Metabolic disorders that increase the risk of atherosclerosis-related arterial disease are characterised by inflammatory and oxidative processes that arise at sites including macrophages in the arterial wall and in vascular endothelium(Reference Libby, Ridker and Maseri1). These may be reflected in the increased levels of circulating biomarkers of inflammation and oxidation(Reference Pischon, Hu and Rexrode2).
Foods that may be associated with a rise in the levels of some of these molecules after consumption of single meals, generally fatty foods(Reference Manning, Sutherland and McGrath3, Reference El-Khoury, Hwaila and Frochet4), have been reported as well as the cross-sectional documentation of certain patterns of habitual eating(Reference Giugliano, Ceriello and Esposito5). We have previously found no such associations with single meals rich in full-fat dairy products including butter, cream, yogurt and cheese when compared with a low-fat dairy meal(Reference Nestel, Pally and MacIntosh6). Some population studies have found associations between at least butter consumption and the risk of CHD(Reference Bernstein, Sun and Hu7). In the recent update of the Nurses' Health Study(Reference Bernstein, Sun and Hu7), high-fat dairy consumption remained associated with adverse (CHD) events, whereas a meta-analysis of seventeen studies has suggested that at least milk consumption may be inversely, albeit weakly, associated with CVD risk(Reference Soedehma-Mutha, Ding and Al-Delaimy8). A large prospective study conducted in the Netherlands has reported increased CHD mortality to be associated with the consumption of butter in women but not in men(Reference Goldbohm, Chorus and Garre9). The intake of cheese appears to have not shown a similar association with CHD(Reference Nestel10), and recent reports have suggested that the consumption of fermented dairy foods may even be protective. A prospective population study conducted in the Netherlands in which an increased 10-year risk for CHD mortality was found with high intakes of dairy fat, especially butter, has shown that the risks for all-cause mortality and possibly of stroke were, however, lower on consumption of fermented dairy foods(Reference Goldbohm, Chorus and Garre9). Another study appears to have confirmed the potential cardioprotective effect of eating fermented dairy foods in a large prospective cohort in Sweden(Reference Warensjo, Jansson and Cederhol11).
In contrast with the nutritional epidemiology relevant to full-fat dairy products and butter, in particular(Reference Noakes, Nestel and Clifton12), the consumption of low-fat dairy foods has been reported to be associated with reduced CVD risk and less incident diabetes mellitus(Reference Elwood, Pickering and Givens13, Reference Liu, Choi and Ford14).
In the present study, we, therefore, investigated the effects of different dairy product-rich diets on novel sets of potential biomarkers of CHD including circulating inflammatory and pro-atherogenic biomarkers as well as a lipid profile derived by performing a lipidomic analysis of plasma. A low-fat dairy diet was compared with two full-fat dairy diets containing predominantly either fermented or non-fermented dairy products.
Experimental methods
Several groups of novel biomarkers were tested: eight recognised inflammatory and pro-atherogenic molecules; twelve classes of plasma lipid classes derived through a lipidomic analysis; plasma F2-isoprostanes, robust parameters of oxidative burden.
In response to an advertisement, twelve subjects volunteered to participate in the study. Requirements were age 40–70 years, BMI 26–35 kg/m2, waist circumference >94 cm for men and >80 cm for women, non-smoker, non-diabetic, stable hypertensive if treated, no other treated chronic disease including statin use, no intake of dietary supplements other than routine dosages of vitamins, drinking < 3 standard alcohol drinks daily, and being familiar and comfortable with foods to be tested.
The baseline characteristics of the subjects are given in Table 1 as means and standard deviations for the group.
Table 1 Characteristics of the twelve studied subjects (Mean values and standard deviations)

BP, blood pressure.
The study was carried out according to the guidelines laid down in the Declaration of Helsinki, and all procedures involving human subjects were approved by the Human Research Ethics Committee of the Alfred Hospital, Melbourne, where the study was conducted. Each volunteer was given full information about the study, and all volunteers provided written consent.
Procedures
The influence of a low-fat dairy diet compared with that of two full-fat dairy diets, one predominantly fermented and the other non-fermented, was chosen to be studied because of suggestions that the type of dairy food may affect CVD risk (see above). The order of the full-fat dairy test diets, fermented and non-fermented, was randomised for each subject, leading to a similar number of subjects being allocated to initial interventions. Each full-fat dairy dietary period was 3 weeks of duration. The low-fat dairy diet was consumed twice, between and at the end of the full-fat dairy dietary periods, for a duration of 2 weeks. The study began with a 2-week run-in familiarisation period during which the volunteers were habituated to the requirements of the study.
