1. Introduction
This paper synthesises the human–landscape interactions in the Netherlands during the first millennium AD. During this period a mainly natural, prehistorical lowland landscape became increasingly dominated by humans. This period furthermore is special because it saw a strong fluctuation in population pressure (Groenewoudt & Van Lanen, Reference Groenewoudt and Van Lanen2018; Van Lanen, Reference Van Lanen2020) and vegetation cover ( e.g. Teunissen, Reference Teunissen1990). Moreover, abundant geomorphological changes took place (Jansma et al., Reference Jansma, Gouw-Bouman, Van Lanen, Pierik, Cohen, Groenewoudt, Hoek, Stouthamer and Middelkoop2014; Pierik, Reference Pierik2017a; Figs 1 and 2). The Netherlands provides a wealth of data to study the interactions between people and the landscape. Changes in the landscape and their potential causes can be inferred from geomorphology – often clearly visible in the current landscape – and from sedimentological records and archaeology in the shallow substrate.
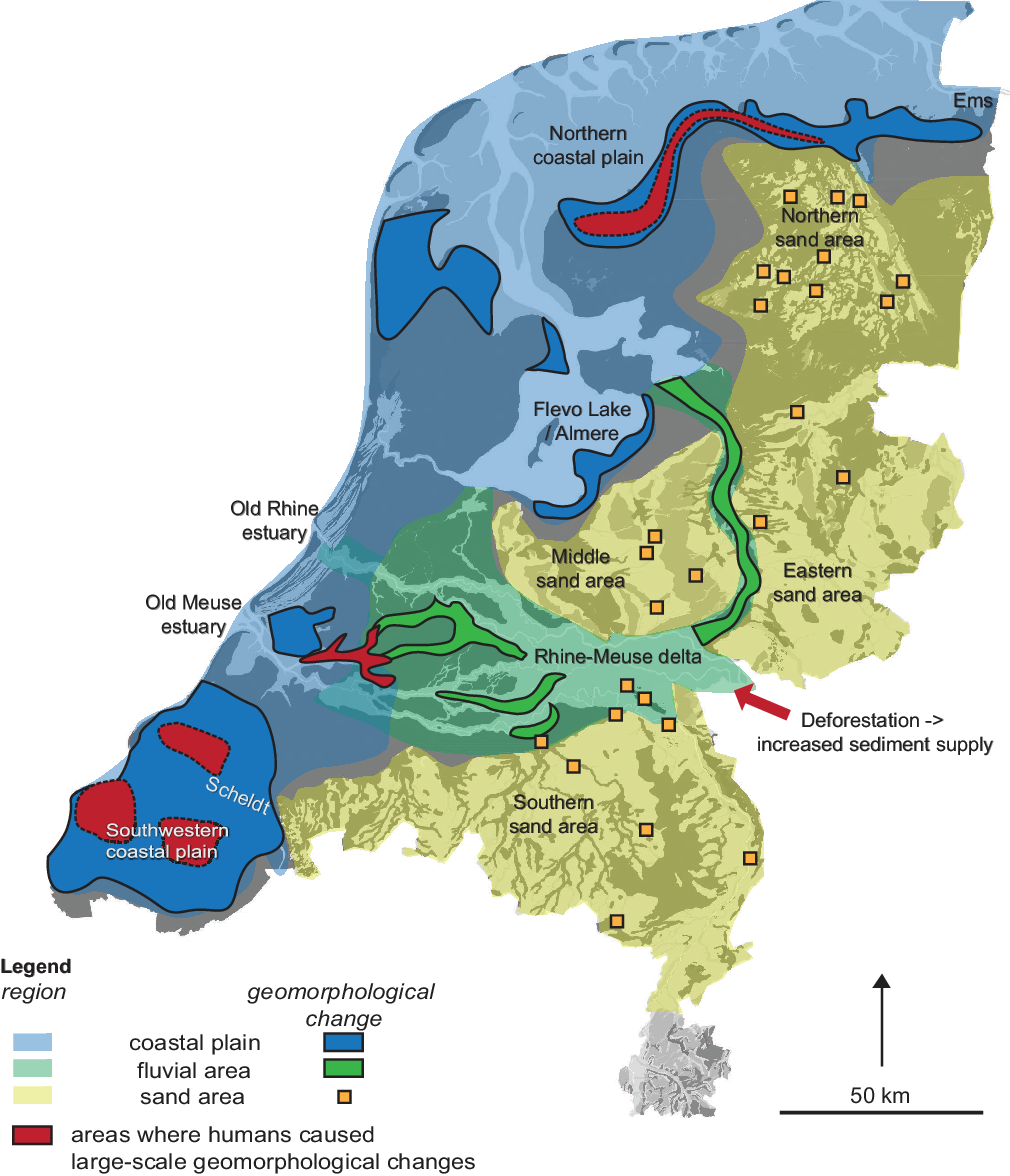
Fig. 1. Main landscape changes in the first millennium AD discussed in this paper. Human land-use activities in the red areas caused landscape changes in the coastal plain and the Rhine–Meuse delta. The yellow squares indicate human-induced local drift-sand activity. Background palaeogeography AD 800 (Vos & De Vries, Reference Vos and de Vries2013).
