Introduction
Junglerice has become one of the top two prevalent weeds in soybean [Glycine max (L.) Merr.] and cotton (Gossypium hirsutum L.) in much of Tennessee and across the mid-south (Perkins et al. Reference Perkins, Mueller and Steckel2021; Tahir Reference Tahir2007). Junglerice and barnyardgrass [Echinochloa crus-galli (L.) P. Beauv.] are the two most common Echinochloa species found in Tennessee (Perkins et al. Reference Perkins, Mueller and Steckel2021) and share many characteristics such as vast seed production, rapid C4 growth, and extended emergence periods. Several populations of junglerice have been tested for glyphosate and clethodim resistance (Perkins et al. Reference Perkins, Mueller and Steckel2021). Those studies have indicated that 15% of the populations have a 2-fold to 8-fold resistant to glyphosate, which is consistent with a report by Nandula et al. (Reference Nandula, Montgomery, Vennapusa, Jugulam, Giacomini, Ray, Bond, Steckel and Tranel2018) who studied selected Mississippi and Tennessee populations.
Many growers have elected to grow soybean and cotton that is resistant to glyphosate + dicamba (Wechsler et al. Reference Wechsler, Smith, McFadden, Dodson and Williamson2019). Applying mixtures of glyphosate with dicamba provides broad-spectrum weed control with the expectation that the dicamba will control glyphosate-resistant broadleaf weeds and glyphosate will control grass weeds. However, many field reports from cotton and soybean growers in Tennessee suggest that grass weed control, particularly junglerice, from glyphosate + dicamba or clethodim + dicamba applications has been unacceptable (Perkins et al. Reference Perkins, Mueller and Steckel2021).
A decrease in herbicidal activity on grasses such as junglerice has been documented from mixtures of dicamba with glyphosate compared with only glyphosate applied alone (Flint and Barrett Reference Flint and Barrett1989; O’Sullivan and O’Donovan Reference O’Sullivan and O’Donovan1980). Colby (Reference Colby1967) has described antagonism as a result of applying two herbicides in combination, which will result in less control than what is expected based on how the individual herbicides perform alone. Herbicides such as glyphosate, dicamba, and 2,4-D and their behavior in various combinations is not fully understood for their weed-control efficacy. Both Flint and Barrett (Reference Flint and Barrett1989) and O’Sullivan and O’Donovan (Reference O’Sullivan and O’Donovan1980) have shown that antagonism can be both dependent on rates of herbicide usage and the species being evaluated. Antagonism plus herbicide resistance can lead to weed control failure after only a few years. Avoiding antagonism from herbicide mixtures can also aid in resistance management.
Antagonism has been observed with graminicides as well when applied with the auxin herbicides (Blackshaw et al. Reference Blackshaw, Harker, Clayton and O’Donovan2006; Fletcher and Drexler Reference Fletcher and Drexler1980; Mueller et al. Reference Mueller, Witt and Barrett1989; Olson and Nalewaja Reference Olson and Nalewaja1981; Todd and Stobbe Reference Todd and Stobbe1980). The physiological antagonism is not believed to be due to graminicide retention or absorption differences but rather to reduced translocation to meristematic tissues (Barnwell and Cobb Reference Barnwell and Cobb1994; Mueller et al. Reference Mueller, Barrett and Witt1990). The contrast between the modes of action of these herbicides has been implicated as the cause of antagonism, wherein the acetyl CoA carboxylase–inhibiting graminicides reduce proton efflux, whereas auxin herbicides stimulate proton efflux (Barnwell and Cobb Reference Barnwell and Cobb1993; Hull and Cobb Reference Hull and Cobb1998). It has been reported that dicamba applications can disrupt phloem loading (Lalonde et al. Reference Lalonde, Tegeder, Throne-Holst, Frommer and Patrick2003). Therefore, this may impact glyphosate translocation throughout the plant. In addition, the synthetic auxin response is a complicated and dynamic pathway that might be causing other physiological changes that in turn can affect the ability of glyphosate to reach its target site (e.g., sequestration). Researchers have also reported that 2,4-D decreased uptake and translocation of glyphosate, thus reducing junglerice control. Moreover, Li et al. (Reference Li, Han, Bai and Yu2020) reported that the glyphosate antagonism from 2,4-D was much higher in glyphosate-resistant junglerice than in glyphosate-susceptible populations. Researchers have reported that pretreatment with 2,4-D can upregulate cytochrome P450 in ryegrass (Lolium rigidum L.) resulting in a 10-fold increase in the plant’s tolerance to glyphosate (Han et al. Reference Han, Yu, Cawthray and Powles2013). Dicamba applications have been known to disrupt the natural hormone signaling, with the stimulation of ethylene biosynthesis occurring within hours of application and then growth inhibition starting within the first 24 h (Grossman Reference Grossmann2010). There has been evidence that abscisic acid, auxins, and gibberellins can be involved with the phloem loading and unloading (Lalonde et al. Reference Lalonde, Tegeder, Throne-Holst, Frommer and Patrick2003). This will disrupt the native hormone signaling, which impacts the herbicide transport. Additionally, glyphosate can inhibit synthesis of the amino acid tryptophan, a precursor involved in the biosynthesis of indole acetic acid (Taiz and Zeiger Reference Taiz and Zeiger2006). Hormone signaling has been described as a complex signal transduction cascade and often involves more than one phytohormone. Therefore, it is possible that the inhibition of auxin biosynthesis with concurrent exposure to high concentrations of a synthetic auxin could result in the antagonism observed.