The subjects were asked to maintain normal physical activity, to avoid unusual or excessive food consumption and to drink no more than two standard drinks of alcohol daily and none for 24 h before blood testing. They weighed themselves daily and reported changes exceeding 1 kg, especially during the run-in phase. They were instructed by a research nurse on the foods that they could choose to consume in addition to the test diets provided and to record the type and amounts of foods eaten (guided by models and charts). Rotating menus devised by a dietitian facilitated compliance. In general, the daily foods consumed in addition to the test dairy foods included four servings of cereal, four servings of vegetables/salad, two servings of fruit, and one to two servings of a protein-rich food (lean meat, fish, pulses or eggs). The low-fat dairy diet required two daily servings of low-fat yogurt and milk. Carbohydrates were derived almost evenly from starches and mono- and disaccharides except for yogurt, which was sweetened with sucrose.
The subjects returned at the end of each dietary period, four times, during which body weight and blood pressure were recorded, the food diaries were inspected and venous blood was collected, with them having fasted for 10–12 h beforehand.
Test diets
Fat content provided between 30 and 35 % of total energy on consumption of the full-fat dairy diets, but only about half as much energy from fat was derived on consumption of the low-fat dairy diet. Table 2 summarises the details of the macronutrients and Na as calculated from the food diaries, which included all dairy product-rich foods required to have been eaten daily and represented the average for each dietary period. The data are discussed in the Results section. The following test foods were pre-packaged and provided to cover each dietary period and stored at home at 4°C: for the fermented dairy dietary period, cheddar cheese (85 g/d) and full-cream yogurt (three servings, 600 g/d) delivered fresh twice weekly; for the non-fermented dairy dietary period, butter (30 g/d) and cream (70 ml/d) delivered fresh twice weekly and small amounts of ice cream; for the low-fat dairy dietary period, 1 % fat milk (400 ml/d) and < 1 % fat yogurt (200 g/d). Unused portions were returned for checking compliance. Ca content was adjusted with Ca supplements to equalise differences derived through foods.
Table 2 Intakes of key nutrients for each study phase according to individual energy requirements (Mean values and standard deviations)
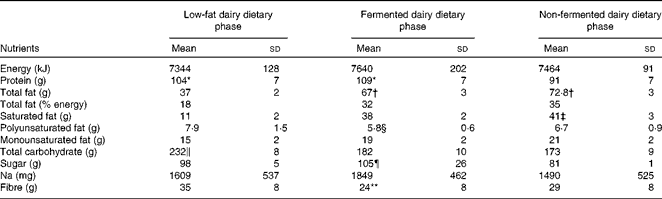
* Mean values were significantly higher than those in the non-fermented dairy dietary phase (P< 0·01; t test).
† Mean values were significantly higher than those in the low-fat dietary phase (P< 0·01) and fermented dairy dietary phase (P< 0·01); values in the fermented dairy dietary phase were also higher than those in the low-fat dietary phase (P< 0·01; t test).
‡ Mean values were significantly higher than those in the low-fat dietary phase (P< 0·01; t test).
§ Mean values were significantly lower than those in the low-fat dietary phase (P< 0·05) and the non-fermented dairy dietary phase (P< 0·01; t test).
∥ Mean values were significantly higher than those in the non-fermented and fermented dairy dietary phases (P< 0·01; t test).
¶ Mean values were significantly higher than those in the non-fermented dairy dietary phase (P< 0·01; t test).
** Mean values were significantly lower than those in the low-fat dietary phase (P< 0·01; t test).
Laboratory procedures
Blood was collected into EDTA tubes before and after each dietary period, and chilled plasma was separated and stored at − 80°C after the addition of 100 μm-butylhydroxytoluene. The concentrations of cytokines were determined using Milliplex MAP kits (Applied Biosystems). The concentrations of IL-6, IL-1β and TNF-α were determined using ‘High Sensitivity’ kits (Invitrogen Australia). The concentrations of two adhesion molecules, soluble intracellular adhesion molecule-1 and soluble vascular cell adhesion molecule-1, monocyte chemoattractant protein-1 (MCP-1) and macrophage inflammatory protein-1α were determined using human cytokine/chemokine kits (Millipore). The concentration of high-sensitivity C-reactive protein was determined on a COBAS Integra plus blood chemistry analyser (C-reactive Protein Latex; Roche Diagnostics). Inter-assay coefficients of variation were calculated for each cytokine from ten separate assays, and they ranged between 4·5 and 14 %. The eight biomarkers are listed out in Table 3.