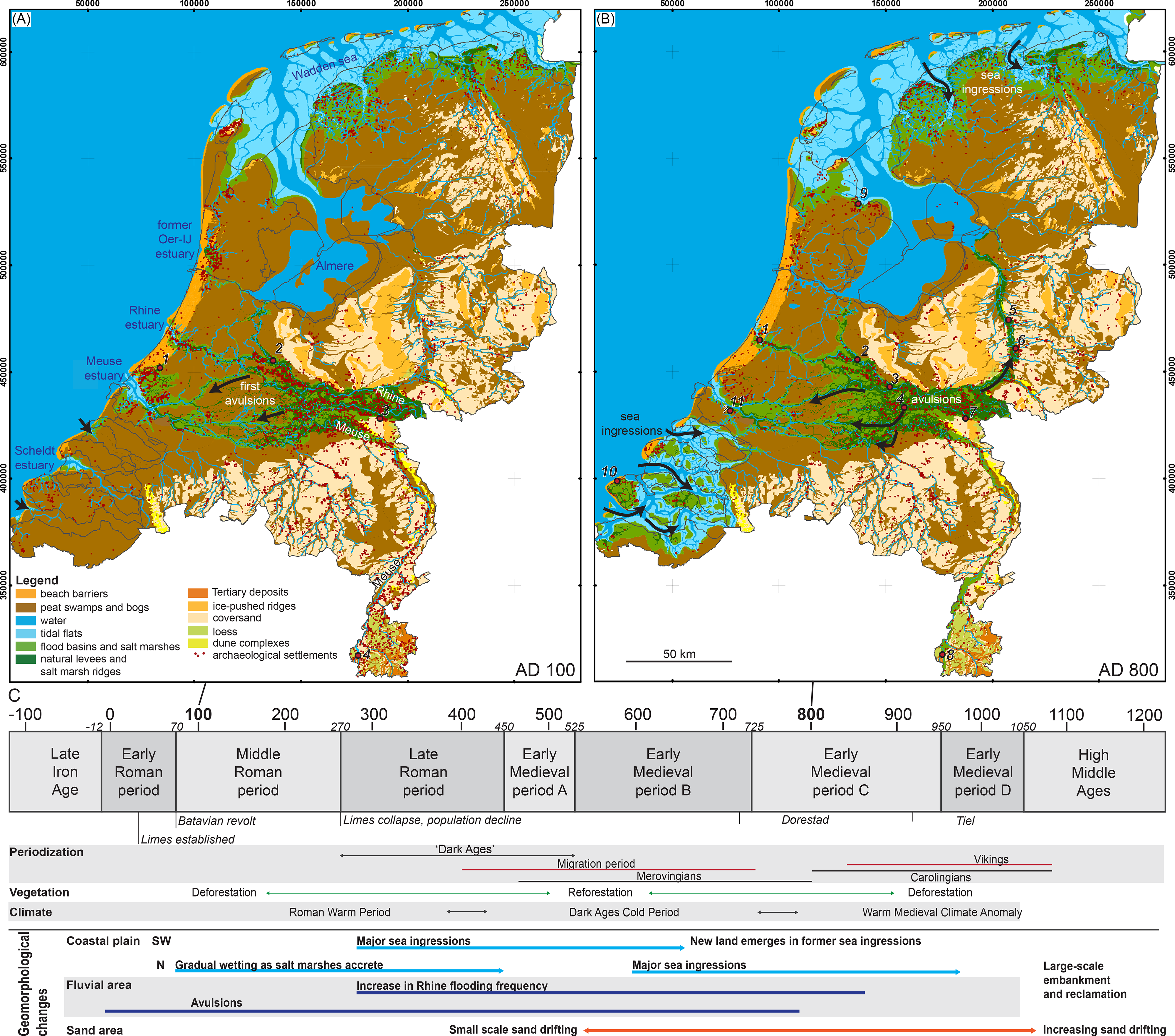
Fig. 2. Palaeogeographical maps of (A) AD 100 and (B) AD 800 (Vos & De Vries, Reference Vos and de Vries2013). Natural levees in Rhine–Meuse delta (Pierik, Reference Pierik2017b ; Pierik et al. Reference Pierik, Stouthamer and Cohen2017b), archaeological settlements of the Roman period and early medieval period respectively from Van Lanen et al. (Reference Van Lanen, Kosian, Groenewoudt and Jansma2015). Important settlements for the Roman period and the Early Middle Ages are numbered A: 1 = Forum Hadriani (Voorburg), 2 = Utrecht, 3 = Nijmegen, 4 = Maastricht; B: 1 = Oestgeest, 2 = Utrecht, 3 = Dorestad, 4 = Tiel, 5 = Deventer, 6 = Zutphen, 7 = Nijmegen, 8 = Maastricht, 9 = Medemblik, 10 = Domburg, 11 = Witla. (C) Timeline with periodisation in the first millennium AD in the Netherlands.
Just before the first millennium AD, in the Late Iron Age (250–12 BC), first reclamation works marked a small-scale, yet distinct human influence in the wetlands of the Netherlands. This involved the construction of dams, culverts, canals and ditches (e.g. Ter Brugge et al., Reference Ter Brugge, Carmiggelt, Guiran and Van Trierum2002; Van Londen, Reference Van Londen2006; Vos, Reference Vos2009). At the start of the first millennium AD, the northern border of the Roman Empire (limes) was located along the river Rhine (Willems, Reference Willems1986; Van Dinter, Reference Van Dinter2013). Around the limes, and also in other parts of the Netherlands, population density was substantially higher than in earlier periods (Groenewoudt & Van Lanen, Reference Groenewoudt and Van Lanen2018; Van Lanen et al., Reference Van Lanen, De Kleijn, Gouw-Bouman and Pierik2018). From the late 3rd century AD, however, depopulation occurred, coinciding with the collapse of the Western Roman Empire (Cheyette, Reference Cheyette2008; Wickham, Reference Wickham2009; Heeren, Reference Heeren2015) and the large-scale migration of tribes throughout NW Europe (e.g. Halsall, Reference Halsall2007). This period has traditionally been referred to as the ‘Dark Ages’ (e.g. James, Reference James1988). This term on one hand refers to a period of cultural decline and disorder, and on the other hand it is used for periods in general from which little information is available. The depopulation trend of the 3rd and 4th centuries AD caused forest regeneration in many parts of NW Europe, including the Netherlands (Teunissen, Reference Teunissen1990; Louwe Kooijmans, Reference Louwe Kooijmans1995; Kaplan et al., Reference Kaplan, Krumhardt and Zimmermann2009). The dip in the number of archaeological finds and population density has traditionally been attributed to cultural (political and economic; e.g. Halsall, Reference Halsall2007) and climatic factors (Büntgen et al., Reference Büntgen, Tegel, Nicolussi, McCormick, Frank, Trouet, Kaplan, Herzig, Heussner, Wanner, Luterbacher and Esper2011, Reference Büntgen, Myglan, Ljungqvist, McCormick, Di Cosmo, Sigl, Jungclaus, Wagner, Krusic, Esper, Kaplan, De Vaan, Luterbacher, Wacker, Tegel and Kirdyanov2016; McCormick et al., Reference McCormick, Büntgen, Cane, Cook, Harper, Huybers, Litt, Manning, Mayewski, More, Nicolussi and Tegel2012; Gouw-Bouman et al., Reference Gouw-Bouman, Van Asch, Engels and Hoek2019 – Dark Ages Cold period). However, major landscape changes may also have played a role (Figs 1 and 2), such as sea ingressions (Vos & Van Heeringen, Reference Vos and Van Heeringen1997; Vos, Reference Vos2015; Pierik et al., Reference Pierik, Cohen, Vos, van der Spek and Stouthamer2017a), reorganising river networks (Pons, Reference Pons1957; Törnqvist, Reference Törnqvist1993; Berendsen & Stouthamer, Reference Berendsen and Stouthamer2000; Pierik et al., Reference Pierik, Stouthamer, Schuring and Cohen2018a) and changing hinterland palaeohydrology (Berendsen & Stouthamer, Reference Berendsen and Stouthamer2000; Erkens et al. Reference Erkens, Hoffmann, Gerlach and Klostermann2011; Toonen et al., Reference Toonen, Foulds, Macklin and Lewin2017; Jansma, Reference Jansma2020). From the 7th century onwards, population numbers and human pressure on the landscape started to increase again. This trend persisted throughout the Middle Ages (Van Bavel, Reference Van Bavel2010; Groenewoudt & Van Lanen, Reference Groenewoudt and Van Lanen2018), and around AD 1100, major parts of the fluvial and coastal area had been reclaimed and embanked (Borger, Reference Borger and Verhoeven1992; De Bont, Reference De Bont2008). From that time onwards, the natural erosional and sedimentary processes were confined to narrow areas in tidal basins or embanked river plains (Hudson et al., Reference Hudson, Middelkoop and Stouthamer2008) and local coastal and inland dune areas (Koster et al., Reference Koster, Castel and Nap1993; Vos et al., Reference Vos, De Koning and Van Eerden2015a).
