Preterm birth is a significant global issue, affecting approximately one in ten births worldwide( 1 ). Late-onset neonatal sepsis (LOS), defined as sepsis >72 h after birth, is predominantly a disease of preterm infants. The prevalence of LOS increases from 2 % of those born at 32–<37 weeks (moderately preterm infants) to 10–20 % of infants born at 28–32 weeks (very preterm infants) and 30–40 % of infants born at <28 weeks (extremely preterm)( Reference Stoll, Hansen and Fanaroff 2 , Reference Camacho-Gonzalez, Spearman and Stoll 3 ). It was proposed by Berg( Reference Berg 4 ) that bacterial translocation from the gut occurs regularly in healthy individuals, but that infections such as sepsis can occur through failures of host defences, which allow bacterial overgrowth (dysbiosis), invasion of host tissues through decreased barrier function and poor immunological responses to the invading microbes. Emerging evidence suggests that LOS pathogens may translocate from the gastrointestinal tract (GI) in preterm infants( Reference Soeorg, Huik and Parm 5 ).
Consumption of human milk provides passive immunity to the newborn GI tract through a large number of soluble and cellular immune components. Milk contains antimicrobial proteins and peptides (AMPs) including lactoferrin (LF), lysozyme (LZ) and defensins( Reference Armogida, Yannaras and Melton 6 , Reference Mehta and Petrova 7 ), which modulate the gut microbiome( Reference Salzman, Hung and Haribhai 8 ). In addition to antimicrobial activity, breast milk may contribute to maintenance of the barrier function of the GI tract, through growth factors and cytokines( Reference Hawkes, Bryan and James 9 ), or to the defence of the GI epithelium through soluble pattern recognition receptors (PRR) such as secretory IgA (sIgA) and soluble CD14 receptor (sCD14), which may prevent bacterial attachment to enteric tissues through steric hindrance( Reference Berg 4 , Reference Vidal, Labeta and Schiffrin 10 , Reference Mantis, Rol and Corthesy 11 ). Low milk concentrations of these factors could be a risk factor for the development of LOS in preterm infants, but the levels of many of these factors in human term and preterm milk are poorly characterised. A dose-dependent relationship between higher breast milk consumption and lower incidence of LOS has been demonstrated( Reference Patel, Johnson and Engstrom 12 ), but not all preterm infants who consume sufficient amounts of breast milk are protected from LOS. Work from our laboratory has described how the viability of pathogens that cause LOS is differentially affected by the varied concentrations of antimicrobial proteins found in milk( Reference Trend, Strunk and Hibbert 13 ) and the correlation between gestation length and cord plasma levels of AMPs( Reference Strunk, Doherty and Richmond 14 ). This raises the question of whether preterm mothers’ milk could be deficient in protective factors compared with term mothers’ milk, contributing to the increased risk of LOS in the preterm population.
Milk composition is variable and may be influenced by a number of factors. Major temporal changes occur, which result in large differences between the composition of colostrum, the early milk rich in immune proteins and cells, to transitional milk, and finally mature milk, a fluid of mostly stable composition( Reference Ballard and Morrow 15 ). Interestingly, the concentration of protein and immune factors such as sIgA and leucocytes are reported to be altered by maternal age, mode of delivery, the volume of milk produced, smoking, BMI, parity and maternal infection( Reference Dizdar, Sari and Degirmencioglu 16 – Reference Hassiotou, Hepworth and Metzger 20 ). Many of these maternal parameters are also risk factors associated with preterm birth( Reference Goldenberg, Culhane and Iams 21 ), and the link between preterm birth and milk composition therefore warrants further investigation.
Several studies have investigated the differences between preterm and term breast milk sampled in the 1st month postpartum, including levels of AMPs, soluble PRR and cytokines( Reference Armogida, Yannaras and Melton 6 , Reference Mehta and Petrova 7 , Reference Ronayne de Ferrer, Baroni and Sambucetti 22 – Reference Broadhurst, Beddis and Black 39 ). However, there are limited data on mothers of extremely preterm and very preterm infants, the infants most at risk of LOS, and no studies have compared AMPs, PRR and cytokines in preterm and term milk through the stages of lactation related to the times when LOS is most likely to occur. In addition, because of major methodological differences between previous studies, comparisons of the outcomes of these investigations, which are sometimes conflicting, are difficult to make. Consequently, the longitudinal immune composition of preterm milk remains insufficiently characterised, and it is unclear whether preterm milk is different from term milk as lactation progresses, and the circumstances that may lead to altered concentrations of immune proteins in these individuals are also unclear.
The aim of this study was to measure and compare the levels of selected AMPs, PRR and cytokines longitudinally between colostrum, transitional milk and mature breast milk, in extremely preterm, very preterm, moderately preterm and term human milk. We hypothesised that preterm mothers’ groups would have deficient concentrations of these immunological factors in their milk compared with term mothers in the early stages of lactation, which coincides with the period of highest LOS incidence for preterm infants.
Methods
Milk sampling, processing and storage
This study was conducted according to the guidelines laid down in the Declaration of Helsinki, and all procedures involving human subjects/patients were approved by the Women and Newborns Health Service Ethics Committee. Written informed consent was obtained from all patients. Mothers giving birth to live infants at King Edward Memorial Hospital between 22 and 41 weeks of gestational age were approached to participate in the study, provided approval was given by the medical and nursing staff caring for the mother and infant at the time, so that participation in research did not interfere with feeding of newborns. Potential participants were excluded if the mother was <18 years of age at the time of consent, not intending to breast-feed, if there were major congenital abnormalities in the mother or infant, or significant clinically relevant immune-related illnesses (such as autoimmune disease including diabetes mellitus or a major identified heritable immune deficiency).
In all, sixty participants giving birth at King Edward Memorial Hospital (Perth, Western Australia) were recruited into one of four WHO-defined infant gestational age groups( 1 ): extremely preterm (<28 weeks of gestation, n 15), very preterm (28–<32 weeks of gestation, n 15), moderately preterm (32–<37 weeks of gestation, n 15) and term (37–41 weeks of gestation, n 15). Milk was collected on days 2–5 (colostrum; ≤3·5 ml), days 8–12 (transitional milk; ≤7 ml) and days 26–30 (mature milk; ≤7 ml) postpartum. Milk was expressed by the mother for collection of research samples according to her regular practice and varied between individuals based on their current feeding practice (either manual expression or breast pump, from either breast, one-off expression). Mothers with infants in the special care nursery or with a preference for bottle-feeding expressed full feeds from which a small research sample was taken, whereas mothers directly breast-feeding expressed only sufficient milk to provide a sample for research. To adjust for this in our models, we recorded the total amount of milk expressed when milk was sampled, where the information was available.
