Introduction
The strictly endoparasitic, holometabolous insect order Strepsiptera, sometimes called ‘twisted wing parasites’, comprises around 630 known species (Kathirithamby, Reference Kathirithamby, Foottit and Adler2018), inhabiting the entire world except for Antarctica and Greenland (Ulrich, Reference Ulrich1929). The group is known for a number of exceptional features like extreme sexual dimorphism with larviform females (Kinzelbach, Reference Kinzelbach1971), hypermetamorphosis with extremely miniaturized primary larvae (Kathirithamby, Reference Kathirithamby1989; Pohl and Beutel, Reference Pohl and Beutel2019), exceptional eyes (Buschbeck et al., Reference Buschbeck, Ehmer and Hoy2003) and traumatic insemination (Peinert et al., Reference Peinert, Wipfler, Jetschke, Kleinteich, Gorb, Beutel and Pohl2016).
In host choice, the species Stylops ater Reichert, 1914 is mostly confined to Andrena vaga Panzer, 1799 (grey-backed mining bee), which is specialized on Salix (willow) pollen, often nests in large aggregations of several thousands and is relatively common in sand-rich regions in central Europe (Jůzová et al., Reference Jůzová, Nakase and Straka2015; Westrich, Reference Westrich2019). In Germany, non-parasitized bees of A. vaga are active between March and May (Westrich, Reference Westrich2019).
At the point of appearance of their host bee, male puparia and female cephalothoraces are already extruded from the host's abdomen, the position regularly being laterally in the intersegmental membrane between the 4th and 5th tergite and rarely between other tergites or even sternites (Linsley and MacSwain, Reference Linsley and MacSwain1957; Jones et al., Reference Jones, Williams and Jones1980; Balzer and Davis, Reference Balzer and Davis2020). Shortly after host emergence, male Stylops emerge and are immediately able to fly while females permanently remain inside, apart from the cephalothorax, and release strong pheromones to attract mates (Cvačka et al., Reference Cvačka, Jiroš, Kalinová, Straka, Černá, Šebesta, Tomčala, Vašíčková, Jahn and Šobotník2012; Tolasch et al., Reference Tolasch, Kehl and Dötterl2012; Löwe et al., Reference Löwe, Beutel and Pohl2016). Stylops males copulate with the extruded female parasite (Fig. 1A). Agile primary larvae with a length of around 200 μm are asynchronously released in high numbers (up to 7000) after ~20–40 days through a ventral brood canal (Linsley and MacSwain, Reference Linsley and MacSwain1957; Jones et al., Reference Jones, Williams and Jones1980; Fraulob et al., Reference Fraulob, Beutel, Machida and Pohl2015; Balzer and Davis, Reference Balzer and Davis2020). They infest new hosts at an early developmental stage (Linsley and MacSwain, Reference Linsley and MacSwain1957; Knauthe et al., Reference Knauthe, Beutel, Hörnschemeyer and Pohl2016) and have been shown to reach them by phoretic transport in the hair, pollen load or even inside the crop of flower-visiting bees (Ulrich, Reference Ulrich1956; Linsley and MacSwain, Reference Linsley and MacSwain1957; Balzer and Davis, Reference Balzer and Davis2019).

Fig. 1. (A) Two male Stylops ater in copulation attempt with an extruded female (not visible in photograph) in a female Andrena vaga [Theaterpark (Braunschweig), 25.02.2021; photograph credit: Robin Schmidt]. (B) Egg of A. vaga with primary larvae of S. ater on pollen provision in brood cell. (C) Dissected abdomen of female A. vaga parasitized by female S. ater. (D) Dissected abdomen of female A. vaga formerly parasitized by male S. ater (ce, cephalothorax; il, ileum; mp, male puparium; re, rectum; sa, Stylops abdomen).
Few studies have looked at the prevalence of stylopized bees in Andrena populations and further traits of parasitization. Jensen (Reference Jensen1971) found a stylopization rate of 18% in a Danish aggregation of A. vaga and remains the only author to examine this specific host–parasite interaction. While the latter did not distinguish between male and female hosts and parasites, Pierce (Reference Pierce1909) found females to be over-represented in stylopized individuals in a population of Andrena crawfordi Viereck, 1909. The prevalence of pluristylopization (≥2 Stylops per host) seems to vary greatly across host species, region and year (Jones et al., Reference Jones, Williams and Jones1980; Balzer and Davis, Reference Balzer and Davis2020).
Due to the short life span of male S. ater, genetic exchange between populations is mainly driven by migration of infested or larva-carrying host bees (Tolasch et al., Reference Tolasch, Kehl and Dötterl2012). Aggregations of A. vaga are in strong genetic exchange in areas meeting the requirements of this specialized bee as shown for Northern Germany (Exeler et al., Reference Exeler, Kratochwil and Hochkirch2008). Therefore, a relatively high genetic homogeneity is to be expected from their parasites but was not investigated in detail so far.
Regarding the morphology of Stylops, Maeta et al. (Reference Maeta, Gôukon, Kitamura and Miyanaga2001) as well as Balzer and Davis (Reference Balzer and Davis2020) found that the female cephalothorax width varied with extrusion position in related Strepsiptera taxa but the effect of host sex and the host's degree of stylopization on this feature was not examined so far.
