Sarcopenia refers to the age-related reduction in muscle quantity and strength(Reference McKendry, Currier and Lim1). The age-related reduction in strength, which is the strongest predictor of health-related outcomes in older adults(Reference Cruz-Jentoft, Bahat and Bauer2), occurs much more rapidly than the decrease in muscle quantity(Reference Mitchell, Atherton and Williams3). Although multi-factorial, contributing factors for sarcopenia include physical inactivity(Reference Cruz-Jentoft, Bahat and Bauer2) and an attenuated anabolic response to dietary protein (i.e., ageing anabolic resistance)(Reference Robinson, Reginster and Rizzoli4), suggesting that the amount of protein consumed by older adults should be increased to offset sarcopenia. It is well established that resistance training improves ageing muscle mass and strength(Reference Bagheri, Rashidlamir and Motevalli5–Reference Bagheri, Forbes and Candow7). Accumulating research indicates that the addition of protein consumption to a resistance training programme can further augment these physiological and neuromuscular adaptations(Reference Finger, Goltz and Umpierre8). Numerous high-quality complete protein sources such as whey(Reference Davies, Carson and Jakeman9), casein(Reference Joy, Vogel and Broughton10), egg(Reference Bagheri, Moghadam and Jo11), beef(Reference Valenzuela, Mata and Morales12), soya(Reference Orsatti, Maestá and de Oliveira13) and potato(Reference Oikawa, Bahniwal and Holloway14) acutely elevate the rates of muscle protein synthesis and skeletal muscle regulatory factors, which over time could lead to significant improvements in muscle accretion and strength. Dairy product is also a complete protein food source comprised mainly of whey and casein proteins with high essential amino acid content(Reference Bridge, Brown and Snider15). Whey is considered a fast-absorbing protein, while casein is a slow-digesting protein, and their combination appears to be ideal for both initiating and sustaining post-exercise aminoacidemia(Reference Dangin, Boirie and Garcia-Rodenas16–Reference Granic, Hurst and Dismore18).
Yogurt is a dairy-based probiotic food source and a cost-effective protein source (primarily casein and whey) compared with other marketed protein supplements(Reference El-Abbadi, Dao and Meydani19). Research is mixed regarding the efficacy of yogurt consumption during a resistance training programme for the improvement of muscular adaptations. One study reported positive outcomes(Reference Bridge, Brown and Snider15), while other investigations reported no significant effects(Reference White, Bauer and Hartz20,Reference Thomas, Wideman and Lovelady21) . Importantly, previous studies were performed in untrained younger adults; and consequently, the effects of yogurt consumption during a resistance training programme in untrained older adults are unknown. While several types of yogurt exist, Icelandic yogurt contains one of the highest concentrations of protein (18 g per 200 g serving), which could serve as an effective adjunct to resistance training for augmenting muscle accretion and strength.
Muscle protein balance is also influenced by various hormones and myokines, which have been suggested to alter the balance between anabolic and catabolic stimuli in muscle, leading to an increase or decrease in muscle mass(Reference Solomon and Bouloux22). Briefly, follistatin (FST) stimulates muscle growth, while myostatin (MST) is a potent negative regulator of muscle accretion(Reference Bagheri, Moghadam and Church23). Moreover, Transforming growth factor-beta 1 (TGF-β1) acts as a skeletal muscle regenerator that contributes to extracellular matrix reconstitution and muscle tissue remodelling(Reference Touvra, Volaklis and Spassis24). Activin A is involved in cellular differentiation, remodelling, proliferation and morphogenesis(Reference Silva, Bueno and Avó25). Growth differentiation factor 15 (GDF15) is a member of the Glial cell-derived neurotrophic factor family, which is bound to Glial cell-derived neurotrophic factor family receptor α-like protein, a transmembrane receptor exclusively expressed in the hindbrain(Reference Baek and Eling26). Insulin-like growth factor 1 (IGF-1) is a regulator of the phosphoinositide 3-kinase and protein kinase B pathway and widely considered to be required for activating the signal transduction for the initiation of muscle protein synthesis following mechanical loading(Reference Spangenburg27). Two studies have indicated that 8 weeks of 3×/week whole-body resistance training increased FST while also decreasing MST concentrations with an enhanced lean mass in middle-aged and sarcopenic elderly males(Reference Bagheri, Rashidlamir and Motevalli28,Reference Negaresh, Ranjbar and Baker29) . Despite the reported positive impact of resistance training on endocrine markers and myokines in different cohorts, the effects of its combination with yogurt consumption are unknown. Therefore, the primary purpose of this study was to investigate the effects of post-exercise Icelandic yogurt consumption on lean mass and muscle strength in healthy untrained older males. A secondary purpose was to explore the effects of the intervention on skeletal muscle regulatory factors (i.e., FST, MST, TGF-β1, Activin A, GDF15 and IGF-1). We hypothesised that post-exercise Icelandic yogurt consumption, which contains 18 g of protein, would augment resistance training adaptations (i.e., lean mass and strength) and alter skeletal muscle regulatory factors compared with a placebo consumption.
