Introduction
From the middle of the 1st century BC, the Lower Rhine became the focus of Roman expansion plans. Although initially not intended to be an external border of the Roman Empire, the first military camps were established on the left bank of the Rhine early on: the Nijmegen army camp (19 BC) and, a few years later, the camps near Neuss and Moers-Asberg (Polak & Kooistra, Reference Polak and Kooistra2013). They were initially intended as a basis for further expansions to the east and north. However, after these plans failed as a result of the defeat of the Roman army under Drusus (9 BC), the Lower Rhine and the Rhine Delta developed into the first river frontier of the Roman Empire. It separated the Roman province of Germania inferior from the not occupied area on the right bank of the river (Germania magna). Until about the middle of the 1st century AD, the entire Lower Rhine and the Rhine Delta were being developed as a river Limes and army camps as well as civilian settlements lined its left bank (Bödecker, Reference Bödecker, Claßen, Rind, Schürmann and Trier2021; Bechert & Willems, Reference Bechert and Willems1995; Breeze, Reference Breeze2011; van Dinter, Reference van Dinter2013; Polak & Kooistra, Reference Polak and Kooistra2013; Polak et al., Reference Polak, Bödecker, Berger, Zandstra and Leene2020) (see Fig. 1). The Romans did not necessarily regard the Rhine valley as a strict borderline but rather as a permeable wide corridor and march area and, above all, as a very important trade route, with the help of which the military and civilians on the Rhine and in the provinces could be supplied with goods (Bödecker, Reference Bödecker, Claßen, Rind, Schürmann and Trier2021; Polak et al., Reference Polak, Bödecker, Berger, Zandstra and Leene2020; Breeze, Reference Breeze2011; Whittaker, Reference Whittaker1994; Rothenhöfer, Reference Rothenhöfer2005). Most of the camps and settlements along the Rhine (e.g. Cologne and Xanten CUT) were therefore built on the edge of the floodplain with direct access to the water and mooring facilities. It can be assumed that both the camps and the cities had harbours that ensured shipping all year round, even at low water level (Mirschenz et al., Reference Mirschenz, Gerlach, Bödecker, Mirschenz, Gerlach and Bemmann2019). So far, only one (genuine) harbour facility, a quay in front of the Colonia Ulpia Traiana (CUT), has been archaeologically ascertained (von Petrikovits, Reference von Petrikovits1952; Leih, Reference Leih, Müller, Schalles and Zieling2008; Selke, Reference Selke, Mirschenz, Gerlach and Bemmann2019). Other wooden structures interpreted as harbour facilities on the banks of the Lower Rhine, however, show characteristics of bank protection systems (see below).

Fig. 1. The Lower German Limes: World Heritage Sites (D/NL) (modified after Bödecker, Reference Bödecker, Claßen, Rind, Schürmann and Trier2021: 46; graphics by Christoph Duntze LVR-RLMB).
After the heyday of the province of Germania inferior, which lasted for about 200 years, the Late Antiquity crisis began in the Western Roman Empire by the end of the 3rd century. The Lower Rhine Limes were threatened by recurring incursions of the Franks, and the Limes now became a frontier that had to be defended constantly, as evidenced by the establishment of new fortified structures (Bödecker, Reference Bödecker, Claßen, Rind, Schürmann and Trier2021; Polak et al., Reference Polak, Bödecker, Berger, Zandstra and Leene2020; Bechert & Willems, Reference Bechert and Willems1995).
Taking a closer look at the location of preserved Roman sites along the Lower Rhine, most of them are located on the Lower Terrace (the so-called high bank) above an undercut bank of a Holocene meander or bend (Fig. 2). An explanatory model for this location, widely accepted in archaeology and geosciences since the beginning of the 1950s, was that Roman settlements and military camps along the Rhine were built at the high bank of abandoned meanders (oxbow lakes) or low-flowing tributary channels, offering a sheltered location for the (requisite) harbour (e.g. Hoppe, Reference Hoppe1970). A location along the active river, following this line of thought, would have meant continuous damage and ultimately the destruction of infrastructure due to the impact of high energy water masses. The present day position of Roman sites like Moers-Asberg, Xanten (CUT), Kalkar-Burginatium or Qualburg direct at the outside edge of the bends of former meanders was considered to be enough evidence for the “abandoned channel” paradigm (e.g.: Tischler, Reference Tischler1952; von Petrikovits, Reference von Petrikovits1952; Steeger, Reference Steeger and von Petrikovits1952; Hoppe, Reference Hoppe1970; Bechert, Reference Bechert1989; Klostermann, Reference Klostermann1986, Reference Klostermann1989; Teunissen, Reference Teunissen1990: 58–68; Knörzer et al., Reference Knörzer, Leichtle, Meurers-Balke and Neidhöfer1994). The location on an oxbow lake was seen as a prerequisite for safe navigability. It was assumed that such oxbow lakes remained connected to the new course of the river for a long time and would have contained a sufficiently high water level to maintain shipping, urgently needed for trade and military activities. However, this is very unlikely, since such abandoned meander channels silt up very quickly at their upstream and later at their downstream ends due to plug bar formation (Lange, Reference Lange1978; Kleinhans & van den Berg, Reference Kleinhans and van den Berg2011; Toonen et al., Reference Toonen, Kleinhans and Cohen2012). Reliable and permanent accessibility for shipping was therefore not guaranteed. In addition, there was the lower discharge; as a result, Roggenkamp (Reference Roggenkamp2016) concluded that the main problem in Roman navigation was not the violence of the river, but the low water level (see below).