Effects of stylopization on the host are dramatic and include changes in phenology and behaviour (Nakase and Kato, Reference Nakase and Kato2021) as well as internal and external morphology. Andrena bees parasitized by Stylops emerge a few weeks earlier than their conspecifics (Pierce, Reference Pierce1909; Brandenburg, Reference Brandenburg1953; Straka et al., Reference Straka, Rezkova, Batelka and Kratochvíl2011). Internal morphological alterations due to stylopization include a slight (in hosts with male Stylops) or strong (in hosts with female Stylops) ovary reduction in females (Brandenburg, Reference Brandenburg1953; Balzer and Davis, Reference Balzer and Davis2021), while male bees showed less alteration in external and internal copulatory organs (Salt, Reference Salt1927). External morphological alterations often include the expression of characteristics of the opposite sex and therefore a phenotypical intersexualization (Pérez, Reference Pérez1886; Pierce, Reference Pierce1909; Salt, Reference Salt1927; Brandenburg, Reference Brandenburg1953). Typical female features associated with pollen transport (scopa and flocculus) are reduced in stylopized females and enhanced in stylopized males, while typical male features such as the bright facial markings present in many Andrena species are reduced in stylopized males and enhanced in stylopized females. These alterations increase in magnitude with a growing number of Stylops per host and have been observed to be more severe in bees hosting male parasites (Salt, Reference Salt1927). The latter also reported a slight decrease in size of the whole body and especially the males' head in stylopized individuals of some Andrena species. The tergal cuticula of stylopized Andrena bears a stronger vestiture, especially near the extrusion location of the parasite (Brandenburg, Reference Brandenburg1953; Pohl et al., Reference Pohl, Gorb and Gorb2020).
Considering the partially poor understanding of the biology of Strepsipterans such as S. ater and its simultaneous relevance due to the possible effects on populations of important pollinators, the objectives of the present study were (1) to assess parasitization of A. vaga by S. ater, including pluristylopization, parasite sex ratio and preferences for host sex and position of extrusion, (2) to gain clarity on the stage and mode of host infestation in Stylops, (3) to examine species diversity of the parasites and genetic variability in S. ater from different A. vaga aggregations and possible correlations of genetic and spatial distance, (4) to investigate the impact of host sex and parasitization level on female Stylops size and (5) to verify the impact of infestation by S. ater on the phenology and morphology of A. vaga and discuss possible implications.
Materials and methods
Sampling
Andrena host specimens were collected in 2 phases of sampling from 23rd February to 3rd March 2021 and from 12th April to 16th May 2021 (for geographic location and details of sampling sites and dates, see Fig. S1 and Tables S1 and S2). All sites comprised of 15 A. vaga aggregations of varying distances in and around the city of Braunschweig (Lower Saxony, Germany) as well as 2 aggregations in Göttingen (Lower Saxony) and 1 in Kassel (Hesse). Bees were collected with hand-held insect nets regardless of visible stylopization. Additionally, free-flying Stylops males were sampled randomly for genetic studies. The 2nd sampling was incomplete and served mainly to sample unstylopized female specimens from 12 of the sites sampled in the 1st phase, for control measurements. Unstylopized male species for comparison were obtained only from 1 site (TU – TU Nordcampus) and comprised of specimens caught in pan-traps during the annual wild bee monitoring of the BeesUp project. Specimens were sacrificed in a freezer and preserved in 96% ethanol.
A total of 25 intact brood cells were excavated from an A. vaga aggregation at the site MS (Melverode Sportplatz) (6 on 4th May 2022 and 19 on 11th May 2022). For that purpose, a surface area of 0.7 m2 was dug out to a depth of around 0.5 m, where most of the cells were found.
Parasitization analysis and search for Stylops larvae
Bees, brood cells and pollen loads were analysed for parasite occurrence (Fig. 1C and D) using a Zeiss Stemi 305 binocular microscope; photographs were captured with a Zeiss Axiocam (Carl Zeiss Microscopy, Oberkochen, Germany). Female Stylops and males that had not yet emerged were removed and stored separately in 96% ethanol. Parasitization by already emerged male Stylops was detected by a hole in the intersegmental membrane and remains of the empty male puparium. Number and sex, as well as segment interval and side of Stylops extrusion in each bee were noted.
Bee eggs and larvae from excavated brood cells were examined from the outside and also dissected to search for parasitic larvae inside. Pollen provisions from 5 brood cells were suspended in ethanol and dispersed on a paper sheet to be gradually examined for Stylops primary larvae. Pollen loads of 20 representative unstylopized A. vaga females from the 2nd sampling period in 2021 (here: 24th April to 15th May) were examined for Stylops primary larvae by thoroughly shaking the bee in an ethanol-filled 2 mL tube and examining the resulting suspension in the same way as the pollen provision from the brood cells.
DNA extraction, polymerase chain reaction (PCR) and phylogenetic analysis
A total of 127 representative Stylops females and 26 males were selected for genetic analysis. DNA was extracted from the cephalothorax using proteinase K (20 mg mL−1) digestion followed by a standard salt DNA extraction protocol (Bruford et al., Reference Bruford, Hanotte, Brookfield, Burke and Hoelzel1992). Amplification for parts of the mitochondrial barcoding gene cytochrome c oxidase subunit 1 (COI) and the nuclear genes H3 and 18S rRNA was realized using gene-specific primers and protocols (Tables S4–S6).
Unidirectional Sanger sequencing of PCR fragments was performed for 153 (COI), 21 (H3) and 23 (18S) individuals by LGC Genomics (Berlin, Germany). Sequences were manually inspected and trimmed using CodonCode Aligner (V.10.0.2, CodonCode Corporation, Centerville, MA, USA). MEGA7 (Kumar et al., Reference Kumar, Stecher and Tamura2016) was used for sequence alignments before blasting them for species assignment using nucleotide BLAST provided by GenBank (NCBI). MEGA7 was also used for constructing a maximum-likelihood (ML) phylogenetic tree for the gene COI, based on the Tamura–Nei model (Tamura and Nei, Reference Tamura and Nei1993) with a discrete Gamma distribution (+G) and analysis of evolutionary invariability (+I), computed with 100 ML bootstrap replications. This model was proposed as the best fit by the model finder provided in MEGA7. Voucher sequences from Smit et al. (Reference Smit, Smit, Raemakers and van der Hoorn2020) and Jůzová et al. (Reference Jůzová, Nakase and Straka2015) were incorporated in the phylogenetic tree for reference and as outgroups.