The sampling time points were selected based on the work of Castellote et al. ( Reference Castellote, Casillas and Ramirez-Santana 36 ). On the basis of pilot data generated in our laboratory showing HBD1 concentration differences between extremely preterm and very preterm mothers’ milk, a sample size of ten mothers per gestation group was determined to be sufficient to detect differences of 0·5 sd with 89 % power, while conducting the regression analysis at α=0·05 significance level and simultaneously adjusting for six covariates (R 2 of 0·2 between the covariates and HBD1; Power and Sample Size Calculation Program for Windows, version 2011). The sample size was increased to fifteen subjects per groups to allow for loss to follow-up and missing samples if participants were affected by poor milk production.
Expressed milk was transferred to a sterile 15-ml conical tube with a sterile Pasteur pipette and stored at 4°C until transported to the research institution (Princess Margaret Hospital, Perth, Australia) in an insulated container with an ice brick (median storage time 8 h). Milk cells were separated from the liquid fraction by centrifugation at 600 g for 15 min at 4°C, and the cell-free milk supernatant was transferred to a fresh container using a sterile Pasteur pipette. The skimmed aqueous fraction of milk was collected by centrifugation at 6000 g for 10 min at 4°C and puncturing the container at the base with a 19 G needle, and by using a syringe to extract the liquid fraction below the fat layer. Concentrated EDTA-free protease inhibitor (Sigma-Aldrich) was diluted one part in fifty into skimmed milk, and aliquots were stored in micronic tubes at −80°C until analysis. For two milk samples, frozen (−20°C), but not fresh, milk was available. These samples were excluded from the final analysis.
The clinical data collected for mothers participating in the study were based on known factors that are associated with premature birth( Reference Velona, Abbiati and Beretta 23 ). Details of the time, date and volume of milk expressed were recorded by participants or research staff, and were used to calculate the number of days postpartum the milk was donated (day of birth=day 1), as well as the length of storage for the sample before processing. When the date but not time of collection was recorded (n 7), the time of collection was assigned 12.00 hours. Details of any current illness and concurrent medications were requested on a separate study questionnaire, or extracted from medical records during hospitalisation. Placentae from births occurring before 30 weeks of gestation at the research hospital were routinely sent for histological review and microbiological examination to determine the presence of chorioamnionitis. Two extremely preterm infants were transferred from other hospitals following birth, and the placentae were not reviewed. Placentae from births after 30 weeks of gestation were reviewed at the discretion of the treating physician in other gestational age groups (4/15 term, 9/15 moderately preterm and 6/9 at 30–32 weeks of gestation). Maternal medical records were reviewed, and following hospital discharge (usually by day 5 postpartum) self-reported infection data were collected. A bacterial infection was defined during inpatient hospitalisation as either histological or microbiological evidence of chorioamnionitis or positive bacterial cultures from pathology specimens tested. After hospital discharge, a bacterial infection was defined as treatment of an infection diagnosed by a physician with antibiotics for symptoms of infection. Donors with infections were classified as having a current infection if the infection occurred within seven days of milk sampling. Where data were available, alcohol and tobacco use were self-reported during prenatal medical reviews and recorded in medical records.
ELISA for soluble immune mediator detection
AMP-LF and human β defensins 1 and 2 (HBD1, HBD2) concentrations were measured using sandwich ELISA, and human α defensin 5 (HD5) was tested using an indirect ELISA method, developed in-house, according to the method previously described( Reference Trend, Strunk and Hibbert 13 ). sCD14 was measured using a sandwich ELISA developed in-house, using rat anti-human sCD14 (551403; BD Biosciences) and biotinylated mouse anti-human sCD14 (551405; BD Biosciences) to detect recombinant sCD14 standard (30R-AS047; Fitzgerald Industries International) or sCD14 in breast milk samples. sIgA was measured according to a modified version of the method described by Hassiotou et al. ( Reference Hassiotou, Hepworth and Metzger 20 ), modified to use smaller (100 μl) well volumes. The limits of detection for these assays were as follows: LF and HD5 (78 pg/ml), HBD1, sCD14 (31 pg/ml), sIgA (3906 pg/ml) and HBD2 (3125 pg/ml). Transforming growth factor-β2 (TGF-β2) ELISA kits were purchased from eBioscience, and samples were tested according to the manufacturer’s instructions. The limit of detection for this assay was 31 pg/ml.
All in-house ELISA assays were carried out in 2 % bovine serum albumin (BSA)-PBS buffer containing 0·5 % Tween-20, and washing was performed in 0·1 % Tween-20 in PBS. Avidin-HRP (eBioscience) was added to biotinylated antibodies for detection, and ELISA were developed using 3,3',5,5'-tetramethylbenzidine substrate (eBioscience), and the reaction was stopped using 1 m-orthophosphoric acid. Absorbance of light at 450-nm wavelength was measured using a spectrophotometer.
Concentrations of molecules of interest in samples were interpolated from the standard curves, fitted with five-parameter logistical curves generated using GraphPad Prism software (La Jolla, www.graphpad.com). Any test samples with absorbance readings that fell outside the standard range were repeated at either a lower or a higher dilution, respectively. Test samples that fell below the standard curve at the lowest dilution achievable in assay buffer (1:1) were assigned the value of the lowest standard (i.e. half the lowest standard×2 (dilution factor)). Inter-assay CV was <5 % for all assays.
Lysozyme measurement
LZ concentration in milk was determined by a turbidity assay modified from Selsted & Martinez( Reference Selsted and Martinez 40 ). The assay measured the loss of turbidity because of the direct lysis of Micrococcus lysodeikticus. Briefly, all standards and skimmed milk samples were diluted with 0·1 m-Na2HPO4–1·1 mm-citric acid (pH 5·8) buffer. Hen egg white LZ (EC 3.2.1.17; Sigma) was used as a standard at a concentration range from 0·75 to 12·5 μg/ml. Milk samples were diluted 10-fold before measurement. A quantity of 25 ml of standard or diluted skimmed milk samples were placed into the wells of a ninety-six-well plate (Greiner Bio One), along with 175 μl of M. lysodeikticus suspension (0·075 % w/v, ATCC no. 4698; Sigma). The plate was immediately incubated with shaking at room temperature (RT) for 1 h, and then the absorbance of light at 450 nm was recorded. The determination limit was 0·007 g/l and the inter-assay CV was 19 %.
Protein detection
Total protein was quantified using the bicinchoninic acid (BCA) assay (Thermo Fisher Scientific), with a standard curve generated from a breast milk sample with protein content determined by the Kjeldahl method( Reference Mitoulas, Kent and Cox 41 ).