Table 3 Concentrations of plasma inflammatory and pro-atherogenic biomarkers after low-fat, fermented and non-fermented dairy dietary periods (Mean values with their standard errors)

MCP-1, monocyte chemoattractant protein-1; MIP, macrophage inflammatory protein; hsCRP, high-sensitivity C-reactive protein; ICAM-1, intracellular adhesion molecule-1; VCAM-1, vascular cell adhesion molecule-1.
* Statistical analysis performed using a one-way repeated-measures ANOVA with a post hoc analysis adjusted for multiple comparisons (Sidak).
† Mean values were significantly increased in the non-fermented dairy dietary phase than in the fermented and low-fat dairy dietary phases (P< 0·05; post hoc analysis).
Plasma was analysed for glucose, total cholesterol, TAG, HDL-cholesterol and LDL-cholesterol concentrations on a COBAS Integra 400 plus blood chemistry analyser (Roche Diagnostics).
Lipidomic analysis was carried out by HPLC and electrospray ionisation-tandem MS using an Agilent 1200 liquid chromatography system (Agilent Technologies) combined with an Applied Biosystems API 4000 Q/TRAP mass spectrometer with a turbo-ionspray source (350°C) and an Analyst 1.5 data system (AB SCIEX)(Reference Meikle, Wong and Tsorotes15). We analysed over 300 individual lipid species representing the major phospholipid, sphingolipid and glycerolipid species. The individual species in each lipid class were summed for statistical evaluation.
Plasma total F2-isoprostane concentrations were measured by GC coupled to electron-capture negative-mode chemical ionisation and MS (GC/MS) using a modification of our previously reported method(Reference Mori, Croft and Puddey16). Briefly, F2-isoprostanes in plasma (0·25 ml) were hydrolysed under N2 with 1 m-KOH in methanol for 40 min at 40°C. The sample was acidified to a pH of 4·0 before being subjected to solid-phase chromatography on a pre-washed 200 mg Certify II column (Varian). After eluting with methanol–water (50:50, v/v) and hexane–ethyl acetate (75:25, v/v), the F2-isoprostanes were eluted with 2 ml ethyl acetate–methanol (90:10), dried and derivatised. F2-isoprostanes were detected by selected ion monitoring using m/z 569 and m/z 573 for endogenous and tetra-deuterated internal standards, respectively.
Statistical methods
For the purpose of comparing data obtained from the two low-fat dairy dietary periods with those obtained from the full-fat dairy dietary periods, the shorter low-fat dairy dietary periods were combined and averaged since none of the variables differed significantly, on average, between these two dietary periods. Thus, three diets were compared: low-fat dairy diet; fermented dairy diet; non-fermented dairy diet.
Differences between the effects of diets on the concentrations of biomarkers and lipid variables at the end of interventions were analysed by a one-way repeated-measures ANOVA and where appropriate by a post hoc analysis with Sidak adjustment for multiple comparisons. Differences in dietary constituents between the three groups were analysed by Student's t test. A value of P< 0·05 (two-sided) was deemed statistically significant.
The appropriate sample size was calculated from our previous study, in which the same eight inflammatory and atherogenic biomarkers were investigated(Reference Nestel, Pally and MacIntosh6), as well as from larger published reports that included interventions and used a similar methodology. The lipidomic sample size was derived from a large data set that our laboratory had obtained from a current prospective Australian study adjusted to resemble the characteristics of the subjects of the present study. A power of 80 % at α = 0·05 to detect differences of 20 % clustered the sample size between < 10 and modestly above 12 for a minority of biomarkers/lipids, justifying the decision to enrol twelve subjects. To the best of our knowledge, there have been no reports of interventions targeting the large number of lipids that were measured in the present study (>300), and limitations of the lipidomic data have been discussed in the study of Meikle et al. (Reference Meikle, Wong and Tsorotes15). Analysis was carried out using SPSS software (SPSS Inc.).