Many of these landscape changes were slow but large responses to a relatively small trigger and forcing (Phillips, Reference Phillips2003; Verstraeten et al., Reference Verstraeten, Broothaerts, Van Loo, Notebaert, D’Haen, Dusar and de Brue2017). Striking differences, however, are found in the degree to which geomorphological changes occurred across the country. Settlement locations suggest that human influence was widespread in the Netherlands throughout the first millennium AD, but only at distinct locations did human land-use practices lead to landscape changes (Fig. 1). These landscape changes are reviewed in this paper. The causes behind them are discussed, as well as the main feedbacks, spatial differences and their varying impact on humans. Human–landscape interactions are compared between three regions within the Netherlands with distinct geomorphological settings: the coastal plain, the fluvial area (Rhine–Meuse delta) and the Pleistocene sand area (Fig. 1). For this regional comparison, the stable states theory (Scheffer et al., Reference Scheffer, Carpenter, Foley, Folke and Walker2001) is used. This theory has been developed for the field of ecology and may also be valuable for describing to what extent external pressures change landscapes. Landscapes may be pushed into alternative states where new feedbacks dominate, in this case a new set of geomorphological processes and human–landscape interactions.
2. Approach and materials in landscape reconstruction
This paper summarises geomorphological changes in the Dutch landscape by building on existing regional-scale landscape reconstructions and on human–landscape interaction studies (e.g. Van Dinter, Reference Van Dinter2013; Vos, Reference Vos2015; Pierik, Reference Pierik2017a; Nieuwhof et al., Reference Nieuwhof, Bakker, Knol, de Langen, Nicolay, Postma, Schepers, Varwijk and Vos2019). These studies integrated stratigraphical, geomorphological and chronological data from often fragmented data (Pierik et al., Reference Pierik, Cohen and Stouthamer2016; Cohen et al., Reference Cohen, Dambrink, De Bruijn, Marges, Erkens, Pierik, Koster, Stafleu, Schokker and Hijma2017), that may include maps, local reports, borehole databases, as well as results from Malta-driven archaeological studies (Pierik & Cohen, Reference Pierik and Cohen2020). Landscape changes from these studies are compared to changes in the archaeological record to unravel human–landscape interactions. This is done for the three regions to better understand the relative effects of initial landscape, as well as human and natural changing conditions.
2.1 Assessing the degree of landscape change
Differences in landscape resilience, thresholds, triggers, feedbacks and impact of the landscape changes are evaluated and compared for the three regions, following definitions from the stable states theory of Scheffer et al. (Reference Scheffer, Carpenter, Foley, Folke and Walker2001). This theory describes how a system – in this case a geographical area with certain human–landscape interactions – can have multiple stable states. For example, a bog peat suited for reclamation can change into drowned tidal areas, which again can change into infilled tidal areas with dry places to live. Each state comprises its own set of distinct feedbacks, i.e. geomorphological processes and human–landscape interactions. Under varying environmental conditions, these areas can shift into different states (regime shift), but this does not happen via a linear response. Instead, these states are separated by a tipping point, essentially a point of no return, flipping the system into a contrasting self-stabilising state with its own distinct processes and feedbacks. Restoring earlier environmental conditions will not automatically recover the system’s previous state. The stability of such a state is known as resilience. A larger resilience will require a larger perturbation to cause a shift to an alternative stable state. The resilience may be affected by gradually changing conditions that may initially have little direct effect on the state of the landscape (e.g. slow peatland subsidence in response to reclamation). Over the course of time, however, such gradual conditions could make a landscape more fragile, so that it can more easily tip into an alternative state by stochastic events (e.g. by flood events). The concepts explained above will be used in this study to compare the size and impact of the landscape changes in the first millennium AD. These may range from almost no change (because of high resilience) to a full regime shift. They may also include significant changes, while still maintaining a comparable landscape with similar feedbacks and human–landscape interactions.
2.2 Data resolution and possible drawbacks
The quality of the reconstruction-based studies that underlie this review heavily depends on the resolution of the underlying landscape reconstructions and the degree to which the main driving factors could be assessed. In the coastal and fluvial area, new integrated GIS landscape reconstructions with a uniform spatial coverage presented in Pierik et al. (Reference Pierik, Cohen and Stouthamer2016, Reference Pierik, Cohen, Vos, van der Spek and Stouthamer2017a,b) show and analyse the main landscape changes. These studies mainly use existing maps in the coastal area, whereas in the fluvial area high-quality borehole data were used, providing high spatial and chronological resolution. In the Pleistocene sand area, a more site-based approach was applied or drift-sand reconstruction rather than a uniformly covering reconstruction (Pierik et al., Reference Pierik, Van Lanen, Gouw-Bouman, Groenewoudt, Wallinga and Hoek2018b), because of the patchy distribution of drift sands before the 17th century. Start and end ages of individual sites are generally dated within a 500-yr range. When optically stimulated luminescence (OSL) dates and/or historical references were used, the accuracy of driftsand activity can be several decades. By combining multi-proxy evidence of regional drift-sand activity from c.140 sites and 340 dates, the trends unravelled in drift-sand activity have a maximum age uncertainty of one to two centuries.
The factors that could have driven landscape changes either are natural or human-induced. Independent sedimentological records, for example, may reveal flood events as natural triggers. Individual severe floods generally were dated more precisely with 30–150 yrs precision, or on the resolution of a single year using dendrochronology (Jansma, Reference Jansma2020), whereas floods dated based on archaeology were dated at a 20–200 yr resolution (Toonen et al., Reference Toonen, Donders, Van der Meulen, Cohen and Prins2013; Cohen et al., Reference Cohen, Toonen and Weerts2016). Settlement dynamics from the archaeological record were used both as an indication for human pressure and as an indication of human response to landscape change. Often, archaeological finds are additionally used to date geomorphological changes. To avoid potential circular reasoning, independent additional proof of new absolute dates and stratigraphical correlations in the data-rich study area were used where possible. For example, archaeological finds in the coastal plain on top of peat covered by clay layers indicate reclamation activity. They were additionally used to date the peatland drowning indicated by the clay layers, assuming that peatland reclamation caused the drowning. The causal relation then had to be proven independently, for example by assessing additional evidence of the identified reclamation (rectangular in-filled tidal channels) and by using additional dates of associated materials (e.g. 14C of the peat or shells inside the tidal deposits). Another challenge in inferring geomorphological processes from reconstructions is that multiple processes can lead to similar outcomes (equifinality) and that local processes propagating to larger areas may be overlooked. This was partly circumvented by regional upscaling to allow comparison of the changes for multiple cases (rivers, tidal systems, sand areas), with well-known differing natural preconditions and forcings.