Multiplex cytokine detection of cytokines
IL-6, IL-10, IL-13, TNF-α and interferon-γ (IFN-γ) were measured using a magnetic bead-based multiplex assay, developed in-house, according to a modified method previously described( Reference D’Vaz, Meldrum and Dunstan 42 ). Briefly, milk samples were diluted 1:1 in a 2 % BSA-PBS buffer containing 0·5 % Tween-20, and duplicate standards containing the cytokines of interest were prepared with concentrations ranging from 3 to 30 000 pg/ml of each cytokine. IL-6, IL-10 and IFN-γ standards were purchased from BD Biosciences, and IL-13 and TNF-α standards were purchased from R&D Systems. Quality controls consisting of known concentrations of standards were run with each plate to measure inter-assay variability. All antibodies were purchased from BD Biosciences. A bead mixture containing 2000 beads each of rat anti-human IL-6 (clone MQ2-13A5, cat. 554543), rat anti-human IL-10 (clone JES3-9D7, cat. 554495), rat anti-human IL-13 (clone JES10-5A2, cat. 554570), mouse anti-human TNF-α (clone MAb1, cat. 551220) and mouse anti-human IFN-γ (clone NIB42, cat. 551221) conjugated to MagPlex XMap Magnetic beads (Luminex Corp.) was prepared in a BSA-PBS buffer, and then 50 μl of this preparation was incubated with 50 μl of each diluted sample in a ninety-six-well plate for 45 min, with shaking at 4000 rpm. All incubations were carried out at RT and protected from light. After incubation, beads were washed three times by placing the plate on top of a magnetic plate for 1 min (LifeSep; Dexter Magnetic Technologies Inc.), removing the supernatant and then replacing with 100 μl of Multiplex washing buffer containing 1 % BSA-PBS with 0·25 % Tween-20 and 0·001 % sodium azide. Beads were incubated for 30 min at RT with 100 μl of secondary antibody mixture, containing biotin-conjugated rat anti-human IL-6 (clone MQ2-39C3, cat. 554546), rat anti-human IL-10 (clone JES3-12G8, cat. 554499), mouse anti-human IL-13 (clone B69-2, cat. 555054), mouse anti-human TNF-α (clone MAb11, cat. 554511) and mouse anti-human IFN-γ (clone 4S.B3, cat. 554550). After washing as previously described, streptavidin-R-phycoerythrin (s-866; Invitrogen) was incubated with beads for 30 min at RT. After a final washing step, samples were resuspended in wash buffer and mean fluorescence intensity was measured for 100 beads in each well in the bead region of interest, using a Bioplex 2000 instrument (Bio-Rad), with doublet discrimination gates set from 6500 to 20 000, using Bioplex manager software (Bio-Rad). The lower limit of detection of the standard curve was 3 pg/ml for IL-10, IL-6 and IL-13, and 30 pg/ml for TNF-α and IFN-γ. The upper limit of detection was 10 000 pg/ml for IL-10, TNF-α and IFN-γ, and 30 000 pg/ml for IL-6 and IL-13. Analysis of curves and interpolation of sample values was performed in the Bioplex manager software using five-parameter curve fit. Standards were excluded if the percentage of observed compared with expected concentration did not fall between 80 and 120 %, and sample values falling below the limit of detection were manually assigned a value of half the lowest level detectable for all cytokines.
Statistical analyses
Univariate analyses
Most continuous data were not normally distributed, as assessed by a Shapiro–Wilk normality test. Therefore, non-parametric methods were used for the univariate analyses. The gestational age of infants and maternal age of the donors recruited to the study, separated into the four gestational age groups, were compared using Kruskal–Wallis tests. The distributions of the number of samples collected or maternal characteristics such as smoking between gestational age groups and milk groups (colostrum, transitional milk and mature milk) were tested using two-sided Fisher’s exact tests.
The volume of milk expressed, and the concentrations of immune proteins and peptides in the four different gestational age groups (by both absolute concentrations and data as a percentage of the total protein content) at each sampling time point, were compared by Kruskal–Wallis tests and, if ANOVA results were statistically significant, individual comparisons were made between preterm groups compared with the term reference group using Dunn’s post-test for multiple comparisons. The temporal changes in milk from colostrum, transitional milk and mature milk in the same donor were compared using non-parametric Friedman’s tests, followed by pair-wise multiple comparisons when appropriate.
Associations between continuous variables including gestation period, concentrations of milk factors (total protein, concentrations of AMPs, PRR, growth factors and cytokines measured) and volume of milk expressed were evaluated using Spearman’s correlation coefficient (ρ). Comparisons between these continuous outcomes were made between individuals with and without infections at the same sampling time point (colostrum, transitional milk or mature milk) using Mann–Whitney U tests. Differences in the number of samples with measurable cytokines between gestational age groups were assessed using Fisher’s exact tests.
Multivariable analyses
Regression analyses were used to assess repeated measures in individuals’ breast milk longitudinally through the 1st month of lactation for continuous (linear regression) and binary outcomes (logistic regression), respectively, and comparisons were made both within and between individuals providing breast milk. A generalised estimating equations (GEE) approach was implemented to account for correlation between repeated samples from the same individual, reducing bias in estimates that may occur using other regression methods( Reference Ballinger 43 ). Covariate imputations were not implemented in the GEE analysis, with the individual milk samples automatically excluded list-wise from the analyses if data were missing on any of the covariates included in the model. The potential effects of missing data were assessed in the multivariable analyses by comparing the number of samples in each gestational age group after removing those with insufficient data using a χ 2 test.
Analyses of milk total protein and immune protein concentrations were adjusted for six covariates that were hypothesised to affect milk or preterm infant outcomes, based on factors both associated with preterm labour( Reference Goldenberg, Culhane and Iams 21 ) and that possibly affect milk composition, based on previously published data. These included gestational age categories( Reference Mehta and Petrova 7 , Reference Velona, Abbiati and Beretta 23 – Reference Goldman, Garza and Nichols 26 , Reference Gross, Geller and Tomarelli 31 , Reference Castellote, Casillas and Ramirez-Santana 36 ), lactation stage (colostrum, transitional milk or mature milk)( Reference Mehta and Petrova 7 , Reference Lemay, Ballard and Hughes 44 ), mode of delivery( Reference Mehta and Petrova 7 , Reference Wang, Cao and Li 30 ), parity( Reference Mehta and Petrova 7 , Reference Montagne, Culliere and Mole 24 ) (multiparous compared to primiparous mothers), maternal bacterial infection( Reference Hassiotou, Hepworth and Metzger 20 ) and the volume of milk expressed at the time of sample collection( Reference Bishara, Dunn and Merko 19 ). A five-parameter model was used to study the mean volume of milk expressed with the other described variables. Smoking during pregnancy and maternal obesity were not adjusted for in the model, although previous research has implicated a potential role in breast milk composition( Reference Bachour, Yafawi and Jaber 18 ), because there was no quantifiable effect in the term comparison group, and because data on maternal BMI were not available, respectively.
