Domestic animals, just like man, are frequently exposed to oxidative stressReference Aurousseau1, involving an overproduction of reactive oxygen species that cause oxidative damage to all macromolecules within the cellReference Moller, Wallin and Knudsen2. In domestic animals, oxidative stress has been associated with the deterioration of many physiological functions including growth and reproduction as well as immunityReference Miller and Brzezinska-Slebodzinska3. The oxidative deterioration of lipids (or lipoperoxidation) is particularly involved in this phenomenon since lipids are the macromolecules that are the most susceptible to peroxidative processes, especially when they are rich in PUFAReference Takahata, Monobe, Tada and Weber4.
Dietary fat supplementation was proposed 40 years ago for ruminants in order to increase, at a low cost, the energetic density of dietsReference Clinquart, Micol, Brundseaux, Dufrasne and Istasse5. This strategy was improved by the use of vegetal sources rich in PUFA from the n-3 family (n-3 PUFA). Indeed, these fatty acids are believed to be beneficial for human health and by giving them to domestic animals it is possible to increase the content of n-3 PUFA in animal products and therefore to improve their nutritional valueReference Wood and Enser6, Reference Bauchart, Gladine, Gruffat, Leloutre, Durand, Hocquette and Gigli7. However, since n-3 PUFA are highly susceptible to peroxidation both in plasma and tissuesReference Frémont, Gozzélino, Franchi and Linard8, Reference Scislowski, Bauchart, Gruffat, Laplaud and Durand9, it appeared that a supply of antioxidants should be recommended to preserve the health of animals and the oxidative stability of their productsReference Wood and Enser6.
Vitamin E is a synthetic antioxidant commonly used in animal nutrition and the bioefficiency of vitamin E supplement to reduce lipoperoxidation in ruminant products has been largely demonstratedReference Wood and Enser6. However, its ability to limit lipoperoxidation is not sufficient when n-3 PUFA intake is increasedReference Allard, Kurian, Aghdassi, Muggli and Royall10, Reference Miret, Saiz and Mitjavila11. This could be due to its restricted site and mechanism of action but it has also been reported that supplementation with vitamin E is efficient until a certain threshold, beyond which vitamin E ingested is catabolized or eliminated in faeces and urineReference Aurousseau1. Moreover, vitamin E has many other biological effects, including modulation of gene expression or inhibition of protein kinase CReference Azzi and Stocker12, and a pro-oxidant action was also observed in vitro Reference Mukai, Sawada, Kohno and Terao13. On the basis of these observations, it is likely that other types of antioxidants, given alone or in association with vitamin E, could be more efficient and less deleterious than the use of megadoses of vitamin E alone to improve the antioxidant protection of animals.
Recent search for new antioxidants focused on natural molecules to satisfy consumer concerns over safety and toxicity. Among natural antioxidants that could be used in animal nutrition, we hypothesized that plant extracts rich in polyphenols (PERP) would be good candidates since polyphenols are abundant in many plants and PERP can then be readily produced at low cost. Moreover, in contrast with vitamin E, polyphenols exhibit various antioxidant propertiesReference Bravo14. Actually, PERP, such as herbs or spices, are already used as antioxidant additives in the food industry, suggesting that this natural approach is relevantReference Andersen, Lauridsen, Skibsted, Johnson and Williamson15, Reference Grün, Ahn, Andrew and Lorenzen16. However, the use of PERP as dietary antioxidants to preserve both the health of animals and the oxidative stability of their products needs further investigations, since studies conducted in rodents and human subjects showed that many polyphenols are poorly absorbed, intensively metabolized or rapidly excretedReference Manach, Scalbert, Morand, Rémézy and Jimenez17. Feeding trials conducted in poultry nevertheless reported beneficial effects of oregano extract in muscle tissueReference Papageorgiou, Botsoglou, Govaris, Giannenas, Iliadis and Botsoglou18–Reference Giannenas, Florou-Paneri, Botsoglou, Christaki and Spais20 but the results cannot be directly extrapolated to ruminants because of the bacterial fermentation processes occurring in rumenReference Jouany21, which could greatly affect the bioavailability of PERP and subsequently their in vivo antioxidant capacity.
The aim of the present study was to investigate the bioavailability and the antioxidant capacity of PERP in the plasma of ruminants made highly susceptible to lipoperoxidation. For this purpose, four PERP, known for their bioefficiency in ratsReference Gladine, Morand, Rock, Bauchart and Durand22, Reference Gladine, Morand, Rock, Gruffat, Bauchart and Durand23, were given, as a single acute dose, directly into the rumen of sheep. Parameters related to bioavailability and antioxidant capacity of PERP were measured in the plasma collected every 3 h during a period of 30 h after the PERP administration.
Materials and methods
Animal management
All experiments were conducted in accordance with the national legislation on animal care (certificate authorization to experiment on Living Animal No. 7740, Ministry of Agriculture and Fish Products, Paris, France). Five castrated Texel sheep (18 months old, 50 (sem 4) kg body weight) were used in a 5 × 5 Latin square design. They were surgically fitted with a rumen cannula (polyvinyl chloride; 60 mm o.d.) and a T-shaped cannula (silicone rubber, 17 mm o.d) in the proximal duodenum. After the post-surgical period (1 month), the sheep were penned individually into metabolism cages and in controlled temperatures (18 to 22°C).
