position of Smith Glacier ice-tongue terminus in 1990 (dashed line). Region in front of the terminus was clear of icebergs in 1990. Stippled iceberg was part of the Smith Glacier ice tongue in 1972 and moved to indicated positions in 1984, 1988 and 1990.
The front of the Smith Glacier ice tongue, and of a small ice tongue between the Smith and Thwaites Glacier ice tongues, receded by about 10km between 1984 and 1990. During this time interval, the Thwaites Glacier ice tongue lost about 60 km of ice due to calving (Reference Ferrigno, Lucchitta, Mullins, Allison, Allen and GouldFerrigno and others, 1993). Between 1973 and 1988, the front of the Dotson Ice Shelf receded 5 7 km. The timing of these calving events may be coincidental. On the other hand, the loss of icebergs and calving of ice tongues is also consistent with a general decrease of shelf ice in the area. Reference Kellogg, Kellogg and HughesKellogg and others (1985) stated that "it appears that the entire Pine Island Bay region experiences a negative net mass balance, primarily from sublimation". Perhaps we are seeing the last stages of an ice shelf that purportedly filled the entire Pine Island Bay only 100years ago (Reference Kellogg and KelloggKellogg and Kellogg, 1987). Perhaps, also, we are observing a process similar to the disintegration of the Wordie Ice Shelf on the Antarctic Peninsula (Reference Duake and VaughanDuake and Vaughan, 1991). There, the disintegration of shelf ice was also accompanied by increased velocities and facilitated by the retreat of the calving front away from pinning points. Doake and Vaughan attributed the disintegration of the Wordie Ice Shelf to increased air temperatures in the region, perhaps coupled with increased ocean temperatures. Ablation and meltwater were supposed to have contributed to the formation of crevasses and rifts, thus facilitating the drifting away of ice blocks.
Summary
The Dotson Ice Shelf velocities changed little over the time period investigated (1972–90) but calving reduced its front by 5–7 km. In contrast, the Smith Glacier ice tongue increased in velocity by about 0.1 km year-1, and calving reduced its front by about 10km. The increase in velocity appears to be confined to the floating part of the glacier below the grounding line. During the mid to late 1980s, the bay in front of the Smith Glacier ice tongue lost partly pinned icebergs that appeared to have buttressed the ice tongue. This process may have freed the calving front and permitted the spreading of the ice tongue, resulting in the apparent increase in velocity. The cause of the general loss of ice in this region is unknown but it may be related to changes in wind direction or warming of the air or ocean.
Acknowledgements
We are grateful for helpful reviews by H. H. Kieffer and D.J. Chadwick, and two anonymous reviewers. J. M. Barrett helped in developing computer programs. The research was funded by the U.S. Geological Survey's Global Change and Climate History Program.