Fig. 2. (a) The Lower Rhine downstream of the Wupper, with sites of the Lower German Limes and Limes road (black line). Red outline = Holocene floodplain after Geological Map 1:100.000 (Geologischer Dienst NRW). (b) Holocene floodplain terraces [GIS: Irmela Herzog, Reiner Lubberich LVR-ABR, Database: Geobasis NRW, LIDAR DEM 1 (executed at 25 × 25 m grid)].
A reassessment of boreholes and palynological data from the river bend at the Colonia Ulpia Traiana (CUT) near Xanten has yielded new insights into this debate. Long seen as an abandoned channel during the Roman period (von Petrikovits, Reference von Petrikovits1952; Steeger, Reference Steeger and von Petrikovits1952; Klostermann, Reference Klostermann1989: 102), two observations lead to reconsideration. Firstly, the presence of Roman finds embedded in the gravels of the river bed shows that the Roman city was situated directly along the active main stream of the Rhine and was even deliberately built on the steep undercut-bank of the bend (Charlier & Leih, Reference Charlier and Leih1996; Gerlach, Reference Gerlach1995). Secondly, this view is supported by the re-evaluation of the sedimentological and archaeobotanical data, showing that the silting up of the riverbed occurred no earlier than the Late Antiquity (Gerlach et al., Reference Gerlach, Herchenbach and Meurers-Balke2016b). This all indicates that the CUT was actually founded at the undercut bank of a main stream of the Rhine and this part of the river transformed into a dead channel only later, during Late Antiquity.
Consequently, it can be proven that the Romans were able to cope with the dynamic processes on the active undercut slope of a large meandering river and were able to take advantage of this typical topographical situation along the Lower Rhine. In this article, we explore the extent to which this observation can be verified or declined – and at which point in time the meanders silted up – using the other Roman sites on the Lower Rhine, some of which were located on far more sinuous meanders. To do this, the incomplete database had to be supplemented first.
Material and methods
Outline of the Rhine-Limes-Project
The Dutch-German initiative to apply for the Lower German Limes as a World Heritage Site set off a large amount of research around the Rhine since 2005, the river that was both the frontier of the Roman Empire and an important trade route. The reconstruction of its course, the fluvial dynamics and the hydrological status of the ancient Lower Rhine (Germany) as well as the reconstruction of its floodplain were the central geoarchaeological and palynological research items in this "Rhine-Limes Project".