To approximate a normal distribution, natural log-transformations were applied to milk variables where outcomes were measurable in all samples (total protein, LF, LZ, sIgA, sCD14, HBD1, TGF-β2 and volume of milk expressed) to normalise the data for GEE analyses. Outcome variables in which significant proportions of the samples contained levels below the limit of detection were transformed into categories of ‘not measurable’ or ‘measurable’, and examined using a binary model in the same manner (HD5, IL-6, IL-10, IL-13, TNF-α and IFN-γ), with ‘not measurable’ as the reference category. The within-subject variable for repeated measurements in the same individual was type of milk (colostrum, transitional milk and mature milk). In all cases, term mothers, colostrum, vaginal delivery, primiparous and healthy individuals were considered the baseline status, and other categories were compared with these groups. After analyses, results were reported using β estimates back-transformed by exponentiation together with their 95 % CI, providing regression coefficients for continuous variables and OR for binary models.
All hypotheses were two sided, and P values<0·05 were considered significant, except where individual comparisons were adjusted for Dunn’s or Friedman’s post-tests. Data analyses were performed using the SPSS software (version 21; IBM).
Results
Sample characteristics
In our cohort, the clinical presentation of preterm mothers was different from term, with the complete absence of preterm premature rupture of membranes, chorioamnionitis, known smoking during pregnancy, previous preterm birth, use of reproductive assistance and preeclampsia in term mothers (Table 1). Two extremely preterm infants of mothers on the study developed LOS; however, there were no cases in other groups.
Table 1 Comparison of clinical data for gestational age groups of mothers in the study (Numbers and percentages; mean values and standard deviations)Footnote †

EP, extremely preterm; VP, very preterm; MP, moderately preterm.
* Statistically significant in ANOVA or χ 2 tests (P<0·05).
† Distributions were compared using two-sided Fisher’s exact tests.
Totally, sixty mothers were recruited to the study; the numbers of donors providing milk at each sampling time point are shown in Fig. 1; thirty-seven individuals donated milk at all three time points; three individuals, two from the extremely preterm group and one from the moderately preterm group, did not provide a sample of milk at any sampling point, because of limited milk production, no longer breast-feeding and withdrawing from the study, respectively. The most common reason for missing samples across all GA groups was maternal reporting of low milk production. The total number of milk samples tested was 149. No significant differences between the numbers of samples collected in each gestational age group across the three time points were found (P=0·98), and therefore, with respect to gestation groups, no significant patterns in the individuals with missing data were detected. However, the number of reported maternal infections decreased after the initial sample, and the number was higher at the time of colostrum collection compared with mature milk (P=0·029). The most common type of infection reported was chorioamnionitis at the colostrum collection time point (Table 2).

Fig. 1 Flowchart showing recruitment numbers and actual number of individuals providing milk samples at each time point, by gestational age group.
Table 2 Number of maternal infections recorded around the time of milk collection in study participants providing breast milk

RTI, respiratory tract infection; GI, gastrointestinal infection; UTI, urinary tract infection.
Volume of milk expressed and total protein concentration
The reported total volumes of milk expressed by the donors at the time of study sample collection are shown in Fig. 2. At all time points, very preterm mothers expressed significantly greater volumes of milk than term mothers when donating to the study. Extremely preterm and very preterm mothers expressed increasing volumes of milk at each visit from colostrum to mature milk (from a median of 17·6–60 ml and 40–200 ml, respectively). In moderately preterm mothers, volumes of milk expressed at the time of research sample collection increased to transitional milk but decreased by mature milk collection (medians of 20, 80 and 75 ml, respectively). In term mothers, expressed milk volumes for research samples remained low throughout the study (median volumes of 4·3, 11 and 10·5 ml, respectively). The volumes of milk expressed in all mothers were positively correlated with the number of days postpartum that it was collected (ρ=0·251; P<0·01). The concentration of protein in the milk was negatively correlated with the number of days postpartum the breast milk was collected (ρ=−0·586; P<0·001). The median concentration of protein in all milk samples collected in the 1st month postpartum was 14·0 (range 7·41–38·09) mg/ml, decreasing from median 16·0 mg/ml in colostrum to 12·9 mg/ml in mature milk (Fig. 2(b)). Multiparous mothers expressed larger volumes of milk than primiparous mothers did (Table 3). The reported volume of milk expressed was negatively correlated with the total protein concentration measured in milk samples (ρ=−0·217; P<0·05). There were no differences between gestational age groups in total protein concentration at the same time point.
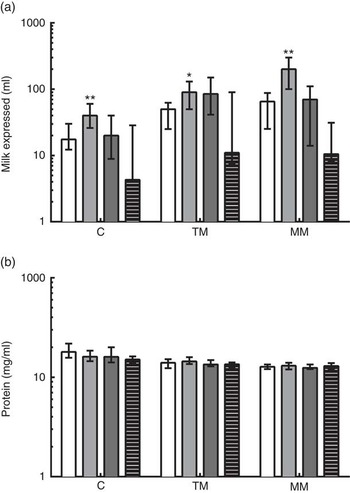
Fig. 2 (a) Reported volumes of milk expressed at time of study sample donation and total protein concentrations measured in preterm and term mothers’ milk, and (b) concentration of protein in milk samples measured by bicinchoninic acid (BCA) assay. Gestational age group is shown on the x-axis. Data are shown as the median values recorded and the interquartile ranges, on a log scale. * Significant (P<0·05) and ** highly significant (P<0·01) differences in preterm groups based on Dunn’s multiple comparison tests, compared with the term reference group. C, colostrum (eight extremely preterm (EP, ), eleven very preterm (VP,
), twelve moderately preterm (MP,
) and twelve term (
) donors, respectively); TM, transitional milk (nine EP, eleven VP, ten preterm and thirteen term donors, respectively); MM, mature milk (eight EP, nine VP, nine preterm and fourteen term donors, respectively).