Diets and plant extracts
Diets distributed to the animals throughout the entire experiment consisted of 840 g/d meadow hay and 360 g/d concentrate, which was composed of barley (43 % diet DM), beet pulp (40 %), soyabean meal (12 %) and molasses (5 %). Diets were distributed twice per d at 0800 and 1600 hours and feed intake was recorded each day.
Four PERP were selected according to the economical and technical requirements of animal nutrition and were supplied by the Phytosynthèse society (Saint-Bonnet de Rochefort, France). The PERP were obtained from rosemary (RO; Rosemarinus officinalis, entire plant used), grape (GP; Vinis vitifera, peel and seed used, aqueous extraction), citrus (CI; Citrus paradisi, fruit used, aqueous extraction) and marigold (MA; Calendula officinalis, flower used). The content of proanthocyanidins in GP represented more than 80 % of the polyphenols, and polymeric proanthocyanidins represented 70 % of the total proanthocyanidins according to the manufacturer's details. Concerning CI extract, it contained more than 95 % naringin. RO was not purified and was then assumed to contain all the usual polyphenolsReference Del Baño, Lorente, Castillo, Benavente-Garcia, Del Rio, Ortuño, Quirin and Gerard24, i.e. diterpenes phenolic and rosmarinic acid. MA was not purified and was then assumed to contain all the usual phenolic acidsReference Matysik, Wojciak-Kosior and Paduch25, i.e. vanillic, caffeic, p-coumaric and ferulic acids. Moreover, MA was analysed for its carotenoid content and xanthophylls represented >1 % of plant. In addition to the manufacturer's details, the polyphenol content (Folin-Ciocalteu assay) as well as the in vitro reducing capacity (1,1-diphenyl-2-picrylhydrazyl assay) of the four PERP were determined as previously reportedReference Gladine, Morand, Rock, Bauchart and Durand22 and are reported in Table 1.
Table 1 Polyphenol content and reducing potential of plant extracts obtained from rosemary (RO), grape (GP), citrus (CI) or marigold (MA)

* Gallic acid equivalent, mg/g DM.
† Trolox equivalent antioxidant capacity, μmol/g DM.
Experimental design
The experiment was divided into two phases. The first phase (phase 1) lasted 23 d and consisted of a continuous linseed oil infusion (4 % DM ingested) via the duodenum cannula. This procedure, developed in our laboratoryReference Scislowski, Bauchart, Gruffat, Laplaud and Durand9, aimed to protect n-3 PUFA from rumen biohydrogenation and to increase the n-3 PUFA content in plasma, making it highly susceptible to lipoperoxidation. During the second phase (phase 2, 22 d), animals were still continuously infused with linseed oil but they were also given one of the four PERP or chopped hay (control treatment), according to a 5 × 5 Latin square design (i.e. 5 d of administration and five sheep). Sub-periods lasted 3 d and the administration was performed before the morning meal. It consisted of one acute dose (10 % DM intake) administrated via the rumen cannula. The use of the rumen cannula aimed to control the time of administration and the amount of PERP administrated.
Blood sampling
A set of four blood samples (BS; 10 ml) was collected by venipuncture from the jugular vein during phase 1 to determine the effect of linseed oil infusion on lipaemia. A first BS was collected before the beginning of the linseed oil infusion (time zero, T0) and the other three were collected 9, 16 and 23 d after the beginning of the infusion (T9, T16, T23).
During phase 2, BS (20 ml) were collected before and after the PERP administration (Fig. 1) as follows: two samples (BS1 and 2) were respectively collected 60 min and 15 min before the PERP administration in the rumen and were designated as reference samples (REF samples). After the PERP administration, BS were collected every 3 h for 30 h (BS3 to BS12). Time of sampling was determined by taking into account the retention times of the liquid and the solid phases of the rumen content, since polyphenols could be eluted with both phases. The sampling procedure was used for each of the five sheep and was repeated for each of the 5 d of administration.

Fig. 1 Scheme of the blood sampling procedure after plant extracts rich in polyphenol (PERP) administration in the rumen of sheep (n 5). Blood sample (BS) 1 and 2 were collected before PERP administration and designated as reference samples (REF samples). PERP (rosemary (RO), grape (GP), citrus (CI) and marigold (MA)) or chopped hay (control) were put, as a single acute dose (10 % DM ingested), directly in the rumen of sheep (n 5) according to a 5 × 5 Latin square design. Pooled blood samples No. 1 and No. 2 constituted for the analysis of the plasma polyphenol patterns and the monitoring of conjugated diene generation.
BS were collected into sterile vacutainer tubes containing: 1) Na2-EDTA (3 mm) for the determination of the lipid classes (TAG; phospholipid (PL); free cholesterol (FC); cholesteryl ester (CE); NEFA) and for polyphenol analysis; 2) lithium heparin (5 UI/ml) for total antioxidant status (TAS) measurement; 3) sodium citrate (18 mm) to monitor the kinetics of conjugated diene (CD) generation. Plasma was separated from blood by centrifugation (1600 g, 10 min at 4°C). The plasma collected for the analysis of polyphenols was immediately acidified to pH 6·8 with 10 mmol/l acetic acid. All plasmas were aliquoted and stored at − 80°C until analysis
Blood sample analysis
Plasma lipids
Concentrations of the major lipid classes were determined in BS collected during the first step of the experimental period. The enzymatic method used to determine TAG contentReference Leplaix-Charlat, Bauchart, Durand, Laplaud and Chapman26 required the Kit PAP 150 (ref. RC 1260-30) supplied by BioDirect (Charbonnières-les Bains, France). PL were determined by the enzymatic method of Trinder using the BioDirect Kit (ref. RC 1254-01; BioDirect). CE and FC were respectively determined enzymatically with the reagent Kit Cholesterol Liquide and Cholesterol Libre Enz. Color supplied by BioMérieux (Chennevièvre-les-Louvres, France). Plasma NEFA were determined enzymatically with the Wako Kit (Unipath, Dardilly, France). Total lipids corresponded to the sum of TAG+PL+FC+CE+NEFA.