Results
Apart from the high average BMI (30·1 kg/m2) and average waist circumferences for men (102 cm) and women (103 cm), no other variables indicated a risk for the metabolic syndrome (Table 1). However, three subjects showed consistently mildly raised systolic blood pressure (140–150 mmHg) despite the treatment, and LDL-cholesterol concentration exceeded 3·6 mmol/l in six subjects, while HDL-cholesterol concentration was < 1·0 mmol/l and TAG concentration was >1·7 mmol/l in one subject.
Test diets
Table 2 shows minor differences in the macronutrient composition of the diets as prescribed. Consumption calculated from the food diaries showed good concordance with that of the prescribed diets. Energy intakes were comparable across the study since body weights were maintained constant with minor adjustments (1 kg being the greatest fluctuation). The non-fermented dairy diet provided significantly more fats than the fermented dairy diet (35 v. 32 % energy), reflecting more saturated fats. Polyunsaturated (PUFA n-6) content was lower in the fermented dairy diet than in the other diets. Protein content was higher in the fermented dairy and low-fat dairy diets than in the non-fermented dairy diet, while total carbohydrate content was higher in the low-fat dairy diet. The low-fat dairy diet clearly led to significantly greater consumption of carbohydrates as anticipated.
Circulating inflammatory and pro-atherogenic biomarkers
There was a non-significant trend for the concentrations of biomarkers to be higher at the end of the intervention periods on consumption of the low-fat dairy diet than on that of the fermented dairy diet, especially for those of five of the eight biomarkers (MCP-1, macrophage inflammatory protein-1α, TNF-α, IL-1β and vascular cell adhesion molecule-1), though none of these differences was significant. By contrast, the concentrations of some of the biomarkers tended to be lower during the low-fat dairy dietary period than during the non-fermented dairy dietary period, especially those of MCP-1, macrophage inflammatory protein-1α and high-sensitivity C-reactive protein, while the concentration of IL-6 was significantly higher on consumption of the non-fermented dairy diet than on that of either the low-fat dairy diet or the fermented dairy diet (P< 0·05; Table 3). On comparing the concentrations of biomarkers at the end of the fermented and non-fermented dairy dietary periods, it was found that only the concentration of IL-6 was significantly lower on consumption of the fermented dairy diet than on that of the non-fermented dairy diet (P< 0·05; Table 3).
Plasma lipids
The concentrations of plasma lipids showed the expected changes (Table 4). Lowest LDL-cholesterol and HDL-cholesterol concentrations were observed on consumption of the low-fat dairy diet, but plasma TAG concentrations did not differ significantly on consumption of all the diets.
Table 4 Concentrations of plasma lipids after low-fat, fermented and non-fermented dairy dietary periods (Mean values with their standard errors)

HDL-C, HDL-cholesterol; LDL-C, LDL-cholesterol.
* P values based on a one-way repeated-measures ANOVA and a post hoc analysis adjusted for multiple comparisons (Sidak).
† Mean values were increased in the non-fermented dairy dietary period compared with the low-fat dairy dietary period, approaching significance (P= 0·058; post hoc analysis).
‡ There were significant increments in both the fermented and non-fermented dairy dietary periods than in the low-fat dairy dietary period (P< 0·05 and P< 0·03, respectively; post hoc analysis).
Lipidomic biomarkers
There were multiple changes in the plasma lipidome associated with the dairy diets (Table 5). Most notable was the concentration of sphingomyelin, which was significantly higher following consumption of the non-fermented dairy diet than following that of the low-fat dairy diet (P< 0·02). Significantly lower concentrations of the two plasmalogen species phosphatidylcholine plasmalogen and phosphatidylethanolamine plasmalogen were found following consumption of the fermented dairy diet than following that of the low-fat dairy diet (P< 0·001 and P< 0·05, respectively).
Table 5 Concentrations of plasma lipid classes after low-fat, fermented and non-fermented dairy dietary periods (Mean values with their standard errors)

* Statistical analysis performed using a one-way repeated-measures ANOVA with a post hoc analysis adjusted for multiple comparisons (Sidak).
† Mean values were significantly increased in the non-fermented dairy dietary period than in the low-fat dairy dietary period (P< 0·02; post hoc analysis).
‡ There were significant increments in both the fermented and non-fermented dairy dietary periods than in the low-fat dairy dietary period (P< 0·02 and P< 0·05, respectively; post hoc analysis).
§ Mean values were significantly decreased in the fermented dairy dietary period than in the low-fat dairy dietary period (P< 0·001; post hoc analysis).