Preparation and morphological measurements
In a total of 133 representative Stylops females from 9 sites, the cephalothorax width as a proxy for body size was measured as shown in Fig. S2.
A representative selection of 157 A. vaga females and 14 males from 5 sampling sites with high numbers of sampled individuals (IW – Inselwall-Park, MS, RK – Rüningen Kirche, SD – Schillstraße Denkmal, TU), either bearing a single female/male or no Stylops, were chosen for morphometric examination of the head, hind legs and abdomen by using a Zeiss Stemi 305 microscope equipped with the Zeiss Axiocam camera module and ZEN Core 2 v2.5 measuring software (Carl Zeiss Microscopy, Oberkochen, Germany). Additionally, from 2 representative sites (TU, RK), the intertegular distances (ITDs) were measured. From 1 representative site (MS), female bees were dissected for the examination of their ovaries. Where possible, all measurements and examinations were conducted without knowledge on the parasitization status of the host bee to avoid bias.
Head width and ITD as 2 proxies for body size/weight (O'Neill, Reference O'Neill1983; Hagen and Dupont, Reference Hagen and Dupont2013) were measured (Fig. S2). For the head width, preliminary tests showed that the deviation of independent measurements was lower than 1%, so that only 1 photograph was captured per bee. For the ITDs, specimens were measured thrice and the average was used for further comparison. The head width was additionally divided by the ITD as a form of normalization to body size.
The width and length of the metabasitarsus were measured (Fig. S2) to obtain their ratio as a proxy for the shape of the whole leg. The length was additionally divided by the ITD as a form of normalization to the body size. To eliminate effects of the photographic angle, the joint was isolated and fixed on a clear plastic foil using clear tape.
Ovaries were isolated from the abdomen and one of the 3 scores (Fig. S3) was attributed to the system in Beani et al. (Reference Beani, Dallai, Mercati, Cappa, Giusti and Manfredini2011). The minimal egg length for an attribution of score 3 (1000 μm) was derived from investigations of Brandenburg (Reference Brandenburg1953).
Finally, abdomens were cleaned and dried using soapy water, acetone and a hairdryer. Hair coverage of the 4th tergite was estimated separately for the left and right half of the segment by attributing one of the 5 pre-designated scores (Fig. S5A).
Statistical analysis
Statistical analyses were performed using standard spreadsheet software (Excel 2016). Ratios of population characterization were analysed using chi-squared (χ 2) tests. Parametric data (measurements on head, thorax and legs of Andrena and cephalothorax of Stylops) were examined for normal distribution using Kolmogorov–Smirnov tests and groups were compared using analysis of variance (ANOVA) and post-hoc Student's t-tests. For non-parametric data (abdominal hair scores), a Kruskal–Wallis test and post-hoc Mann–Whitney U-tests were used. Possible correlation of Stylops cephalothorax width and Andrena head width was examined by Pearson correlation. All P values obtained from multiple testing were corrected using the Bonferroni–Holm method.
Results
Parasitization analysis and search for Stylops larvae
In the 1st sampling phase, all 508 A. vaga individuals were stylopized without exception and hosted a total number of 574 present or emerged (male puparia) Stylops. The sex ratio of hosts and parasites as well as the prevalence of pluristylopized hosts among different sampling sites is shown in Fig. 2. Variability among sites was rather high but showed clear trends in host and parasite sex ratios.

Fig. 2. Summary of 14 A. vaga aggregations in Braunschweig, Göttingen and Kassel (see Table S1 for details) from the 1st sampling phase. (A) Percentage of A. vaga infested by different numbers of Stylops (different shades of grey). (B) Percentage of male and female Stylops in male and female A. vaga (different shades of red and blue).
On average, among the sampled stylopized individuals, females had a significantly higher share (61.6%) than males (χ 2 test: χ 2(1) = 26.22, n = 487, P = 0.004). The percentage of female Stylops was significantly higher (64.1%) in female Andrena (χ 2(1) = 25.15, n = 315, P = 0.006) while that of male Stylops was significantly higher (61.7%) in male Andrena (χ 2(1) = 10.49, n = 193, P = 0.013). Out of 249 parasitizations by male Stylops, only 12 were still present and the cephalotheca of their puparium externally visible.
Overall, the percentages of hosts with 1 parasite were 89.57% (454 bees), with 2 parasites was 8.86% (46 bees), with 3 parasites was 1.38% (7 bees) and only 0.2% (1 bee) were infested with 4 Stylops. The prevalence of pluristylopization was slightly higher in female hosts (10.84%) than in male hosts (9.73%) and slightly higher among female Stylops (7.77%) than among male Stylops (5.08%). Of the 46 bees infested by 2 Stylops, 10 hosted 2 males, 16 hosted 2 females and 20 hosted a male and a female.
The position of extrusion was usually the intersegmental membrane between the 4th and 5th abdominal tergite, only 15 (2.6%) of the Stylops extruded from between the 3rd and 4th tergite. In that case, apart from 1 female and 1 male Stylops, the host was always pluristylopized. Regarding the side of extrusion (left or right) of single Stylops from the abdomen of their host, there was a general tendency for females to extrude from the right half of the abdomen (57.20%) and for males to extrude from the left half of the abdomen (61.31%), which was significant only in the latter case (χ 2(1) = 10.18, n = 199, P = 0.014). Only 8 Stylops emerged from the middle of the tergite intersegmental membrane. Of these, 6 developed in hosts with at least 3 parasites and were therefore displaced from the usual side position.
In the 2nd sample phase, only 2 of 150 A. vaga were infested, both with a female Stylops. These Stylops were strongly enlarged and contained eggs and weakly pigmented embryos in high quantities.