Polyphenol pattern determination
This analysis was performed in plasma collected during phase 2 and pooled as follows: a first pooled sample (pooled BS No. 1) was constituted by mixing the two REF samples collected before the PERP administration (BS1 and 2, see Fig. 1), a second pooled sample (pooled BS No. 2) consisted of the mixture of the samples collected 12, 15, 18 and 21 h after PERP administration in the rumen (BS6, BS7, BS8, BS9, see Fig. 1). This period of time corresponded to the period during which TAS reached the maximal value for most experimental groups (see Fig. 2). For each day of PERP administration (n 5), two pooled samples (BS1 and BS2) were then constituted for each sheep (n 5). At the end of the experimental period, we finally pooled all together the BS1 samples from sheep given the same PERP (five final pooled samples constituted) and the BS2 samples from sheep given the same PERP (five final pooled samples constituted).

Fig. 2 Evolution with time (from 3 to 30 h) of plasma total antioxidant status (expressed as incremental area under the curve (iAUC) calculated by using the linear trapezoidal rule) of sheep (n 5) given, directly in the rumen, as a single acute dose (10 % DM ingested), one of the four plant extracts rich in polyphenols (PERP): □, control; , rosemary;
, grape;
, citrus;
, marigold. Values are means with their standard errors for five sheep. Experimental values were significantly different from the control value: *P < 0·05. For details of diets and procedures, see Materials and Methods.
These two pooled samples were prepared for each extract (n 5) and for each day of administration (n 5). Finally, we pooled the five pooled BS No. 1 and the five pooled BS No. 2 obtained from the five sheep receiving the same extract, leading to ten final pooled samples, which were used for polyphenol pattern analysis.
For the polyphenol analysis, plasma samples (180 μl) were acidified to pH 4·9 with 20 μl 0·58 mol/l acetic acid and were incubated at 37°C with a mixture of β-glucuronidase (1000 units) and sulfatase (100 units) from Helix pomatia (Sigma, Chesnes, France). This enzymatic procedure hydrolysed the circulating form of polyphenols (glucuronides and sulphate) to release the aglycone form. Incubation times were selected according to the main polyphenols known to be present in each extractReference Morand, Manach, Donovan and Rémézy27: RO (rosmarinic acid), GP (proanthocyanidins), CI (naringin) or MA (phenolic acids) and were set as follows: 30 min for plasma collected after GP administration; 4 h for plasma collected after RO and MA administration; 5 h for plasma collected after CI administration. The aglycones released were then extracted with 500 μl methanol/HCl 200 mmol/l and centrifuged at 14 000 g for 4 min at 4°C. Polyphenols in the resulting supernatant fractions were analysed by HPLC 8-electrodes-CoulArray Model 5600 system (Eurosep, Cergy, France) fitted with a 150 × 4·6 Hypersil BDS C18-5 μm column (Interchim, Montluçon, France). The mobile phases consisted of 5 % acetonitrile in 30 mmol/l NaH2PO4 pH 3·0 for solvent A and 50 % acetonitrile in 30 mmol/l NaH2PO4 pH 3·0 for solvent B. The analysis was performed using a gradient elution procedure as follows: flow rates 0·80 ml/min; 0–3 min: solvent A 100 %; 3–73 min: from solvent A 100 % to solvent B 100 %; 73–76 min: solvent B100 %; 76–95 min solvent A 100 %. For detection, electrode potentials were set at 0, 120, 240, 360, 480, 600, 720 and 840 mV. These chromatographic and electrochemical conditions allow the separation of both phenolic acids and flavonoids. The antioxidant capacity of the polyphenols was relative to their electrochemical behaviour, the molecules detected on electrodes set at low potentials (from 0 to 480 mV) being the most antioxidant. Polyphenols in plasma of experimental groups were visualized by comparing with the control group as well as by comparing the chromatographic profiles obtained from plasma collected before and after PERP administration. Moreover, standards of phenolic acids (rosmarinic, chlorogenic, ferulic, isoferulic, caffeic, p-coumaric, vanillic acids) and of flavonoids (proanthocyanidins B1, B2 and B3, catechin, epicatechin, naringenin) were used to identify specific polyphenols potentially present in plasma.