∥ Mean values were significantly decreased in the fermented dairy dietary period than in the low-fat dairy period (P< 0·05; post hoc analysis).
The concentration of phosphatidylcholine containing the odd-chain fatty acids 15 : 0 and 17 : 0 (odd-phosphatidylcholine) was significantly increased on consumption of both full-fat dairy diets, as would be expected from the higher intakes of these two fatty acids from the dairy fat. No significant differences were observed in the concentrations of phosphatidylinositol, phosphatidylserine, phosphatidylglycerol or those of glycosphingolipids and mono-, di- and trihexosylceramide (data not shown).
Plasma F2-isoprostanes
Mean plasma F2-isoprostane concentrations during the three dietary periods were not significantly different: 1843 (sd 320) pmol/l for the low-fat dairy dietary period; 1915 (sd 314) pmol/l for the fermented dairy dietary period; 1951 (sd 351) pmol/l for the non-fermented dairy dietary period.
Discussion
The present study was undertaken partly to demonstrate whether a low-fat dairy diet would be associated with lower concentrations of novel circulating biomarkers indicative of inflammation, atherogenesis and oxidative stress based on reports that dietary fat may raise the concentrations of several of the biomarkers tested in the study. A second hypothesis was that fermented dairy foods would represent a lower CVD risk than non-fermented dairy products on the basis of recent epidemiological reports. In most studies, including that which we reported previously(Reference Nestel, Pally and MacIntosh6), single fat meals that contained a variety of fats were tested. Mostly only the concentrations of two to four biomarkers were measured over 2–8 h after consumption of meals, and the findings were equivocal. The concentrations of some biomarkers such as IL-6, IL-1β and TNF-α increased, whereas in other studies the concentrations of high-sensitivity C-reactive protein, IL-6 and TNF-α either did not change or decreased (summarised in Nestel et al. (Reference Nestel, Pally and MacIntosh6)). In an earlier study, we had attempted to simplify the design by testing the postprandial responses of the biomarkers (which were also tested in the present study) after consumption of four individual dairy foods as breakfasts delivering similar amounts of fat(Reference Nestel, Pally and MacIntosh6). However, the concentrations of none of the biomarkers increased significantly and, in fact, the concentrations of several biomarkers decreased at 3 h.
In the present longer-term study, the panel of eight biomarkers that have been postulated as being cytokines, adhesion molecules and chemokines linking fat consumption with increased cardiovascular risk provided little evidence for lower biomarker concentrations after consumption of the low-fat dairy diet than after that of the full-fat dairy diets (Table 3). Furthermore, in comparison with those observed in the fermented dairy dietary period, the concentrations of six of the eight biomarkers were higher, albeit not significantly, during the low-fat dairy dietary period. The concentrations of two plasmalogens, lipids postulated to reflect a lower oxidation burden, were significantly higher on consumption of the low-fat dairy diet than on that of the fermented dairy diet (Table 5). However, the concentrations of two important biomarkers, IL-6 and sphingomyelin, reflecting inflammation and atherosclerosis, respectively, were significantly lower on consumption of the low-fat dairy diet than on that of the full-fat fermented dairy diet. These findings suggest that consumption of the low-fat dairy diet does not confer clear benefits when compared with that of the full-fat fermented dairy diet but may do so when compared with that of the full-fat non-fermented dairy diet. The higher concentration of phosphatidylcholine following consumption of the full-fat dairy diets (Table 5) reflects higher levels of the ruminant-specific fatty acids 15 : 0 and 17 : 0.
However, with the low-fat dairy diet being a carbohydrate-rich diet also, these findings may indicate an adverse influence of a higher sugar plus starch intake rather than a beneficial effect of fermented dairy foods. Nevertheless, no such trend was observed when the concentrations of biomarkers during the non-fermented dairy dietary period were compared with those of the biomarkers after the low-fat dairy dietary period.
A parallel question was whether fermented and non-fermented dairy foods influence the biomarkers differently. Only the concentration of cytokine IL-6 was significantly lower during the fermented dairy dietary period than during the non-fermented dairy dietary period (Table 3).