When two herbicides are applied together in a mixture, the interactions can be described by the use of Colby’s method (Colby Reference Colby1967). However, in circumstances in which one herbicide has no activity on one of the species, then Colby’s method cannot be used because the model requires control greater than 0% from both of the herbicides. Though a significant decrease in herbicidal activity from the mixture (e.g., glyphosate plus dicamba) compared with the herbicides alone with activity (e.g., glyphosate) can be considered antagonism. This methodology was used by both Flint and Barrett (Reference Flint and Barrett1989) and O’Sullivan and O’Donovan (Reference O’Sullivan and O’Donovan1980).
Another factor to consider in herbicide antagonism is the formulation of an active ingredient. For example, Kudsk and Mathiassen (Reference Kudsk and Mathiassen2004) have reported higher levels of synergism for mixtures of commercial products compared with the technical grade laboratory products. In this scenario, the adjuvants in the commercial products may be improving the uptake of one or both products in the mixture. Nalewaja and Matysiak (Reference Nalewaja and Matysiak1992) reported that interactions between herbicides can also differ between commercial formulations of the same active ingredient. Finally, a change in herbicide formulation cannot only impact the interaction among herbicides in its chemical structure, but could also alter the droplet spectra. Mueller and Womac (Reference Mueller and Womac1997) reported differences in the droplet size produced between different formulations of glyphosate.
One more possible source of reduced junglerice control could be due to label application directions. Due to off-target movement (OTM) concerns, label specified dicamba formulations that may be applied POST in Xtend crops are labeled to be applied using ultracourse nozzles and a drift reduction agent (DRA; Anonymous 2019a; Anonymous 2019b). These mandated application parameters may reduce OTM, but researchers have observed a reduction in weed control (Carter et al. Reference Carter, Prostko and Davis2017). It is known that small droplet size is more important for spray retention on upright grass weeds compared with broadleaf weeds that have a horizontal leaf structure (Etheridge et al. Reference Etheridge, Hart, Hayes and Mueller2001; McKinlay et al. Reference McKinlay, Ashford and Ford1974).
DRAs are used to modify spray characteristics to reduce spray drift, usually by minimizing small droplet formation. Previous research has shown that DRA use can increase the volume median diameter of sprays and thereby reduce spray drift (Zhu et al. Reference Zhu, Dexter, Fox, Reichard, Brazee and Ozkan1997). Another study found a reduction in total drift deposits in field evaluations for wind speeds ranging from 2.9 to 4.9 m/s by 15% to 50% with low concentrations and up to 70% to 80% with high concentrations (Bode et al. Reference Bode, Butler and Goering1976). Fietsam et al. (Reference Fietsam, Young and Steffen2004) reported that the use of a DRA with glyphosate reduced spray coverage by 6%.