Total antioxidant status
TAS was measured in plasma samples collected before (BS 1 and 2, see Fig. 1) and during (BS3 to 12, see Fig. 1) the 30 h following the ruminal administration of each PERP. This measurement was performed to determine the contribution of PERP to the oxidative stability of plasma and to provide a first indication of PERP bioavailability. The analytical method was based on the absorbance of the ABTS°+ (2,2′-azinobis-3-ethylbenzothiazoline-6-sulphonic acid)Reference Miller, Rice-Evans, Davies, Gopinathan and Milner28 cation. Briefly, production of ABTS°+ was initiated by addition of 450 μm-hydrogen peroxide in 1 ml quartz cuvette, containing 12 μl plasma, 500 μm-ABTS and 48·8 μm-metmyoglobin diluted in degassed PBS (5 mm, pH 7·4). Absorbance at 732 nm was measured immediately (At0) and 3 min (At3) after the addition of H2O2 at 37°C using a double-beam spectrophotometer (Uvikon 923; Kontron Analysis Division, Zurich, Switzerland). Absorbance of a blank (i.e. containing degassed-PBS instead of plasma) was also determined to calculate the inhibition percentage of the reaction as follows:

Inhibition percentage was converted into μmol of trolox equivalent antioxidant capacity using a trolox standard curve ranging from 0 to 2500 μm.
Conjugated dienes
Susceptibility of plasma PUFA to peroxidation was determined by measuring the kinetics of CD generation on plasma collected during Phase 2. The analysis was performed on fifty pooled plasma samples, which corresponded to the pooled BS No. 1 and the pooled BS No. 2 constituted for each sheep (n 5) at each day of administration (n 5) as described earlier. Oxidation process was initiated by the addition of CuCl2 (10 mm)Reference Schnitzer, Pinchuk, Fainaru, Schafer and Lichtenberg29. CD absorbance was continuously monitored at 234 nm at 37°C for 10 h using the double-beam spectrophotometer (Uvikon 923; Kontron Analysis Division). The kinetics of CD generation were divided into two phases, from which two parameters were calculated as previously describedReference Esterbauer, Striegl, Puhl and Rotheneder30: 1) the length of lag phase (min) corresponding to the resistance time of PUFA against peroxidation; 2) the maximum rate of peroxidation (A234/min), which corresponds to the slope of the curve and represents the speed of the propagation phase.
Statistical analysis and calculation
All data were subjected to ANOVA analysis by using the general linear model procedure of SAS (SAS Institute, Cary, NC, USA). Significance was declared at P < 0·05 and P ≤ 0·10 was considered as a trend. When the effect was declared as a trend or significant, means of the five groups were compared using Student's t testReference Schnitzer, Pinchuk, Fainaru, Schafer and Lichtenberg29.
The evolution of TAS over the 30 h following the plant extract administration was expressed as an incremental area under the curve (iAUC). The initial calculation was done to determine the evolution between the TAS determined before (REF samples BS1 and BS2, TASREF, see Fig. 1) and after (BS3 to BS12, TAS+3 h to +30 h) the PERP administration. Then, iAUC were calculated, for each period of 3 h, using the linear trapezoidal rule as follows:
1 AUC0 to 3 h = TAS+3 h − TASREF
2 iAUC0 to 6 h = AUC0 to 3 h+(TAS+6 h − TASREF)
3 iAUC0 h to n h = AUC0 h to n − 3 h+(TASn h − TASREF)
For each time of plasma sample analysis, means of the five groups were compared using Student's t testReference Snedecor and Cochran31.
Results
Throughout the experimental period, mean daily intake of sheep was 755 (sem 99) g/d for hay and 343 (sem 24) g/d for concentrate. The administration of PERP did not modify feed intake.
Lipaemia evolution in response to linseed oil infusion
The evolution of lipid concentration in plasma, i.e. TAG, PL, FC, CE and NEFA over the first 3 weeks of linseed oil infusion (Phase 1) is shown in Table 2. Before infusion, total lipids (sum of TAG, PL, FC, CE and NEFA) were distributed as follows: 8·2 % TAG; 41·8 % PL; 0·6 % FC; 48·7 % CE; 0·8 % NEFA. After 23 d of linseed oil infusion, plasma exhibited an overall hyperlipaemia (+40 % total lipids), which was particularly due to the increase of the major lipids, i.e. PL and CE (+43 %, P < 0·05). In addition to the modifications of the lipid profile, the kinetics of evolution over the 3-week period differed between lipid classes. Indeed, TAG, FC and NEFA were exclusively increased during the first week of infusion (+53 %, P < 0·05;+173 %, NS; +67 %, P < 0·05 respectively) and then decreased during the last 2 weeks, whereas PL and CE concentrations were continuously enhanced until the end of the infusion period.
Table 2 Effect of duodenal linseed oil infusion (4 % DM ingested) for 9, 16 and 23 d in castrated sheep (n 5) on plasma concentrations (mg/dl) of TAG, phospholipid, free cholesterol, cholesteryl ester and NEFA* (Mean values with their standard errors)

Data were subjected to general one-way ANOVA with time as the main factor. When the effect was declared as a trend (P ≤ 0·1) or significant (P ≤ 0·05), means of the four times were compared using Student's t test. Mean values within a row with unlike superscript letters were statistically different (P < 0·05).
* For details of diets and procedures, see Materials and methods.
Total antioxidant status evolution during the 30 h following plant extracts rich in polyphenol administration
The evolution of TAS during the 30 h following PERP or chopped hay (control) administration is reported in Fig. 2. The plasma of sheep given the PERP exhibited an overall increase of TAS (mean value for the four experimental groups at +30 h: +134 iAUC) whereas, in control, TAS decreased over the 30 h period ( − 72 iAUC). The effect of PERP on TAS evolution from +3 h to +30 h was particularly pronounced with GP (+341 iAUC) while RO and CI both exhibited a rise of 81 iAUC and MA only increased TAS by 35 iAUC. Moreover, GP was the only PERP to significantly modify TAS (P < 0·05) in comparison with control.