Of the 19 excavated brood cells, presumably of A. vaga, 7 contained recently hatched Andrena larvae of ~3–4 mm. Two cells contained a different kind of bee larvae that were tentatively assigned to Nomada lathburiana Kirby, 1802, a specialized cleptoparasite of A. vaga. The other 10 cells contained elongated eggs of ~2.5 mm length. In 4 of them, dark primary larvae (or their exuviae) of ~200 μm length were visible (Fig. 1B; 3 contained 1 primary larva each, 1 contained 3 primary larvae). A closer examination showed that they all were situated in the gap between the chorion and the developing embryo. While most Andrena larvae were alive, the Stylops larvae found in the eggs were motionless. Further dissection of bee eggs and larvae did not reveal any more Stylops larvae.
Five examined pollen provisions (3 from cells containing stylopized eggs and 2 additional ones) did not contain any Stylops primary larvae, nor did any of the 20 investigated pollen loads of provisioning A. vaga females.
Phylogenetic analysis
Blasting of COI sequences revealed a genetic identification as S. ater for all 127 sequenced female Stylops from A. vaga and 26 free-flying males. Therefore, it can be assumed that all consequences for the bees shown in this study were caused by this single parasite species. Sequences for COI, H3 and 18S were extremely conserved among individuals. Species unity and very high conservation can also be seen in the ML phylogenetic tree for COI, including earlier voucher sequences for S. ater and Stylops spp. (Fig. 3). Sequences of representative specimens are accessible from GenBank (NCBI) under their IDs (OQ333011–OQ333020).
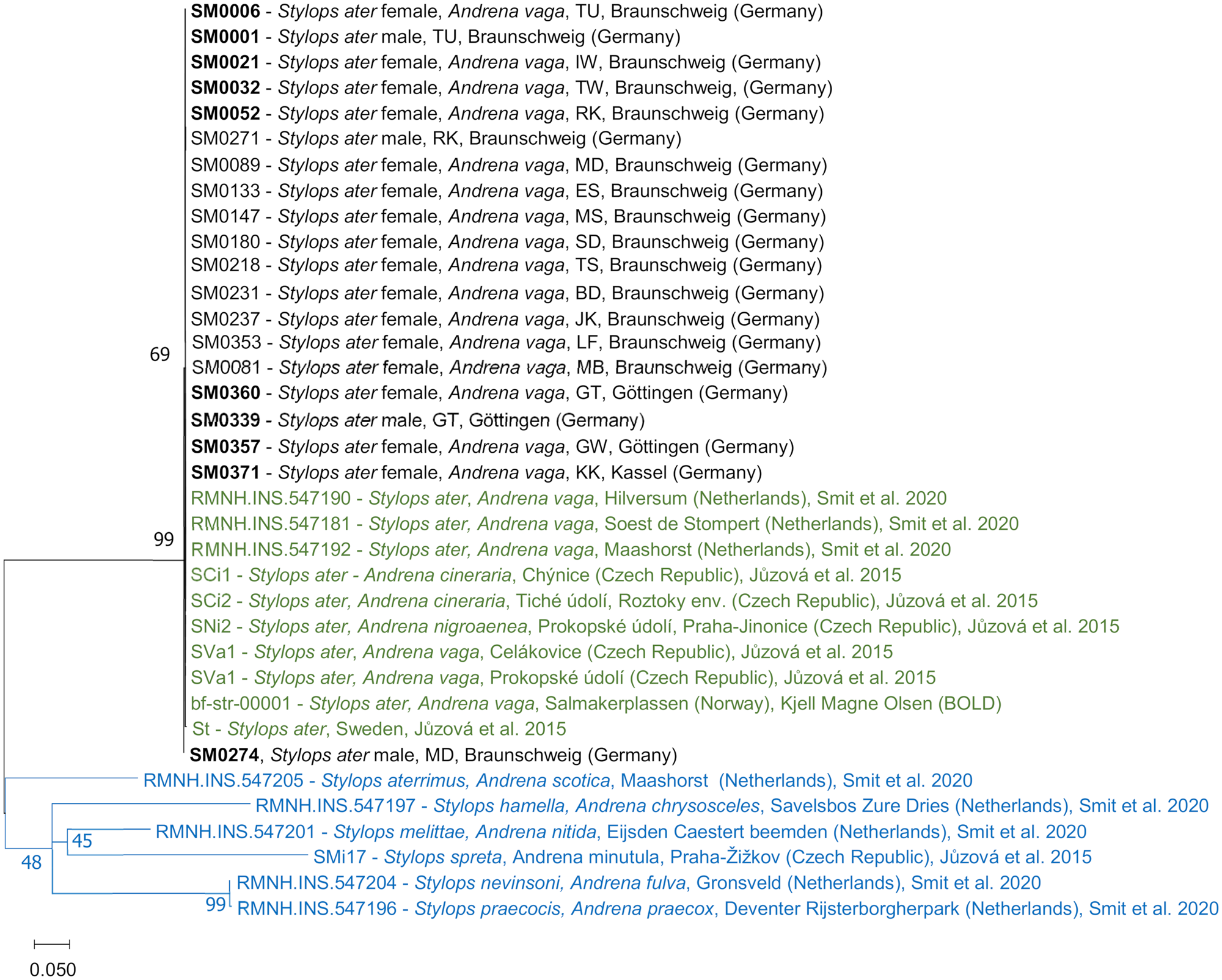
Fig. 3. ML phylogenetic tree for the gene COI of selected Stylops specimens. Based on the Tamura–Nei model with a discrete Gamma distribution [5 categories (+G, parameter = 0.934)] and analysis of evolutionary invariability [(+I), 62.29% sites], computed with 100 bootstraps, with a total of 535 positions in the final dataset. Branch lengths are measured in the number of substitutions per site. Labels show Stylops species and sex (if known), host species, site, country and (if applicable) source of sequences. Database-obtained samples of S. ater are shown in green; database-obtained samples of other Stylops species that serve as an outgroup are shown in blue. Voucher sequences uploaded to NCBI GenBank are in bold.