Junglerice prevalence in the mid-southern United States has increased recently (Perkins et al. Reference Perkins, Mueller and Steckel2021; Tahir Reference Tahir2007). This could be attributed to the evolution of glyphosate resistance and the potential dicamba antagonism of glyphosate. Reduced junglerice control could be due to the labeled ultracourse droplet nozzles and DRAs that are mandated to be used for dicamba applications on dicamba-resistant soybean and cotton. The majority of hectares in Tennessee and across the mid-south receive a glyphosate + dicamba application. The U.S. Department of Agriculture reports (Wechsler et al. Reference Wechsler, Smith, McFadden, Dodson and Williamson2019) that in 2018, 71% of the hectares were planted with dicamba-tolerant soybean, with more than 2.2 million kg of dicamba used in the United States in this crop. With 16 million soybean hectares planted with Xtend varieties in 2019, the use of dicamba increased. A recent memorandum issued by the U.S. Environmental Protection Agency on the benefits of dicamba in dicamba-tolerant soybean production suggested that 97% of dicamba applications were mixed with glyphosate in 2018 and 2019 (Orlowski and Kells Reference Orlowski and Kells2020). This herbicide mixture and application could be causing the reduced grass control recently observed with glyphosate and clethodim. Finally, growers reporting failure to control Palmer amaranth with glyphosate + dicamba applications have resulted in some producers using higher dicamba rates (Steckel Reference Steckel2019). Although using higher dicamba rates may improve Palmer amaranth control, it could also decrease glyphosate effectiveness on junglerice.
Therefore, the objective of this research was to 1) assess junglerice control with mixtures containing dicamba and 2) assess whether labeled nozzles and DRAs used in dicamba applications are reducing control; and 3) examine whether increased rates of dicamba in these mixtures resulted in less junglerice control.
Materials and Methods
Field Component
This field experiment was replicated across three locations and 2 yr for a total of six experimental site-years. The research was arranged in a randomized complete block design with a two factor-factorial treatment structure with nozzle selection and herbicide treatment as the main factors. Plot size was 1.5 m wide and 9.1 m long in Jackson at the West Tennessee Research and Education Center (WTREC). Plots at other two locations, Milan Research and Education Center (MREC) and a grower field (Burlison, TN), were 1.5 m wide and 6 m long. Depending upon location, there were three (MREC and Burlison) or four (WTREC) replications. The two nozzles tested were Turbo Teejet Induction (TTI) 11003 nozzles and the air induction extended range (AIXR) 11003 flat-fan nozzles. The TTI 11003 nozzle is the labeled nozzle type for dicamba applications in Xtend crops and was applied at 275 kpa, which produces an ultracourse droplet (Anonymous 2021a). Likewise, the nozzle size for the AIXR was 03-orifice as well. The AIXR at the operating pressure of this boom produced a course droplet (Anonymous 2021a) and is not labeled for dicamba applications on Xtend crops. The labeled orifice size for these applications is 025 and higher (Anonymous 2021b). We chose the 03-orifice size to be able to apply these applications as directed by the label. The second factor was herbicide treatment and included a nontreated (check), glyphosate (Roundup Powermax®, Bayer Crop Protection, St. Louis, MO), clethodim (Intensity®, Loveland Products, Greenville, MS), glyphosate + clethodim, glyphosate + dicamba (Engenia, BASF Corporation, Ludwigshafen, Germany), clethodim + dicamba, glyphosate + clethodim + dicamba, glyphosate + dicamba + DRA (OnTarget®, Winfield United, Arden Hills, MN), and clethodim + dicamba + DRA. Herbicide rates were consistent throughout with glyphosate at 870 g ha−1, dicamba at 560 g ha−1, and clethodim at 105 g ha−1. DRA was used at 0.25% vol/vol. Applications were made with a CO2 backpack sprayer calibrated to apply at 142 L ha−1. Each herbicide treatment was evaluated using each nozzle previously mentioned. Herbicides were applied when junglerice plants were 8 to 10 cm in height. Control of junglerice was visually estimated on a scale of 0% to 100% where 0% = no injury and 100% = plant death at 7, 14, and 21 d after treatment. Aboveground, fresh weight biomass data was collected 21 to 28 d after treatment in a 0.2-m area by clipping plants at the soil surface. Biomass was collected and weighed using fresh weights and measured in grams. Only latest evaluations are presented here for brevity.