Table 3 Outcomes of linear regression modelling with repeated measures for continuous variables associated with milk (β-Coefficients and 95 % confidence intervals)Footnote †

MP, moderately preterm mothers (32–<37 weeks of gestation); VP, very preterm mothers (28–<32 weeks of gestation); EP, extremely preterm mothers (<28 weeks of gestation); MM, mature milk (days 26–30); C, colostrum (days 2–5); TM, transitional milk (days 8–12); LF, lactoferrin; LZ, lysozyme; sIgA, secretory IgA; sCD14, soluble CD14 receptor; HBD1 and HBD2, β defensins 1 and 2; TGF-β2, transforming growth factor-β2; GEE, generalised estimating equations.
* Statistically significant variable comparisons: P<0·05.
† Results of GEE modelling of linear (natural log-transformed variables) models, describing exponentiated estimated β-coefficients, 95 % CI and significance for the six covariates simultaneously modelled and the concentrations of molecules measured in milk, or five covariates simultaneously modelled in the analysis of expressed milk volume. Expressed milk volume was measured in units of ml in all cases.
Range of detected immune factor concentrations in milk samples
The most abundant protein measured was LF, at a median concentration of 4·65 (range 0·97–11·24) mg/ml. sIgA, LZ and sCD14 were also relatively abundant at median concentrations of 1·06 (range 0·26–31·81) mg/ml, 91·8 (range 20·7–266·5) μg/ml and 31·2 (range 6·4–91·8) μg/ml, respectively. Together, these proteins made up 60, 43 and 30 % (median) of the total protein content in colostrum, transitional milk and mature milk, respectively.
Defence peptides HBD1 and HBD2 were present in lower quantities compared with the larger proteins, at medians of 138·4 (range 4·85–4475) ng/ml for HBD1 and 379·6 (range 3·13–33 520) ng/ml for HBD2, respectively. Cytokine and growth factor expression in milk was generally low (pg/ml) relative to other molecules, although TGF-β2 was the most abundant at median 12·56 (range 1·83–335·89) ng/ml. Median values of IL-10, IL-13, TNF-α, IFN-γ and HD5 were at the limit of detection, as these molecules were measurable in fewer than half of the breast milk samples (maximum values of these molecules 4383 pg/ml of IL-10, 9545 pg/ml of IL-13, 8746 pg/ml of TNF-α, 18 857 pg/ml of IFN-γ and 3655 pg/ml of HD5). Significant correlations between total protein concentration in milk with levels of LF, sIgA, sCD14, HBD1, HBD2, TGF-β2, IL-6, IL-10, IL-13, TNF-α and IFN-γ were observed (P<0·01).
Effect of lactation stage on immune factor concentration in milk samples
Individual AMPs, PRR and cytokine concentrations in breast milk were significantly different when measured in colostrum, intermediate or mature milk from the same mother, being highest in colostrum and the lowest in mature milk, with the exception of LZ and HD5, which did not significantly change over the sampling period. When expressed as a percentage of the total protein in the breast milk, the relative percentage of all molecules except TGF-β2, IL-10, IL-13 and TNF-α were highest in colostrum, and decreased significantly during the 1st month postpartum (P<0·05, not shown).
Effect of prematurity on milk composition
The median concentration of soluble immunological proteins in breast milk samples in colostrum (n 48), transitional milk (n 55) and mature milk (n 46) are reported for each GA group in Fig. 3–5, showing AMPs, PRR and cytokines, respectively. The median values in each sample group are reported in online Supplementary Table S1. The median concentrations of HBD1 and TGF-β2 in colostrum were significantly higher in extremely preterm than in term breast milk samples at the same collection period (Fig. 3 and 5; P<0·05). As we observed that total protein levels varied and immune content was correlated with total protein content, we also analysed the levels of immune factors as a percentage of the total protein. In colostrum, extremely preterm milk contained significantly higher percentages of HBD1 and TGF-β2 than term milk (mg/mgprotein; P<0·05, not shown). In transitional milk, the percentage of LF was higher in extremely preterm than very preterm milk (P<0·05), and the absolute concentration of LF followed the same pattern, although this was not statistically significant (P=0·058). In addition, the percentages that IL-10 and TNF-α represented as a fraction of the total protein were significantly lower in very preterm compared with term transitional milk (not shown). In mature milk (days 26–30), there were no significant differences between gestational age groups in the percentages of measured immune proteins as a percentage of total protein.

Fig. 3 Range of concentrations of antimicrobial proteins and peptides measured in breast milk collected in the 1st month postpartum, separated into gestational age categories. Data are shown as medians and interquartile ranges of extremely preterm (EP, ), very preterm (VP,
), moderately preterm (MP,
) and term (
) gestation mothers’ milk. * Statistically significant comparison in Dunn’s post-test comparing preterm groups with the term reference group. C, colostrum (ten EP, thirteen VP, thirteen preterm and twelve term donors, respectively); TM, transitional milk (eleven EP, fifteen VP, fourteen preterm and fifteen term donors, respectively); MM, mature milk (ten EP, twelve VP, nine preterm and fifteen term donors, respectively); LF, lactoferrin; HBD1 and HBD2, β defensins 1 and 2; HD5, α defensin 5.

Fig. 4 Range of concentrations of pattern recognition receptors measured in breast milk collected in the 1st month postpartum, separated into gestational age categories. Data are shown as median and interquartile ranges of extremely preterm (EP, ), very preterm (VP,
), moderately preterm (MP,
) and term (
) gestation mothers’ milk. C, colostrum (ten EP, thirteen VP, thirteen preterm and twelve term donors, respectively); TM, transitional milk (eleven EP, fifteen VP, fourteen preterm and fifteen term donors, respectively); MM, mature milk (ten EP, twelve VP, nine preterm and fifteen term donors, respectively); sIgA, secretory IgA; sCD14, soluble CD14 receptor.

Fig. 5 Range of concentrations of transforming growth factor-β2 (TGF-β2) and percentage of collected samples with detectable cytokines measured in breast milk collected in the 1st month postpartum, separated into gestational age categories. Data for TGF-β2 shown as median and interquartile ranges and other cytokines show the percentage of tested samples containing detectable cytokine in extremely preterm (EP, ), very preterm (VP,
), moderately preterm (MP,
) and term (
) gestation mothers’ milk. * Statistically significant comparison in Dunn’s post-test comparing preterm groups with the term reference group. C, colostrum (ten EP, thirteen VP, thirteen preterm and twelve term donors, respectively); TM, transitional milk (eleven EP, fifteen VP, fourteen preterm and fifteen term donors, respectively); MM, mature milk (ten EP, twelve VP, nine preterm and fifteen term donors, respectively); IFN-γ, interferon-γ.
