Introduction
Crinoids are a diverse, long-lived clade of echinoderms with a fossil record spanning nearly half a billion years and are represented by more than 600 species living in marine ecosystems today (Hess et al., Reference Hess, Ausich, Brett and Simms1999). The geologic history of crinoids is revealed through a highly complete, well-sampled fossil record (Foote and Raup, Reference Foote and Raup1996; Foote and Sepkoski, Reference Foote and Sepkoski1999) displaying a complex pageant of evolutionary radiation, extinction, ecologic innovation, and morphologic diversification (Ausich and Bottjer, Reference Ausich and Bottjer1982; Ausich et al., Reference Ausich, Kammer and Baumiller1994; Foote, Reference Foote1999; Peters and Ausich, Reference Peters and Ausich2008; Deline and Ausich, Reference Deline and Ausich2011; Gorzelak et al., Reference Gorzelak, Salamon, Trzęsiok, Lach and Baumiller2015). The spectacular fossil record of crinoids is greatly enriched and complemented by detailed biologic studies on living species. These studies facilitate opportunities to synthesize information from fossil and extant forms. For example, comparative studies between fossil and living crinoid species have provided insight into species ecology and niche dynamics (Meyer and Macurda, Reference Meyer and Macurda1977; Ausich, Reference Ausich1980; Roux, Reference Roux1987; Kitazawa et al., Reference Kitazawa, Oji and Sunamura2007; Baumiller, Reference Baumiller2008), established developmental bases for morphologic homologies (Shibata et al., Reference Wright2015a), and informed phylogenetic hypotheses (Simms and Sevastopulo, Reference Simms and Sevastopulo1993; Rouse et al., Reference Rouse, Jermiin, Wilson, Eeckhaut, Lanterbecq, Oji, Young, Browning, Cisternas, Helgen, Stuckey and Messing2013). Thus, crinoids form a data-rich model system for exploring major questions in the history of life.
Given their general significance and broad scientific utility across multiple disciplines of inquiry, it is paramount that the biological classification of crinoids reflects their evolutionary heritage. Numerous emendations and informal suggestions for major taxonomic revisions have been opined over the past few decades (e.g., Kelly, Reference Kelly1986; Simms and Sevastopulo, Reference Simms and Sevastopulo1993; Ausich, Reference Ausich1998a, Reference Ausich1998b; Webster and Jell, Reference Webster and Jell1999; Hess and Messing, Reference Hess and Messing2011), but the most recent comprehensive revision to crinoid classification is the 1978 Treatise on Invertebrate Paleontology (Moore and Teichert, Reference Moore and Teichert1978). Since publication of the Treatise, the value of revising rank-based systematic classifications to be consistent with phylogenetic hypotheses and/or the explicit use of phylogenetic taxonomy (sensu de Quieroz and Gauthier, Reference de Queiroz and Gauthier1990; Sereno, Reference Sereno1999, Reference Sereno2005) has become increasingly common in paleontology (e.g., Smith, Reference Smith1984, Reference Smith1994; Holtz, Reference Holtz1996, Reference Holtz1998; Sereno, Reference Sereno1997; Padian et al., Reference Padian, Hutchinson and Holtz1999; Brochu and Sumrall, Reference Brochu and Sumrall2001; Carlson, Reference Carlson2001; Carlson and Leighton, Reference Carlson and Leighton2001; Brochu, Reference Brochu2003; Forey et al., Reference Forey, Fortey, Kenrick and Smith2004; Sereno et al., Reference Sereno, McAllister and Brusatte2005; Butler et al., Reference Butler, Upchurch and Norman2008; Kelley et al., Reference Kelley, Fastovsky, Wilson, Laws and Raymond2013). We agree with these authors that all named taxa in a biological classification system should ideally represent clades (i.e., monophyletic groups). The development of phylogeny-based classifications is not without difficulties or criticism (e.g., Benton, Reference Benton2000, Reference Benton2007). However, we advocate that recent advances in understanding the phylogenetic relationships of major crinoid lineages make the biological classification of the Crinoidea ripe for revision.
A great strength of so-called ‘phylogenetic taxonomy’ is its potential for increasing nomenclatural stability (de Quieroz and Gauthier, Reference de Queiroz and Gauthier1994; Brochu and Sumrall, Reference Brochu and Sumrall2001). Under a phylogeny-based system of classification, groups of taxa are organized by their patterns of shared common ancestry rather than diagnostic traits. This is a particularly useful aspect of phylogenetic taxonomy: if named evolutionary units are defined by their history of common ancestry, they do not change if new information comes to light that necessitates modification of taxonomic diagnoses. For example, new fossil discoveries and/or more nuanced understandings of phylogenetic relationships may alter the distribution of synapomorphies among members of a clade but do not alter the definition of the clade. Moreover, by naming taxa on the basis of cladogram topologies, phylogenetic taxonomy can provide a precise definition for groups previously difficult to diagnose by a unique combination of synapomorphies, such as the Articulata (Simms, Reference Simms1988; Webster and Jell, Reference Webster and Jell1999; Rouse et al., Reference Rouse, Jermiin, Wilson, Eeckhaut, Lanterbecq, Oji, Young, Browning, Cisternas, Helgen, Stuckey and Messing2013). To avoid potential instability in taxonomic nomenclature and/or the proliferation of clade names, we advocate that major changes in crinoid systematics should: (1) be based on well-supported phylogenetic hypotheses inferred using rigorous and repeatable quantitative techniques, and (2) employ widely used names and/or names with historical precedence if available.
In this paper, we propose a series of stem-based and node-based clade definitions to help standardize nomenclature for crinoid higher taxa. The clade definitions proposed herein are informed by a series of recent phylogenetic analyses (Ausich et al., Reference Ausich, Kammer, Rhenberg and Wright2015; ColeReference Cole , 2017; WrightReference Wright , Reference Wright2017) and represent the first attempt to classify crinoids using the principles of phylogenetic taxonomy (de Queiroz and Gauthier, Reference de Queiroz and Gauthier1992, Reference de Queiroz and Gauthier1994).
Although Linnaean classifications lack rigorous criteria for assigning ranks, they can nevertheless provide useful (if coarse) reflections of phylogenetic relatedness and divergence among taxa, particularly in paleontology (Smith, Reference Smith1984; Potter and Freudenstein, Reference Potter and Freudenstein2005; Jablonski and Finarelli, Reference Jablonski and Finarelli2009; Soul and Friedman, Reference Soul and Friedman2015). Given the widespread use of rank-based classifications among invertebrate paleontologists in both alpha taxonomy and paleobiological studies, it is prudent to present a phylogenetically informed revision of the rank-based classification of the Crinoidea. These revisions modify the existing Linnaean classification of crinoids to better represent the set of nested hierarchies implied by phylogenetic trees (Ausich et al., Reference Ausich, Kammer, Rhenberg and Wright2015; ColeReference Cole , 2017; WrightReference Wright , Reference Wright2017).
In their review of progress made in crinoid research during the twentieth century, Ausich and Kammer (Reference Ausich and Kammer2001, p. 1167) stated the “immediate challenge for the [twenty-first century] study of crinoids is to establish a phylogenetic classification for the entire class.” It is our hope that the dual classification systems presented herein will provide a foundation for future studies employing phylogenetic nomenclature in crinoid research and promote the use of an improved classification system among researchers who choose to work with the Linnaean system.
The dredge and the hammer: a brief history of crinoid classification
The whole history of the attempts to classify the Crinoidea shows … the gradual emancipation from the older habit of lumping forms together because they are alike in structure without considering how the likeness arose.
–F.A. Bather (Reference Bather1898, p. 339)
Formal scientific description and classification of crinoids began in 1821 when J.S. Miller recognized fossilized stalked echinoderms from the “environs of Bristol” as a distinct group. Although he did not include comatulids in his original conception of the Crinoidea, he anticipated that they were crinoids: “The combination of these results with those from the Crinoidea made me anxious to examine the Comatulae … an animal which would be defined with sufficient precision as a Pentacrinus destitute of the column” (Miller, Reference Miller1821, p. 127). Further, he judged Marsupites ornatus Miller, Reference Miller1821 (an unstalked crinoid of Cretaceous age) to be the ‘link’ between comatulids and his Crinoidea (Miller, Reference Miller1821, p. 139). Extant stalked crinoids were unknown until the mid- to late 1860s, when their discovery during oceanic dredging expeditions provided fodder for early debates regarding the efficacy of Darwin’s (Reference Darwin1859) then recently proposed theory of natural selection (see Alaniz, Reference Alaniz2014; Etter and Hess, Reference Etter and Hess2015). Thus, the original description, definition, and diagnosis of the Crinoidea relied entirely on fossil remains. Despite the morphological diversity and deep phylogenetic divergences among groups of extant species, the inclusion of living crinoids with fossil forms has not fundamentally altered Miller’s (Reference Miller1821) concept. Following subsequent inclusion of the comatulids and extant stalked crinoids with fossil forms, the Crinoidea has withstood nearly 200 years of scrutiny as a distinct group within the Echinodermata.
In contrast with their long-term recognition as a clade, the classification of taxa within the Crinoidea has been widely debated since the nineteenth century (Müller, Reference Müller1841; Angelin, Reference Angelin1878; Wachsmuth and Springer, Reference Wachsmuth and Springer1897; Bather, Reference Bather1899; Springer, Reference Springer1913; Jaekel, Reference Jaekel1918). With few exceptions, debates on crinoid classification have primarily been based on disagreements over phylogenetic affinities among taxa rather than systematic practices among researchers (see Bather, Reference Bather1899 for a counter example). The intensity of early debates over crinoid classification is best epitomized by the frequent yet acrimonious exchanges between Wachsmuth and Springer (e.g., 1885, Reference Wachsmuth and Springer1891, Reference Wachsmuth and Springer1897) and Bather (e.g., Reference Bather1898, Reference Bather1899, Reference Bather1900). Attempts to resolve these debates among nineteenth-century systematists have largely shaped the last ~70 years of crinoid research (Ausich and Kammer, Reference Ausich and Kammer2001).