Kinetics of TAS evolution differed with the PERP given. Indeed, in the GP group, TAS raised regularly from the beginning (+3 h) to the end (+30 h) of the blood collection period and the differences with the control group were significant (P < 0·05) from 15 h until 30 h after GP administration in rumen of sheep. In contrast, in RO, CI and MA groups, TAS was maximal between 12 and 21 h after PERP administration and it tended to decrease from 21 h to 30 h.
Recovery of polyphenols in plasma after the plant extracts rich in polyphenol administration
Polyphenol patterns (Figs. 3 and 4) were determined in plasma collected between 12 and 21 h, which corresponded to the period during which TAS reached the maximal value for most experimental groups (Fig. 2).

Fig. 3 HPLC chromatograms with multi-electrode coulometric detection of aglycone polyphenols from (a) chopped hay (control); (b) plasma in which standards were added or from pooled plasma of sheep given, directly in the rumen, as a single acute dose (10 % DM ingested); (c) rosemary (RO); (d) grape (GP); (e) marigold (MA). Electrode potentials were set at 0 mV (black), 120 mV (pink), 240 mV (dark blue), 360 mV (red), 480 mV (light green), 600 mV (yellow), 720 mV (dark green) and 840 mV (light blue) and only the electrodes on which signals were recorded are represented. For details of plasma pooling and analysis procedures, see Materials and methods section.
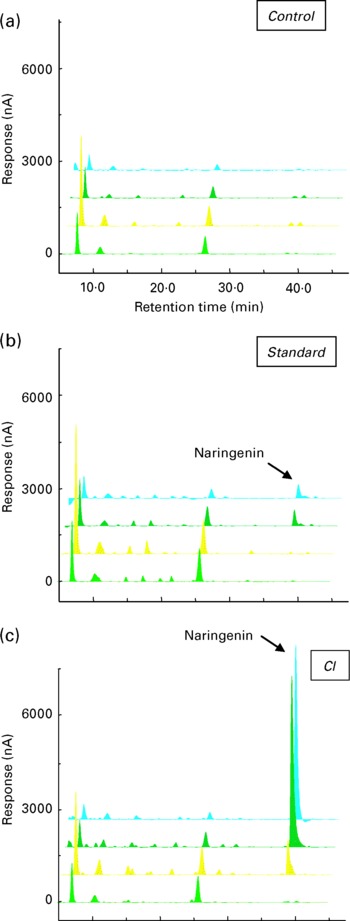
Fig. 4 HPLC chromatograms with multi-electrode coulometric detection of aglycone polyphenols from (a) chopped hay (control), (b) plasma in which standards were added or from pooled plasma of sheep given, directly in the rumen, as a single acute dose (10 % DM ingested) or (c) citrus (CI). Electrode potentials were set at 0 mV (black), 120 mV (pink), 240 mV (dark blue), 360 mV (red), 480 mV (light green), 600 mV (yellow), 720 mV (dark green) and 840 mV (light blue) and only the electrodes on which signals were recorded are represented. For details about plasma pooling and analysis procedures, see Materials and methods.
All the PERP tested led to the appearance of new polyphenols in comparison with control (Figs. 3(b) and 4(b)). With RO administration (Fig. 3(c)), five phenolic compounds were detected. One was eluted very early (at 9·0 min, unknown A) and exhibited a high signal on the electrode set at 120 mV. Four other small signals were recorded on the electrode set at 360 mV at 10·0 min (unknown B), 14·2 min (unknown C), 29·6 min (unknown D) and 31·6 min (unknown E). Despite the use of a large panel of standards (including rosmarinic acid), none of the five new phenolic molecules recovered in plasma of the RO group has been identified.
After GP administration (Fig. 3(d)), among the five signals recorded, the first two peaks detected had the same retention times (9·0 and 10·0 min) and were detected on the same electrodes (set at 120 and 360 mV) as the first two peaks recorded in RO plasma (unknown A and B). Moreover, three other signals were recorded on the electrode set at 120 mV at 15·3, 16·9 (unknown F) and 19·4 min (unknown G). Among these six phenolic molecules detected, the fourth eluted (at 15·3 min) was identified as epicatechin.
With MA treatment (Fig. 3(e)), five new phenolic molecules appeared in plasma. The first two (recorded at 9·0 and 10·0 min) had the same chromatographic and electrochemical behaviours as the first molecules detected with both RO and GP extracts (unknown A and B). The third and the fourth molecules detected had the same chromatographic and electrochemical behaviours as the unknown C from RO plasma and the unknown F from GP plasma. Finally, a fifth signal was recorded at 24·9 min. Despite the use of many standards of flavonoids and phenolic acids, none of the five molecules detected has been identified.
Finally, with CI (Fig. 4(c)), a unique signal with a high intensity was recorded at 40·0 min simultaneously on the electrodes set at 600, 720 and 840 mV. This molecule was identified as naringenin.
Effect of plant extracts rich in polyphenols on lipoperoxidation in plasma
The effect of PERP on lipoperoxidation was evaluated ex vivo by measuring the kinetics of CD generation in pooled plasma collected before and after the administration of PERP in the rumen. Lag phase and oxidation rate, which respectively represent the length of the resistance phase and the speed of the propagation phase of lipoperoxidation, are reported in Table 3.