The concentrations of LZ (ρ=−0·385; P=0·007), HBD1 (ρ=−0·305; P=0·035) and TGF-β2 (ρ=−0·451; P=0·001) in colostrum, and LF (ρ=−0·284; P=0·036), sIgA (ρ=−0·294; P=0·029) and LZ (ρ=−0·332; P=0·013) in transitional milk, were negatively correlated with gestational age. In contrast, LF concentrations in mature milk were positively correlated with gestational age (ρ=0·374; P=0·01), whereas LZ concentration was negatively correlated with gestational age in mature milk (ρ=−0·346; P=0·018).
There were no significant differences in the percentage of samples with measurable cytokines across gestational age groups at any time point (P>0·05 for all tests; Fig. 5).
Maternal infection and immune composition of milk
Most maternal infections were chorioamnionitis or other genitourinary infections, with only two cases of mastitis in the cohort (Table 2). Colostrum concentrations of the antimicrobial peptide HBD2 and the percentage of HBD2 relative to total protein expression were significantly lower in mothers reporting a recent infection (Fig. 6; P<0·05). We did not detect effects of maternal infection for any other measured molecules in colostrum, or in transitional milk or mature milk immune factors.

Fig. 6 Colostrum concentration of β defensin 2 (HBD2) in healthy mothers (n 32) compared with donors with recent bacterial infections (n 16). * P<0·05 in Mann–Whitney U test.
Effects of multiple predictor variables on concentrations of immunological proteins and peptides in milk
The linear and logistic regression models shown in Tables 3 and 4 included data from 126 (of 149 available) milk samples, with data included for all samples collected except for the individual samples in which covariate data were missing on the volume of milk expressed (twenty-three samples). There were no significant differences between the number of samples with missing volume expressed data in each gestational age group (P=0·216). The relative effects of the gestational age group, stage of lactation, mode of delivery, parity, bacterial infection and expressed milk volume on the concentration of immune factors measured across the three stages of lactation are presented in Table 3.
Table 4 Results of logistic regression modelling with repeated measures of variables with binary (measurable/not measurable) outcomes (Odds ratios and 95 % confidence intervals)Footnote †
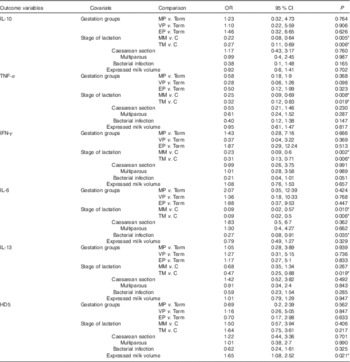
MP, moderately preterm mothers (32–<37 weeks of gestation); VP, very preterm mothers (28–<32 weeks of gestation); EP, extremely preterm mothers (<28 weeks of gestation); MM, mature milk (days 26–30); C, colostrum (days 2–5); TM, transitional milk (days 8–12); IFN-γ, interferon-γ; HD5, α defensin 5.
* Statistically significant differences in odds of detecting cytokine or defensin, found in low concentrations in milk for each of six covariates simultaneously included in the models (P<0·05).
† Expressed milk volume was measured in units of ml in all cases.
The stage of lactation was a major factor affecting the concentration of immune factors in milk (Table 3). Controlling for the relevant covariates, the concentrations of protein, LF, sIgA, sCD14 and HBD2 were significantly lower in transitional milk and mature milk compared with colostrum, and concentrations of HBD1 and TGF-β2 were significantly lower in mature milk but not transitional milk when compared with colostrum. As shown in Table 4, IL-10, IL-6, TNF-α and IFN-γ were more likely to be measurable in colostrum than either transitional or mature milk samples, and IL-13 was more likely to be recovered in colostrum than in transitional milk.
Another major contributing factor to milk composition was the volume of milk expressed by the donor (Table 3). Transitional and mature stages of lactation were associated with higher mean volumes of milk expressed compared with colostrum. Compared with term mothers, we observed increased expression of milk in extremely preterm mothers, very preterm mothers and moderately preterm mothers. Furthermore, controlling for other variables, the mean concentrations of several proteins and peptides showed dependent inverse linear relationships with the volume of milk expressed, including LF, sIgA, sCD14 and HBD1. The volume of milk expressed at sample donation was higher in mothers with measurable HD5. In addition, we observed that the volume of milk expressed at sampling time points was significantly higher in multiparous individuals compared with primiparous mothers.
Importantly, preterm birth resulted in statistically significant increases in the concentrations of immune proteins after adjusting for other variables. Compared with term mothers’ milk, the mean concentration of LZ increased in extremely preterm and very preterm mothers’ breast. In addition, compared with term mothers, the concentration of HBD1 was higher in moderately preterm breast milk, and very preterm mothers had increased sCD14 compared with term mothers’ milk.
Giving birth by caesarean section was associated with higher breast milk levels of HBD2 compared with vaginal delivery (β=6·8; 95 % CI 2·71, 16·96; P<0·001). The presence of (mostly non-mastitic) maternal infections significantly reduced the odds of IL-6 detection, and in IFN-γ a similar effect was observed, but this was not statistically significant. The concentrations of total protein, LF and sCD14 were lower in multiparous mothers after adjusting for other parameters.
Discussion
There are limited data available on the immunological composition of preterm milk, particularly in extremely preterm mothers, although understanding the variation in the levels of protective antimicrobial and immunological factors in preterm breast milk is important to optimise feeding guidelines and to harness their use in future interventions to protect against inflammatory diseases such as sepsis( Reference Neville, Anderson and McManaman 45 ). This study aimed to determine the levels of AMPs, PRR and cytokines in preterm and term mothers’ colostrum, transitional and mature milk, in the context of relevant clinical parameters commonly associated with preterm birth. We found that the concentrations of immune proteins are highest in colostrum and lowest in mature milk, and that the concentrations of some AMPs, PRR and TGF-β2 in colostrum and transitional milk are higher in preterm mothers’ milk than in term mothers’ milk. Although minor effects of maternal infection and caesarean section birth were found for individual factors, these made little difference to milk composition relative to the stage of lactation, the volume of milk expressed, parity and prematurity in multivariate models.