In their seminal work Evolution and Classification of Paleozoic Crinoids, Moore and Laudon (Reference Moore and Laudon1943) presented a classification that incorporated aspects of both Frank Springer’s and Francis Bather’s ideas (see discussion in Ausich and Kammer, Reference Ausich and Kammer2001). With few modifications, Moore and Laudon’s (Reference Moore and Laudon1943) publication formed the basis of the Treatise on Invertebrate Paleontology (Moore and Teichert, Reference Moore and Teichert1978). Following publication of the 1978 Treatise, the classification of crinoids entered a protracted yet frail era of nomenclatural stability. Although few authors have advanced major revisions or comprehensive modifications, many have voiced contention with the Treatise classification (Kelly, Reference Kelly1982, Reference Kelly1986; Kolata, Reference Kolata1982; McIntosh, Reference McIntosh1984, Reference McIntosh1986, Reference McIntosh2001; Ausich, Reference Ausich1986, Reference Ausich1998a, Reference Ausich1998b; Donovan, Reference Donovan1988; Simms, Reference Simms1988; Simms and Sevastopulo, Reference Simms and Sevastopulo1993; Brower, Reference Brower1995; Webster and Jell, Reference Webster and Jell1999; Guensburg and Sprinkle, Reference Guensburg and Sprinkle2003; Hess and Messing, Reference Hess and Messing2011; Guensburg, Reference Guensburg2012; Ausich et al., Reference Ausich, Kammer, Rhenberg and Wright2015). With the exception of Simms and Sevastopulo (Reference Simms and Sevastopulo1993), these studies have been readjustments of the Moore and Teichert (Reference Moore and Teichert1978) classification to accommodate rank changes, the addition of new groups, and delineation of clade membership defined by phylogenetic studies of extant species.
The study of extant crinoids remains in the shadow of A. H. Clark, who published more than 100 publications on their morphology, taxonomy, and classification during the early to middle twentieth century (e.g., Clark, Reference Clark1915, Reference Clark1921; Clark and Clark, Reference Clark and Clark1967). The advent and application of molecular phylogenetic methods to crinoid phylogeny has recently thrown light on relationships among extant species (Cohen et al., Reference Cohen, Ameziane, Eleaume and de Forges2004; Hemery et al., Reference Hemery, Roux, Ameziane and Eleaume2013; Rouse et al., Reference Rouse, Jermiin, Wilson, Eeckhaut, Lanterbecq, Oji, Young, Browning, Cisternas, Helgen, Stuckey and Messing2013; Summers et al., Reference Summers, Messing and Rouse2014). However, these analyses also point toward the need for extensive taxonomic revisions and an improved understanding of morphologic traits among living species (Messing and White, Reference Messing and White2001; David et al., Reference David, Roux, Messing and Ameziane2006; Roux et al., Reference Roux, Eleaume, Hemery and Ameziane2013; Summers et al., Reference Summers, Messing and Rouse2014; Hays et al., Reference Hays, Rouse, Thomas and Messing2015). Remarkably, there has been little previous work to combine molecular phylogenetic studies of extant crinoids with paleontologic data to assemble a more complete picture of post-Paleozoic crinoid evolutionary history. Efforts to integrate these rich sources of information present both challenges and opportunities for future researchers to resolve patterns and processes shaping the crinoid tree of life (Lee and Palci, Reference Lee and Palci2015; Pyron, Reference Pyron2015).
Crinoid origins and classification
Extant echinoderms include the Crinoidea, Echinoidea, Ophiuroidea, Asteroidea, and the Holothuroidea, with the latter four comprising the Eleutherozoa. Although it has been long established that crinoids form the sister group to the Eleutherozoa, the relationships among many fossil and extant echinoderm groups are controversial (Paul and Smith, Reference Paul and Smith1984; Sumrall Reference Sumrall1997; David et al., Reference David, Lefebvre, Mooi and Parsley2000; Smith, Reference Smith2005; Pisani et al., Reference Pisani, Feuda, Peterson and Smith2012; Telford et al., Reference Telford, Low, Cameron, Ortega-Martinez, Aronowicz, Oliveri and Copley2014; Zamora and Rahman, Reference Zamora and Rahman2014; Feuda and Smith, Reference Feuda and Smith2015; Reich et al., Reference Reich, Dunn, Akasaka and Wessel2015). The phylogenetic position of crinoids within the Echinodermata was contested throughout the late twentieth century, with a focal question whether the Pelmatozoa (i.e., stalked echinoderms including blastozoans and crinoids) and/or the Blastozoa are monophyletic groups or a ‘grade’ of body plan organization. This is a fundamental question not only for understanding the origin of crinoids but also for resolving phylogenetic relationships among clades within the Echinodermata. One hypothesis of crinoid origins postulates that crinoids and blastozoan echinoderms independently evolved pelmatozoan-grade body plans (e.g., Sprinkle, Reference Sprinkle1973, Reference Sprinkle1976; Mooi and David, Reference Mooi and David1998, Reference Mooi and David2008; David et al., Reference David, Lefebvre, Mooi and Parsley2000; Guensburg and Sprinkle, Reference Guensburg and Sprinkle2003; Guensburg, Reference Guensburg2012). This hypothesis proposes that blastozoans and crinoids each comprise distinct monophyletic groups. By contrast, an alternative hypothesis postulates that blastozoans and crinoids are members of an inclusive pelmatozoan clade, with crinoids nested within a paraphyletic Blastozoa (Leuckart, Reference Leuckart1848; Bather, Reference Bather1899, Reference Bather1900; Paul and Smith, Reference Paul and Smith1984; Smith, Reference Smith1984; Paul, Reference Paul1988; Smith and Jell, Reference Smith and Jell1990; Smith, Reference Smith1994; Sumrall, Reference Sumrall1997; Ausich, Reference Ausich1998a, Reference Ausich1998b; Clausen et al., Reference Clausen, Jell, Legrain and Smith2009; Zamora and Smith, Reference Zamora and Smith2011; Kammer et al., Reference Kammer, Sumrall, Zamora, Ausich and Deline2013; O’Malley et al., Reference O’Malley, Ausich and Chin2016). In this hypothesis, the blastozoan body plan represents a grade of organization within the more inclusive Pelmatozoa, a clade comprising all blastozoan-grade echinoderms and crinoids (including the crown group). Although the inclusive group of nominal ‘blastozoan’ taxa is not monophyletic, there are undoubtedly assemblages of blastozoan taxa that do correspond to monophyletic groups (Smith, Reference Smith1984; Sumrall and Wray, Reference Sumrall and Wray2007; Zamora and Smith, Reference Zamora and Smith2011; Sumrall and Waters, Reference Sumrall and Waters2012; Zamora et al., Reference Zamora, Sumrall, Zhu and Lefebvre2016).
Important to this debate are the differences among researchers with respect to their underlying taxonomic principles and systematic practices (see Smith, Reference Smith1988). Those who support the monophyly of the Blastozoa and Crinoidea embrace systematic practices that emphasize differences (rather than similarities) among taxa, recognize plesiomorphic traits as taxonomically informative characters, exclude character data from consideration of relationships because of a priori beliefs regarding the distribution of homoplastic traits, and conflate sister group hypotheses with ancestor–descendant relationships (e.g., Guensburg and Sprinkle, Reference Guensburg and Sprinkle2003, Reference Guensburg and Sprinkle2007; Guensburg, Reference Guensburg2012; Guensburg et al., Reference Guensburg, Blake, Sprinkle and Mooi2016). These practices differ considerably from those that infer the Pelmatozoa as a clade. These workers tend to emphasize similarities (rather than differences) among taxa, minimize a priori assumptions regarding hypotheses of character evolution, and utilize the principles of phylogenetic systematics to rigorously test whether apparent similarities in form reflect synapomorphies or homoplasy (e.g., Sumrall and Waters, Reference Sumrall and Waters2012; Sumrall, Reference Sumrall2014; Ausich et al., Reference Ausich, Kammer, Rhenberg and Wright2015). Given the recent advances in homology assessment among pentaradiate echinoderms (e.g., Sumrall, Reference Sumrall1997, Reference Sumrall2008, Reference Sumrall2010, Reference Sumrall2014; Sumrall and Waters, Reference Sumrall and Waters2012; Kammer et al., Reference Kammer, Sumrall, Zamora, Ausich and Deline2013) and computational phylogenetic analyses of echinoderm taxa based on a large ensemble of characters, it is becoming increasingly clear that a blastozoan-grade taxon likely forms the closest immediate outgroup to the Crinoidea (Kammer et al., Reference Kammer, Sumrall, Zamora, Ausich and Deline2013; Sumrall, Reference Sumrall2014). In the future, new developments in phylogenetic research along with a continued search for the oldest ‘crinoid’ fossils will continue to play a role in uncovering the sequence of morphologic transitions behind the assembly of the crinoid body plan.
Despite desultory disagreements regarding crinoid origins (Sprinkle, Reference Sprinkle1973; Ubaghs, Reference Ubaghs1978; Donovan, Reference Donovan1988; Ausich, Reference Ausich1998a, Reference Ausich1998b; Ausich and Babcock, Reference Ausich and Babcock1998; Guensburg and Sprinkle, Reference Guensburg and Sprinkle2007, Reference Guensburg and Sprinkle2009; Guensburg, Reference Guensburg2012; Kammer et al., Reference Kammer, Sumrall, Zamora, Ausich and Deline2013; Ausich et al., Reference Ausich, Kammer, Rhenberg and Wright2015; Guensburg et al., Reference Guensburg, Blake, Sprinkle and Mooi2016), there is nevertheless considerable agreement among workers regarding the pattern of branching relationships within the crinoid ingroup. For example, the recent phylogenetic analyses of Guensburg (Reference Guensburg2012) and Ausich et al. (Reference Ausich, Kammer, Rhenberg and Wright2015) reveal highly congruent patterns of branching relationships among crinoid higher taxa despite the use of alternative outgroups, different data sets, and alternative interpretations of homologous morphologic characters. We surmise this growing consensus stems from the improved taxonomic sampling of the oldest known crinoids (Guensburg and Sprinkle, Reference Guensburg and Sprinkle2003, Reference Guensburg and Sprinkle2009; Guensburg, Reference Guensburg2010) and implementation of more rigorous quantitative approaches to testing phylogenetic hypotheses (Guensburg, Reference Guensburg2012; Ausich et al., Reference Ausich, Kammer, Rhenberg and Wright2015; ColeReference Cole , 2017; WrightReference Wright , Reference Wright2017).
We conclude that congruence observed among tree topologies obtained from researchers with different perspectives indicates strong support for these patterns. Although questions surrounding crinoid origins remain, this debate is moot with respect to the phylogeny-based definitions and classification presented herein and ultimately has no bearing on the focus and conclusions of this paper.