Experimental methods
Participants
Thirty healthy untrained older males (age = 68 ± 4 years) volunteered for the study. The Human Subject Committee of the Ferdowsi University of Mashhad approved the study protocol (IR.UM.REC.1399.053). Participants were informed of the benefits, risks and purpose of the study before their written consent was obtained. Study procedures were in accordance with the Declaration of Helsinki. Inclusion criteria included being ≥ 60 years of age and untrained (performing < 1 h of exercise per week for 12 months prior to the start of the study). Participants were excluded if they were smokers, consumed alcohol regularly, had medical issues that would alter hormonal or muscle biology were lactose intolerant or consumed dietary supplements containing protein or creatine for 24 weeks beforehand. Participants were also excluded if they were unwilling to comply with the nutritional intervention or resistance training procedures or wanted to engage in additional exercise (independent of the study intervention). Moreover, participants reported no history of smoking or hormonal replacement therapy. Participants were instructed not to alter their lifestyle or habitual dietary intake throughout the study. A physician administered a health and medical questionnaire to determine participant health status. Before the intervention started, participants were familiarised with the testing and experimental procedures.
Design
The study used a randomised, double-blind, placebo-controlled parallel design. Participants were randomly assigned, using a computer programme (www.randomizer.org) to consume Icelandic yogurt (IR; n 15) or placebo (PR; n 15) during 8 weeks of resistance training. Prior to and following the study, measures of lean mass, muscle strength and skeletal muscle regulatory factors were made. Post-testing measurements occurred 72 h after the last training session.
Resistance training
Before the initiation of the resistance training programme, participants performed three familiarisation training sessions (supervised) with the resistance training equipment. After the familiarisation phase, participants performed whole-body resistance training (3×/week; supervised) according to guidelines and recommendations established for older individuals(Reference Chodzko-Zajko, Proctor and Singh30,31) . Prior to each training session, participants performed a 10-min warm-up consisting of light stretching. Participants then performed three sets of 8–12 repetitions for eight exercises (leg press, leg extension, lying leg curl, chest press, shoulder press, seated rows, biceps curl and sit-ups) at 60–80 % of 1-repetition maximum with 90-s rest intervals between sets (Table 1)(Reference Bagheri, Moghadam and Church23,Reference Mayer, Scharhag-Rosenberger and Carlsohn32) . All training sessions occurred between 16.00 and 18.00 hours. Training volume was calculated using the following formula (Training volume = (repetitions (n) × sets (n) × load or selected weight (kg))(Reference Cunha, Nunes and Tomeleri33).
Table 1. Resistance training programme
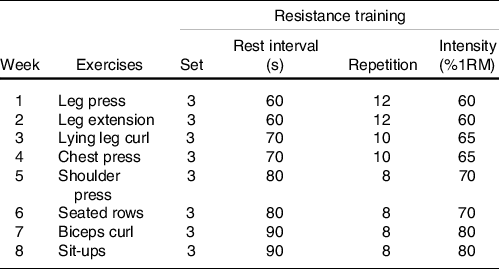
1RM, one-repetition maximum.
Nutritional intervention
Participants in the IR group consumed Icelandic yogurt (200 g serving; 18 g protein, 0 g fat, 4 g carbohydrates, Kalleh Industry, health license number: 49/14305), while participants in the PR group consumed a carbohydrate-based pudding (placebo (contained maltodextrin and water); 200 g serving: 0 g protein, 0 g fat, 2 g carbohydrates) immediately following each resistance training session (in the presence of an exercise supervisor) and at the same time on non-training days. To monitor compliance on non-training days, supplement packages were returned to the researchers at the next subsequent training session(Reference Bridge, Brown and Snider15) and documented in logs. Compliance was calculated by dividing the number of consumed servings by the expected number of servings. Icelandic yogurt was consumed immediately following each training session because post-exercise protein supplementation is important for augmenting gains in muscle mass in older adults(Reference Esmarck, Andersen and Olsen34). The Icelandic yogurt formulation was verified by independent laboratory testing (ViroMed Laboratory). Icelandic yogurt and placebo were in opaque containers and were very similar in taste (flavoured with vanilla) and appearance. All personnel involved in the study were blinded to group allocations.