Only in 1 male S. ater from the site MD (Madamenweg Discgolfanlage, voucher ID: SM0274), a single base transition (G–A) was found in the COI sequence at position 292. Another transition (C–T) was found in the COI sequence (position 276) of an S. ater from Sweden (sample ID: st) from Jůzová et al. (Reference Jůzová, Nakase and Straka2015).
Size variation of Stylops females
Cephalothorax widths of Stylops females from monostylopized male and female hosts were normally distributed (Kolmogorov–Smirnov test: n = 65, Z = 0.11, P = 0.385) and no site effect was found in male or female hosts (ANOVA: d.f.female = 64, P female = 0.568, d.f.male = 33, P male = 0.319). Consequently, Stylops from rarer parasitization situations (2 females, 1 female and 1 male) were pooled among sites. The average cephalothorax width of Stylops females was significantly higher (5.22%) in monostylopized female A. vaga hosts compared to monostylopized male hosts [Student's t-test: t(48) = 4.13, P = 0.002] (Fig. 4). The Stylops' cephalothorax was significantly narrower when another female Stylops was present in the female host [3.93%, t(81) = 4.31, P = 0.002] or when it previously shared the host with a male Stylops [2.97%, t(73) = 2.64, P = 0.042]. The sex of the 2nd parasite had no effect on cephalothorax width [t(26) = −0.66, P = 0.501]. There was no significant correlation between the head width of monostylopized female host bees and the cephalothorax width of their parasite (Pearson correlation, n = 46, r 2 = 0.055, P = 0.718).

Fig. 4. Cephalothorax width variation in S. ater females. (A) Female extracted from the host abdomen (ce, cephalothorax; bo, birth opening; bc, brood canal). (B) Cephalothorax width of Stylops females in different host–parasite situations. Boxplots show minima and maxima (whiskers), medians and 1st and 3rd quartiles (boxes), means (cross) and outliers (empty circles) (*P < 0.05, **P < 0.01).
Impact of stylopization on host morphology
In all dissected unstylopized A. vaga females (n = 10), ovaries were normally developed and contained small as well as fully developed eggs (score 3, Fig. S3). Bees infested by a single female Stylops (n = 10) showed no obvious egg development in their tube-like ovaries, which were not reduced in length (score 1, Fig. S3). The ovaries of bees infested by a single male Stylops (n = 10) contained small, poorly developed eggs (score 2, Fig. S3).
Average ITDs of A. vaga females were normally distributed (n = 55, Z = 0.121, P = 0.368). There was no significant difference between infested and uninfested bees [ANOVA: F(5) = 1.610, P = 0.175] (Fig. S4A).
Head widths of unstylopized bees were normally distributed (n = 150, Z = 0.084, P = 0.231) but at 2 sites (MS, SD) differed significantly from one another in unstylopized A. vaga females [ANOVA and post-hoc Student's t-tests: t(22) = 3.40, P = 0.031]. With all sites pooled, head width was significantly reduced (by around 2.39%) in bees with a male Stylops compared to unstylopized individuals [t(101) = 3.56, P = 0.001] (Fig. 5A). Looking at the groups of stylopization across sites, a low mean head width of bees without Stylops and high mean head width of bees with female Stylops was noticeable for the site MS. Therefore, this site was defined as an outlier and removed from the pool for an alternative model shown in Fig. S4B, where the difference in head width became clearer and even female Stylops reduced their hosts’ head width significantly. For comparison, average head width of unstylopized males at the site TU was clearly lower but also more variable (3774.87 ± 222.25 μm, n = 14).

Fig. 5. Summary of host bee morphological changes. (A) Head width of A. vaga females of different stylopization status. (B) Head width/ITD ratio of A. vaga females of different stylopization status (site TU). (C) Metabasitarsus length/width ratio of A. vaga of different groups. (D) Metabasitarsus length/ITD ratio of A. vaga of different groups. Boxplots show minima and maxima (whiskers), medians and 1st and 3rd quartiles (boxes), means (cross) and outliers (empty circles) (*P < 0.05, **P < 0.01, ***P < 0.001). (E) Hind legs of different A. vaga individuals (I, unstylopized female; II, stylopized female; III, unstylopized male; co, coxa; fe, femur; fl, flocculus; ml, metabasitarus length; mw, metabasitarsus width; sc, scopa; ti, tibia; tr, trochanter).
Average head width/ITD ratio was significantly higher in bees with a female Stylops compared to bees without a Stylops [t(15) = 3.63, P = 0.019] (Fig. 5B). This means their heads, even though tending to be smaller in absolute numbers, were proportionally larger than that in unstylopized females. In a greater extent, this was also the case in unstylopized A. vaga males, where this ratio averaged to 1.856 ± 0.139 (n = 14).