Toward a phylogenetic classification of the Crinoidea
From the perspective of their geologic history, crinoids are a bottom-heavy clade (Gould et al., Reference Gould, Gilinsky and German1987). In contrast to the tremendously diverse assemblage of stem lineages, comparatively few species are encompassed within the crown group (Fig. 1). Because of the enormous diversity of the stem group relative to the crown group, fossil crinoids have received much systematic attention compared to their extant representatives (but see Clark, Reference Clark1915; David et al., Reference David, Roux, Messing and Ameziane2006; Hess and Messing, Reference Hess and Messing2011; Hemery et al., Reference Hemery, Roux, Ameziane and Eleaume2013; Rouse et al., Reference Rouse, Jermiin, Wilson, Eeckhaut, Lanterbecq, Oji, Young, Browning, Cisternas, Helgen, Stuckey and Messing2013). Aside from a number of smaller studies examining relationships among species of middle to late Paleozoic genera (e.g., Gahn and Kammer, Reference Gahn and Kammer2002; Kammer and Gahn, Reference Kammer and Gahn2003; Ausich and Kammer, Reference Ausich and Kammer2008), most investigations of crinoid phylogeny have focused on discerning relationships among Ordovician taxa (Brower, Reference Brower1995; Ausich Reference Ausich1998b; Guensburg, Reference Guensburg2012; Ausich et al., Reference Ausich, Kammer, Rhenberg and Wright2015; ColeReference Cole , Reference Cole2017). The Ordovician Period represents a key interval in crinoid evolution because species belonging to various groups of traditionally named taxa first appear in rocks of the Lower Ordovician (Tremadocian) (Guensburg and Sprinkle, Reference Guensburg and Sprinkle2003, Reference Guensburg and Sprinkle2009; Guensburg, Reference Guensburg2010) and the majority of well-studied groups had originated prior to its close.

Figure 1 Taxa representing major crinoid clades: (1) Pentacrinites fossilis Blumenbach, Reference Blumenbach1804, articulate, from Goldfuss (1831); (2) Taxocrinus colletti White, Reference White1881, flexible, from Springer (Reference Springer1920); (3) Actinocrinites jugosus (Hall, Reference Hall1859), monobathrid camerate, from Wachsmuth and Springer (Reference Wachsmuth and Springer1897); (4) Synbathocrinus swallovi Hall, Reference Hall1858, disparid, from Wachsmuth and Springer (Reference Wachsmuth and Springer1897); (5) Dendrocrinus caduceus Hall, Reference Hall1866, eucladid, from Meek (Reference Meek1873); (6) Hybocystites eldonensis Parks, Reference Parks1908, hybocrinid, from Springer (Reference Springer1911); (7) Porocrinus shawi Schuchert, Reference Schuchert1900, porocrinid, from Kesling and Paul (Reference Kesling and Paul1968); (8) Archaeocrinus microbasalis (Billings, Reference Billings1857), diplobathrid camerate, from Wachsmuth and Springer (Reference Wachsmuth and Springer1897). Scale bars=0.5 cm and applicable as indicated.
The divergence between camerate and non-camerate lineages forms a fundamental, early split in the history of crinoid evolution (Jaekel, Reference Jaekel1918; Donovan, Reference Donovan1988; Guensburg, Reference Guensburg2012; Ausich et al., Reference Ausich, Kammer, Rhenberg and Wright2015; ColeReference Cole , Reference Cole2017; WrightReference Wright , Reference Wright2017) (Fig. 1). For example, in the recent phylogeny of Ausich et al. (Reference Ausich, Kammer, Rhenberg and Wright2015), taxa belonging to the Camerata (sensu Moore and Teichert, Reference Moore and Teichert1978) form the sister clade to all other crinoids, including the protocrinoids (Guensburg and Sprinkle, Reference Guensburg and Sprinkle2003). Disparids were recovered as sister to a clade comprised of most ‘cladid’ taxa, and hybocrinids were recovered as sister to a group of ‘cyathocrine’ cladids (sensu Moore and Teichert, Reference Moore and Teichert1978). A similar pattern was recovered by Guensburg (Reference Guensburg2012, fig. 2).
Building on these studies, Reference ColeCole (Reference Cole2017) further assessed the basal split between camerates and non-camerates and tested the taxonomic status of the Monobathrida and Diplobathrida (Fig. 1). Reference WrightWright’s (Reference Wright2017) analysis of relationships among non-camerate crinoids offers a more nuanced perspective of this portion of the crinoid tree than previously recovered. Notably, many so-called Ordovician clades of Guensburg (Reference Guensburg2012) and Ausich et al. (Reference Ausich, Kammer, Rhenberg and Wright2015) do not retain their status of monophyly when post-Ordovician taxa are considered (WrightReference Wright , Reference Wright2017).
Recent molecular phylogenetic studies indicate broad relationships among major clades of extant crinoids are also reaching a consensus, with the Isocrinida representing the sister clade to all other extant crinoids (Rouse et al., Reference Rouse, Jermiin, Wilson, Eeckhaut, Lanterbecq, Oji, Young, Browning, Cisternas, Helgen, Stuckey and Messing2013, Reference Rouse, Carvajal, Oji and Messing2015). It is interesting to note that divergence time estimation based on relaxed molecular clock models suggests the split between isocrinids and other extant groups took place some 231–252 million years ago (Rouse et al., Reference Rouse, Jermiin, Wilson, Eeckhaut, Lanterbecq, Oji, Young, Browning, Cisternas, Helgen, Stuckey and Messing2013). Thus, molecular phylogenetic analyses and paleontological evidence are in general agreement regarding an ancient origin of the crinoid crown group.
A summary tree based on results presented by Rouse et al. (Reference Rouse, Jermiin, Wilson, Eeckhaut, Lanterbecq, Oji, Young, Browning, Cisternas, Helgen, Stuckey and Messing2013), Ausich et al. (Reference Ausich, Kammer, Rhenberg and Wright2015), Reference WrightWright (Reference Wright2017), and Reference ColeCole (2017) is depicted in the form of a simplified cladogram in Figure 2. This cladogram is annotated with the clade names we propose below. Terminal taxa in the cladogram were carefully chosen to maximize stability in phylogenetic nomenclature (Table 1). Sereno (Reference Sereno2005) listed numerous criteria for choosing taxon specifiers in clade definitions. These recommendations include choosing specifiers that are nested rather than basal (if possible), represented by well-known or readily available material, and using multiple specifiers where necessary to accommodate phylogenetic uncertainty and/or alternative hypotheses. We have carefully chosen our clade definitions to not hinge on labile phylogenetic hypotheses or specific interpretations of unusual and/or problematic taxa.
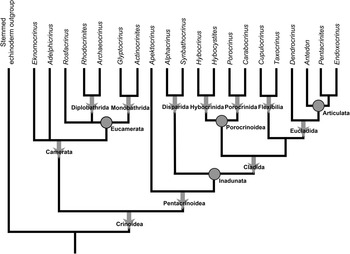
Figure 2 Cladogram depicting phylogenetic relationships among species used to define major clades within the Crinoidea. Terminal tips correspond to species listed in Table 1. Clades given stem-based definitions are indicated with a downward-facing arrow; clades given node-based definitions are indicated with a circle. Note that many clades named are nested inside other more inclusive clades. Graphical notation of stem- and node-defined clades follows Sereno (Reference Sereno2005).
Table 1 Species name, least inclusive clade, and first appearance interval for each taxon depicted in Figure 2.

Classes of clade definitions used in phylogenetic taxonomy and their graphical representations used herein closely follow Sereno (Reference Sereno1999, Reference Sereno2005). Node-based clade definitions circumscribe the most recent common ancestor of at least two taxa and all of its descendants. Thus, node-based definitions form the least inclusive clade containing a minimum of two specifiers. By contrast, stem-based definitions circumscribe the most inclusive clade containing at least one internal specifier. In both cases, additional precision is obtained by identifying external specifiers falling outside the clade (i.e., the outgroup). For example, a stem-based definition for hypothetical Clade A with two internal and one external taxon specifiers can be stated as ‘all species sharing a more recent common ancestor with species X and Y than Z,’ where X and Y are internal taxon specifiers and Z is an external specifier. In other words, Clade A is stem-defined as the most inclusive clade containing X and Y but not Z. Note the presence of one species as an external specifier effectively eliminates the entire clade to which it belongs. By definition, a clade cannot contain an ancestor of its sister group.
In phylogenetic taxonomy, clade membership is not determined by the presence or absence of a ‘key’ morphologic feature unless that apomorphy (or set of apomorphies) is listed in the definition as a qualifying clause (Sereno, Reference Sereno2005). We avoid apomorphic qualifiers in our definitions for several reasons. First, incomplete preservation may lead to cases where it is unknown whether a fossil species has the key feature diagnostic of the clade in question. Thus, the inclusion or exclusion of a fossil species depends on character state optimizations rather than direct data. Second, a trait may be ‘absent’ in a taxon either because it was truly absent or because it was secondarily lost. Similarly, a trait may be ‘present’ because of convergent evolution. Moreover, stem group taxa commonly have highly heterogeneous distributions of apomorphic traits, which may lead to instability when new taxa are sampled and/or alternative topologies are equally likely. Finally, the timing of a divergence event may not correspond with the acquisition of a diagnostic apomorphy. For example, the blastozoan Macrocystella is widely recognized as a basal glyptocystitoid rhombiferan even though it lacks the respiratory structures traditionally ‘diagnostic’ of the Glyptocystida (Paul, Reference Paul1968; Sprinkle, Reference Sprinkle1973; Zamora et al., Reference Zamora, Sumrall, Zhu and Lefebvre2016). All of these considerations are highly important when considering patterns of character evolution but may lead to nomenclatural instability if incorporated into clade definitions.
Although we avoid the use of apomorphies to define clades, we do discuss morphological traits potentially useful for taxonomic diagnoses. In some cases, our proposed clade definitions retain much of their traditional meaning and taxonomic content, with constituent taxa sharing numerous synapomorphies that form unambiguous taxonomic boundaries (e.g., the Flexibilia). However, in other cases, either substantial revision was necessary and/or a list of unambiguous diagnostic characters was difficult or impossible to obtain (e.g., the Articulata). These challenges highlight the utility of phylogenetic taxonomy. For example, many authors have remarked that the Articulata has lacked a concise, unambiguous definition since it was first erected by Miller nearly 200 years ago (Simms, Reference Simms1988; Webster and Jell, Reference Webster and Jell1999; Hess and Messing, Reference Hess and Messing2011; Rouse et al., Reference Rouse, Jermiin, Wilson, Eeckhaut, Lanterbecq, Oji, Young, Browning, Cisternas, Helgen, Stuckey and Messing2013). A phylogenetic definition of the Articulata provides a clearer criterion for clade membership and results in a framework for future phylogenetic research assessing relationships among hypothesized stem clades, crown group synapomorphies, and subsequent morphologic transitions among crown group subclades.