Body composition
Participants fasted for 12 h with at least 8 h of sleep before body composition was assessed. Upon arrival at the laboratory, participants were required to void their bladder. Body mass was measured with a digital scale (Seca, Germany) to the nearest 0·1 kg. Stature was measured with a stadiometer (Race Industrialization) to the nearest 0·1 cm. BMI, fat mass and lean mass were determined by a multi-frequency bioelectrical impedance device (Inbody 770). The test–retest reliability of the bioelectrical impedance method was r = 0·96 to 0·99.
Strength
Maximal strength (1-repetition maximum) was determined 24 h after body composition, and skeletal muscle regulatory factors were measured. Participants were asked to abstain from alcohol for 48 h, caffeinated drinks for 12 h, food and drink (water was allowed ad libitum) for 2 h prior to testing. Following a light aerobic warm-up, participants performed two sets of repetitions to volitional fatigue (<10 repetitions) on the leg press and bench press (Technogym equipment). Each set was separated by 5 min of passive rest (intraclass correlation coefficient (ICC): 0·96 to 0·98). Maximal strength was estimated using the following formula: 1-repetition maximum = weight/(1·0278–0·0278 × repetitions)(Reference Murach and Bagley35). Bench press and leg press exercises were used to measure upper and lower body strength(Reference Krause, Crognale and Cogan36), and 1-repetition maximums were used to determine individualised resistance training prescriptions.
Skeletal muscle regulatory factors
Fasting blood samples (10 ml) were collected from the antecubital vein using standard procedures. Following blood sampling, the samples were placed at room temperature for 15 min to clot. Samples were centrifuged at 3000 rpm for 10 min, and serum was stored at −80°C for future analysis. Commercially available ELISA kits were used to determine IGF-1 (CUSABIO; sensitivity: < 1·95 ng/ml), Activin A (CUSABIO; sensitivity: 3·9 pg/ml), FST (CUSABIO; sensitivity: 0·025 ng/ml), MST (CUSABIO; sensitivity: 0·312 ng/ml), TGF-β1 (CUSABIO; sensitivity: 0·747 ng/ml) and GDF15 (CUSABIO; sensitivity: 1·95 pg/ml). All serum sample concentrations were measured with a microplate reader (GDV, Germany) at a wavelength of 450 nm. The intra- and inter-assay CV for IGF-1 was < 10 % and < 12 %, Activin A, TGF-β1, and GDF15, < 8 % and 10 %, FST and MST < 12 %, respectively.
Diet
Participants filled out dietary logs (two weekdays and one weekend day) at baseline and immediately after the study (daily dietary habits and supplements’ nutrients). Food items were entered and analysed (Diet Analysis Plus, version 10; Cengage) to determine changes in total energy (kcal), carbohydrate, fat and protein over time(Reference Bagheri, Rashidlamir and Motevalli5).
Statistical analyses
The normality of the data was confirmed using the D’Agostino & Pearson test. Based on data from previous studies evaluating muscular outcomes following resistance training combined with different protein supplementations in older adults(Reference Krause, Crognale and Cogan36,Reference Maltais, Perreault and Courchesne-Loyer37) , it was calculated that twelve participants per group would provide 80 % power (two-sided α = 0·05) to detect 7 % between-group changes in lean mass and muscular strength. Unpaired t tests examined a comparison of baseline descriptive characteristics. The effect of group (placebo, Icelandic yogurt) and time (prior, post) was examined throughout using repeated measures (within (time), between (group)) ANOVA. Significant interactions were followed up using Bonferroni post hoc analyses. Pearson’s linear regression was used to examine the relationship between continuous variables with an r 2 value of > 0·02, 0·13 and 0·26 as the threshold for a weak, moderate and substantial effect(Reference Cohen38). A P-value of < 0·05 was considered significant throughout. GraphPad Prism (version 8.4.3) was used for all statistical analysis and figure production.