In stylopized A. vaga females, compared to unstylopized individuals, all hind leg joints appeared more slender and the basitarsus was less tapered apically. The hair, especially the flocculus on the trochanter and the scopa on the tibia, but also on the basitarsus, were shorter and less dense. While its colour seems slightly darkened on the coxa, trochanter and femur, it appears partly brightened on the tibia and basitarsus. In all the above characteristics, the leg looks like a transitional form between that of unparasitized females and males (Fig. 5E). The metabasitarsus lengths and widths of unstylopized bees were normally distributed (n = 50, Z length = 0.113, Z width = 0.0801, P length = 0.505, P width = 0.880). Sites were pooled as the ratios did not differ significantly among unstylopized bees [ANOVA: F(4) = 1.597, P = 0.194]. In comparison to unstylopized females, the ratio was significantly higher in bees with a female Stylops [t(15) = 19.48, P = 0.002] as well as bees with a male Stylops [t(18) = 19.80, P < 0.001] (Fig. 5C). The latter 2 did not differ from each other [t(15) = 0.02, P = 0.417]. This means that the legs of stylopized A. vaga females are 16.5% leaner compared to those of unstylopized individuals. With this, they direct towards the higher ratio in A. vaga males (6.013 ± 0.066, n = 14). The metabasitarsus length/ITD ratio, in comparison to unstylopized females, was significantly higher in bees with a female Stylops [t(15) = 5.39, P = 0.002] and with a male Stylops [t(17) = 7.14, P < 0.001] (Fig. 5D). The 2 latter also differed significantly from each other [t(14) = 2.43, P = 0.045]. The ratio in bees with a male Stylops did not differ from that in A. vaga males [0.961 ± 0.133, t(21) = 0.2, P = 0.828].
A Kruskal–Wallis test and post-hoc Mann–Whitney U-tests revealed a significantly lower mean (left and right side) hair score for unstylopized A. vaga from the site MS compared to several other sites. Again, MS was excluded from further tests to avoid possible site effects. Currently, we have no explanation for the observed variance at site MS, except for the late sampling. The difference in hairiness of the 4th tergite is shown in Fig. S5. On the half where the Stylops was extruded, in comparison to unstylopized individuals, hair scores were generally higher in bees infested with a Stylops of any sex; the same was obvious for the opposite side of the segment. The difference between the 2 halves of the segment was significant (multiple Mann–Whitney U-tests, all P < 0.001) (Fig. S5). Stylops females had a significantly higher impact on the abdominal hairiness compared to males, both on the side of extrusion (U = 247, P < 0.001) as well as on the opposite side (U = 247, P = 0.004).
Discussion
Population characteristics
The rate of 100% stylopization in the 1st sampling phase is similar to the high rates found by Straka et al. (Reference Straka, Rezkova, Batelka and Kratochvíl2011) early in the season, but in comparison to the rate found by the latter, also takes into account male A. vaga. The phenomenon of early emergence triggered by stylopization seems to be stronger in this species than in other Andrena species such as Andrena minutula and Andrena milwaukeensis, where according to Straka et al. (Reference Straka, Rezkova, Batelka and Kratochvíl2011) and Balzer and Davis (Reference Balzer and Davis2020), stylopized females emerge at a similar time as unstylopized males. The general advantages of synchronized, early nest emergence for the Stylops are firstly a higher chance for males to find a receptive female within their very short lifetime and secondly more time for the embryogenesis of the larvae that immediately need to reach a host in a recently constructed Andrena brood cell after emerging from their mother (Kinzelbach, Reference Kinzelbach1978; Straka et al., Reference Straka, Rezkova, Batelka and Kratochvíl2011).
The slightly higher prevalence of females among stylopized A. vaga that was found here is congruent with findings of Pierce (Reference Pierce1909). If this is not an effect of the sampling period (stylopized males might emerge later than stylopized females), a possible explanation might be that male hosts die more often due to stylopization during hibernation because of their smaller size as suggested by Straka et al. (Reference Straka, Rezkova, Batelka and Kratochvíl2011). The current study, however, found that male A. vaga more often host male Stylops while at the same time, the negative impact on bee head width tended to be stronger with male Stylops at least in female hosts.
The apparent preference of female Stylops for female hosts and preference of male Stylops for male hosts seems advantageous for the parasite. This might be explained by the fact that Andrena females live longer than males (Westrich, Reference Westrich2019) and therefore allow Stylops females a longer time for offspring production while for male Stylops, which emerge right away, the longevity of their host is less crucial. Additionally, an infestation of a single host with both female and male Stylops may be unfavourable for the female Stylops as the host might die from desiccation or secondary infections after male emergence which leaves behind an open wound in the bee's abdomen (Beani et al., Reference Beani, Dallai, Mercati, Cappa, Giusti and Manfredini2011). Another advantage is the presumably higher egg production possible for Stylops females in heavier female hosts. However, looking at the minute size and low probability of host-finding connected to phoretic transport of the Stylops primary larvae, it seems unlikely for them to refuse invading an egg of the unpreferred sex even if recognition of the latter seems possible due to the presence of olfactory pits (Pohl and Beutel, Reference Pohl and Beutel2019). Brandenburg (Reference Brandenburg1953) doubted that female Stylops are even able to reproduce at all when parasitizing Andrena males. However, since even females of A. vaga are reported to live only for ~2 weeks in the wild (Paxton, Reference Paxton1991; Straka et al., Reference Straka, Černá, Macháčková, Zemenová and Keil2014), the duration of embryogenesis in S. ater and other central European Stylops species must be distinctly shorter than that found in the investigated American species (Linsley and MacSwain, Reference Linsley and MacSwain1957; Balzer and Davis, Reference Balzer and Davis2020), unless Stylops can prolong the lifespan of their hosts similar to other Strepsiptera genera (Kathirithamby, Reference Kathirithamby1977; Beani et al., Reference Beani, Dallai, Cappa, Manfredini, Zaccaroni, Lorenzi and Mercati2021). A finding seemingly contradicting the latter is the near absence of stylopized A. vaga even on the 1st day of the 2nd sampling phase in the current study, which might also be a result of an aberrant daytime behaviour of stylopized bees later in the season as described by Ulrich (Reference Ulrich1956).
The rate of around 10% pluristylopization is approximately twice as high as the one found for A. milwaukeensis by Balzer and Davis (Reference Balzer and Davis2020) who only recorded female Stylops. This rate is most likely positively correlated with the general prevalence of Stylops in their host population and thereby subject to periodical fluctuations as found by Jones et al. (Reference Jones, Williams and Jones1980).