This study confirms previous research that immune factor concentrations during lactation are highest in colostrum and lowest in mature milk( Reference Castellote, Casillas and Ramirez-Santana 36 ). The median concentrations of immune factors in this study decreased more rapidly as lactation progressed than the decrease in total milk protein would predict, revealing initial up-regulation of expression of immune proteins, as demonstrated by the increased relative abundance of mRNA for immune proteins in colostrum by Lemay et al. ( Reference Lemay, Ballard and Hughes 44 ). There is approximately 15 mg/ml protein in milk, and AMPs and PRR make up large amounts of this protein content; for example, LF, sIgA, sCD14 and LZ together make up more than half of the total protein content of colostrum, suggesting that they have a major functional role. The functions of soluble PRR in milk such as sCD14, sIgA and sTLR2( Reference LeBouder, Rey-Nores and Rushmere 46 ) may include steric hindrance in the presence of colonising bacteria by binding and therefore avoiding contact with the neonatal gut epithelium and subsequent bacterial translocation to the blood( Reference Blais, Harrold and Altosaar 47 , Reference Maxson, Jackson and Smith 48 ). We have recently demonstrated that AMPs found in milk can limit the growth of neonatal sepsis-causing bacteria in vitro ( Reference Trend, Strunk and Hibbert 13 ), and Salzman et al. ( Reference Salzman, Hung and Haribhai 8 ) have shown that AMPs regulate the gut microbiota in vivo. Their increased concentration in colostrum suggests a response by the mammary gland epithelium to local mammary or systemic immune signals. During both term and preterm spontaneous labour, inflammatory markers are detectable in placental tissues and systemic circulation, although expression is higher in preterm labour( Reference Yadav, Chaudhari and Warke 49 , Reference Tency 50 ). Interestingly, recently published data from mouse models have demonstrated that toll-like receptor 4 (TLR4) activation through both microbial-dependent and microbial-independent pathways is associated with infection-induced preterm labour and normal labour, respectively( Reference Wahid, Dorian and Yin Chin 51 ). The downstream circulating inflammatory signals resulting from TLR4 activation are also a plausible cause of the generally higher colostrum levels of immune factors after labour, the relatively higher levels of immune factors in infection-induced preterm mothers milk and the low milk production in the postpartum and preterm groups, and could be investigated in future work. If immune signals are up-regulated in colostrum in response to spontaneous labour, then this may explain why we did not observe further up-regulation of immune protein expression during maternal infections in colostrum, when most maternal infections occurred in this study.
Cytokines are present in milk in much lower concentrations than AMPs or PRR. The limited detection of cytokines associated with T helper 1 (Th1) responses (e.g. TNF-α and IFN-γ) in our study are consistent with reported Th2 skewing of leucocyte cytokine responses during pregnancy and in preterm infants( Reference Sykes, MacIntyre and Yap 52 , Reference Currie, Curtis and Strunk 53 ). However, TGF-β2 was found in relatively high concentrations. The levels of TGF-β2 were highest in extremely preterm mothers, and were still higher in the GEE model after adjusting for milk volume, although this was not statistically significant (P=0·08). One previous study of TGF-β2 reported that it was found in higher concentrations in preterm (30–37 weeks of gestation) compared with term (>37 weeks) or very preterm (<30 weeks) colostrum( Reference Castellote, Casillas and Ramirez-Santana 36 ). TGF-β2 is an anti-inflammatory cytokine that suppresses endotoxin-induced cytokine responses of intestinal macrophages from the preterm infant gut in vitro, and protects rat pups from intestinal injury in vivo ( Reference Maheshwari, Kelly and Nicola 54 ). Interestingly, the role of TGF-β2 in the mammary gland may extend beyond immune responses. In mouse models, expression of TGF-β2 is up-regulated in the mammary gland during pregnancy, but expression rapidly decreases during lactation, and it is implicated in the hormonal regulation of initiation of lactation( Reference Robinson, Silberstein and Roberts 55 – Reference Monks 57 ). Therefore, this work suggests that higher levels of TGF-β2 in milk from preterm mothers could be associated with, or a cause of, delayed onset of secretory activation in preterm mothers. The large proportion of samples in this study with levels of other cytokines below the limit of detection may have reduced our capacity to measure differences between populations in our analyses, and it is a limitation of this work.
In contrast to our hypothesis, the concentrations (or percentage of total protein) of some immune proteins in milk were higher in preterm mothers than in their term counterparts; in univariate comparisons for LF, HBD1 and TGF-β2, higher concentrations or percentages of total protein were confined to extremely preterm and very preterm groups. No previous research has compared HBD1 in extremely preterm mothers’ milk to term mothers’ milk. Wang et al. ( Reference Wang, Cao and Li 30 ) found that HBD1 and HBD2 were in higher concentration in mature preterm milk than in term milk, supporting our findings for HBD1. However, Armogida et al. ( Reference Armogida, Yannaras and Melton 6 ) did not detect differences in HBD1 levels between term and preterm mothers. Given that we included extremely preterm mothers, and had a larger sample size, we may have had greater statistical power to detect the differences in the populations compared with previous studies. It should be noted, however, that similar to Armogida et al.( Reference Armogida, Yannaras and Melton 6 ), we also found that HD5 levels were not affected by preterm birth, which suggests that expression levels of HD5 and HBD1 are differentially regulated, or that our limited ability to detect HD5 affected our ability to detect differences in the populations.
After adjusting for other variables in the GEE model, LF was not affected by gestation, but by the volume of milk expressed, supporting a dilution effect of milk, as well as stage of lactation and parity. This demonstrates that the use of multivariate models to differentiate the effects of individual variables in breast milk research may be appropriate in future research, as milk composition is dependent on several variables. In contrast, after accounting for other variables in models, HBD1 concentration was higher in preterm milk, suggesting that there is a true effect of preterm birth on milk composition, independent of the potentially confounding variables that we investigated. For LF, a lack of reproducibility in conclusions about whether preterm milk contains higher or lower relative levels than term milk has hindered progress in understanding and developing the use of this molecule for interventions in LOS( Reference Mehta and Petrova 7 , Reference Velona, Abbiati and Beretta 23 , Reference Dawarkadas, Saha and Mathur 25 ). However, the finding that LF levels are not influenced by gestation after adjusting for milk volume suggests that expression is regulated by a pathway different from the other AMPs we tested in the mammary gland, as, if their expression was linked, we would have also expected to observe higher concentrations of LF in preterm milk. Therefore, mothers who deliver preterm should be able to produce milk with similar LF concentrations to term mothers if similar volumes of milk are expressed.