3. Geomorphological evolution during the first millennium AD
3.1 Coastal plain
In the first millennium AD, large tidal areas were present in the coastal plain with channels, shoals, supratidal saltmarshes, and coastal peat swamps (Figs 2 and 3). The coastal plain width ranged from c.60 km in the southwest and Holland to c.30 km in the northern Netherlands and includes the Almere lagoon in the middle part of the Netherlands. In the northern Netherlands the Wadden Sea was fringed by saltmarshes that became inhabited c.650 BC (Taayke, Reference Taayke1996). This area became one of the most densely populated areas in the Netherlands during the first millennium AD (Groenewoudt & Van Lanen, Reference Groenewoudt and Van Lanen2018). Settlements here were mainly confined to artificial dwelling mounds that protected the inhabitants from the highest water levels (Van Giffen, Reference Van Giffen1940; Gerrets, Reference Gerrets2010; Nieuwhof et al., Reference Nieuwhof, Bakker, Knol, de Langen, Nicolay, Postma, Schepers, Varwijk and Vos2019). Other densely populated areas in the coastal plain were tidal natural levees and abandoned tidal creeks around the estuaries of the Rhine, Meuse and Oer-IJ (Figs 2 and 3).

Fig. 3. (A) Three landscape states in the coastal plain (after Pierik et al., Reference Pierik, Cohen, Vos, van der Spek and Stouthamer2017a; De Haas et al., Reference De Haas, Pierik, Van der Spek, Cohen, Van Maanen and Kleinhans2018). From pristine peatlands to sea ingression dominant in the southwestern and northern Netherlands (discussed in this paper), to fully embanked and reclaimed in the Late Middle Ages. (B–F) GIS-derived coastal development since 600 BC (Pierik et al., Reference Pierik, Cohen and Stouthamer2016, Reference Pierik, Cohen, Vos, van der Spek and Stouthamer2017a). Pie charts demonstrate relative areal extent of the landscape units per coastal segment; colours correspond to the map legend units.
The tidal areas were fringed by large freshwater peat swamps. In the southwestern and northern coastal plain, these peatlands were reclaimed and cut for fuel on a large scale from the onset of the first millennium AD (Vos & Van Heeringen, Reference Vos and Van Heeringen1997; Vos & Knol, Reference Vos and Knol2015). These activities caused major landscape changes and eventually transformed a large part of the pristine coastal plain peatlands into tidal areas (Vos, Reference Vos2015; Pierik et al., Reference Pierik, Cohen, Vos, van der Spek and Stouthamer2017a – ‘sea ingression state’ in Fig. 3). These sea ingressions were mainly the effect of peatland subsidence caused by groundwater level lowering during reclamation. This was followed by considerable land losses, mainly during storm surges, as tidal channels initiated and grew by erosion of the tidal flow. The largest land losses occurred in the SW Netherlands, which was drowned for multiple centuries from AD 270 onwards; people abandoned large parts of this plain. In the northern Netherlands, ingression in peatlands probably started in the Roman age and expanded between AD 700 and 1000 (Vos & De Langen Reference Vos and de Langen2010; Vos & Knol, Reference Vos and Knol2015), most likely in response to fringing peatland reclamation from the 4th century BC onwards (Nieuwhof et al., Reference Nieuwhof, Bakker, Knol, de Langen, Nicolay, Postma, Schepers, Varwijk and Vos2019). The presence of supratidal saltmarsh levees fringing the Wadden Sea caused ingressions to be relatively smaller. Also in the NW coastal plain, barrier erosion combined with peat drainage caused tidal area expansion (Vos et al., Reference Vos, Van der Heijde and Stuurman2015b). Over the course of centuries, sediment import caused the ingressions to fill. The rate and scale of infilling depended on the extent of the ingressed area, sediment supply, flood dominance, and subsidence feedbacks (Pierik et al., Reference Pierik, Cohen, Vos, van der Spek and Stouthamer2017a). In the SW Netherlands, tidal sedimentation reached supratidal levels that became inhabited again around AD 700 (Vos & Van Heeringen, Reference Vos and Van Heeringen1997).
While most of the coastal plain experienced peatland loss in the first millennium AD, phases of peat expansion have also been observed. The natural closure of the Oer-IJ estuary around 200–150 BC (Vos et al., Reference Vos, De Koning and Van Eerden2015a) led to drainage problems generating peat expansion on the fringing saltmarshes at the onset of the first millennium. Other examples are found around the Meuse estuary (Vos, Reference Vos2015; Moree et al., Reference Moree, Van Trierum and Carmiggelt2018) and in the northern Netherlands (Layer of Tinga – Vos & De Langen, Reference Vos and de Langen2008). Both peat regrowth events have been linked to depopulation and subsequent discontinuation of maintenance of reclamation works, causing rewetting of the landscape. In the northern Netherlands, wetness of the landscape additionally increased as water routing towards the sea was hampered. This was the result of continued northward saltmarsh accretion combined with gradual sea-level rise (Vos & Knol, Reference Vos and Knol2015; Nieuwhof et al., Reference Nieuwhof, Bakker, Knol, de Langen, Nicolay, Postma, Schepers, Varwijk and Vos2019). These environmental factors may have contributed to the major population decline in the 3rd century in the northern Netherlands, besides being caused by cultural factors and large-scale migration (Nieuwhof, Reference Nieuwhof2016).
3.2 Rhine–Meuse delta
In the Rhine–Meuse delta, several river branches changed their courses around the first millennium AD (avulsion; Figs 2, 4 and 5 – Berendsen & Stouthamer, Reference Berendsen and Stouthamer2000, Reference Berendsen and Stouthamer2001; Van Dinter et al., Reference Van Dinter, Cohen, Hoek, Stouthamer, Jansma and Middelkoop2017). Stepwise, the main discharge was routed away from the Old Rhine river in the north (forming the Roman limes) to the Meuse estuary in the south of the delta. The formation of the Hollandse IJssel and Lek in the downstream delta between c.AD 1 and 300 is one of the first steps. For 3000 years, a large swamp had separated the Old Rhine and Old Meuse rivers here until these two new Rhine branches traversed it. Human-induced peat subsidence (in the current Rotterdam area) and wetland deforestation likely contributed to the avulsion success of these rivers (Pierik et al., Reference Pierik, Stouthamer, Schuring and Cohen2018a). Human-induced increased suspended sediment supply – related to deforestation in Germany – to the rivers and estuaries caused additional peat subsidence by loading processes in the lower delta, thereby probably accelerating the avulsion process. In the apex region, the Waal river gradually gained more discharge at the expense of the Nederrijn (Weerts & Berendsen Reference Weerts and Berendsen1995). The Gelderse IJssel formed, flowing outside the delta towards the central Netherlands lagoon (Almere), connected to the Wadden Sea in the north (Makaske et al., Reference Makaske, Maas and Van Smeerdijk2008; Cohen et al., Reference Cohen, Stouthamer, Hoek, Berendsen and Kempen2009, Reference Cohen, Pierik, Toonen, van der Meulen, Bomers, Gouw-Bouman, Van Popta, Jansma, Erkens, Busschers, Schuring, Volleberg, Stouthamer and Hoek2021). All these developments contributed to the gradual abandonment of the Old Rhine, which had been the main Rhine branch in the delta until the first millennium AD (Van Dinter et al., Reference Van Dinter, Cohen, Hoek, Stouthamer, Jansma and Middelkoop2017; De Haas et al., Reference De Haas, van der Valk, Cohen, Pierik, Weisscher, Hijma, van der Spek and Kleinhans2019).