In the current study, the strongly prevailing extrusion position between the 4th and 5th tergite was mostly only deviated from when several Stylops had to share the space in the host's abdomen and displaced each other from favourably extrusion positions. In the case of triple stylopization, apart from 2 bees, the 3rd Stylops was always situated in the middle in a space-saving way, supported by the other 2 like Balzer and Davis (Reference Balzer and Davis2020) documented. The observed slight preference of each Stylops sex for 1 side of the abdomen to extrude from is surprising and was not found by Balzer and Davis (Reference Balzer and Davis2020) who had a smaller sample size. Since all organs in the bee abdomen are pairwise or centrally located (Carreck et al., Reference Carreck, Andree, Brent, Cox-Foster, Dade, Ellis, Hatjina and van Englesdorp2013), this preference of Stylops for 1 side can only be caused by a position of the midgut that could be laterally shifted by default or must have other non-organic reasons.
The results from this population characterization confirmed the peculiarity of S. ater within its genus regarding host-manipulation and raised new questions about reasons for preferences in host sex and parasitization position.
Insights into infestation mode
The observation of Stylops primary larvae invading a host egg is remarkable and has only been reported for an American Stylops species parasitizing Panurginus melanocephalus Cockerell, 1926 (Linsley and MacSwain, Reference Linsley and MacSwain1957). The same behaviour was also documented for the Strepsiptera genus Pseudoxenos in the egg of a solitary wasp by Maeta et al. (Reference Maeta, Gôukon, Kitamura and Miyanaga2001). This confirms the hypothesized morphological adaptation of the head of Stylops primary larvae to egg adhesion and penetration (Pohl and Beutel, Reference Pohl and Beutel2019). Given that live host larvae were found in cells, it seems unlikely that the motionless Stylops might have died, from stress caused by excavation. They might have died from the exhaustion in penetrating the chorion as Maeta et al. (Reference Maeta, Gôukon, Kitamura and Miyanaga2001) observed in Pseudoxenos, were actually alive and only resting before their 1st moult like the Xenos primary larvae documented by Manfredini et al. (Reference Manfredini, Giusti, Beani and Dallai2007). Alternatively, only the 1st instar's exuviae were observed as by Linsley and MacSwain (Reference Linsley and MacSwain1957) and the secondary larvae were not found inside the eggs due to their small size and pale coloration similar to the host embryo.
The lack of further Stylops primary larvae in the pollen provision of brood cells containing stylopized A. vaga eggs is surprising and indicates a smaller risk of dying for the minute primary larvae in the final step of host-seeking within the brood cell than previously expected. The complete absence of primary larvae in pollen loads of unstylopized A. vaga is surprising, considering the high prevalence of S. ater at the sampling sites and the high number of larvae produced by female Stylops (Jones et al., Reference Jones, Williams and Jones1980). Stylops primary larvae were reported to be released asynchronously (Balzer and Davis, Reference Balzer and Davis2021) and in the current study, pollen provisions were collected within a period of around 3 weeks where primary larvae were found inside mature host eggs. Still, it is possible that the peak of prevalence of Stylops primary larvae on blossoms visited by foraging bees was missed. Another possible explanation is that the primary larvae mostly travelled within the bee's crop as Linsley and MacSwain (Reference Linsley and MacSwain1957) observed and not in the hair.
By examining the brood cells of A. vaga, the peculiar host invasion mode of S. ater primary larvae was confirmed and documented for the first time. Regarding the mode of phoresy for this host–parasite system, at least travelling of numerous primary larvae in the pollen load of provisioning bees within the period of host invasion could not be confirmed.
High conservation of considered genes
The high uniformity of sequences in COI, H3 and 18S, even between populations from different cities and countries, is congruent with the findings of Jůzová et al. (Reference Jůzová, Nakase and Straka2015) and represents a high conservation of the genes and/or high genetic exchange between A. vaga aggregations (Exeler et al., Reference Exeler, Kratochwil and Hochkirch2008). The single-base substitution found in the S. ater male from the site MD has to be interpreted as a spontaneous mutation. The high variability of COI in the Strepsipteran Xenos vesparum, even within 1 host nest (Vannini et al., Reference Vannini, Carapelli, Frati and Beani2008), opposed to the homogeneity in the examined S. ater, is remarkable. The proposed bivoltine life cycle of X. vesparum (Manfredini et al., Reference Manfredini, Giusti, Beani and Dallai2007) and the resulting shorter generation time might play a role here (Thomas et al., Reference Thomas, Welch, Lanfear and Bromham2010).
The examination of genetic variability in S. ater confirms results from studies in other countries and herein endorses that relatively closely related species with different ecology can have different levels of genetic variability within 1 gene locus.
Size variation of Stylops females
The comparatively lower cephalothorax width of Stylops females in male A. vaga is most likely due to the smaller weight of the host, which is in turn caused by a smaller brood cell provision (Danforth, Reference Danforth1990). The decrease in cephalothorax width in Stylops females in bistylopized hosts might be the consequence of intra-host competition for nutrients between the 2 parasites. Unexpectedly, female Stylops cephalothoraxes are not significantly narrower co-parasitizing with a male Stylops compared to a 2nd female, even though the presumably higher energy consumption of males has been shown here as well as in Beani et al. (Reference Beani, Dallai, Mercati, Cappa, Giusti and Manfredini2011). However, if the total egg production is positively correlated with body size/cephalothorax width as in other insects (Jervis and Ferns, Reference Jervis and Ferns2004), reproductive success of female Stylops decreases in male or pluristylopized hosts.
The effect of host size and sex on Stylops size, which had rarely been mentioned in earlier studies, was proven here and might play a role in preferences in host-seeking due to effects on the parasite's fitness.