Results
Compliance, adverse events, diet and training volume
Compliance with the nutritional interventions and resistance training programme was > 90 %. One participant from each group withdrew because of personal reasons not related to the study. No adverse events were reported from Icelandic yogurt, placebo, or the resistance training programme. There were no significant differences at baseline between groups for any variable (Table 2). There were group × time interactions for total energy (kcal; P = 0·002), absolute protein (g/d; P < 0·001) and relative protein intake ((g/kg per d; P < 0·001), (Table 3)). Total energy intake was higher at the end of the study (compared with baseline) in the IR group (baseline: 1755·8 ± 46·7 kcal/d; post: 1812 ± 43·7 kcal/d, P = 0·002) with no change in the PR group (baseline: 1716·6 ± 50·1 kcal; post: 1729 ± 72 kcal/d, P = 0·648). Similarly, absolute protein intake increased in the IR group over time (baseline: 78·7 ± 5·5 g/d; post: 97·2 ± 5·9 g/d, P < 0·001) with no change in the PR group (baseline: 78·0 ± 5·1 g/d; post: 79·1 ± 4·1 g/d, P = 0·809). Compared with baseline, relative protein intake increased in the IR group over time (pre 1·3 ± 0·9 g/kg per d, post 1·6 ± 0·1 g/kg per d) with no change in the PR group (pre 1·2 ± 0·1 g/kg per d, post 1·3 ± 0·1 g/kg per d). There were no differences between groups for total training volume performed over the eight weeks of resistance training (IR: 106 555 ± 7171 kg; PR: 102 184 ± 6361 kg; P = 0·100).
Table 2. Descriptive characteristics of participants’ values represent mean and standard errors. P-values indicate unpaired sample t test (IR v. PR)
(Mean values and standard errors, n 14 per group)
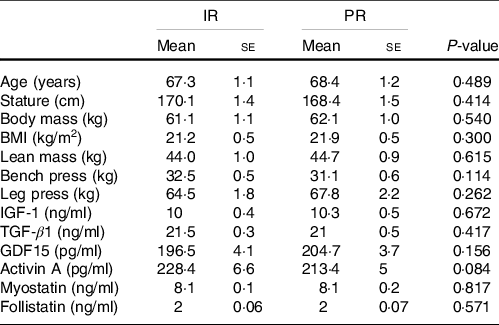
IGF-1, Insulin-like growth factor 1; TGF-β1, Transforming growth factor-beta 1; GDF15, Growth differentiation factor 15; IR, Icelandic yogurt; PR, placebo.
Table 3. Energy and macronutrients
(mean values and standard deviations)
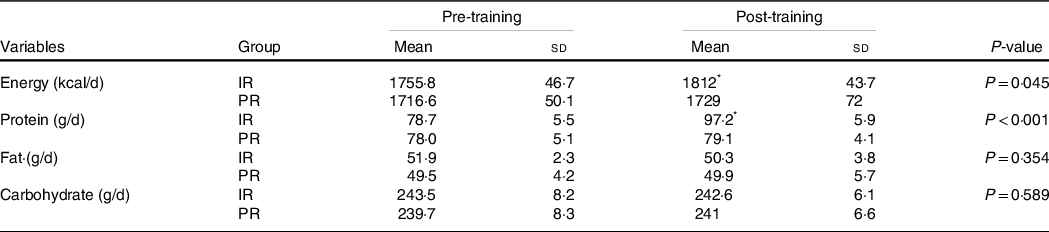
IR, Icelandic yogurt; PR, placebo. ‘P value’ column indicates condition × time interaction.
* Indicates difference between time points within group (P < 0·05).
Body composition and physical performance
There were significant group × time interactions (P < 0·05) for body mass (IR: Δ 1 kg, 95 % CI: 0·5, 1·4; PR: Δ 0·7 kg, 95 % CI: 1·2, 0·3), BMI (IR: Δ 0·3 kg/m2, 95 % CI: 0·2, 0·5; PR: Δ 0·2 kg/m2, 95 % CI: 0·4, 0·1), lean mass (IR: Δ 1·3 kg, 95 % CI: 0·9, 1·6; PR: Δ 0·6 kg, 95 % CI: 0·2, 1), bench press (IR: Δ 4 kg, 95 % CI: 2·8, 5; PR: Δ 2·3 kg, 95 % CI: 1·7, 2·9) and leg press (IR: Δ 4·2 kg, 95 % CI: 3·4, 5·1; PR: Δ 2·5 kg, 95 % CI: 2·1, 3). Participants in the IR group experienced greater changes in body mass (P < 0·001; Fig. 1(b)), BMI (P < 0·001; Fig. 1(d)), lean mass (P = 0·012; Fig. 1(f)), bench press (P = 0·012; Fig. 1(h)) and leg press (P = 0·001; Fig. 1(j)) compared with those in the PR group. However, fat mass remained unchanged in both groups (P > 0·05).