The clade definitions and revised classification proposed herein represent the present state of knowledge, but systematics is a dynamic science and taxonomic theories are commonly reinterpreted in light of new discoveries. We fully expect our definitions to be refined and/or modified as more information becomes available. Some places of the crinoid tree still require extensive taxonomic revisions, such as upper Paleozoic ‘cladids’ (sensu Moore and Laudon, Reference Moore and Laudon1943) and stem articulates (WrightReference Wright , Reference Wright2015b). Despite these potential vicissitudes in the taxonomic content and/or definitions within our proposed classification, we agree with G.G. Simpson’s sentiment: “It is pusillanimous to avoid making our best efforts today because they may appear inadequate tomorrow” (Reference Simpson1944, p. xxx [sic]).
Systematic paleontology
Crinoidea Miller, Reference Miller1821
Definition
The Crinoidea is stem-defined as the most inclusive clade containing Rhodocrinites verus Miller, Reference Miller1821, Actinocrinites triacontadactylus Miller, Reference Miller1821, and Pentacrinites fossilis Blumenbach, Reference Blumenbach1804 but not Rhopalocystis detombesi Ubaghs, Reference Ubaghs1963, Echinosphaerites aurantium (Gyllenhaal, Reference Gyllenhaal1772), Eumorphocystis multiporate Branson and Peck, Reference Branson and Peck1940, Protocrinites ouiformis Eichwald, Reference Eichwald1840, Cheirocystis antiqua Paul, Reference Paul1972, Glyptocystella loeblichi (Bassler, Reference Bassler1943), Cambraster cannati Miquel, Reference Miquel1894, and Cambroblastus enubilatus Smith and Jell, Reference Smith and Jell1990.
Remarks
This definition captures J. S. Miller’s (Reference Miller1821) original concept based on fossil specimens and retains the name ‘Crinoidea’ as the clade comprising the crown group plus all extinct species sharing a more recent common ancestor with a living crinoid than any echinoderm taxon listed in the preceding as external specifiers (Fig. 2). Further, this definition closely resembles the traditional use and taxonomic content of the Crinoidea as used by both biologists and paleontologists (Bather, Reference Bather1899; Clark, Reference Clark1915; Jaekel, Reference Jaekel1918; Moore and Teichert, Reference Moore and Teichert1978; Hess et al., Reference Hess, Ausich, Brett and Simms1999; Rouse et al., Reference Rouse, Jermiin, Wilson, Eeckhaut, Lanterbecq, Oji, Young, Browning, Cisternas, Helgen, Stuckey and Messing2013) and accommodates the current state of uncertainty regarding their nearest extinct sister group. In the interest of preserving the taxonomic content and common meaning of a widely used name, our Clade Crinoidea is preferred over Sumrall’s (Reference Sumrall1997) similarly defined Crinoidoformes (see Cantino and de Queiroz, Reference Cantino and de Queiroz2010, p. 42). The Crinoidea is comprised of two major clades, the Camerata and the Pentacrinoidea, reflecting the early divergence between camerate and non-camerate crinoids (Jaekel, Reference Jaekel1918; Donovan, Reference Donovan1988; Guensburg, Reference Guensburg2012; Ausich et al., Reference Ausich, Kammer, Rhenberg and Wright2015). Because we provide the Crinoidea with a stem-based definition, the discovery of stemward fossils is accommodated within this definition.
Internal taxon specifiers were chosen because they were included in Miller’s (Reference Miller1821) original description and represent well-known, well-preserved, and highly nested members of their respective subclades. In contrast to the internal taxon specifiers, the choice of external specifiers is more complex. The use of external specifiers in this definition spanning various ‘blastozoan’ and edrioasteroid-grade groups reflects the current difficulty involved in postulating the nearest definitive sister group as well as the uncertain state of relationships among extinct stemmed echinoderms (Smith, Reference Smith1984; Sumrall, Reference Sumrall1997, Reference Sumrall2014; Ausich, Reference Ausich1998a, Reference Ausich1998b; Guensburg and Sprinkle, Reference Guensburg and Sprinkle2009; Kammer et al., Reference Kammer, Sumrall, Zamora, Ausich and Deline2013; Ausich et al., Reference Ausich, Kammer, Rhenberg and Wright2015; Guensburg et al., Reference Guensburg, Blake, Sprinkle and Mooi2016; O’Malley et al., Reference O’Malley, Ausich and Chin2016).
The analysis of Ordovician crinoids by Ausich et al. (Reference Ausich, Kammer, Rhenberg and Wright2015) took a conservative approach to outgroup selection by sampling broadly across taxa nested within the Clade Pelmatozoa (Kammer et al., Reference Kammer, Sumrall, Zamora, Ausich and Deline2013; Sumrall, Reference Sumrall2014). Similarly, we have chosen species from multiple pelmatozoan groups as external specifiers to help provide nomenclatural stability in the presence of phylogenetic uncertainty. Other taxa hypothesized to represent the crinoid sister group include the stylophorans (David et al., Reference David, Lefebvre, Mooi and Parsley2000) and edrioasteroids (Guensburg and Sprinkle, Reference Guensburg and Sprinkle2009; Guensburg et al., Reference Guensburg, Blake, Sprinkle and Mooi2016). Stylophorans have long been considered non-radiate stem group echinoderms (e.g., Paul and Smith, Reference Paul and Smith1984; Smith, Reference Smith1984, Reference Smith2008) and have been cogently demonstrated to lack crown group synapomorphies (Smith, Reference Smith2005). Thus, we do not consider the stylophoran hypothesis further. Guensburg and Sprinkle (Reference Guensburg and Sprinkle2009) and Guensburg et al. (Reference Guensburg, Blake, Sprinkle and Mooi2016) regard edrioasteroid echinoderms, such as the stromatocystidid Cambraster or the edrioblastoid Cambroblastus, to possess apomorphies indicating they share a more recent common ancestor with crinoids than with other echinoderms. Although this hypothesis contrasts with previous studies regarding edrioasteroids as stem group eleutherozoans (Paul and Smith, Reference Paul and Smith1984; Smith Reference Smith1984, Reference Smith1985, Reference Smith1990; Smith and Zamora, Reference Smith and Zamora2013), recent investigations suggest that edrioasteroids may comprise a para- or polyphyletic group (Kammer et al., Reference Kammer, Sumrall, Zamora, Ausich and Deline2013; Zamora, Reference Zamora2013; Zamora and Rahman, Reference Zamora and Rahman2014). Some edrioasteroids, such as the isorophids, may be closely related to gogiid eocrinoids, whereas other edrioasteroids, such as Cambraster, may be closer to glyptocystitoid blastozoans and crinoids (Kammer et al., Reference Kammer, Sumrall, Zamora, Ausich and Deline2013; Zamora et al., Reference Zamora, Sumrall and Vizcaino2013; Zamora and Rahman, Reference Zamora and Rahman2014). Because a comprehensive, up-to-date phylogeny of pentaradiate echinoderm lineages is currently lacking, we tentatively follow Guensburg and Sprinkle (Reference Guensburg and Sprinkle2009) and Guensburg et al. (Reference Guensburg, Blake, Sprinkle and Mooi2016) by including both Cambraster and the edrioblastoid Cambroblastus as additional external taxon specifiers.
Identifying synapomorphies of the Clade Crinoidea requires a phylogenetic hypothesis of their position within the broader echinoderm clade. As discussed above, this remains an open question. Basal members of both the Camerata and Pentacrinoidea have a dicyclic calyx with an irregular field of plates intercalating between fixed proximal brachials, suggesting these may be plesiomorphic traits (cf. Apektocrinus, Cnemecrinus, Glenocrinus) (Guensburg, Reference Guensburg2012, Ausich et al., Reference Ausich, Kammer, Rhenberg and Wright2015; ColeReference Cole , Reference Cole2017; WrightReference Wright , Reference Wright2017), but a definitive list of shared derived traits cannot be provided here. Moreover, it is challenging to propose a list of apomorphies that unambiguously differentiate crinoids from other echinoderm taxa because many traits are not exclusive to crinoids. Crinoids have been traditionally recognized as distinct from blastozoan-grade echinoderms in having true ‘arms,’ where arms are defined as coelomic extensions of the body cavity (Sprinkle, Reference Sprinkle1973). However, morphologic observations of solute and diploporitan echinoderms such as Eumorphocystis and the discovery of various Cambrian ‘blastozoans’ with arm-like appendages strongly suggest that arms may not be an apomorphy unique to crinoids (Clausen et al., Reference Clausen, Jell, Legrain and Smith2009; Zamora and Smith, Reference Zamora and Smith2011; Sumrall, Reference Sumrall2014; Zamora and Rahman, Reference Zamora and Rahman2014).
We anticipate future phylogenetic research will help resolve these broader issues in echinoderm phylogeny and evolution. Improved knowledge of relationships among extinct pentaradiate echinoderms may also help refine our definition of the Clade Crinoidea by removing pleonastic external specifiers. We await its refinement.
Camerata Wachsmuth and Springer, 1885
Definition
The Camerata is stem-defined as the most inclusive clade containing Actinocrinites triacontadactylus Miller, Reference Miller1821 and Rhodocrinites verus Miller, Reference Miller1821 but not Pentacrinites fossilis Blumenbach, 1804.
Remarks
Camerate crinoids represent a diverse, morphologically distinct ‘stem clade’ (sensu Sereno, Reference Sereno1999, Reference Sereno2005) ranging from the Lower Ordovician to Permian and contain all taxa traditionally placed within the Diplobathrida and Monobathrida (Moore and Teichert, Reference Moore and Teichert1978; ColeReference Cole , Reference Cole2017). Camerates are most easily differentiated from pentacrinoids in having calyx plates united by rigid sutures, a heavily plated tegmen surface covering the mouth, and a medial plate (or series of plates) in the posterior (i.e., CD) interray. Unlike pentacrinoids, the camerate posterior plate series has no proximal topographic affinity with the C ray, although some camerate posterior plates may be homologous with those of pentacrinoids (see Jaekel, Reference Jaekel1918, p. 46; Moore and Laudon, Reference Moore and Laudon1943; Brower, Reference Brower1973, p. 301–304; Guensburg and Sprinkle, Reference Guensburg and Sprinkle2003). In addition, typical camerate species have fixed proximal brachials, interradials, and sometimes intrabrachials, whereas most derived pentacrinoid clades lack these features.
Multiple studies indicate strong support for camerate monophyly (Ausich, Reference Ausich1998b; Ausich et al., Reference Ausich, Kammer, Rhenberg and Wright2015; ColeReference Cole , Reference Cole2017). However, Reference ColeCole’s(Reference Cole2017) analysis of Ordovician camerates did not find support for a strict division between monocyclic and dicyclic forms. Reference ColeCole’s(Reference Cole2017) phylogenetic revision proposed narrower restrictions on clade membership to render these taxa monophyletic. Following revision, the Monobathrida and Diplobathrida are sister clades that together comprise the more inclusive Eucamerata (ColeReference Cole , Reference Cole2017). Thus, the stem-based definition of the Camerata contains the Clade Eucamerata and their stem taxa, including representatives of the oldest known crinoid fossils (e.g., Eknomocrinus, Cnemecrinus), and genera placed within the problematic Reteocrinitidae (see ColeReference Cole , Reference Cole2017), and may or may not contain the protocrinoids (see Guensburg and Sprinkle, Reference Guensburg and Sprinkle2003; Guensburg, Reference Guensburg2012; Ausich et al., Reference Ausich, Kammer, Rhenberg and Wright2015; ColeReference Cole , Reference Cole2017).