Impact of stylopization on host morphology
The documented ovary underdevelopment of 2 different degrees is in line with the findings of Brandenburg (Reference Brandenburg1953), even though the unstylopized bees examined here are arguably older than the stylopized ones and their ovaries and eggs had more time to develop. The latter hypothesized the stronger ovary suppression in bees with female Stylops to be a result of a higher protein demand for egg production. Brandenburg (Reference Brandenburg1953) observed that the eggs in ovaries of bees with male Stylops, larger at emergence already, continued growth after the parasite emerges and concluded that the concerned bees can most likely reproduce. Therefore, female stylopization might more fatal in terms of fitness for female hosts than male stylopization. For male hosts it is the other way around: they seem to stay fertile (Salt, Reference Salt1927) despite stylopization but the exit wound resulting from male stylopization likely poses a risk of infection and desiccation. The ovary reduction is linked to a decrease in size of the juvenile hormone-producing corpora allata as Brandenburg (Reference Brandenburg1953) and Strambi et al. (Reference Strambi, Girardie and Grasse1973) showed experimentally.
The trend for a reduced head width in stylopized A. vaga females compared to unstylopized ones may represent a loss of nutrients during development. That this effect was stronger in bees with male Stylops is congruent with the findings of Beani et al. (Reference Beani, Dallai, Mercati, Cappa, Giusti and Manfredini2011) who showed that in Polistes, the lipid stores of wasps hosting male Xenos were smaller than in those hosting females. A plausible reason might be the higher energy demand for complete metamorphosis of male Strepsiptera compared to the larviform females (Lease and Wolf, Reference Lease and Wolf2011). The observed higher head width/ITD ratio in A. vaga with female Stylops could be interpreted as a 1st masculinized feature, even though it is not as obvious as the following one. However, it is contrary to the findings of Salt (Reference Salt1927) who reported the heads of some stylopized Andrena salictaria Robertson, 1905 to be ‘ridiculously small’ but also stated that this effect is only measurable in male bees.
The hind legs of stylopized females are clearly overall masculinized in shape and size in relation to their body. A stronger impact of male Stylops in this aspect, as reported in Pierce (Reference Pierce1909), was not found. Since at least A. vaga females with a male Stylops are suspected to be able to reproduce after the emergence of their parasite, this permanent alteration of the shape and vestiture of their legs, which are associated with provisioning their offspring with pollen, may lead to a further decrease of their reproductive fitness.
The denser and longer tergal hair of stylopized A. vaga is very conspicuous and even visible on photographs. The fact that this effect was strongest near the cephalothorax of female Stylops might be a hint towards the masculinization being a substance-induced host manipulation instead of a mere by-product of nutrient depletion or spatial gonad restriction. Whether it is only a side effect of other aspects of host manipulation or is of advantage for Stylops remains unclear. Pohl et al. (Reference Pohl, Gorb and Gorb2020) tested the hypothesis that it represents a parasitic manipulation that facilitates adhesion of the male Stylops to the host's body during copulation but did not find a difference in traction force of male tarsi to smooth and hairy surfaces. They suggested that the increase in hairiness could still be a parasitic manipulation that facilitates phoretic transport of the Stylops primary larvae to flowers.
By examining different traits of stylopized A. vaga females and comparing them to unstylopized individuals, morphological alterations of different suspected origins were found. It seems plausible that the main aim of Stylops manipulation of their Andrena host is a shift in emergence to gain time for embryogenesis by exploitation of the pre-existing protandry in this genus and that all morphological alterations are merely a by-product (with a potential advantage for the parasite) of their impact on the host's endocrine system (Strambi et al., Reference Strambi, Girardie and Grasse1973; Straka et al., Reference Straka, Rezkova, Batelka and Kratochvíl2011).
To resolve some of the addressed issues concerning population structure and host manipulation in S. ater and its host A. vaga, future studies should perform a different type of sampling that reduces various sources of bias by using emergence traps over a longer time span. Host marking experiments and successive examination of Stylops egg development may answer questions whether female parasites have a life-prolonging effect on its host, whether male bees live long enough to ensure offspring dispersal for Stylops females, and whether the lifespan of bees formerly hosting a male Stylops is reduced due to emergence wounding. Biochemical studies focusing on hormone levels of the developmental stages of the host and its parasite might shed light on the exact ways of host manipulation that lead to all of the before mentioned effects and thereby add to a better understanding of complex host–parasite interactions.
Supplementary material
The supplementary material for this article can be found at https://doi.org/10.1017/S0031182023000483.
Acknowledgements
We thank Miguel Vences (Zoological Institute, Technische Universität Braunschweig) for supervision and help in planning the genetic analysis of samples; and Robin Schmidt for support in the genetic laboratory and data analysis as well as for granting permission to use his macro photography. We want to thank everyone who helped with sampling of Andrena specimens, namely Jana Deierling, Harmen P. Hendriksma, Felix Klaus and Dennis Leer (all Julius Kühn Institute) as well as Ira Waldow (Ottoneum Kassel). We are grateful for the permission to sample and excavate bees and their brood cells, granted by the local (UNB Braunschweig) and regional nature conservation agency (NLWKN).
Author's contribution
M. H., He. G. and S. E. conceived and designed the study. M. H., Ha. G. and He. G. conducted sampling. M. H. performed data gathering as well as formal and statistical analyses, and wrote the 1st draft of the article. Ha. G. and He. G. provided material, methodology and resources. All authors were involved in review and editing of the article.
Financial support
This study received financial support from the BeesUp project, which is funded by the Federal Agency for Nature Conservation (within the Federal Biological Diversity Programme) with funds from the Federal Ministry for the Environment, Nature Conservation, Nuclear Safety and Consumer Protection (Grant Number: 3520685A29); and the Zoological Institute of the Technische Universität Braunschweig. We acknowledge support by the Open Access Publication Funds of Technische Universität Braunschweig.
Conflict of interest
The authors declare there is no conflict of interest.