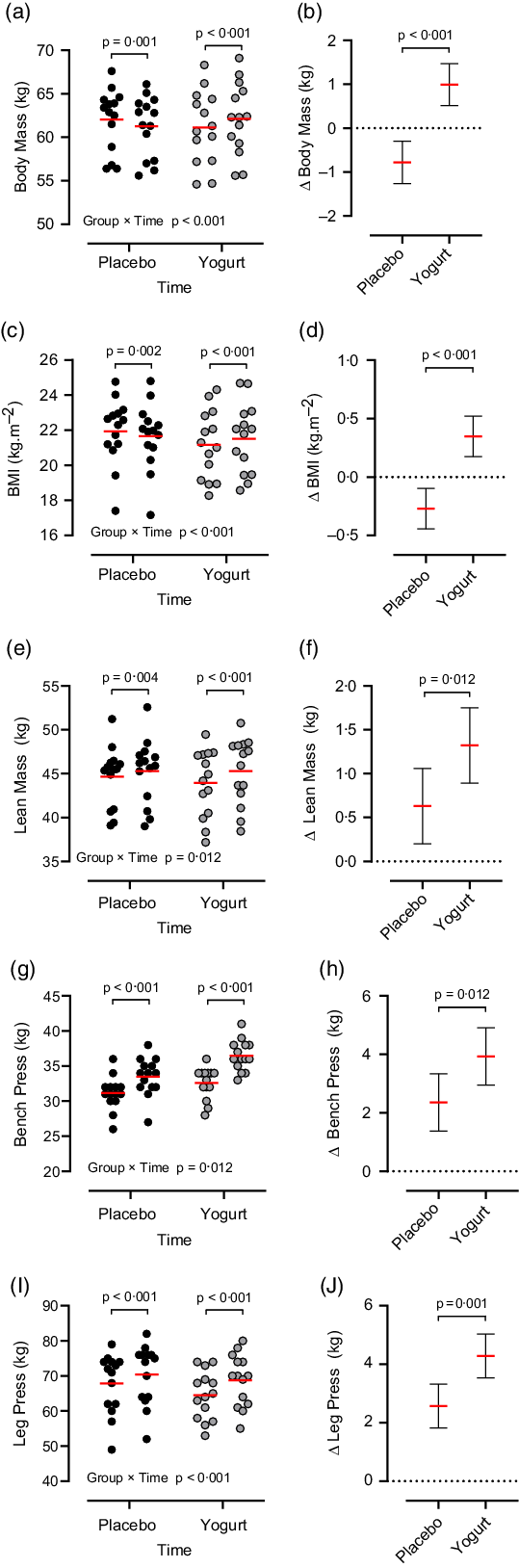
Fig. 1 Effect of training time (pre, post) and experimental group (Icelandic yogurt, placebo) on body composition and strength. Red horizontal lines indicate group means; error bars indicate 95 % CI. n 14 per group, placebo indicated by black circles, yogurt indicated by grey circles. (a) Body Mass and (b) Δ Body Mass, (c) BMI and (d) Δ BMI (kg/m2), (e) Lean Mass and (f) Δ Lean Mass (kg), (g) Bench Press and (h) Δ Bench Press (kg), and (i) Leg Press and (j) Δ Leg Press (kg). , Placebo;
, Yogurt.
Skeletal muscle regulatory factors
There were significant group × time interactions (P < 0·05) for IGF-1 (IR: Δ 0·5 ng/ml, 95 % CI: 0·3, 0·6; PR: Δ 0·1 ng/ml, 95 % CI: 0·08, 0·2), TGF-β (IR: Δ –0·2 ng/ml, 95 % CI: –0·2, –0·1; PR: Δ –0·1 ng/ml, 95 % CI: –0·1, –0·05), GDF15 (IR: Δ –10·3 pg/ml, 95 % CI: –13·6, –7; PR: Δ –4·8 pg/ml, 95 % CI: –9, –0·7), Activin A (IR: Δ –9·8 pg/ml, 95 % CI: –12·3, –7·2; PR: Δ –2·9 pg/ml, 95 % CI: –5·6, –0·2), MST (IR: Δ –0·1 ng/ml, 95 % CI: –0·1, –0·08; PR: Δ –0·04 ng/ml, 95 % CI: –0·06, –0·02) and FST (IR: Δ 0·09 ng/ml, 95 % CI: 0·06, 0·1; PR: Δ 0·03 ng/ml, 95 % CI: 0·02, 0·05). The changes in IGF-1 (P < 0·001; Fig. 2(b)), TGF-β1 (P = 0·003; Fig. 2(d)), FST (P = 0·002; Fig. 2(l)), GDF15 (P = 0·034; Fig. 2(f)), Activin A (P < 0·001; Fig. 2(h)) and MST (P < 0·001; Fig. 2(j)) were significantly greater in the IR compared with PR group.