Table 3 Evolution* of lag phase and oxidation rate in plasma of sheep given one of the four plant extracts rich in polyphenols (PERP) as a single acute dose (10 % DM ingested) directly in the rumen†(Mean values with their standard errors for five animals)

Data were subjected to general one-way ANOVA with diet as the main factor. When the effect was declared as a trend (P ≤ 0·1) or significant (P ≤ 0·05), means of the four times were compared using Student's t test. Mean values within a row with unlike superscript letters were statistically different at P < 0·05.
* Expressed as differential lag phase and differential oxidation rate, which corresponds to the difference of values determined in plasma collected before and after rumen PERP administration.
† For details of diets and procedures, see Materials and methods.
RO, rosemary; GP, grape; CI, citrus; MA, marigold.
All the PERP tested increased the length of the lag phase when compared with the control group (+5·9 min, P < 0·02). This rise of the resistance phase was particularly pronounced with MA and RO, which respectively increased the length of lag phase by 78 % (P < 0·01) and 69 % (P = 0·01), while CI and GP increased lag phase by 46 % (P = 0·03) and 29 % (P = 0·05) respectively.
The speed of the propagation phase, as represented by the oxidation rate, was reduced with the four PERP tested ( − 1·7 A234/min, P < 0·01) in comparison with the control. This parameter was particularly reduced with MA ( − 57 %, P < 0·01) while RO and GP both reduced the oxidation rate by 16 % (P < 0·01) and CI extracts by 14 % (P < 0·01).
Discussion
The present experiment was conducted to investigate the bioavailability and the antioxidant capacity of four PERP in ruminants. Considering that most studies in this field of research have been performed in monogastric species, the relevance of the present results will be discussed by referring to data obtained from monogastric studies.
To make plasma highly susceptible to lipoperoxidation, linseed oil (4 % DM) was continuously infused in the duodenum of sheep. After 3 weeks of treatment, plasma of the sheep was characterized by hyperphospholipaemia as well as hypercholesterolaemia. Similar experiments were previously performed in the laboratory on steers and led to the same modifications of lipid profile (+63 % PL and +100 % CE)Reference Scislowski, Bauchart, Gruffat, Laplaud and Durand9. The alteration of the lipid profile was associated with a six-fold increase of linolenic acid (18 : 3n-3) concentration in plasma and significant reduction of lag phase ( − 40 %), suggesting a higher susceptibility of plasma lipids to lipoperoxidationReference Scislowski, Bauchart, Gruffat, Laplaud and Durand9.
In vivo antioxidant capacity of plant extracts rich in polyphenols
The addition of PERP in the rumen of sheep, and more especially of GP, increased the TAS of plasma, whereas the plasma TAS of sheep given chopped hay (control) regularly decreased during the 30 h period. This is not consistent with a recent experimentReference O'Grady, Maher, Troy, Moloney and Kerry32 conducted in Charolais bulls given RO extract, but the low level of extract administered (0·02 % DM ingested) as well as the time of plasma collection (3 h after the extract administration) were probably not appropriate to induce a significant evolution of TAS in the plasma of bulls. In the present experiment, we deliberately chose to test a supranutritional dose (10 % DM ingested) but further investigations should be performed to determine which dose of our PERP should be recommended for a nutritional treatment. In rodents, we have previously shown that PERP (RO, GP, CI and MA) given at a nutritional dose (0·5 % DM) did not increase TAS in plasma collected in the post-absorptive stateReference Gladine, Morand, Rock, Bauchart and Durand22. In the same experiment, the level of malondialdehyde (a final metabolite of lipoperoxidation) was nevertheless reduced, suggesting that the PERP were bioefficient antioxidants and it has been concluded that the level of polyphenols (or their metabolites) in plasma was too low to increase TAS. A human study showed that a unique acute dose (240–300 g) of various polyphenol-rich foods significantly increased TAS (+7–25 %) during the 4-h period following consumptionReference Cao, Russell, Lischner and Prior33. Then, the dose of PERP rather than the time of blood collection may be the limiting parameter to modify TAS in plasma.
Among the four PERP tested, GP was by far the most efficient PERP to improve TAS in sheep plasma. This difference could have been expected since GP had the greatest content in polyphenols but, on the basis on a previous study conducted in ratsReference Gladine, Morand, Rock, Bauchart and Durand22, it was assumed that polymeric proanthocyanidins (the main polyphenol in our GP extract) were not bioavailable. The present experiment then demonstrated that, contrary to monogastrics, ruminants can make polymeric proanthocyanidins bioavailable and benefit from their strong antioxidant capacity. This may involve a hydrolysis of proanthocyanidin polymers into bioavailable and bioactive monomers (catechin or epicatechin) by hydrolysed rumen micro-organisms, since it has been shownReference Terrill, Waghorn, Woolley, Nabb and Barry34 that polymeric proanthocyanidins are poorly recovered after their transit through the rumen.
The kinetics of TAS evolution were different between the four PERP. Indeed, with RO, CI and MA the highest value of TAS was reached between 12 and 21 h, whereas with GP, TAS kept on rising for 30 h. These differences might be due to the high level of proanthocyanidins in GP, which can form stable complexes with proteinsReference Singh, Bhat and Singh35 and bind to the plant particles constituting the solid phase in the rumenReference McAllister, Bae, Jones and Cheng36. In contrast, the other PERP might have been rapidly transferred, together with the liquid phase, towards the small intestine.