A second group of field experiments was conducted in 2020 at three locations. The purpose of this study was to analyze the effect of increasing the dicamba rate with mixtures of glyphosate. The same methods were used as described for the previous field experiment. Herbicide treatments include a nontreated (check), glyphosate, glyphosate + dicamba (1× rate), glyphosate + dicamba (1.5× rate), glyphosate + dicamba (2× rate), glyphosate + dicamba (1× rate) + DRA, glyphosate + dicamba (1.5× rate) + DRA, and glyphosate + dicamba (2× rate) + DRA. Control of junglerice was visually estimated on a scale of 0% to 100% where 0% = no injury and 100% = plant death at 7, 14, and 21 d after treatment. Aboveground, fresh weight biomass data was collected 21 to 28 dd after treatment in a 0.2-m area by clipping plants at the soil surface. Biomass was collected and weighed using fresh weights and measured in grams. Only latest evaluations are presented here for brevity.
A third field experiment was conducted in 2019 at Jackson at WTREC and then repeated in 2020 again at WTREC and MREC. The purpose of this study was to analyze antagonism from glyphosate/clethodim + dicamba mixtures compared with applications of glyphosate/clethodim + 2,4-D (Enlist, Corteva Agrisciences, Wilmington, DE). The same methods were used as described for the previous field experiment. Herbicide treatments include a nontreated (check), glyphosate, clethodim, glyphosate + clethodim, glyphosate + dicamba, clethodim + dicamba, glyphosate + 2,4-D, and clethodim + 2,4-D. Herbicide rates were consistent throughout with glyphosate at 870 g ha−1, dicamba at 560 g ha−1, and clethodim at 105 g ha−1. Control of junglerice was visually estimated on a scale of 0% to 100% where 0% = no injury and 100% = plant death at 7, 14, and 21 d after treatment. Aboveground, fresh weight biomass data was collected 21 to 28 d after treatment in a 0.2-m area by clipping plants at the soil surface. Biomass was collected and weighed using fresh weights and measured in grams. Only latest evaluations are presented here for brevity.
Greenhouse Research
The main field experiment was replicated in a greenhouse (Vero Beach, FL) to determine what role mixing glyphosate + dicamba or clethodim + dicamba has on control of Echinochloa species. Touchdown Hi-Tech® (glyphosate, Syngenta Crop Protection, Greensboro, NC) treatments included 0.25% vol/vol nonionic surfactant and Select Max® (clethodim, Valent U.S.A LLC., Walnut Creek, CA) treatments included 1% vol/vol crop oil concentrate in this experiment. In addition, either glyphosate or clethodim was mixed with dicamba with and without a DRA. Treatments were applied in a Generation 4 Research Track Sprayer (DeVries Manufacturing, Inc., Hollandale, MN). The track sprayer speed was calibrated to deliver 142 L ha−1 and the nozzle height was set to be spraying approximately 40 to 45 cm above the crop canopy. Herbicides were applied when plants reached 8 to 10 cm in height. Junglerice control was visually estimated on a scale of 0% to 100% where 0% = no injury and 100% = plant death at 28 d after treatment. Biomass was taken 28 to 35 d after treatment. Biomass was collected by clipping plants at the soil surface and fresh weight data collected.
Data Analysis
This greenhouse study was arranged in a randomized complete block design with a two factor-factorial treatment structure with nozzle selection and herbicide treatment being the factors. It was blocked on site due to Echinochloa spp. population density and glyphosate resistance. Fixed effects were herbicide treatment and nozzles. Environment, replications, and any interactions of fixed by random effects were considered random in the model. Each year-location combination was considered an environment sampled at random from a population as described by Carmer et al. (Reference Carmer, Nyquist and Walker1989). Designating the environments random will broaden the possible inference space the experimental results are applicable to (Carmer et al. Reference Carmer, Nyquist and Walker1989). Mean separation for individual treatment differences was performed using Fisher’s protected LSD test at P < 0.05. Post-ANOVA single degree of freedom contrasts were then used (SAS v9.4; SAS Institute, Cary, NC) to compare herbicide applications with and without dicamba as well as nozzle selection comparing AIXR flat-fan to TTI nozzles, averaged across six environments to measure the response from the addition of dicamba to the spray tank and using TTI nozzles.