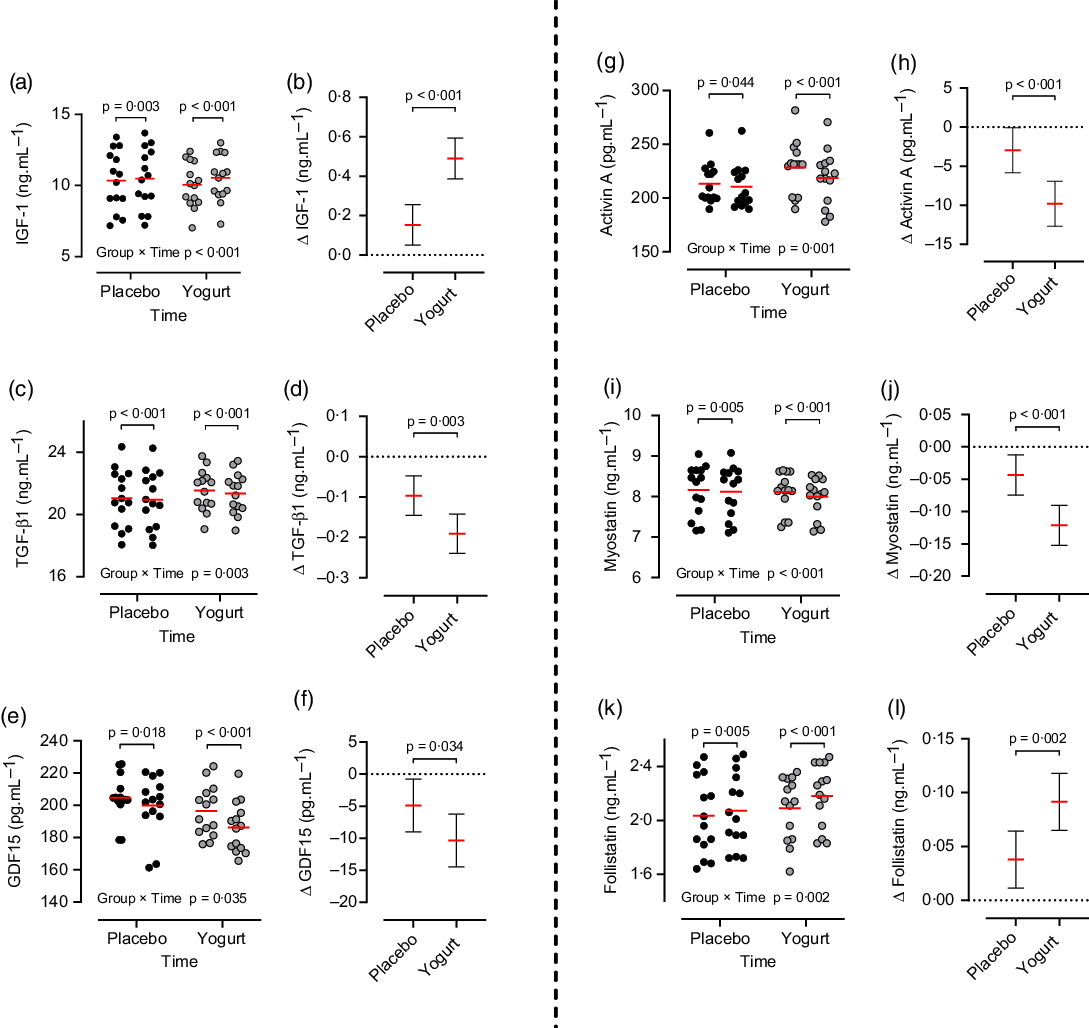
Fig. 2 Effect of training time (pre, post) and experimental group (Icelandic yogurt, placebo) on skeletal muscle regulatory factors. Red horizontal lines indicate group means; error bars indicate 95 % CI. n 14 per group, placebo indicated by black circles, yogurt indicated by grey circles. (a) IGF-1 and (b) Δ IGF-1 (ng/ml), (c) TGF-β1 and (d) Δ TGF-β1 (ng/ml), (e) GDF15 and (f) Δ GDF15 (pg/ml), (g) Activin and (h) Δ Activin A (pg·ml1), (i) Myostatin and (j) Δ Myostatin (pg/ml), and (k) Follistatin and (l) Δ Follistatin (ng/ml). , Placebo;
, Yogurt.
Correlations
There was a moderate correlation (r 2 = 0·160; P = 0·035) between the change in Activin A concentration and change in lean mass over time (Fig. 3(d)), with no other significant correlations (P > 0·05).