Fig. 4. Geomorphological reconstructions for AD 100, 500 and 900, for the central and upper Rhine–Meuse delta (Pierik, Reference Pierik2017b; Pierik et al., Reference Pierik2017b). Cities: 1 = Utrecht, 2 = Nijmegen, 3 = Dorestad, 4 = Tiel.

Fig. 5. (A, B) River network around AD 100 and AD 900 (Jansma et al., Reference Jansma, Van Lanen and Pierik2017; Pierik et al., Reference Pierik2017b); (C) activity of major rivers in the research area after Cohen et al. (Reference Cohen, Stouthamer, Pierik and Geurts2012), Van Dinter et al. (Reference Van Dinter, Cohen, Hoek, Stouthamer, Jansma and Middelkoop2017), Pierik et al. (Reference Pierik, Stouthamer, Schuring and Cohen2018a); (D) Holocene recurrence time of floods from black to light grey: 250 yr (very severe flood), 100 yr (severe flood), 50 yr (moderately severe flood), after Toonen et al. (Reference Toonen, Donders, Van der Meulen, Cohen and Prins2013) and Cohen et al. (Reference Cohen, Toonen and Weerts2016); here the events are grouped per archaeological subperiod, and the ages of the three largest floods are indicated; orange bars indicate flood events with >50 and >100-yr recurrence intervals, derived from wood samples in the Netherlands (i.e. also outside the delta) (Jansma, Reference Jansma2020); (E) delta-wide average settlement elevation (Pierik & Van Lanen, Reference Pierik and Van Lanen2019); (F) elevation difference between persistent and abandoned settlements shows that settlements at higher locations persist more often (Pierik & Van Lanen, Reference Pierik and Van Lanen2019); (G) reconstructed number of inhabitants in the study area (after Van Lanen et al., Reference Van Lanen, De Kleijn, Gouw-Bouman and Pierik2018).
Habitation in the Rhine–Meuse delta mainly occurred on alluvial ridges, which formed the higher and drier parts of the delta (Modderman, Reference Modderman1948; Pierik & Van Lanen, Reference Pierik and Van Lanen2019). These alluvial ridges, consisting of crevasse splays and natural levees, were not only essential for delta inhabitants, they also largely controlled floodwater routing and sediment deposition (Pierik et al., Reference Pierik2017b). In the upper and central parts of the delta, avulsion paths were controlled by flood-basin and natural-levee topography. While the levees along young branches grew relatively high during the first millennium AD, the flood basins and fossil levees collected a substantial clay cover causing delta-topography levelling.
After AD 270, severe depopulation and relocation of settlements took place in the fluvial area (Fig. 5G; Hendrikx, Reference Hendrikx1983; Willems, Reference Willems1986; Van Lanen et al., Reference Van Lanen, De Kleijn, Gouw-Bouman and Pierik2018). The major part of the area was even abandoned during the 3rd century (Heeren, Reference Heeren2015). Besides cultural factors, this was possibly influenced by the varying environmental conditions. Between AD 270 and 850, preferred settlement locations on the alluvial ridges contracted and shifted towards the higher parts of the ridges, which is attributed to increased flooding intensity for this period (Fig. 5E and F; Pierik & Van Lanen, Reference Pierik and Van Lanen2019; Jansma, Reference Jansma2020). These settlement shifts hardly affected the route networks, since almost all alluvial ridges remained habitable and accessible. The strongest trends are observed in the lowest areas (Bommelerwaard, southern part of the delta). This area was initially dominated by the Meuse which had relatively low flooding activity between AD 250 and 950 (Peng et al., Reference Peng, Prins, Kasse, Cohen, Van der Putten, van der Lubbe, Toonen and van Balen2019). Around AD 450 the Waal (Rhine) branch became more dominant in this area, likely bringing higher and more frequent floods. Here, relatively many settlements were abandoned and the route networks were reorganised after the late Roman period (Pierik & Van Lanen, Reference Pierik and Van Lanen2019; Van Lanen & Pierik, Reference Van Lanen and Pierik2019).
3.3 Pleistocene sand area
The Pleistocene sand area consists of modestly elevated sandy uplands (several metres to tens of metres above sea level), with peat-infilled lower plains and brook valleys (Figs 1 and 2). Compared to the adjacent fluvial and coastal areas, this area was much less dynamic from a geomorphological perspective in the first millennium AD. Probably peat bogs were already cut for fuel locally, but large-scale peat cutting did not start before the Late Middle Ages (Spek, Reference Spek2004; Van Beek, Reference Van Beek2009). The onset of drift-sand formation – often related to deforestation or intense land use – was a second geomorphological change over the last few thousand years (Fig. 6; Koster et al., Reference Koster, Castel and Nap1993; Sevink et al., Reference Sevink, Koster, Van Geel and Wallinga2013). Drift-sand activity was mainly caused by human land-use practices (De Keyzer & Bateman, Reference De Keyzer and Bateman2018). This is evident from a peak in activity after c.AD 900 that coincided with higher population densities and larger-scale deforestation, especially in southern and middle sand areas where population increase was strongest. More frequent occurrence of drift-sand activity close to settlements and route corridors additionally indicates human pressure as an important cause behind sand drifting (Pierik et al., Reference Pierik, Van Lanen, Gouw-Bouman, Groenewoudt, Wallinga and Hoek2018b). Drift-sand activity and its impact on people in the first millennium AD were restricted to the local scale. The mid-Roman period with a high population pressure, however, counter-intuitively has low drift-sand activity. This may be linked to migration from sandy to slightly loamier areas, associated with abandonment of Celtic fields (Pierik et al., Reference Pierik, Van Lanen, Gouw-Bouman, Groenewoudt, Wallinga and Hoek2018b).

Fig. 6. Drift-sand locations in Roman Age (A) and Early Middle Ages (B). Data available via Pierik (Reference Pierik2017c).