Results and Discussion
Field Component
There was an overall herbicide treatment effect (P < 0.001) among treatments with glyphosate. Single-degree-of-freedom contrasts were then used to compare treatments with and without dicamba and to measure the nozzle effect and herbicide antagonism in these parameters. When analyzing treatments across both nozzles, there was a difference between treatments that contained dicamba and treatments that did not. Initially, glyphosate alone provided 75% control (Table 1). The addition of dicamba to glyphosate resulted in 21% less control (P = 0.047). The addition of a DRA to this mixture numerically reduced control an additional 20% (P = 0.059). These results suggest that applying dicamba mixed with glyphosate as directed by the registrant’s labels (Anonymous 2019a; Anonymous 2019b) would reduce junglerice control by 40% compared with glyphosate (Anonymous 2019c) alone as directed by its label.
Table 1. Single degree of freedom contrasts comparing glyphosate and/or clethodim to those herbicides mixed with dicamba or dicamba plus DRA on junglerice across six environments in Tennessee. a All applications made with TTI nozzles.

a Abbreviations: DRA, drift reduction agent; TTI, Turbo Teejet Induction.
An application of clethodim alone provided the highest control of junglerice at 88%. A glyphosate + clethodim mixture provided similar results at 87%. Dicamba mixed with clethodim numerically reduced junglerice control by 11% from visual observations and increased junglerice biomass 45% compared to clethodim alone. Similar to glyphosate, when clethodim + dicamba was applied as directed by the dicamba registrants’ labels using the TTI nozzles and DRA, junglerice control was reduced by 22% with biomass increases of 80% compared to applying clethodim as directed by the label (Anonymous 2019d). However, it is notable that treatments containing glyphosate had higher biomass measurements. The authors suggest this could be due to more regrowth occurring in these treatments compared to clethodim.
When analyzing the two nozzles (AIXR flat-fan and TTI), there was a 7% junglerice reduction in control using the TTI nozzles (Table 2). These results are similar to those reported by Carter et al. (Reference Carter, Prostko and Davis2017) who observed a 5% to 6% reduction in grass control. This 7% control reduction was additive to the 16% (antagonism) loss when using dicamba (Table 1), giving a total loss of 23%. These TTI nozzles are labeled for in-crop dicamba applications. This would suggest that growers should switch from TTI to AIXR nozzles if junglerice is present when using glyphosate and/or clethodim alone. In addition, these findings suggest that to achieve better junglerice control, do not mix dicamba with glyphosate and/or clethodim. These field locations are all considered to be glyphosate susceptible populations due to still achieving relatively good control, although only 75%.
Table 2. Single degree of freedom contrasts comparing AIXR flat-fan nozzles to TTI nozzles to those herbicides mixed with dicamba or dicamba plus DRA on junglerice across six environments in Tennessee. a

a Abbreviations: AIXR, air induction XR; DRA, DRA, drift reduction agent; TTI, Turbo Teejet Induction.
Evaluation of Increased Dicamba Rate on Glyphosate Efficacy
The 1× dicamba rate (560 g ha−1) mixed with glyphosate provided 56% junglerice control (Table 3). The 1.5× use rate (840 g ha−1) of dicamba mixed with glyphosate reduced grass control by an additional 14% (P < 0.001). A 2× use rate (1,120 g ha−1) reduced control by an additional 9%. Biomass measurements mirrored the herbicide efficacy ratings and significantly increased as the dicamba rate increased. These results show that increased rates of dicamba resulted in decreased grass control. The addition of a DRA resulted in the greatest grass control loss compared to glyphosate + dicamba.
Table 3. Observed antagonism with increasing rates of dicamba mixtures with glyphosate (870 g ha−1) with/without a DRA on junglerice control across three locations in 2020 in Tennessee. All applications made with TTI nozzles. a,b
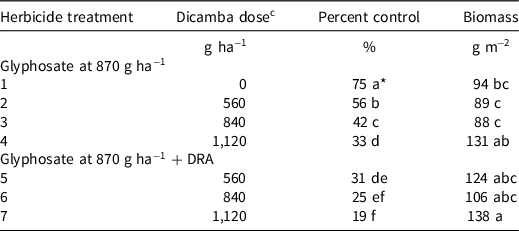
a Abbreviations: DRA, DRA, drift reduction agent; TTI, Turbo Teejet Induction.
b Means followed by the same letter are not significantly different according to Fisher’s protected LSD at P < 0.05.
c 560 g ha−1 represents a 1× labeled use rate of dicamba.