Fig. 3 Relationship between Δ lean mass (kg) and Δ skeletal muscle regulatory factors. Solid red line indicates linear regression; red-shaded area indicates 95 % CI. n 14 per group, placebo indicated by black circles, yogurt indicated by grey circles. (a) Δ IGF-1 (ng/ml), (b) TGF-β1 (ng/ml), (c) GDF15 (pg/ml), (d) Δ Activin A (pg/ml), (e) myostatin (ng/ml), and (f) follistatin (ng/ml). , Placebo;
, Yogurt.
Discussion
This was the first study to examine the effects of Icelandic yogurt consumption during a supervised whole-body resistance training programme in healthy untrained older males who were consuming > 1·2 g/kg per d of dietary protein. Results showed that Icelandic yogurt consumption augmented resistance training gains in lean mass and strength and influenced skeletal muscle regulatory factors compared with placebo. There were no adverse events reported from the nutritional intervention or resistance training programme. Icelandic yogurt consumption was also effective at increasing protein and total energy consumption, which is evident by the higher values on these markers in the IR compared with the PR group (Table 3).
The greater increase in lean mass and strength from Icelandic yogurt consumption in healthy untrained older males supports previous findings in untrained younger males. For example, Bridge et al. showed that Greek yogurt consumption (20 g protein/serving, 3 servings on training days (60 g of protein in total) and 2 servings on non-training days (40 g of protein in total)) during a supervised whole-body resistance training programme (3 times per week for 12 weeks) significantly increased fat-free mass (P = 0·046), elbow flexor muscle thickness (P = 0·004) and measures of strength (chest press (P = 0·026) and leg extension (P = 0·004)) in males (18–25 years) compared with those on an isoenergetic placebo (pudding; 0 g protein)(Reference Bridge, Brown and Snider15). Greek yogurt resulted in greater absolute and relative protein intake over time compared with those on placebo (P < 0·001) and total energy intake (pre v. post), but this did not reach statistical significance. Mechanistically, the mechanical stimulus from resistance training increases the rates of muscle protein synthesis, which are further elevated in the presence of dietary proteins(Reference Damas, Phillips and Vechin39). Over time (i.e., a resistance training programme) could lead to significant muscle accretion and strength. In addition to its protein content, yogurt also contains Ca and vitamin D. In a systematic review performed by vanDronkelaar et al. (2018), Ca levels were inversely associated with the incidence of sarcopenia, possibly because of altered Ca absorption or homoeostasis in ageing muscle(Reference van Dronkelaar, van Velzen and Abdelrazek40). Furthermore, Ca is dependent on vitamin D for absorption, and vitamin D levels have been shown to be lower in older adults with sarcopenia(Reference Robinson, Reginster and Rizzoli4,Reference van Dronkelaar, van Velzen and Abdelrazek40) . Further, vitamin D supplementation improved tasks of muscle function in older adults(Reference Robinson, Reginster and Rizzoli4).
The present study involving Icelandic yogurt consumption (18 g of protein) is somewhat comparable to other dairy-based interventions in older adults. Nakayama et al. (2020) showed that 6 months of milk protein consumption (10 g/d) during body weight and medicine ball exercise training significantly increased lean mass over time in older adults (n 61; 71 years; relative protein intake: 1·28 g/kg per d) compared with no change for those consuming a placebo (n 61; 70 years; relative protein intake: 1·23 g/kg per d)(Reference Nakayama, Saito and Sanbongi41). In addition, 6 months of high-intensity resistance training combined with whey protein improved muscle cross-sectional area and strength in mobility-limited older adults (70–85 years) who were consuming 1·2 g/kg per d of protein at the end of the intervention(Reference Chalé, Cloutier and Hau42). Twelve weeks of whey protein combined with resistance training significantly increased muscle mass, muscular strength and functional capacity in older women who consumed 1·4 g/kg per d of protein(Reference Nabuco, Tomeleri and Sugihara Junior43). Furthermore, Hevia-Larraín et al. showed that a protein intake of about 1·6 g/kg per d (regardless of protein source) had a positive effect on gains in muscle mass and strength(Reference Hevia-Larraín, Gualano and Longobardi44). In the present study, participants consumed 1·6 g/kg per d of protein (including Icelandic protein), which resulted in lean mass and strength gains. Collectively, findings across studies indicate that older adults may experience some muscle benefits when consuming > 1·2 g/kg per d of protein, including dairy-based protein food sources. Presently, there is a lack of research directly comparing different dairy food sources in conjunction with resistance training on muscle adaptations.