Eucamerata ColeReference Cole , Reference Cole2017
Definition
The Eucamerata is node-defined as the least inclusive clade containing Actinocrinites triacontadactylus Miller, Reference Miller1821, Rhodocrinites verus Miller, Reference Miller1821, and Rosfacrinus robustus Le Menn and Spjeldnaes, Reference Le Menn and Spjeldnaes1996.
Remarks
Reference ColeCole(Reference Cole2017) revised the Monobathrida and Diplobathrida to represent monophyletic groups while attempting to preserve the greatest number of taxa traditionally included within each (Moore and Teichert, Reference Moore and Teichert1978). The name ‘Eucamerata’ was proposed to identify the clade of camerates comprised of the sister groups Monobathrida and Diplobathrida, which necessarily excludes stem taxa such as Cnemecrinus and Reteocrinus (ColeReference Cole , Reference Cole2017). The Eucamerata comprise the majority of camerate taxa and span the Ordovician through Permian. Eucamerates are characterized generally by the traits listed above for the Camerata, but differ in typically having more strongly ankylosed calyx plate sutures, primaxils on the second primibrachial, holomeric stems, and pinnulate arms (cf. Actinocrinites and Rhodocrinites with Eknomocrinus and Reteocrinus).
In an attempt to preserve the stability of sister group relationships between monobathrid and diplobathrid clades, we provide a node-based definition for the Eucamerata and stem-based definitions for the Monobathrida and Diplobathrida. The internal taxon specifiers Actinocrinites and Rhodocrinites are highly nested constituents of their respective monobathrid and diplobathrid subclades (Moore and Laudon, Reference Moore and Laudon1943; ColeReference Cole , Reference Cole2017). Rosfacrinus is cautiously included as an additional external specifier because it occupies a somewhat uncertain position at the base of the eucamerate tree (see discussion in ColeReference Cole , Reference Cole2017).
Monobathrida Moore and Laudon, Reference Moore and Laudon1943
Definition
The Monobathrida is stem-defined as the most inclusive clade containing Glyptocrinus decadactylus Hall, Reference Hall1847 and Actinocrinites triacontadactylus Miller, Reference Miller1821 but not Rhodocrinites verus Miller, Reference Miller1821 and Archaeocrinus lacunosus (Billings, Reference Billings1857).
Remarks
When revising Bather’s (Reference Bather1899) polyphyletic division of crinoids into the Monocyclica and Dicyclica, Moore and Laudon (Reference Moore and Laudon1943) placed all camerates with monocyclic calyces into the Monobathrida. Reference ColeCole’s(Reference Cole2017) phylogenetic analysis of Ordovician camerate crinoids indicates a strict adherence to Moore and Laudon’s (Reference Moore and Laudon1943) concept of the Monobathrida is not monophyletic. However, removal of the stemward camerates Eknomocrinus and Adelphicrinus renders the Monobathrida a clade (ColeReference Cole , Reference Cole2017). The internal and external specifiers defining this stem-based clade ensure the taxonomic content closely matches Moore and Laudon (Reference Moore and Laudon1943).
Monobathrids are a taxonomically diverse group of camerates ranging from the Ordovician to Permian and are traditionally diagnosed as monocyclic camerates. Although other clades had similar trends in circlet reduction (e.g., the Disparida), the transformation from a dicyclic to monocyclic calyx likely represents a veritable synapomorphy of monobathrid camerates, as the dicyclic crinoid Gaurocrinus was recovered as the sister taxon to the Monobathrida by Reference ColeCole(Reference Cole2017). Additional features diagnostic of a typical monobathrid species include having radial plates larger than other calyx plates, an upright basal circlet, an uninterrupted radial circlet (except in the posterior interray), and a posterior interray with anitaxis plating and an anitaxial ridge.
Diplobathrida Moore and Laudon, Reference Moore and Laudon1943
Definition
The Diplobathrida is stem-defined as the most inclusive clade containing Archaeocrinus lacunosus (Billings, Reference Billings1857) and Rhodocrinites verus Miller, Reference Miller1821 but not Actinocrinites triacontadactylus Miller, Reference Miller1821 and Glyptocrinus decadactylus Hall, Reference Hall1847.
Remarks
Similar to the discussion above, Moore and Laudon (Reference Moore and Laudon1943) placed all of Bather’s (Reference Bather1899) dicyclic camerate crinoids within the Diplobathrida. As with the monobathrids, Reference ColeCole’s(Reference Cole2017) phylogenetic analysis of Ordovician camerates revealed Moore and Laudon’s (Reference Moore and Laudon1943) Diplobathrida required revision. To achieve monophyly of diplobathrids while retaining much of Moore and Laudon’s (Reference Moore and Laudon1943) taxonomic content, all dicyclic taxa equally related to both monobathrid and diplobathrid camerates sensu Reference ColeCole(Reference Cole2017) are removed from the Diplobathrida (e.g., Eknomocrinus, Reteocrinids, etc.). Following Reference ColeCole’s(Reference Cole2017) suggested revision, our stem-based definition stabilizes the long-held hypothesis that monobathrids and diplobathrids represent sister clades (Moore and Laudon, Reference Moore and Laudon1943; ColeReference Cole , Reference Cole2017).
Diplobathrids range from the Ordovician through lower Carboniferous (Serpukhovian). Reference ColeCole’s(Reference Cole2017) discussion on the taxonomic distribution of diplobathrid morphologies suggests they are generally characterized by a combination of character states, including a dicyclic calyx, a concave calyx base either concealing or partially concealing the infrabasal plates, and the presence of additional plates interrupting the radial circlet in all interrays (e.g., Rhodocrinites). Some diplobathrids sensu Reference ColeCole(Reference Cole2017), such as the Dimerocrinitidae, are similar to monobathrids in having their radial circlet interrupted only in the posterior interray but can easily be distinguished by their dicyclic calyx. A closer examination of post-Ordovician species indicates a substantial revision of subclades within the Diplobathrida is needed and additional research is currently underway (ColeReference Cole , Reference Cole2015)
Pentacrinoidea Jaekel, Reference Jaekel1918
Definition
The Pentacrinoidea is stem-defined and as the most inclusive clade containing Apektocrinus ubaghsi Guensburg and Sprinkle, Reference Guensburg and Sprinkle2009 and Pentacrinites fossilis Blumenbach, Reference Blumenbach1804 but not Rhodocrinites verus Miller, Reference Miller1821 and Actinocrinites triacontadactylus Miller, Reference Miller1821.
Remarks
The name ‘Pentacrinoidea’ originates from Jaekel’s (Reference Jaekel1894, Reference Jaekel1918) prescient observation that camerate and non-camerate crinoids form distinct clades. Although authors after Jaekel (Reference Jaekel1918) did not adopt this name in subsequent classifications (see Lane, Reference Lane1978; Ausich and Kammer, Reference Ausich and Kammer2001), Jaekel’s usage coincides with this strongly supported clade (Guensburg, Reference Guensburg2012; Ausich et al., Reference Ausich, Kammer, Rhenberg and Wright2015; ColeReference Cole , Reference Cole2017; WrightReference Wright , Reference Wright2017). Thus, we propose to reinstate the name Pentacrinoidea with the preceding definition.
We have chosen two phylogenetically distant non-camerate species as internal specifiers. Pentacrinites fossilis is a well-known fossil species from rocks of Jurassic age and is closely related to extant isocrinid crinoids (David et al., Reference David, Roux, Messing and Ameziane2006), placing it within the Crown Crinoidea (see Articulata below). The species Apektocrinus ubaghsi is a Lower Ordovician fossil and ranks among the stratigraphically oldest known crinoids (Guensburg and Sprinkle, Reference Guensburg and Sprinkle2009). However, all phylogenetic research indicates it is closer to non-camerates than to camerates and diverges stemward of other basal ‘cladid’ (sensu Moore and Teichert, Reference Moore and Teichert1978) taxa such as Aethocrinus (Guensburg and Sprinkle, Reference Guensburg and Sprinkle2009; Guensburg, Reference Guensburg2012; Ausich et al., Reference Ausich, Kammer, Rhenberg and Wright2015; WrightReference Wright , Reference Wright2017). Our stem-based definition recognizes Jaekel’s (Reference Jaekel1918) priority of this concept and effectively places all known non-camerate species within the Pentacrinoidea.
Pentacrinoids are a spectacularly diverse and morphologically heterogeneous clade ranging from the Early Ordovician to present-day marine communities. The primary apomorphies differentiating pentacrinoids from camerates relate to their distinctive posterior plating patterns, the degree of calyx plate suturing, and oral region rigidity (‘tegmen’ terminology here is from Ausich and Kammer, Reference Ausich and Kammer2016). Posterior plates among pentacrinoids display a proximal relationship with the C-ray radial plate (Guensburg, Reference Guensburg2010; WrightReference Wright , Reference Wright2015a). Subclades within the Pentacrinoidea express this affinity differently (cf. Cladida and Disparida), and extant crinoids do not retain posterior plates as adults. However, the ontogenetic trajectory of posterior plate development in extant crinoids is tightly linked with morphologic patterns among their Paleozoic precursors (WrightReference Wright , Reference Wright2015a). Pentacrinoid calyx plates are less closely sutured (i.e., ankylosed) than camerates and typically have a non-rigid to flexible oral region. In many pentacrinoids, the mouth is directly exposed on the oral surface rather than beneath a tegmen (Ausich and Kammer, Reference Ausich and Kammer2016).
There are several other morphologic features less diagnostic than those described above but still useful for distinguishing most pentacrinoid species from camerates. For example, some basal pentacrinoids such as Apektocrinus, Aethocrinus, and Alphacrinus incorporate additional plates within the calyx (similar to camerates). However, the overwhelming majority of pentacrinoid clades do not. A major exception occurs among flexible crinoids, but flexibles are a derived group of pentacrinoids and can be differentiated from camerates by other apomorphies (see Flexibilia below). Similarly, eucamerate crinoids have pinnules, but most early to middle Paleozoic pentacrinoids do not. Pinnulation evolved at least once (and probably several times) during the middle to late Paleozoic among the subclade Cladida (WrightReference Wright , Reference Wright2015b), but these taxa can readily be distinguished from eucamerates in having a pentacrinoid-like posterior plating pattern and free arms above the radials.