4. Discussion
The landscape changes in the first millennium AD occurred in response to changing natural and anthropogenic conditions. Besides resulting from changing conditions, some geomorphological changes were also caused by autogenic behaviour (e.g. channel belt maturation, increasing levee height with time) (Stouthamer & Berendsen, Reference Stouthamer and Berendsen2007). On top of this, changing conditions exerted pressure on the landscape, initially without having a direct effect, but over time they made the landscape less resilient for stochastic high-energy events (e.g. storms or floods). In some cases, landscapes even shifted into an alternative state with new feedbacks (cf. Scheffer et al., Reference Scheffer, Carpenter, Foley, Folke and Walker2001). This happened via a cascade of positive feedbacks – that made the change difficult to reverse – and resulted in a new arrangement of landscape elements and their interaction with people in the landscape. This section outlines this changing interplay for the three lowland areas treated in this paper, and discusses to what extent landscapes changed or even shifted into a different state (Table 1).
Table 1. Causes, consequences, and feedbacks of geomorphological changes studied in this paper for the coastal plain, the delta plain and the sand area. (+) = positive feedback, (−) = negative feedback.

4.1 How and why did landscapes change?
4.1.1 Role of the initial landscape state in its resilience
The degree of geomorphological change under increasing human pressure strongly depends on the strength of feedbacks associated with the substrate and the geomorphology of the area. In the coastal plain, the tidal area size increased from 30% to 75%, making it the largest geomorphological change (considering areal extent) in the Netherlands in the first millennium AD. The tidal area even expanded beyond the extent of the initially reclaimed and subsided areas where the sea ingressions were provoked (compare red and blue areas in Fig. 1). The compaction-prone nature of peat in response to artificial drainage was the main factor in this landscape change. Its large areal extent in the coastal plain was furthermore decisive in the extent of the sea ingressions (Pierik et al., Reference Pierik, Cohen, Vos, van der Spek and Stouthamer2017a; Table 1). Subsequently, three strong positive feedbacks – linked to the geological and geomorphological setting of the area – accelerated a regime shift in this landscape (Pierik et al., Reference Pierik, Cohen, Vos, van der Spek and Stouthamer2017a; Table 1): (i) tidal incursion in the peat area caused higher water-level differences, leading to stronger flow enlarging the tidal channels; (ii) the weight of the imported sediments caused additional subsidence by loading (peat compression is limited by the thickness of the peat); (iii) flanking peatlands could collapse by drainage when the reclaimed peatlands had subsided (limited by the areal peatland extent). These positive feedbacks also stimulated Hollandse IJssel and Lek avulsion crossing lower delta peatlands. Protecting back barriers in the western parts of the Netherlands or by tidal levees in the western and northern Netherlands could somewhat hamper these developments. This explains why large sea ingressions did not develop in all back-barrier areas with wide peatlands, such as the SW coastal plain (Pierik et al., Reference Pierik, Cohen, Vos, van der Spek and Stouthamer2017a). Apart from the peaty downstream area, the landscape in the Rhine–Meuse delta was relatively resilient because of the presence of protective natural levees (Fig. 4). Although flood frequencies and sediment supply changed over the first millennium AD (Fig. 5), with potential consequences for inhabitants, the morphology of the landscape did not change considerably (Figs 4 and 5). These examples show that landscape resilience strongly depends on the geomorphological and geological setting as the initial landscape state.
4.1.2 Role of changing anthropogenic conditions in landscape resilience
Humans changed conditions in the peaty coastal plain and lower Rhine–Meuse delta to the degree that it became less resilient to perturbations (e.g. storms and floods). Peatland reclamation and water-level lowering caused subsidence of these areas. This process was locally initiated in the Late Iron Age (250–12 BC), but the consequences started only after AD 250 as soon as the first storm surge reached this lowered peatland, eventually leading to the large-scale development of new tidal systems (Pierik et al., Reference Pierik, Cohen, Vos, van der Spek and Stouthamer2017a) and river courses (Pierik et al., Reference Pierik, Stouthamer, Schuring and Cohen2018a).
In the delta, avulsion took place once in every few centuries during the Holocene (Stouthamer & Berendsen, Reference Stouthamer and Berendsen2007). Human-induced sediment load increase over the preceding centuries, however, resulted in more frequent avulsion during the first millennium AD. This changing condition was not induced in the area itself, but far more upstream. Here, deforestation in the catchments caused increased sediment supply towards the delta from c.500 BC (Erkens et al., Reference Erkens, Hoffmann, Gerlach and Klostermann2011). This caused increased overbank deposition that probably accelerated the natural avulsion process by creating a larger elevation difference between parent channel and floodbasin. Downstream, it additionally facilitated the sediment-loading feedback in the peat area in addition to subsidence by drainage following reclamation. In this way, human-enhanced sediment supply meant that the new rivers gained a gradient advantage over the old courses, increasing avulsion potential and success (Pierik et al., Reference Pierik, Stouthamer, Schuring and Cohen2018a). In contrast to the coastal and fluvial areas, drift-sand intensity in the sand area was comparable to to that in the preceding centuries; it occurred rather locally and probably reacted shortly after the overexploitation that caused it. In the coastal dune and inland drift-sand areas, deforestation and intensive land use enhanced susceptibility to aeolian erosion by storms (Pierik et al., Reference Pierik, Van Lanen, Gouw-Bouman, Groenewoudt, Wallinga and Hoek2018b). Under Holocene climatic conditions and natural vegetation development, a bare substrate required for sand drifting could hardly be formed. This makes it most likely that the local drift-sand occurrences during the first millennium AD were human-induced. In all these examples, humans slowly made the landscape more sensitive (i.e. less resilient) to geomorphological change resulting from stochastic extreme events, although its scale and impact varied.
4.1.3 Role of large events: changing natural conditions or triggers?
Extreme events may affect the state of a landscape directly (cf. Scheffer et al., Reference Scheffer, Carpenter, Foley, Folke and Walker2001). This means that more or more frequent intense storms and floods (e.g. related to climate change) may have affected geomorphological development as well, without human-induced changes in landscape resilience being the main cause. The formation of large-scale coastal dune complexes, for example, has been linked to intense storm periods (e.g. Younger Dunes in the Holland coast; Jelgersma et al., Reference Jelgersma, De Jong, Zagwijn and Van Regteren Altena1970) and formation of marine incursions (US east coast – Mallinson et al., Reference Mallinson, Culver, Leorri, Mitra, Mulligan and Riggs2018). Especially after the first millennium AD, during the Little Ice Age (LIA; AD 1570–1850), storms may have affected dune formation in coastal and inland areas (Pierik et al., Reference Pierik, Van Lanen, Gouw-Bouman, Groenewoudt, Wallinga and Hoek2018b; Jackson et al., Reference Jackson, Costas and Guisado-Pintado2019). For the first millennium AD, considering the scale of vulnerable peat occurrence, human impact seems more important. To further explore the role of storm frequency in landscape change, sedimentological records of individual floods and storm surges in the lower delta and coastal plain are needed, which are currently sparse (Jelgersma et al., Reference Jelgersma, Stive and Van der Valk1995; Cunningham et al., Reference Cunningham, Bakker, Van Heteren, Van der Valk, Van der Spek, Schaart and Wallinga2011). For tidal systems, reconstructing high water levels is especially complicated, since they strongly depend on tidal and storm dynamics. Additionally, tidal resonance effects determined by past estuary and tidal-basin shape also affect flood levels. To incorporate the shape of these tidal systems, the palaeogeographical situation has to be known in great detail.