Comparing Antagonism Between Dicamba and 2,4-D
There was no interaction between nozzles and herbicide treatment. Regardless of herbicide treatment junglerice control was always lower when applied with the ultracourse TTI nozzle. No statistical differences were found between these mixtures. Junglerice control was similar with the glyphosate + dicamba treatment compared to the glyphosate + 2,4-D mixture (Table 4). Numerically, there was less antagonism from glyphosate + 2,4-D mixtures compared to the dicamba mixtures. However, there was numerically more antagonism observed from the clethodim + 2,4-D mixtures compared to dicamba.
Table 4. Single degree of freedom contrasts comparing herbicide applications on junglerice control across three locations in 2019 and 2020 in Tennessee. a,b

a Abbreviations: DRA, DRA, drift reduction agent; TTI, Turbo Teejet Induction.
b All applications were made with TTI nozzles.
Greenhouse Experiments
Reductions in junglerice control due to dicamba being added to glyphosate mixtures were observed in the greenhouse. However, they were not as pronounced compared with those in the field (Table 5). Glyphosate alone provided 96% control of junglerice compared with 84% control with glyphosate + dicamba (P < 0.001). However, no differences were observed (P = 0.090) when comparing clethodim (96%) to a clethodim + dicamba (97%) application. A glyphosate + dicamba application provided 84% control on junglerice, however, a glyphosate + dicamba + DRA application increased control (91%), which was similar to glyphosate alone (96%; P = 0.012). The addition of a DRA to clethodim + dicamba did not influence control (P = 0.173). There were no differences in the control with clethodim alone or in mixture (P = 0.726). The biomass data supported these results with no differences detected. The overall better control observed particularly with the DRA in the greenhouse compared to the field would be consistent with results reported by Combellack (Reference Combellack1982) that due to the environment and application variability in the field, less control in the field was observed compared with greenhouse applications. These results are also consistent with those reported by Perkins et al. (Reference Perkins, Mueller and Steckel2021) who achieved better junglerice control with glyphosate and clethodim in the greenhouse compared to the same populations in field research.
Table 5. Single degree of freedom contrasts on herbicides applied with TTI nozzles comparing herbicides averaged across six populations in the greenhouse. a

a Abbreviations: DRA, DRA, drift reduction agent; TTI, Turbo Teejet Induction.
There were observed control differences between nozzles (AIXR flat-fan vs. TTI) of 6% (P = 0.015; Table 6). These results are similar to what we observed in the field component of this research.
Table 6. Single degree of freedom contrast statement comparing nozzles for junglerice control averaged across six populations in the greenhouse. a

a Abbreviations: AIXR, air induction XR; TTI, Turbo Teejet Induction.
In conclusion, the addition of dicamba decreased junglerice control of clethodim and glyphosate in field studies. These field data suggest that mixtures with dicamba result in 15% less junglerice control. An additional 7% control loss was observed when the TTI nozzles were used, and an additional 16% loss occurred when a DRA was added to the spray tank. Moving forward, these data suggest separating glyphosate and/or clethodim applications with dicamba. Recommendations presented in Extension publications vary from 1 to 7 d on the length of time needed to mitigate antagonism between application of herbicides primarily used for broadleaf control compared to the herbicide targeting grasses (Barber et al. Reference Barber, Butts, Boyd, Cunningham, Norsworthy, Burgos and Bertucci2020; Loux et al. Reference Loux, Doohan, Dobbels, Johnson, Young, Zimmer and Hager2020). Future research designed to evaluate application timing of glyphosate or clethodim compared to dicamba and 2,4-D could help applicators plan the best strategy for achieving consistent grass control. A recent survey showed that on average, 40% of the fields in Tennessee have both Palmer amaranth plus Echinochloa spp. (Perkins et al. Reference Perkins, Mueller and Steckel2021). Growers want to control all weeds with one application of glyphosate + dicamba. However, these data show that the addition of dicamba with glyphosate or clethodim applied with labeled nozzles and DRA is resulting in reduced junglerice control and should be avoided.
Acknowledgments
We thank Syngenta for providing us the access to conduct research at their facilities in Vero Beach, Florida. In addition, we especially thank Ethan Parker, Marshall Hay, Gracee Hendrix, and all employees at the Vero Beach research station for their assistance and guidance throughout this process. We also thank the support staff and technicians at the University of Tennessee West Tennessee Research and Education Center for their assistance. This research was funded in part by the Tennessee Soybean Promotion Board and Cotton Incorporated. No other conflicts of interest are noted.