This research provides the first evidence that the concentration of sCD14, a soluble receptor for a range of pro-inflammatory signals including bacterial lipopolysaccharide( Reference Di Gioia and Zanoni 58 ), is higher in preterm than in term milk. Interestingly, unlike HBD1 and TGF-β2, comparisons between LZ and sCD14 levels in preterm and term milk were not significant in univariate analyses, but emerged as different when accounting for other variables. For LZ, the stage of lactation had no effect on concentrations, and only gestation had a significant effect on levels in the model, whereas sCD14 concentration was also influenced by the stage of lactation and volume of milk expressed. LZ has previously been found in higher concentrations in transitional preterm milk( Reference Montagne, Culliere and Mole 24 , Reference Goldman, Garza and Nichols 26 ), although one study reported lower concentrations in preterm mothers colostrum( Reference Dawarkadas, Saha and Mathur 25 ), and others report no difference between groups( Reference Mehta and Petrova 7 , Reference Velona, Abbiati and Beretta 23 , Reference Hsu, Chen and Lin 27 ). The major differences in definitions of prematurity and methods of milk storage and LZ detection make comparisons between these studies difficult. These data suggest that the GEE model was better at detecting differences between the maternal donor populations than standard univariate comparisons, in which the effect of preterm birth may be masked by other confounding variables. A study by Castellote et al. ( Reference Castellote, Casillas and Ramirez-Santana 36 ) found that sIgA levels in colostrum were significantly lower in those giving birth at <30 weeks of gestation compared with other maternal groups, and that TNF-α, IL-6 and IL-10 levels were significantly higher in preterm compared with very preterm mothers’ colostrum. We did not observe any differences with sIgA levels in preterm and term milk, in concordance with Hsu et al.( Reference Hsu, Chen and Lin 27 ), although several others have reported higher levels in preterm colostrum, transitional milk and mature milk( Reference Mehta and Petrova 7 , Reference Montagne, Culliere and Mole 24 , Reference Araujo, Goncalves and Cornetta Mda 32 , Reference Ronayne de Ferrer, Slobodianik and Lopez 33 ).
The volume of milk expressed at the time of research sample donation was associated with changes to the concentrations of LF, sIgA, sCD14, HBD1 and HD5 in milk in our multivariate models. Higher concentrations of these factors were observed in smaller milk volumes, even when accounting for shorter gestation and earlier stages of lactation, which are associated with reduced milk production( Reference Anderson, Williams and Merkatz 59 , Reference Parker, Sullivan and Krueger 60 ). Unlike fat, the concentration of protein in milk does not appear to be affected by sampling of fore- or hind-milk( Reference Mitoulas, Kent and Cox 41 ), and so this is unlikely to be the cause of the differences observed in our study. However, it is a potential limitation of this work that we could not account for some sampling effects in the multivariate model. Primiparous mothers and those at earlier stages of lactation were more likely to report collection of smaller milk volumes at the time of research sampling. In previous studies, caesarean delivery, older maternal age and lower pumping frequency were reported to be predictors of low milk volume in preterm mothers( Reference Murase, Nommsen-Rivers and Morrow 61 ). We did not observe any effect of caesarean section on milk volume in our model. We did not have volume data for all of our donors, which could have affected our results. In addition, our reported milk volumes are indicative, but not necessarily a true reflection, of the capacity of individuals to produce milk, as mothers had the choice to express a small sample if directly breast-feeding their infant. Therefore, preterm groups in this study actually expressed larger milk volumes than term mothers. It is unclear whether the associations between increasing milk volumes and decreasing immune factor concentrations are causative (as in dilution). Alternatively, the observation may be a result of an extrinsically linked confounding variable; for example, in mastitis, milk output is decreased and expression of immune factors is increased through TLR4 activation( Reference Ingman, Glynn and Hutchinson 62 ). This study demonstrates that the levels of immune molecules in milk are not solely dependent on volume, but supports previous work that demonstrates that milk volume can be a confounding variable when studying milk composition( Reference Gross, Geller and Tomarelli 31 ). However, we did not have access to accurate BMI data, and we could not account for effects of co-occurring variables with preterm birth such as smoking, whose effects could be investigated in future in a study designed to assess this.
It has been suggested that differences between preterm and term mothers’ milk have evolved as a compensatory mechanism, whereby the mammary gland senses the preterm labour and adjusts immune content accordingly to protect the immature newborn( Reference Hsu, Chen and Lin 27 , Reference Pamblanco, Ten and Comin 29 , Reference Araujo, Goncalves and Cornetta Mda 32 , Reference Castellote, Casillas and Ramirez-Santana 36 , Reference Read, Francis and Wallace 38 ). However, as stated by Molinari et al.( Reference Molinari, Casadio and Hartmann 63 ), the selection pressures that would be required for an evolutionary adaptation to occur have only existed since the last century, making adaptation to increase preterm infant survival highly improbable. On the basis of our data, we believe the strongest argument for the cause of the increased levels of immune proteins in preterm milk to be that the proximal causes of preterm labour such as inflammation release signals into systemic maternal circulation that have effects on the mammary gland, as suggested by Goldman et al. ( Reference Goldman, Garza and Nichols 26 ).
To our knowledge, this is the largest and most comprehensive study of the relationships between the concentrations of immune proteins in human milk and prematurity to date. We found no evidence of deficiency in antimicrobial immune factors in preterm milk, indicating that low levels of milk consumption in this group may be the most relevant nutritional contributor to LOS in the preterm population( Reference Patel, Johnson and Engstrom 12 , Reference Lavoie 64 ). This work suggests that up-regulation of expression of immunological proteins in the mammary gland during production of colostrum may occur through similar molecular mechanisms as the up-regulation of immune protein expression in milk from preterm mothers that we observed, and requires further investigation.
Acknowledgements
The authors are grateful for the guidance and assistance of the King Edward Memorial Hospital Postnatal Ward and Special Care Nursery staff in carrying out this research. The authors would like to thank all of the study participants who generously donated milk and gave their time to this research.
The authors of this work were supported by funding from the Women and Infants Research Foundation (T. S. and S. T. New Investigator Grant, S. T. postgraduate scholarship), the University of Western Australia Centre for Neonatal Research and Education (postgraduate scholarship S. T.), the PMH foundation (S. T. and M. L. L.), the Telethon New Children’s Hospital Research Fund (T. S., A. C. and K. S.) and Medela AG (D. T. G. and C. T. L.). D. T. G. and C. T. L. receive a salary from an unrestricted research grant from Medela AG administered by UWA. The funding bodies had no role in the design, analysis or writing of this article.
S. T., T. S., A. C., P. R. and K. S. designed the study. S. T., M. L. L. and C. H. K. recruited participants and collected samples and clinical data. S. T., D. A. D. and T. S. designed statistical analysis and S. T. carried out with assistance from D. A. D., D. T. G. and C. T. L. planned and carried out all lysozyme work. S. T. carried out all other experimental work. J. M. provided protocols and technical assistance for cytokine experiments. S. T. wrote the manuscript with input from other authors. Critical review and editing of the manuscript was performed by A. C., T. S., K. S., D. A. D., M. L. L. and D. T. G.
The authors have no conflict of interest to declare.
Supplementary Material
For supplementary material/s referred to in this article, please visit http://dx.doi.org/10.1017/S0007114516000234