Inadunata Wachsmuth and Springer, 1885
Definition
The Inadunata is node-defined as the least inclusive clade containing Synbathocrinus conicus Phillips, Reference Phillips1836 and Dendrocrinus longidactylus Hall, Reference Hall1852.
Remarks
Wachsmuth and Springer (1885) placed non-articulate fossil crinoids with free arms above the radial plates within the Inadunata. Subsequent classifications divided the Inadunata into the Cladida and Disparida according to the number of circlets in the calyx (Moore and Laudon, Reference Moore and Laudon1943; Moore and Teichert, Reference Moore and Teichert1978). In a pioneering study on phylogenetic approaches to crinoid classification, Simms and Sevastopulo (Reference Simms and Sevastopulo1993) pointed out the Inadunata of Moore and Teichert (Reference Moore and Teichert1978) was paraphyletic and recommended the name be abandoned. In addition, Simms and Sevastopulo’s (Reference Simms and Sevastopulo1993) revision resolved the paraphyly of cladid inadunates by including the Flexibilia and Articulata within the Cladida.
The division between the Camerata and Pentacrinoidea (discussed above) indicates disparids and cladids are more closely related to one another than to camerates (Fig. 2). Indeed, recent phylogenetic analyses of Ordovician crinoids recover a sister group relationship between disparids and cladids (sensu Moore and Laudon, Reference Moore and Laudon1943), with hybocrinids nested within the Cladida (Fig. 2) (Guensburg, Reference Guensburg2012; Ausich et al., Reference Ausich, Kammer, Rhenberg and Wright2015; WrightReference Wright , Reference Wright2017). Our definition of the Inadunata combines Wachsmuth and Springer’s (1885) original concept with Simms and Sevastopulo’s (Reference Simms and Sevastopulo1993) revision of the Cladida to include flexibles and articulates. Note that this definition places stemward pentacrinoids, such as Apektocrinus, outside the Inadunata. We combine a node-based definition of the Inadunata with stem-based definitions for the subclades Disparida and Cladida to form a node-stem triplet to increase the stability of sister relationships between these taxa (Sereno, Reference Sereno1999).
The Clade Inadunata ranges from the Early Ordovician to the present and are as a whole well characterized by Wachsmuth and Springer’s (1885) general concept of crinoids with free arms above the radial plates. Exceptions to this diagnosis occur but are mostly restricted to a few stemward taxa and the Flexibilia, which represent a derived group of inadunates (Springer, Reference Springer1920).
Disparida Moore and Laudon, Reference Moore and Laudon1943
Definition
The Disparida is stem-defined as the most inclusive clade containing Synbathocrinus conicus Phillips, Reference Phillips1836 but not Dendrocrinus longidactylus Hall, Reference Hall1852.
Remarks
Disparids comprise a diminutive but morphologically and taxonomically diverse clade of fossil crinoids ranging from the Ordovician through Permian. Moore and Laudon (Reference Moore and Laudon1943) erected the Disparida to include all monocyclic inadunates. Disparid monophyly is well supported by phylogenetic analyses of Ordovician crinoids (Guensburg, Reference Guensburg2012; Ausich et al., Reference Ausich, Kammer, Rhenberg and Wright2015; WrightReference Wright , Reference Wright2017) and contains all species closer to Synbathocrinus than the cladid Dendrocrinus. Given the similar topologies across these studies, the Clade Disparida retains taxa traditionally placed within disparids (sensu Moore and Laudon, Reference Moore and Laudon1943) except for the hybocrinids.
A major synapomorphy and useful diagnostic trait of disparid crinoids is the presence of a single circlet of plates below the radials. All other pentacrinoids are either dicyclic (cladids), pseudomonocyclic (hybocrinids) (see Sprinkle, Reference Sprinkle1982b), or otherwise phylogenetically distant from disparids (some derived articulates may not develop infrabasals, see Lahaye and Jangoux, Reference Lahaye and Jangoux1987). Disparids also have simple or compound radial plates, typically lack pinnules, and have approximate bilateral symmetry between rays oriented in one of several possible planes (see Moore et al., Reference Moore, Lane, Strimple and Sprinkle1978b). As pentacrinoids, disparids have posterior plates in a proximal position to the C ray but differ from cladids in having plates positioned above rather than below or in line with the C-ray radial plate. However, posterior plate homologies among disparids and between inadunate clades are presently obscured by a set of descriptive terms opaque to homology. Whether the proximal C-ray posterior plate is an anibrachial,’ a ‘radianal,’ an ‘anal X,’ a ‘superradial,’ or a ‘radial’ is uncertain (Moore, Reference Moore1962; Moore and Teichert, Reference Moore and Teichert1978; Ausich, Reference Ausich1996). Future work is needed to help clarify primary posterior plate homologies among disparids and between cladids and disparids. The results of Reference WrightWright’s(Reference Wright2017) analysis of Ordovician through Devonian pentacrinoid taxa support Guensburg’s (Reference Guensburg2010) assessment of Alphacrinus as a lower Tremadocian crinoid phylogenetically close to the base of the disparid clade. Guensburg (Reference Guensburg2010) considered the posterior of Alphacrinus to express a transitional form between ‘typical’ pentacrinoid posterior plates and the ray-like extensions common among disparid taxa. A re-examination of the posterior interray of basal taxa combined with studies on disparid ontogeny may help resolve this issue.
Cladida Moore and Laudon, Reference Moore and Laudon1943
Definition
The Cladida is stem-defined as the most inclusive clade containing Dendrocrinus longidactylus Hall, Reference Hall1852 but not Synbathocrinus conicus Phillips, Reference Phillips1836.
Remarks
The Cladida were originally defined by Moore and Laudon (Reference Moore and Laudon1943) to comprise a tremendously diverse and long-ranging (Ordovician–Triassic) assemblage of dicyclic inadunates with their mouths covered with primary peristomial cover plates (Ausich and Kammer, Reference Ausich and Kammer2016). Moore and Laudon’s (Reference Moore and Laudon1943) original concept and taxonomic content of the Cladida is paraphyletic, as they agreed with Springer’s (Reference Springer1920) earlier assessment that flexible crinoids were more closely related to some cladids than others but did not place the Flexibilia within the Cladida. Moreover, post-Paleozoic crinoids within Miller’s (Reference Miller1821) Articulata have long been considered descendants of Paleozoic cladids (Jaekel, Reference Jaekel1918; Moore et al., Reference Moore, Lalicker and Fischer1952; Rassmussen, Reference Rasmussen1978; Simms, Reference Simms1988). Simms and Sevastopulo (Reference Simms and Sevastopulo1993) conducted a cladistic analysis of Paleozoic cladids, flexibles, and articulate crinoids and subsequently remedied cladid paraphyly by placing the Flexibilia and the Articulata within the Cladida (sensu Moore and Laudon, Reference Moore and Laudon1943). Although many authors have followed Simms and Sevastopulo’s (Reference Simms and Sevastopulo1993) interpretation of relationships among these taxa, only a few authors have since followed their revised rank-based classification (e.g., Brower, Reference Brower2001, Reference Brower2002; Donovan and Harper, Reference Donovan and Harper2003).
Our stem-based definition of the Cladida is similar in taxonomic content to Simms and Sevastopulo’s (Reference Simms and Sevastopulo1993) because it includes all species closer to Dendrocrinus than to the disparid Synbathocrinus. Thus, the Cladida spans the Ordovician to the Recent and contains the major subclades Porocrinoidea, Flexibilia, and Articulata. Cladids are most easily distinguished from their sister group, the Disparida, in typically having a dicyclic calyx and posterior plates (as adults or during development) located below and/or in line with the radial plate circlet (WrightReference Wright , Reference Wright2015a). Lastly, many middle Paleozoic to Recent cladids have pinnules, whereas most disparids do not (Frest et al., Reference Frest, Strimple and McGinnis1979).
Porocrinoidea WrightReference Wright , Reference Wright2017
Definition
The Porocrinoidea is node-defined as the least inclusive clade containing Carabocrinus radiatus Billings, Reference Billings1857 and Hybocrinus conicus Billings, Reference Billings1857.
Remarks
In their description of crinoids belonging to Bather’s (Reference Bather1899) ‘Cyathocrinina’, Moore and Laudon (Reference Moore and Laudon1943) speculated that ‘primitive’ cyathocrinids such as Carabocrinus might be closely related to the enigmatic taxon Hybocrinus. Sprinkle (Reference Sprinkle1982b) argued the stem and calyx morphology of Hybocrinus suggested hybocrinids were ‘pseudomonocyclic’ and listed a number of characters linking hybocrinids with cladids. Although hybocrinids have not traditionally been classified within the Cladida, many phylogenetic analyses of Ordovician crinoids have recovered a clade of ‘cyathocrine’ grade cladids and hybocrinids (Guensburg, Reference Guensburg2012; Ausich et al., Reference Ausich, Kammer, Rhenberg and Wright2015; WrightReference Wright , Reference Wright2017). Reference WrightWright’s(Reference Wright2017) phylogenetic analysis of Ordovician through Devonian pentacrinoids recovered a clade comprised of Porocrinus, Carabocrinus, and the hybocrinids Hybocrinus and Hybocystites. Notably, this clade is stemward of the split between flexible and other cladid crinoids. Thus, Reference WrightWright(Reference Wright2017) proposed the name ‘Porocrinoidea’ to encompass this early diverging and morphologically unique clade of Ordovician crinoids.
Our node-based definition of the Porocrinoidea sets up a node-stem triplet that stabilizes the sister clade relationship among the Porocrinida and Hybocrinida recovered by Ausich et al. (Reference Ausich, Kammer, Rhenberg and Wright2015), which had denser taxon sampling of Ordovician crinoids than Reference WrightWright(Reference Wright2017). The Clade Porocrinoidea is likely limited to the Ordovician Period, but additional analyses sampling younger species are needed to test the extent of their geologic duration. Porocrinoids are a subclade of cladids characterized by globose, conical, or ovate calyces that possess a number of apomorphies convergent with blastozoan echinoderms, such as having thecal respiratory structures, reduction in arm number and calyx plates, and/or recumbent ambulacra (see Moore and Teichert, Reference Moore and Teichert1978; Sprinkle, Reference Sprinkle1982a, Reference Sprinkle1982b).
Porocrinida Miller and Gurley, Reference Miller and Gurley1894
Definition
The Porocrinida is stem-defined as the most inclusive clade containing Porocrinus conicus Billings, Reference Billings1857 and Carabocrinus radiatus Billings, Reference Billings1857 but not Hybocrinus conicus Billings, Reference Billings1857.