At the start of the project, a database of sufficient quantity and quality for the entire Lower Rhine was not yet available. Therefore, various data on the palaeogeography of the Rhine plain from secondary and primary sources were compiled, reassessed and integrated in GIS (MapInfo) in a meta-study. In addition to literary sources, data came from the archives and databases of the LVR-Amt für Bodendenkmalpflege im Rheinland (Heritage Authority of Rhineland) (archaeological database BODEON), the Geologischer Dienst Nordrhein-Westfalen (Geological Survey NRW) (borehole logs: DABO database https://www.bohrungen.nrw.de/ and palynological data) and the Institut für Ur- und Frühgeschichte, Universität zu Köln (Department of Prehistoric Archaeology of the University of Cologne) (palynological and dendrochronological data). Initially, the focus was on the simple age delimitation of floodplain areas and palaeomeanders. Which of them existed already in Roman times and which were younger (Gerlach et al., Reference Gerlach, Becker, Meurers-Balke, Herzog and Thiel2008)? All further evaluations, hypotheses and results – as well as this article – then resulted from this growing body of data. However, this database is not to be seen as completed, because new results from excavations or drillings are supplementing and correcting the existing data over and over. They will also do so in the future. These data and the already existing geological floodplain mappings on different scales and with different stratigraphic resolutions were brought together and formed the basis of a new map for the floodplain of the Lower Rhine. This is used as the background for some of the figures and is based methodically and cartographically on the model of Berendsen & Stouthamer (Reference Berendsen and Stouthamer2001) and its update by Cohen & Stouthamer (Reference Cohen and Stouthamer2012). The exact morphological delimitation and relative stratigraphy of the individual meanders and floodplain terraces were checked and corrected (cross-cutting principles) using the relief features in the Lidar derived Digital Elevation Model (DEM). We use the DEM 10 (10 m grid) edition 2005–2011 from Geobasis NRW, archived in the LVR-Amt für Bodendenkmalpflege im Rheinland and the DEM 1 (1 m grid) (https://www.opengeodata.nrw.de/produkte/geobasis/hm/dgm1_xyz). The DEM also enables a clear identification of the last stage of the meander before its cut-off, because not all oxbow lakes can be identified as such today by means of an oxbow fen or typical channel filling (see below, Alpen-Drüpt). A more precise chronological fixing of the individual floodplain terraces (see below) was made primarily on the basis of archaeological finds (database BODEON) as well as on the basis of published and unpublished 14C, OSL and dendrochronological data. All 14C data were calibrated in calendar years with Oxcal 4.4, IntCal 20 (1 sigma) (van der Plicht et al., Reference van der Plicht, Bronk Ramsey, Heaton, Scott and Talamo2020). Besides, historical maps and written sources were used for more recent periods.
Dating the palaeomeanders
The focus of this study, however, is not on the floodplain terraces but on the palaeomeanders. There is often an abrupt change from the fluvial clastic sediments (sand and gravel) below to the fine and organic-rich abandoned channel fill sediments as a result of a sudden cut-off of the meander. By dating the beginning of the organic-rich fill, the end of the previous active fluvial phase is also determined (Berendsen & Stouthamer, Reference Berendsen and Stouthamer2001). After the cut-off, the meander ends are plugged with sediment by the river and beyond the plug bars a still water phase with sedimentation begins. Especially in the residual pool at the tip of the meander (oxbow lake) silty to clayey sediments, upwards increasingly organic up to fen peat layers, are deposited. In those wet and fine grained sediments, pollen and other plant remains are usually well preserved (e. g. Bos et al., Reference Bos, Dambeck, Kalis, Schweizer and Thiemeyer2008; Toonen et al., Reference Toonen, Kleinhans and Cohen2012) and can be dated palynologically or radiometrically.
When interpreting 14C dates on plant remains or sediments from Rhine deposits, two sources of error must be taken into account: reworked allochthonous plant material and the hard-water effect.
-
i. Fluvial deposits are often “contaminated” with older plant remains due to the reworking of older sediments by the river. This can lead to misinterpretations, as in the case of the boreholes in front of the CUT near Xanten (see below).
-
ii. Due to the calcareous water of the Rhine, the 14C content in water- and shore plants is lowered by the so-called hard-water effect, resulting in wrong 14C-derived ages. The difficulties of evaluating 14C-dates from Dutch Rhine sediments were already observed by D. Teunissen: “the lime-rich water of the Rhine has obviously made the 14C data too old” (Teunissen, Reference Teunissen1986). In the 1980s, the extent of this hard-water-effect was still unknown. Only in 2002, H. van der Plicht was able to quantify it at around 1200 14C-years (Cappers et al., Reference Cappers, Bottema, Woldring, van der Plicht, Streurman, Cappers and Bottema2002). With this correction factor in mind, a 14C-dated pollen diagram from the Rhine plain in the Netherlands near Horssen-Laagveld was plausibly reconciled with the known chronology of the settlement history (Kalis et al., Reference Kalis, Karg, Meurers-Balke, Teunissen-van Oorschot, Müller, Schalles and Zieling2008, see also Bos et al., Reference Bos, Dambeck, Kalis, Schweizer and Thiemeyer2008).
A further verification of the vegetation-historical dating through 14C dating is of course no longer possible when using archive data, nor was it absolutely necessary for our study.