Also in the fluvial area, floods may have caused the tipping point to occur earlier, while the main cause for the Lek and Hollandse IJssel to cross vast peatlands was anthropogenic. Relatively large Holocene floods had been identified in the upper and central delta (Toonen et al., Reference Toonen, Donders, Van der Meulen, Cohen and Prins2013, Reference Toonen, Foulds, Macklin and Lewin2017), and seem to correlate with the initial phase of the Gelderse IJssel and Lek, and with the maturation of the Waal (Fig. 5; Cohen et al., Reference Cohen, Toonen and Weerts2016; Jansma, Reference Jansma2020). Similar to the effects of storms in the coastal and sand areas, individual floods most likely served as triggers for avulsion in the fluvial area. They formed breaches in natural levees and enhanced channel maturation by enlarging the channel, thereby stimulating avulsion success (Makaske et al., Reference Makaske, Maathuis, Padovani, Stolker, Mosselman and Jongman2012). In summary, the tipping points in landscape evolution mainly occurred after a trigger, e.g. a flood or storm, but these were generally not the primary causes of the observed landscape changes. In most cases during the first millennium AD, reduced resilience caused the landscape to become more susceptible to stochastic events as triggers.
4.2 New landscape states?
We see landscape changes in all three regions, but to a varying degree. Did these mainly human-induced changes cause the landscape to shift into an alternative state with its own processes and feedbacks? The answer to that question strongly depends on the spatial scale that one considers. Here I consider the landscape changes on the scale of the three study areas.
By spatial extent, sea ingression in the coastal plain was the largest geomorphological change that occurred in the Netherlands in the first millennium AD. After and during their formation, a new set of feedbacks set in, making the situation irreversible (Table 1). Not only did this transform the area into a totally different landscape with tidal channels, levees and tidal flats. Eventually, sediment import compensated for ingression several centuries later, filling up the coastal area again with sands and clays. Replacement of compaction-prone peat by these sediments, often deposited up to the high-water line (saltmarshes, levees), made the parts of the coastal landscape where these ingressions took place more resilient to potential new ingressions. Meanwhile, the fringing peatlands further inland remained vulnerable and were drowned in the last millennium (e.g. Dollard (Vos & Knol, Reference Vos and Knol2015) and the Biesbosch (Gottschalk, Reference Gottschalk1975; Kleinhans et al., Reference Kleinhans, Weerts and Cohen2010)). Some more local effects of sea ingression are creek network changes by short-cutting of drainage routes from the Pleistocene upland or hinterland rivers towards the sea. Expanding tidal channels could connect to streams and brooks already present in these areas. Examples of new tidal channels that functioned as new outlets for fluvial discharge are the Lauwers ingression in the northern coastal area (from c.AD 500 onwards) and Honte-Westerschelde in the SW Netherlands (from c.AD 1500 onwards) (Fig. 3).
Unlike sea ingressions in the coastal plain, avulsions did not transform the delta into a totally different landscape (Fig. 4). The avulsion process was likely accelerated, e.g. due to extra sediment load, but it was not enough to shift the entire delta into a new regime as avulsion-related feedbacks remained comparable to earlier periods. Still, avulsions reorganised the delta network considerably and irreversibly (Table 1). The avulsions, for example, led to annexation of new areas (e.g. the Lek/Hollandse IJssel and Gelderse IJssel; Berendsen & Stouthamer, Reference Berendsen and Stouthamer2000; Cohen et al., Reference Cohen, Stouthamer, Hoek, Berendsen and Kempen2009, Reference Cohen, Pierik, Toonen, van der Meulen, Bomers, Gouw-Bouman, Van Popta, Jansma, Erkens, Busschers, Schuring, Volleberg, Stouthamer and Hoek2021), thereby affecting the distribution of sediment through the delta. As a result of newly formed natural levees around the new river branches, the total area of natural levees increased by c.5% during the first millennium AD (Pierik et al., Reference Pierik2017b). These young levees, especially, built up relatively high, whereas the topographical expression of more distal-inherited natural levees gradually reduced, spatially shifting flooding dynamics and opportunities for habitation.
In the Pleistocene sand area, geomorphological changes were small before human influence started. The sandy substrate, however, became susceptible to aeolian erosion when deforestation and overexploitation took place (Pierik et al., Reference Pierik, Van Lanen, Gouw-Bouman, Groenewoudt, Wallinga and Hoek2018b). In the first millennium AD, drift-sand activity mainly occurred locally and briefly (Fig. 6). As in the fluvial and coastal areas, positive feedbacks enhanced sand drifting in the Pleistocene sand area. Once a large storm had caused parts of the sands to be laid bare, these parts were more susceptible to the next storm, a process that finally resulted in the formation of large, active dune fields. This feedback was especially effective when the storm frequencies increased and vegetation was less well recovered. The drift-sand activity became more prominent after AD 1500 when the spatial extent of drift-sands increased, reaching a climax in the 19th century (Koster, Reference Koster2009). Compared to the surface affected by sea ingressions and new river branches, however, these dune fields were still relatively small (i.e. not larger than several square kilometres). Although the drift-sand activity in the first millennium AD itself did not cause a regime shift from a geomorphological point of view, it is an expression of a soil deterioration trend over the course of the last millennia. This trend is associated with increasing exploitation, changing soil structure and ecology of the Pleistocene uplands, with major implications for suitability of the landscape for habitation and agriculture (Spek, Reference Spek2004).
In summary, sea ingressions in the coastal plain had the largest effect on the landscape during the study period, bringing the landscape to an alternative state with new landscape elements, feedbacks and human–landscape interactions. Avulsion had a significant effect on delta evolution, without shifting the state of the delta landscape, and sand drifting still occurred on a relatively small scale in the first millennium AD. From these examples it becomes clear that the degree of change largely depends on geomorphological feedbacks associated with the type of substrate and surrounding geomorphology.
4.3 Impact on humans
When the impact of landscape changes on humans is expressed as settlement shifts, it becomes clear that the impact of landscape change on its inhabitants generally was proportional to the area over which the change took place. Some changes had a negative effect on habitation or on the accessibility of the landscape, whereas others had a positive effect. These landscape changes may have accelerated already ongoing cultural shifts changes (related to political and economic factors) in the current Netherlands that are associated with the collapse of the Roman Empire.
The formation of new tidal inlets and systems in a former freshwater peat swamp transformed the coastal landscape into a brackish tidal area, which had major implications for the ecosystems and people in this area. In the SW coastal plain, people lived on such drowning peatlands. Not only the former peat area was abandoned, but also the flanking dune areas which probably became more isolated. Because the initial landscape in the northern coastal plain contained large supratidal flats and levees with dwelling mounds, the direct impact of sea ingressions was much smaller here. However, the drowning of the flanking peatlands may have hampered landscape accessibility, obstructing the connection between the northern coastal plain and the northern sand area. The landscape had probably become wetter already before large-scale sea ingressions occurred, because of naturally accreting saltmarsh ridges. This likely resulted in settlement abandonment during the 4th century (Nieuwhof et al., 2019).