Remarks
The Porocrinida comprise a small clade of Ordovician porocrinoids with apomorphic endothecal and/or exothecal respiratory structures. Sprinkle (Reference Sprinkle1982a) pointed to many similarities among Carabocrinus, Palaeocrinus, and the Porocrinidae and hypothesized they may be closely related. Ausich et al. (Reference Ausich, Kammer, Rhenberg and Wright2015) recovered a topology supporting this hypothesis with the euspirocrinid Illemocrinus as their sister taxon. However, Reference WrightWright(Reference Wright2017) recovered Euspirocrinus outside the porocrinid clade within a different clade of ‘cyathocrine’ grade cladids. Thus, Illemocrinus is tentatively placed within the Porocrinida, but other taxa within the Euspriocrinidae should not be placed within the Porocrinida at this time as additional revisions are necessary. Guensburg (Reference Guensburg2012) recovered a similar tree to Ausich et al. (Reference Ausich, Kammer, Rhenberg and Wright2015) that suggested Perittocrinus may be also be a porocrinid.
The stem-based definition of the Porocrinida makes them sister to the Hybocrinida and retains the taxonomic membership of this clade recovered in Ausich et al. (Reference Ausich, Kammer, Rhenberg and Wright2015) and Guensburg (Reference Guensburg2012). Porocrinids can easily be distinguished from hybocrinids in having a dicyclic calyx and the presence of thecal respiratory structures (Kesling and Paul, Reference Kesling and Paul1968; Sprinkle, Reference Sprinkle1982a).
Hybocrinida Jaekel, Reference Jaekel1918
Definition
The Hybocrinida is stem-defined as the most inclusive clade containing Hybocrinus conicus Billings, Reference Billings1857 and Hybocystites problematicus Wetherby, Reference Wetherby1880 but not Porocrinus conicus Billings, Reference Billings1857 and Carabocrinus radiatus Billings, Reference Billings1857.
Remarks
Hybocrinids comprise a small yet morphologically disparate clade of Ordovician crinoids. Although the monocyclic hybocrinids have been either considered disparids or classified outside the Inadunata (Moore and Laudon, Reference Moore and Laudon1943; Moore and Teichert, Reference Moore and Teichert1978; Ausich, Reference Ausich1998b), Sprinkle (Reference Sprinkle1982b) suspected hybocrinids might be ‘pseudomonocyclic’ and potentially related to ‘cyathocrine’ cladids (see Sprinkle, Reference Sprinkle1982a, Reference Sprinkle1982b). Phylogenetic analyses by Guensburg (Reference Guensburg2012), Ausich et al. (Reference Ausich, Kammer, Rhenberg and Wright2015), and Reference WrightWright(Reference Wright2017) all support the monophyly of the Hybocrinida and their sister group relationship with taxa placed in the Porocrinida (see Sprinkle, Reference Sprinkle1982b).
In addition to having a pseudomonocyclic calyx (infrabasals absent), hybocrinids are characterized by a number of unusual apomorphies that distinguish them from Porocrinids (and all other crinoids). Many of these traits are similar to those typically present in blastozoan echinoderms, including reduction in the number of arms, modification of food-gathering appendages to be recumbent (sometimes extending downward over calyx plates), and reduction in the number of calyx plates (Sprinkle and Moore, 1978).
Flexibilia Zittel, Reference Zittel1895
Definition
The Flexibilia is stem-defined as the most inclusive clade containing Taxocrinus macrodactylus (Phillips, Reference Phillips1841) but not Dendrocrinus longidactylus Hall, Reference Hall1852.
Remarks
Flexible crinoids are a morphologically homogeneous clade that originated sometime during the Middle to Late Ordovician and range through the Permian. Springer (Reference Springer1911, Reference Springer1920) was the first to recognize that flexible crinoids were closely related to inadunates. In his comprehensive 1920 monograph, The Crinoidea Flexibilia, Springer compared morphologic characteristics of the inadunate Cupulocrinus with the earliest known flexible Protaxocrinus, citing numerous similarities in calyx plating, interradial areas, and the arrangement of posterior plates. Springer (Reference Springer1920) concluded Cupulocrinus was potentially a transitional fossil that linked inadunates with flexibles, stating, “there is clearly an intermingling of the characters … and it is evident that in Cupulocrinus we have to deal with a transition [sic] form whose exact status is difficult to decide” (Springer, Reference Springer1920, p. 89). Subsequent taxonomic treatments have also recognized Cupulocrinus as occupying a proximal position to the base of the flexible tree (Moore and Laudon, Reference Moore and Laudon1943; Moore and Teichert, Reference Moore and Teichert1978).
Phylogenetic analyses sampling flexible and other crinoid taxa have invariably recovered tree topologies supporting Springer’s (Reference Springer1911, Reference Springer1920) hypothesis, with Cupulocrinus recovered as the sister taxon to the Flexibilia (Brower, Reference Brower1995, Reference Brower2001; Ausich, Reference Ausich1998b; Ausich et al., Reference Ausich, Kammer, Rhenberg and Wright2015; WrightReference Wright , Reference Cole2017). Reference WrightWright’s(Reference Wright2017) analysis used Bayesian methods to estimate the probability of Cupulocrinus being ancestral (sensu Foote, Reference Foote1996) to the flexible clade. Results strongly support Cupulocrinus as occupying an ancestral position (posterior probability=0.99) (WrightReference Wright , Reference Wright2017). Given these results and our stem-based definition of the Flexibilia, species of Cupulocrinus are now placed within the flexibles.
Flexible crinoids have loosely sutured calyx plating and a remarkably uniform set of apomorphies relative to other crinoid clades. For example, flexibles differ from cladids in having interradial and intrabrachial plates and differ from other dicyclic crinoids in typically having their lowermost circlet comprised of three (rather than five) infrabasal plates. One infrabasal plate, the ‘azygous’, is smaller than the other two, and is located in the C ray (except for the derived Forbesiocrinus). Many flexibles retain posterior plate arrangements similar to other cladids, but posterior plates are sometimes absent in more derived flexibles. In contrast with cladids, arms of flexible crinoids are universally uniserial and lack pinnules, and the stem is nearly always transversely circular (Springer, Reference Springer1920).
Eucladida WrightReference Wright , Reference Wright2017
Definition
The Eucladida is stem-defined as the most inclusive clade containing Dendrocrinus longidactylus Hall, 1952 and Pentacrinites fossilis Blumenbach, Reference Blumenbach1804 but not Taxocrinus macrodactylus (Phillips, Reference Phillips1841).
Remarks
The revision of the Cladida to be monophyletic requires placing the subclades Porocrinoidea, Flexibilia, and Articulata within a more inclusively defined Clade Cladida (Simms and Sevastopulo, Reference Simms and Sevastopulo1993; WrightReference Wright , Reference Wright2017). However, the Cladida (sensu Moore and Laudon, Reference Moore and Laudon1943) is traditionally conceived as a Paleozoic-age paraphyletic group that excludes the Flexibilia. The Eucladida was proposed by Reference WrightWright(Reference Wright2017) to comprise all species within the Clade Cladida sharing a more recent common ancestor with Dendrocrinus and Pentacrinites than with Taxocrinus. Thus, the stem-defined clades Flexibilia and Eucladida are sister to one another and articulates are nested within the Eucladida. This Eucladida retains much of the meaning and taxonomic content of Moore and Laudon’s (Reference Moore and Laudon1943) concept for Paleozoic cladids while eschewing paraphyly.
In the Treatise on Invertebrate Paleontology, Moore et al. (Reference Moore, Lane and Strimple1978a) recognized three rank-based taxa within the Cladida: the Dendrocrinida, the Cyathocrinida, and the Poteriocrinida. However, it has long been questioned whether these taxa represent monophyletic groups (McIntosh, Reference McIntosh1986, Reference McIntosh2001; Sevastopulo and Lane, Reference Sevastopulo and Lane1988; Kammer and Ausich, Reference Kammer and Ausich1992, Reference Kammer and Ausich1996; Simms and Sevastopulo, Reference Simms and Sevastopulo1993; WrightReference Wright , Reference Wright2015a, Reference Wright2015b; Reference WrightWright, Reference WrightandAusich, Reference Wright and Ausich2015). Indeed, the Poteriocrinida is depicted in the Treatise as a polyphyletic group (Moore et al., Reference Moore, Lane and Strimple1978a, fig. 412). A phylogenetic analysis of Ordovician through Devonian pentacrinoids by Reference WrightWright(Reference Wright2017) has confirmed the doubts over the monophyly of these taxa. Much of the problem arises from ambiguous and/or uninformative apomorphies chosen for these taxa that perpetuate taxonomic anarchy via ‘undiagnostic diagnoses’ (WrightReference Wright , Reference Wright2015b; see Lane, Reference Lane1978, p. T295). Although much revision is needed, recent analyses indicate there is nevertheless considerable phylogenetic structure among subclades of Paleozoic cladids, and additional work is under way to revise this diverse group (WrightReference Wright , Reference Wright2015b).
Articulata Miller, Reference Miller1821
Definition
The Articulata is node-defined as the least inclusive clade containing Endoxocrinus parrae (Gervais, Reference Gervais1835) and Antedon bifida (Pennant, Reference Pennant1777).
Remarks
The Articulata was proposed by Miller (Reference Miller1821) and has since developed a longstanding reputation as a problematic group that lacks a concise and unambiguous definition (Rasmussen, Reference Rasmussen1978; Simms, Reference Simms1988; Simms and Sevastopulo, Reference Simms and Sevastopulo1993; Webster and Jell, Reference Webster and Jell1999; Rouse et al., Reference Rouse, Jermiin, Wilson, Eeckhaut, Lanterbecq, Oji, Young, Browning, Cisternas, Helgen, Stuckey and Messing2013). Although all extant crinoids are invariably recognized as articulates, much confusion surrounds the recognition of fossil articulates and the timing of their origin. The primary difficulties surround which apomorphy (or combination of apomorphies) is useful for diagnosing the Articulata. For example, it is widely appreciated that no apomorphy or unique set of apomorphies can presently diagnose fossil articulates without ambiguity (Simms, Reference Simms1988; Simms and Sevastopulo, Reference Simms and Sevastopulo1993; Webster and Jell, Reference Webster and Jell1999; Rouse et al., Reference Rouse, Jermiin, Wilson, Eeckhaut, Lanterbecq, Oji, Young, Browning, Cisternas, Helgen, Stuckey and Messing2013). Most crinoid workers have obviated this problem by simply treating the Articulata as synonymous with post-Paleozoic crinoids (see Simms and Sevastopulo, Reference Simms and Sevastopulo1993). However, this usage is problematic because this definition is not based on any explicit phylogenetic hypothesis. Moreover, many Paleozoic groups of fossil cladids share different combinations of traits typically listed as ‘diagnostic’ for the Articulata (Webster and Jell, Reference Webster and Jell1999; Webster and Lane, Reference Webster and Lane2007). If the concept of what defines the Articulata depends on the choice of a particular combination of apomorphies alone, then questions regarding the ‘origin of the Articulata’ will always depend on which specific combination was chosen a priori to be diagnostic. Without a phylogenetic definition, it is impossible to objectively specify a precise set of synapomorphies for the Articulata. Thus, we propose herein to define the Articulata as the crinoid crown group containing the last common ancestor of the extant isocrinid Endoxocrinus parrae and the comatulid Antedon bifida, and all of its descendants.