We selected the eight biomarkers because they characterise some of the inflammatory and atherogenic processes in tissues, including the arterial wall, that have been associated with clinical CHD events in large prospective studies and in intervention trials. In a large meta-analysis, circulating C-reactive protein has been shown to be associated with future cardiovascular events(17), although this was not confirmed in the large trial of simvastatin in 20 536 patients in the Heart Protection Study(18). Among elderly individuals in the Health ABC Study, the inflammatory biomarkers IL-6 and TNF-α appeared to be the predictors of incident CHD(Reference Cesari, Pennix and Newman19). The concentration of IL-6 was also significantly associated with clinical atherosclerotic disease in other studies(Reference Tzoulaki, Murray and Lee20, Reference Empana, Canoui-Poitrine and Luc21). MCP-1, the protein that mediates the recruitment of monocytes to the arterial wall, has been associated with future peripheral arterial disease(Reference Hoogveen, Morrison and Boerwinkle22). In the Health Professionals Follow-up Study(Reference Pischon, Hu and Rexrode2), the adhesion molecule intracellular adhesion molecule-1 has been reported to be independently predictive of future CHD. Therefore, it seemed important to investigate the possible modifying effects on these biomarkers of dietary factors and dairy foods, in particular.
The consumption of carbohydrates may also affect inflammatory biomarkers. The indices of inflammation represented by the expression of TNF-α and IL-1β in mononuclear cells increased following consumption of single glucose or cream meals(Reference Depurkar, Ghanim and Friedman23). Relevant to the present study, a longer intervention with carbohydrates delivering a low glycaemic load has been shown to lower TNF-α, MCP-1 and IL-6 concentrations, whereas high glycaemic loads consumed over the 12-week period raised the TNF-α concentration in insulin-resistant subjects(Reference Kelly, Haus and Solomon24). The estimated glycaemic index in the present study averaged 64 ± 8 units, which is a near-median value for Australian diets. These observations with both short-term and long-term consumption of high-glucose drinks or a high-carbohydrate diet suggest that we need to take the possible effect of the high carbohydrate content in the low-fat dairy diet into consideration. It is of interest that in an 8-week comparison of low-fat dairy foods and carbohydrate-rich meals, van Meiji & Mensink(Reference Van Meiji and Mensink25) did not find differences in the concentrations of TNF-α, soluble intracellular adhesion molecule-1, soluble vascular cell adhesion molecule-1, MCP-1 or IL-6.
Of the complex lipids identified by the lipidomic analysis to differ among the diets, sphingomyelin and plasmalogens are of interest. Sphingomyelin is a complex lipid that has been identified in several population and case–control studies to be associated with an increased risk for CHD and subclinical atherosclerosis(Reference Jiang, Paultre and Pearson26, Reference Nelson, Jiang and Tabas27). By performing a lipidomic analysis of atherosclerotic plaques, Stegemann et al. (Reference Stegemann, Drozdov and Shalhoub28) reported on the sphingomyelin content of endarterectomy specimens in which the sphingomyelin species showed the greatest enrichment in a twenty-four-lipid profile forming part of the ‘vulnerable plaque lipid signature’. The mechanism of this is not clear, but sphingomyelin has been reported to influence lipid metabolism through several pathways such as by inhibiting lecithin cholesteryl acyltansferase and potentially disrupting reverse cholesterol transport(Reference Bolin and Jonas29). Plasmalogens are considered to be vulnerable to oxidation with their high content of PUFA and alkenyl linkage(Reference Skaff, Pattison and Davies30). Thus, the reduction in plasma plasmalogen concentration following the fermented dairy dietary period may be favourable by lowering a potential oxidative burden, which is a key factor in atherogenesis and has been associated with increased CVD risk(Reference Meisinger, Baumert and Khuseyinova31). Alternatively, this may reflect an altered plasmalogen synthesis in response to fermented dairy products. Stancliffe et al. (Reference Stancliffe, Thorpe and Zemel32) have recently reported reduced markers of oxidative stress in subjects consuming dairy foods. However, we did not observe differences in plasma F2-isoprostane concentrations (an excellent biomarker of in vivo oxidative damage) on consumption of the low-fat dairy diet and the two dairy diets, and so the relevance of oxidative stress in this study is unclear.
Population studies that relate the consumption of dairy foods to inflammatory biomarkers are few and two recent reports appear to be contradictory. In the Greek ATTICA study, the concentrations of circulating inflammatory markers were lowest among the highest consumers of dairy foods(Reference Panagiotacos, Pitsavos and Zampelas33). By contrast, a 6-month intervention with practical amounts of dairy products, three to five daily portions that doubled the intakes of cheese, yogurt and milk, failed to show reduction in the concentrations of the plasma inflammatory biomarkers high-sensitivity C-reactive protein, IL-6 and TNF-α(Reference Wennersberg, Smedman and Turpeinen34).