While direct mechanistic actions of muscle protein synthesis and breakdown were not measured in this study, we did measure several skeletal muscle regulatory factors purported to alter muscle accretion. MST is a potent inhibitor of muscle growth and binds to muscle Activin Type II receptors activating the intracellular SMAD protein signalling pathway(Reference Elliott, Renshaw and Getting45). MST may inhibit muscle hypertrophy by decreasing the mechanistic target of rapamycin complex 1 and increasing forkhead box protein O1. FST acts as an antagonist to MST with both paracrine and autocrine effects and is purported to increase muscle accretion(Reference Tortoriello, Sidis and Holtzman46). Our findings support previous studies showing both reduction in MST and an increase in FST(Reference Bagheri, Rashidlamir and Motevalli5,Reference Pourabbas, Bagheri and Hooshmand Moghadam47–Reference Bagheri, Forbes and Candow50) following resistance training. We observed a reduction in MST, TGF-β1, Activin A and GDF15 and an increase in IGF-1 and FST in both groups. These aforementioned decrements and increments were significantly greater in the Icelandic yogurt consumption group compared with the placebo. The reduction in TGF-β1 may be associated with alterations in MST since there is a co-regulatory relationship within skeletal muscle(Reference Zhu, Li and Shen51). In addition, FST has been indicated to stimulate muscle hypertrophy through the proliferation of satellite cells and MST and Activin A inhibition(Reference Gilson, Schakman and Kalista52). Further, the expression of IGF-1 within skeletal muscle following resistance training has been suggested to play a critical role in skeletal muscle accretion(Reference Barbé, Kalista and Loumaye53). IGF-1 is a regulator of the phosphoinositide 3-kinase and protein kinase B pathway and is widely considered required for activating the signal transduction for the initiation of muscle protein synthesis following mechanical loading(Reference Spangenburg27). In agreement with our findings, a recent systematic review and meta-analysis reports the positive association of increments of IGF-1 with resistance training(Reference Jiang, Lou and Hou54). Interestingly, despite resistance training clearly altering several known regulators of muscle accretion, we only found one modest correlation (r 2 = 0·160; P = 0·035) between the change in Activin A and lean mass. Future research is warranted to directly measure acute and chronic alterations following resistance training with muscle protein synthesis.
Many older individuals experience several barriers to exercise(Reference Hickey and Mason55); therefore, one important strength of this study was the participants’ high adherence level to our exercise intervention. This investigation is limited by the absence of measurements of skeletal muscle anabolism (mechanistic target of rapamycin complex 1 signalling, MPS) which would have assisted in the explanation of our outcomes. However, it has been proposed that promotions in circulating concentrations of signalling molecules increase the likelihood of a receptor interaction, and therefore a biological effect within skeletal muscle(Reference Kraemer, Marchitelli and Gordon56,Reference Ahtiainen, Pakarinen and Alen57) . Second, we did not include a yogurt-only group. However, the effects of regular yogurt consumption have been previously indicated(Reference White, Bauer and Hartz20,Reference Thomas, Wideman and Lovelady21) , which showed no further effects on muscular gains after regular resistance training. The lack of positive effects of regular yogurt consumption may be due to the lower amounts of protein (5 g of protein per serving), highlighting the importance of higher amounts of protein to induce significant effects on muscular gains. Given the importance of higher protein intakes in older adults due to the prevalence of anabolic resistance(Reference Bauer, Biolo and Cederholm58,Reference Deutz, Bauer and Barazzoni59) and a lower amount of protein in regular yogurt, we did not incorporate regular yogurt consumption. Additionally, bioelectrical impedance was used to measure body composition, which is not as precise as dual-energy X-ray absorptiometry (the gold standard technique for body composition measurement); however, previous studies have shown that it is a valid and reliable method(Reference Ling, de Craen and Slagboom60,Reference Jackson, Pollock and Graves61) .
In conclusion, post-exercise Icelandic yogurt consumption augmented resistance training gains in lean mass, strength and altered skeletal muscle regulatory factors in healthy untrained older males. This is critical for older populations as increases in lean mass and strength may prevent sarcopenia as well as improve the risk of falls and enhance independent living(Reference McKendry, Currier and Lim1,Reference Talar, Hernández-Belmonte and Vetrovsky62) . Future research should investigate the effects of Icelandic yogurt consumption, with and without resistance training on measures of muscle and bone in younger and older adults.
Acknowledgements
The authors wish to thank all the participants in this research project.
This work was financially supported by a grant (50916/2) from the Vice Chancellor for Research Affairs, Ferdowsi University of Mashhad.
A. R. and B. H. M. conceived and designed research. B. H. M. and A. R. conducted experiments. A. R. and R. B. contributed new reagents or analytical tools. B. T. E. analysed data. R. B. wrote the manuscript. A. W., D. G. C., S. C. F., and D. A. L. revised the manuscript. All authors read and approved the manuscript.
The authors declare no conflicts of interest.