The southern sand area and in the river area are considered to have been almost completely abandoned in the 3rd and 4th centuries (Heeren, Reference Heeren2015). Depopulation was most likely strongly influenced by political developments, but in the delta it is very likely that the impact of floods also changed the habitation conditions considerably. A period of intense floods occurred between AD 250 and 850, which is very likely to have influenced habitable areas and caused at least local settlement shifts to somewhat higher parts of alluvial ridges in most parts of the delta. Jansma (Reference Jansma2020) demonstrated that intense floods on the scale of the entire Netherlands probably started somewhat earlier, in the second half of the middle Roman period (Fig. 5D). Evidence of settlement abandonment in larger areas over multiple centuries is restricted to fossil alluvial ridges near large rivers. Best example is the Bommelerwaard area, where the influence of the Rhine became stronger after the formation of the Waal branch (Pierik & Van Lanen, Reference Pierik and Van Lanen2019). Most alluvial ridges, however, remained in use as route-network corridors throughout the first millennium AD (Van Lanen & Pierik, Reference Van Lanen and Pierik2019), indicating a degree of resilience to floods and changes in the landscape.
The impact of drift-sand activity on communities in the Pleistocene sand area seems to have been relatively small before the Late Middle Ages (AD 1050–1500). Only around Kootwijk in the central Netherlands indications have been found that habitation was interrupted by drift-sand activity by the end of the Early Middle Ages (Heidinga, Reference Heidinga1984). Certainly from c.AD 1500 onwards, drift-sand activity became a supra-local phenomenon, since extensive drift-sand dune fields developed making larger areas unsuitable for land use and transport. The way in which these landscape changes were managed by humans differs from that in other areas. In the sand areas, relatively small adaptations in land use could already prevent sand drifting (i.e. maintaining small hedges (e.g. De Keyzer & Bateman Reference De Keyzer and Bateman2018)). Such mitigation was for example more difficult in the coastal plain peat areas, where subsidence was irreversible and occurred on too large a scale to permit effective measures.
A positive economic effect of the formation of new tidal and fluvial channels was the formation of potential trade routes over water (Jansma et al., Reference Jansma, Van Lanen and Pierik2017). Examples of new route opportunities were new creeks between the SW part of the coastal plain and the Meuse estuary. Also, the Lek and Hollandse IJssel provided new route connections that later (after the 7th century) provided the early-medieval city of Dorestad in the central Netherlands with suitable (long-distance) transport options fundamental to its development (Kosian et al., Reference Kosian, Van Lanen and Weerts2016). The expanding Vlie inlet and Almere lagoon and the formation of the Gelderse IJssel (in the 7th century) connected the upstream part of the delta to the northwestern and northern coastal plain. Along the new Gelderse IJssel after c.AD 800 the cities Zutphen and Deventer emerged (Groothedde, Reference Groothedde2013; Fig. 2B).
In short, changing landscapes, especially in the coastal and delta area, generated pressure on top of NW European cultural and political changes. Major sea ingressions in the coastal plain caused large-scale abandonment of settlements. In the fluvial area, settlements modestly shifted after changing flooding frequencies and avulsions, the latter creating opportunities for new water routes. In the sand area, no large-scale trends in settlement shifts can be linked to drift-sand activity for the first millennium AD.
5. Conclusions
In the Netherlands, the first millennium AD was a period of large-scale changes in the physical landscape. The causes behind these were mainly anthropogenic, while the triggers were natural. This generated additional pressure on inhabitants during the Dark Ages, on top of cultural political changes in NW Europe.
-
Most prominent change took place in the coastal area where large-scale sea ingressions formed. These were provoked by peatland subsidence caused by prehistoric reclamation and anthropogenic groundwater level lowering. Because of this, a new landscape formed with new geomorphological feedbacks and human–landscape interactions, making this a true regime shift in the landscape.
-
Significant landscape changes also occurred in the Rhine–Meuse delta. Human-induced increased suspended sediment supply to the delta helped the initiation of the Gelderse IJssel, Lek and Hollandse IJssel branches. The latter two were also aided by downstream human-induced peat subsidence and wetland deforestation. Although the rearrangement of the delta network was irreversible, these avulsions marked geographical reorganisations of the same landscape elements in the delta, and therefore cannot be seen as full regime shifts on delta scale. In the Pleistocene sand area, drift-sand activity locally occurred close to settlements and route networks, indicating that they were induced by human land-use practices. Although they are part of a larger trend of soil deterioration, sand drifting was still local in the first millennium AD and does not mark a regime shift in this period.
-
The preconditions of the landscape (i.e. the substrate composition and geomorphology) are the most prominent factor to determine the degree of geomorphological changes. These (i) determined landscape resilience to human influence and (ii) controlled the strength of feedbacks that may push the landscape into an alternative state. For example, vast peatlands were sensitive to large-scale flooding and ingression after – often locally initiated – anthropogenic subsidence. This non-linear response occurred because of irreversible peat compaction and non-linear tidal inlet growth once the sea gained access to these former peatlands.
-
The impact of landscape changes on humans depended on the scale, irreversibility and recovery time of the geomorphological change. Sea ingressions caused large-scale settlement abandonment. Modest settlement shifts are observed in the delta in reaction to wetter conditions, and avulsion changed sediment depocentre locations and delta-network properties for trade routes. In the sand area, no major settlement shifts can be linked to the drift sands in the studied period.
-
Further integration of geoscientific, archaeological, ecological and historical data and approaches on landscape changes is the way forward to further understand human–landscape interactions in past landscapes. More accurate dates of shifting geomorphological changes and their possible causes and numerical models could help testing hypotheses. This integrative and interdisciplinary approach can be upscaled for any period in data-rich areas to generate more cases for validation and to see larger patterns of human–landscape interaction.
Acknowledgements
This paper is part of the special issue Dark Ages of the Lowlands, that synthesises and embeds the results of the NWO project ‘The Dark Ages in an interdisciplinary light’ (project nr. 360-60-110). It is based on chapter 10 of the PhD thesis of H.J. Pierik (Utrecht University - Pierik, Reference Pierik2017a), funded by the same project. I would like to thank members of the Dark Ages project for their collaboration, and also Friederieke Bungenstock and Jakob Wallinga for their valuable comments on the manuscript.
Data availability
DANS archive dataset natural levees in the Rhine–Meuse delta: https://doi.org/10.17026/dans-zg9-nqfx
DANS archive dataset drift sands: https://doi.org/10.17026/dans-z2x-phq5
PhD thesis HJ Pierik: https://dspace.library.uu.nl/handle/1874/354561