As has been indicated above, several recent reports have emphasised the differential effects of fermented dairy and non-fermented dairy foods on cardiovascular outcomes. A 10-year prospective study conducted in the Netherlands has found butter consumption to be significantly associated with future CHD mortality in women but not in men, whereas all-cause mortality and possibly stroke were reduced in both men and women among those eating the most fermented dairy foods(Reference Goldbohm, Chorus and Garre9). A large prospective case–control study conducted in Sweden was consistent with the Netherlands study, but showed more clearly a cardioprotective effect of fermented dairy foods(Reference Warensjo, Jansson and Cederhol11).
The consumption of low-fat dairy foods has been encouraged for their nutritive value while avoiding the adverse effects on CVD risk as in the recent updated analysis of the Nurses' Health Study(Reference Bernstein, Sun and Hu7). Of considerable additional interest has been the recent suggestion from several trials that consuming dairy fat foods and low-fat dairy foods, in particular, may diminish the risk of developing type 2 diabetes(Reference Elwood, Pickering and Givens13, Reference Liu, Choi and Ford14). In a nested case–control study within a large population derived from eight European nations, the risk of type 2 diabetes has been reported to be inversely related to the consumption of fermented dairy products (cheese, yogurt and buttermilk) but not to that of total dairy foods(Reference Sluijs, Forouhi and Beulens35). In the present study, there were no significant differences in blood glucose levels on consumption of the three diets.
Possible limitations in the interpretation result from several differences in the macronutrient composition of the three diets. However, the main conclusions are based on the presence or absence of differences in biomarker concentrations between low-fat dairy and full-fat dairy diets reflected by definition differences in fat intake compensated by differences in carbohydrate intake. Low-fat and fermented full-fat dairy diets had similar amounts of protein, although the non-fermented dairy diet contained less protein; however, this minor difference is unlikely to have had an impact on the differential effects of low-fat and full-fat dairy diets. The small difference in the duration of dietary periods is also unlikely to have influenced the results. The two full-fat dairy dietary periods were randomised and of equal duration in order to test the possible differences between fermented and non-fermented dairy foods. We chose to interpolate two low-fat dairy dietary periods, each of 2 weeks, between and at the end of the full-fat dairy dietary periods in order to have a low-fat dairy dietary period follow each of the full-fat dairy dietary periods.
A further limitation is related to the sample size of twelve subjects, although the cross-over design among the three diets strengthens our findings. Nevertheless, the calculated sample sizes to achieve significant desired changes clustered about the number enrolled for nearly all of the biomarkers and lipids in the present study (see the Statistical methods section).
In conclusion, comparisons of diets comprising predominantly low-fat dairy and full-fat dairy products have failed to demonstrate a clearly unfavourable profile of inflammatory biomarkers and complex lipids with full-fat dairy diet consumption. The intake of the fermented dairy diet did appear to provide a more favourable biomarker profile than that of the non-fermented dairy diet. The comprehensive nature of the biomarker analyses including a large number of plasma lipids measured by lipidomics appears to have provided a novel evaluation.
Acknowledgements
The following food companies provided partial support through the Dairy Health and Nutrition Consortium, with several of them providing bulk supplies of butter, cheese and ice cream: Bega Cheese/Tatura Milk Industries; Lion Dairy & Drinks; Fonterra Australia; Parmalat Australia; Murray Goulburn Co-operative; Warrnambool Cheese and Butter; Geoffrey Gardiner Foundation; Dairy Australia; Dairy Innovation Australia.
We thank Sonja Middleton for dietetic advice. The authors' responsibilities are as follows: P. J. N. and P. J. M. were responsible for devising and conducting the study and for the writing of the manuscript; G. W. was responsible for the statistical analysis; K. C. and T. A. M. were responsible for the F2-isoprostane measurements and interpretation; S. P. was the research nurse and N. M. and C. K. B. were the laboratory scientists responsible for the measurements. The sponsor provided funds directly to the Institute. Author disclosures: P. J. M. and P. J. N. received grant-in-aid for the present study from the Dairy Health and Nutrition Consortium. N. M., S. P., G. W., C. K. B., K. C. and T. A. M. report no conflicts of interest.