As discussed by Ruta et al. (Reference Ruta, Coates and Quicke2003), the concepts of stem groups and crown groups are sometimes misinterpreted or misused in the paleontological literature. Used properly, crown groups are defined by extant taxon specifiers. Notably, crown groups may be comprised of many (or mostly) extinct fossil species. For example, if a fossil crinoid is more closely related to some extant species than others, it is a member of the crown group. According to Rouse et al. (Reference Rouse, Jermiin, Wilson, Eeckhaut, Lanterbecq, Oji, Young, Browning, Cisternas, Helgen, Stuckey and Messing2013), the most recent common ancestor of all extant crinoids lived sometime during the Middle to Upper Triassic. Thus, our node-based definition eliminates the non-phylogenetic concept of ‘post-Paleozoic Crinoidea’ while retaining the majority of post-Paleozoic crinoids traditionally included within the Articulata. The Clade Articulata is synonymous with the Crown Crinoidea (Sumrall, Reference Sumrall2014), and we advocate workers use these terms interchangeably depending on context (e.g., discussing relationships among crinoids or between crinoids and non-crinoids). Traits that may be present in the Articulate ancestor are listed in Simms (Reference Simms1988), Simms and Sevastopulo (Reference Simms and Sevastopulo1993), Webster and Jell (Reference Webster and Jell1999), and Rouse et al. (Reference Rouse, Jermiin, Wilson, Eeckhaut, Lanterbecq, Oji, Young, Browning, Cisternas, Helgen, Stuckey and Messing2013).
The Articulata likely contains most post-Paleozoic taxa traditionally considered articulates, including the ~600 or so extant species. Although we define Articulata with precision and phylogenetic stability (Rouse et al., Reference Rouse, Jermiin, Wilson, Eeckhaut, Lanterbecq, Oji, Young, Browning, Cisternas, Helgen, Stuckey and Messing2013, Reference Rouse, Carvajal, Oji and Messing2015), it remains difficult in practice to unambiguously identify fossil articulates, particularly among specimens near the base of the articulate tree. However, such difficulties are already present and have long obfuscated the origin of the crinoid crown group. The more important problem is resolving the phylogenetic position of the common ancestor of extant crinoids within the myriad of fossil lineages. Our definition provides a useful framework for future phylogenetic research to uncover relationships between potential stem articulates, extinct crown group lineages, and extant species.
A revised rank-based classification of the Crinoidea
Crinoid clades identified herein confirm many long-held views on the major divisions among crinoids from both the foundational work of Moore and Laudon (Reference Moore and Laudon1943) and Moore and Teichert (Reference Moore and Teichert1978) to more recent analyses (i.e., Ausich, Reference Ausich1998a, Reference Ausich1998b; Guensburg and Sprinkle, Reference Guensburg and Sprinkle2003; Guensburg, Reference Guensburg2012; Ausich et al., Reference Ausich, Kammer, Rhenberg and Wright2015). Results from all of these studies recognized the Camerata, Diplobathrida, Monobathrida, Hybocrinida, Disparida, Cladida, and Flexibilia. The challenge is to represent these widely recognized clades in a rank-based Linnaean classification scheme that maximizes common usages of names for crinoid lineages (Moore and Teichert, Reference Moore and Teichert1978) and is consistent with a phylogenetic understanding of relationships (Wiley and Lieberman, Reference Wiley and Lieberman2011). In our revision, the Crinoidea remain a class and every attempt is made to retain orders as recognized in Moore and Teichert (Reference Moore and Teichert1978). Unfortunately, the tree topology of Figure 2 prevented the attainment of the latter in all instances, but the addition of intermediate Linnaean ranks makes it easier to apply a phylogenetic perspective to rank-based crinoid classification. The use of intermediate ranks (e.g., Parvclass) follows traditional use in pre-existing taxonomic literature (see Carroll, Reference Carroll1988; Sibley, Reference Sibley1994; Benton, Reference Benton2005). Two older taxonomic names, the Pentacrinoidea Jaekel, Reference Jaekel1918 and Inadunata Wachsmuth and Springer, 1885, are formally reinstated herein because they represent meaningful clades as described above.
Post-Ordovician cladids (sensu Moore and Laudon, Reference Moore and Laudon1943) and the Protocrinoida (Guensburg and Sprinkle, Reference Guensburg and Sprinkle2003) remain problematic groups. Because the rank for a monophyletic Cladida must be above flexibles and articulates (Simms and Sevastopulo, Reference Simms and Sevastopulo1993), we propose the name Cyathoformes to contain taxa traditionally placed within the Cladida that are sister to the Articulata. Relationships among these taxa are the subject of future phylogenetic research (WrightReference Wright , Reference Wright2015b) and are not treated further here. From their initial description (Guensburg and Sprinkle, Reference Guensburg and Sprinkle2003), the protocrinoids have been an important but confounding group of crinoids that display characteristics of both crinoids and other stalked echinoderms. Guensburg and Sprinkle (Reference Guensburg and Sprinkle2003) regarded the protocrinoid as an “order (plesion)”. The validity of the protocrinoids was later questioned by Guensburg and Sprinkle (Reference Guensburg and Sprinkle2009) and led Guensburg (Reference Guensburg2012) to formally place them within the Camerata. However, Ausich et al. (Reference Ausich, Kammer, Rhenberg and Wright2015) recovered a sister group relationship between Titanocrinus and Glenocrinus, but with protocrinoids more closely related to non-camerates than camerates. In contrast, Reference ColeCole’s(Reference Cole2017) analysis of Ordovician crinoids recovered the protocrinoids as more closely related to camerates than non-camerates. Thus, we have carefully chosen our clade definitions to not depend on a particular phylogenetic hypothesis or morphologic interpretation of these significant but problematic taxa. For the moment, we tentatively place both protocrinoid taxa as Crinoidea incertae sedis subclass Protocrinoida.
In our present understanding of crinoid evolution, the first major divergence occurs between camerates and all other crinoids (Fig. 2). The subclass rank is retained for the Camerata; and the subclass Pentacrinoidea Jaekel, Reference Jaekel1918 is proposed for its sister group (Table 2). Within the Camerata, the orders Diplobathrida and Monobathrida are retained as sister groups, and the infraclass Eucamerata Reference ColeCole(Reference Cole2017) unites these two orders. In phylogenetic analyses of camerates, several taxa are not placed within the Monobathrida and Diplobathrida (sensu ColeReference Cole , Reference Cole2017). Thus, they are considered here to be stem eucamerates (see remarks for Eucamerata above). The subclass Camerata unites these stem taxa with eucamerates.
Table 2 Revised rank-based classification of the Crinoidea.

In terms of species richness, the subclass Pentacrinoidea is the largest crinoid clade. This includes the Disparida, Cladida, Hybocrinida, and Articulata of Moore and Teichert (Reference Moore and Teichert1978), which coincides exactly with Jaekel’s (Reference Jaekel1918) concept of the Pentacrinoidea (see Lane, Reference Lane1978). Hence, we have proposed the reinstatement of this name. The Pentacrinoidea is comprised of the infraclass Inadunata and their stem taxa (e.g., Apektocrinus). The concept for the Inadunata in Moore and Teichert (Reference Moore and Teichert1978) united the Disparida and the Cladida. Here, the infraclass Inadunata unites the Disparida, Cladida, and all of their descendants. This usage circumvents the non-phylogenetic usage of the Inadunata (sensu Moore and Teichert, Reference Moore and Teichert1978) and is consistent with the phylogenetic conclusions of Simms and Sevastopulo (Reference Simms and Sevastopulo1993). With the Inadunata an infraclass, the Disparida and Cladida are both parvclasses. Taxa placed within the Disparida are in need of revision, and current work is underway to establish relationships among sublcades (Ausich and Donovan, Reference Ausich and Donovan2015).
Within the Cladida, the Hybocrinida, Porocrinida, Taxocrinida Springer, Reference Springer1913 and Sagenocrinida Springer, Reference Springer1913 are orders (Table 2). The orders Hybocrinida and Porocrinida are sister groups forming the superorder Porocrinoidea Reference WrightWright(Reference Wright2017). The Flexibilia are transferred to the superorder rank, which is comprised of the two sister groups, order Taxocrinida and order Sagenocrinida. The Cyathoformes and Articulata comprise the magnorder Eucladida (WrightReference Wright , Reference Wright2017). The Eucladida retains most cyathocrinids, dendrocrinids, and poteriocrinids of Moore and Teichert (Reference Moore and Teichert1978). As discussed above, phylogenetic relationships within this clade await further study (WrightReference Wright , Reference Wright2015b). Analyses detailing the late Paleozoic and early Mesozoic crinoid phylogeny are needed to understand this crucial period of crinoid evolution. Lastly, the Articulata is considered a superorder within the Cladida.
Conclusions
A phylogeny-based revision of crinoid systematics is proposed to clarify the definition of clades and inform a major revision of the rank-based Linnaean classification. These revisions are based on recent computational phylogenetic analyses that build on the historic subdivision of crinoids into major lineages. It is hoped that the phylogenetic classification schemes presented herein will help provide a framework for future research on crinoid phylogeny and offer guidance to crinoid workers and non-specialists alike interested in using this fascinating group of echinoderms to study evolutionary patterns and processes.
Acknowledgments
We thank the organizing committee of the 2015 Progress in Echinoderm Paleobiology conference and guest editors S. Zamora and I.A. Rahman for inviting us to submit this contribution. G.D. Sevastopulo and an anonymous reviewer are thanked for their helpful reviews and useful comments. I.A. Rahman provided careful editorial assistance and made several suggestions that improved the manuscript. This research was supported by grants from numerous organizations, including the National Science Foundation (DEB 1036416, WIA), the Paleontological Society’s N.G. Lane award (DFW, SRC), Sigma Xi (DFW, SRC), Palaeontological Association (DFW, SRC), the American Federation of Mineralogical and Geological Societies Student Scholarship (DFW, SRC), and The Ohio State University School of Earth Sciences’ Friends of Orton Hall Scholarship (DFW, SRC). DFW was also supported by an Ohio State University Presidential Fellowship.