Recovery of polyphenols in plasma
The administration of the four PERP induced marked changes in the polyphenol patterns in sheep plasma. Moreover, the present experiment revealed that polyphenols were still present in sheep plasma more than 10 h after administration. Until now, bioavailability of polyphenols in ruminants has been poorly documented and only one experiment, conducted in dairy cows and goats, reported the presence of isoflavones in milk, suggesting that polyphenols could be absorbed in ruminantsReference Sakakibara, Viala, Doreau and Besle37.
RO contains mainly rosmarinic acid as well as some diterpene phenolicsReference Gladine, Morand, Rock, Bauchart and Durand22. In monogastrics, it has been shownReference Baba, Osakabe, Natsume, Yasuda, Muto, Hiyoshi, Takano, Yoshikawa and Terao38, Reference Konishi, Hitomi, Yoshida and Yoshioka39 that rosmarinic acid is mostly recovered intact in plasma within 30 min and its metabolites (i.e. ferulic, caffeic and coumaric acids) appear within 8 h. The sheep plasma collected between 12 and 21 h after RO administration did not contain rosmarinic acid or its metabolites but other phenolic compounds were recovered. Two hypotheses could explain these contrasting results between monogastrics and ruminants. First, it is possible that rosmarinic acid and its metabolites were absorbed in sheep but were already excreted when we collected the plasma. Second, the presence of unknown polyphenols in sheep plasma may suggest that rosmarinic acid was metabolized in rumen and the anaerobic microbial activity of the rumen may explain the differences with monogastrics. Concerning the phenolic diterpenes, they could not be identified with the standard used in the present experiment but their presence cannot be excluded.
Proanthocyanidins (or condensed tannins) are the most abundant phenolic compounds in GP. They are oligomeric or polymeric flavanols composed of catechin and epicatechin. The GP extract used in the present experiment contained more than 80 % proanthocyanidins, which were mostly under a polymeric form (>70 %). The administration of GP directly in the rumen of sheep led to the appearance of five different phenolic compounds in plasma, among which epicatechin was identified. The absorption and the metabolism of polymeric proanthocyanidins have been extensively studied in monogastrics. It has been reportedReference Déprez, Brezillon, Rabot, Philippe, Mila, Lapierre and Scalbert40 that polymeric proanthocyanidins are catabolized into phenolic acids of low molecular weight by human colonic microflora. Other authors have suggested that polymeric proanthocyanidins are hydrolysed by gastric fluids to lower molecular compounds such as catechin and epicatechinReference Spencer, Chaudry, Pannala, Srai, Debnam and Rice-Evans41, Reference Spencer, Schroeter, Shenoy, Srai, Debnam and Rice-Evans42 but it was not confirmed in a recent studyReference Rios, Bennett, Lazarus, Rémézy, Scalbert and Williamson43 showing that procyanidins are stable during gastric transit in human subjects. Finally, it was reportedReference Donovan, Manach, Rios, Morand, Scalbert and Rémézy44, Reference Nakamura and Tonogai45 that polymeric proanthocyanidins are not decomposed into bioavailable monomers in the monogastric digestive system and it was concluded that only the monomeric components of GP (catechin and epicatechin) are absorbed in monogastrics. Considering that our GP extract contained a minor proportion of monomeric components, it is likely that the relatively high level of epicatechin recovered in the plasma of sheep came from the biodegradation of polymeric proanthocyanidins by rumen micro-organisms. This would suggest that, contrary to monogastrics, ruminants can benefit from the strong antioxidant properties of polymeric proanthocyanidins by metabolizing them into bioavailable compounds with intact flavonoid-ring structure (epicatechin).
The CI extract given to sheep in the present experiment contained more than 95 % naringin (naringenin-7-rhamnoglucoside), which is a glycosylated flavanone specific to citrus fruitsReference Ortuño, Garcia-Puig, Fuster, Pérez, Sabater, Porras, Garcia-Lidon and Del Rio46. In monogastrics, the glycosylated form cannot be absorbed by the small intestine and naringin is transferred to the large intestine, where the colonic microflora hydrolyse the glycosidic fraction and release the aglycone (naringenin), which is then absorbedReference Felgines, Texier, Morand, Manach, Scalbert, Régerat and Rémézy47. In the present experiment, naringenin was recovered in a large proportion in the plasma of sheep and no other metabolite was detected. This suggests that ruminal metabolism did not modify the flavanone structure of naringin and that, as in monogastrics, the hydrolysis of the glycosidic fraction was required before absorption. However, in contrast with monogastrics, it is likely that the hydrolysis of the glycosidic fraction occurred in the rumen rather than in the large intestine. Indeed, the delays of naringenin absorption were relatively similar in monogastricsReference Felgines, Texier, Morand, Manach, Scalbert, Régerat and Rémézy47 and in our sheep (around 10 h after administration), although it should have been much longer in sheep due to the rumen retention of the CI extract. However, further experiments using ruminal fluid should be performed to confirm that rumen micro-organisms can hydrolyse the glycosidic fraction of naringin and release the aglycone form without metabolizing it further.
MA contains many antioxidant compounds, including phenolic acids such as vanillic, caffeic, chlorogenic, p-coumaric and ferulic acidsReference Matysik, Wojciak-Kosior and Paduch25. We detected five polyphenols in the plasma of sheep given MA but none of them was identified as the phenolic acids listed earlier, suggesting that these molecules were further metabolized in the rumen. However, feeding trials with MA extract have never been reported in literature and no data on the circulating polyphenols are available.
Finally, the analysis of polyphenol patterns in plasma of sheep given RO, GP and MA revealed the presence of common phenolic compounds, among which the unknown compound A (first molecule eluted) represented a major polyphenol, exhibiting a high polarity, a low molecular weight and a high reducing capacity (according to its response at low potentials). This common metabolite could not be identified in the present experiment but further analysis should be performed since this phenolic compound may be the final metabolite of many polyphenols in sheep and it could play a crucial antioxidant function in sheep plasma.
Bioefficiency of plant extracts rich in polyphenols to limit lipoperoxidation
The four PERP tested reduced the susceptibility of plasma to lipoperoxidation since lag phase (a parameter related to the resistance of lipids to peroxidation) was significantly increased after PERP administration in the rumen. This effect was particularly pronounced with MA, which is rather surprising since MA did not exhibit the strongest reducing capacity in vitro and was not associated with a significant increase of TAS in plasma of sheep. In contrast, GP was the most efficient in vitro and significantly improved TAS but was not more bioefficient than the other PERP to increase lag phase. Since we previously demonstrated that GP's polyphenols (or their metabolites) were relatively well absorbed (see Polyphenol patterns), it is likely that the chemical structure of the bioactive compounds from GP was not appropriate (e.g. too hydrophile) to prevent lipid oxidation. In our previous experiment conducted in ratsReference Gladine, Morand, Rock, Bauchart and Durand22, neither MA nor GP significantly increased lag phase in plasma, and RO was the only bioefficient extract concerning this parameter. This conclusively shows that the bioefficiency of PERP to prevent lipoperoxidation cannot be predicted from in vitro measurement. Moreover, the differences observed between sheep and rats provided further evidence that rumen metabolism of PERP generated specific polyphenol metabolites with different biological properties than the metabolites present in rat plasma.
The ability of PERP to protect lipids from peroxidation also suggests that the polyphenols or their metabolites were integrated into the lipid structure or at the aqueous–lipid interface. This would suggest that, contrary to the majority of polyphenols, those brought by our PERP and recovered in the plasma of sheep were not bound to proteinsReference Manach, Scalbert, Morand, Rémézy and Jimenez17 but were either localized at the aqueous–lipid interface as previously reported for ( − )-epigallocatechin-3-gallateReference Aldini, Yeum, Carini, Krinsky and Russell48 or incorporated in lipoproteins, as was described with genisteinReference Kaamanen, Adlercreutz, Jauhiainen and Tikkanen49. Moreover, the stronger effect of MA may be due to the presence of carotenoids in addition to polyphenolsReference Hadden, Watkins, Levy, Regalado, Rivadeneira, van Breemen and Schwartz50.
The four PERP tested, and more especially MA, reduced the oxidation rate, a parameter related to the propagation phase of lipoperoxidation. This effect was not observed in ratsReference Gladine, Morand, Rock, Bauchart and Durand22, confirming that polyphenol metabolites generated in ruminants were different from the ones recovered in the plasma of rats. In ruminants, PERP were efficient to prevent both the initiation and the propagation of lipoperoxidation, suggesting their ability to quench both the reactive oxygen species (or any other initiator) and the lipoperoxide generated. This biological property, usually attributed to vitamin EReference Khalil51, confirms that the polyphenols were integrated into the lipid structure or at the aqueous–lipid interface, since they would not have been able to quench lipoperoxides by being linked to proteins.
In conclusion, the present experiment showed that the four PERP tested kept their antioxidant capacity in vivo in ruminants. Moreover, in the case of GP, the action of rumen micro-organisms was beneficial, since they improved the bioavailability of polymeric proanthocyanidins. Rumen also has a beneficial effects by delaying the absorption of GP polyphenols, leading to a longer time of action than the other PERP, which were more rapidly transferred into the plasma of sheep. The four PERP tested in the present experiment were also particularly efficient to limit plasma susceptibility to lipoperoxidation and should therefore represent a specific interest in ruminants fed n-3 PUFA-rich diets. The MA extract was the most bioefficient, probably because of the lipophilicity of its bioactive compounds.
The present work was an essential step to investigate the interest of polyphenols as new antioxidants in animal nutrition and their essential role to improve growth performances and product quality. The encouraging results obtained concerning the biological activity of the four PERP selected lead us to envisage further work to elucidate the nature and the structure of the active metabolites found in plasma and to better understand the metabolic pathways of polyphenols in ruminants. Moreover, a supranutritional dose was deliberately used in the present experiment but a dose–response study should be conducted in order to determine the optimum level of administration.
Acknowledgements
This experiment was financially supported by the European Union (via a EUREKA research programme). We thank our industrial partner Phytosynthese (Saint-Bonnet de Rochefort, France) for supplying PERP. We also wish to address specific thanks to Mrs Anne-Sophie Bage, Françoise Duboisset, Christiane Legay, Marinett Martinaud and Agnès Thomas for their technical assistance as well as to Mr Daniel Thomas, Sébastien Alcouffe and Miche Fabre for the animal care.