A suitable dating method is the relative chronological assignment of palynological data (e. g. Bos et al., Reference Bos, Dambeck, Kalis, Schweizer and Thiemeyer2008; Erkens, Reference Erkens2009), by comparing pollen spectra from the fills with well-dated standard profiles from the region (see below, Xanten Tricensima). Therefore, original counting protocols of pollen analyses from the archives of the Geological Survey NRW and the Archaeobotany Laboratory of the University of Cologne were reassessed, standardised and redrawn in the Tilia program (Grimm, Reference Grimm2004), so that they could be better stratified in terms of their vegetation history (for method see also Bos et al., Reference Bos, Dambeck, Kalis, Schweizer and Thiemeyer2008). The samples from the Geological Survey NRW were taken in the field from boreholes or outcrops in order to obtain an initial estimation of the age of the fine-grained, noticeable organic-rich silting layers in a former river branch or meander. In addition, some new data were obtained by us from boreholes in former meanders of the Rhine to further clarify the pollen stratigraphy (e. g. Burginatium meander, see below). The new samples were processed (following Faegri & Iversen, Reference Faegri and Iversen1989) and analysed in the Archaeobotany Laboratory of the University of Cologne.
The accompanying sedimentological descriptions can either be taken from the profile sketches or are availabe in the reports of the pollen laboratories in Cologne and Krefeld, or – in the case of some boreholes – complete strata lists are available from the Geological Survey (DABO database).
In this paper, we use the nomenclature and periodisation from the LVR-Heritage Authority’s (LVR-ABR) database BODEON (Table 1). The chronological limits differ only slightly from those of the Dutch Archeological Basic Register (e.g. Pierik & van Lanen, Reference Pierik and van Lanen2019).
Table 1. Roman periodisation based on the archaeological database in the Rhineland BODEON (LVR-Amt für Bodendenkmalpflege im Rheinland / LVR-ABR).

The term "Late Antiquity" refers to the period that encompasses the end of the 3rd century to AD 700. The “Migration Period” is also defined in the BODEON database covering a period from Late Antiquity to the Early Middle Ages (AD 375–568). However, the terms “Migration Period” and “Dark Ages” are not common in Rhenish archaeology and are therefore not used here either.
Landscape setting
The Lower Rhine is the river between the northern border of the city of Bonn (the mouth of the River Sieg) and the Dutch border (Fig. 1). Here, the river flows with a low gradient in its own clastic deposits of the glacial Lower Terraces (descriptions see by Klostermann, Reference Klostermann1992 and Schirmer, Reference Schirmer1995) or – near Kleve and Elten – through ice-pushed ridges from the penultimate glacial period and thus has the opportunity to shape and reshape the floodplain – its natural activity area in the Holocene – by shifting the river bed, mainly by meander migration.
How deep the river can incise, how wide the Holocene floodplain is and how strongly the river is tending to form meanders depends as a general rule, among other things, on the fluvial gradient and the erosion resistance of the Lower Terrace sediments, both of which are decreasing towards the north (Brunnacker, Reference Brunnacker1978: 436; Roggenkamp, Reference Roggenkamp2016: 27).
The river gradient between Bonn and Cologne is still relatively steep (0.023%). As a result, only a narrow Holocene floodplain (width 0.5–1 km) with a few active meanders but without palaeomeanders has formed in this section (width of the Holocene floodplain according to the geological map 1: 100,000 of the Geological Survey NRW, see also Fig. 2). A wider floodplain (3–4 km), now with abandoned meanders (meander belt), begins north of the mouth of the River Wupper near Cologne-Worringen. Downstream of the mouth of the River Ruhr near Duisburg, the meander belt expands considerably (7–12 km), which is account of the significant lower gradient (0.015–0.008%) and the more sandy and easily erodible Lower Terraces (Brunnacker, Reference Brunnacker1978: 436; Roggenkamp, Reference Roggenkamp2016: 27). As a result, the size and number of present-day and abandoned meanders increase downstream. Near the border with the Netherlands, the gradient is only 0.008%. Here, with the bifurcation of Rhine and Waal, the meandering river system of the Lower Rhine Plain ends and the multiple channel Delta-Rhine begins. In a geological sense, the apex of the Delta begins near Rees with the tectonically stable hinge zone between the (modest) uplifting tendency of the Lower Rhine Plain and the subsiding North Sea Basin (Stouthamer & Berendsen, Reference Stouthamer and Berendsen2000, Reference Stouthamer and Berendsen2001; Erkens et al., Reference Erkens, Hoffmann, Gerlach and Klostermann2011). The tectonic influences on the Holocene river history are, however, rather insignificant (Klostermann, Reference Klostermann1992; Erkens et al., Reference Erkens, Hoffmann, Gerlach and Klostermann2011).
Since the beginning of the Holocene, the floodplain is the area most affected by floods, settlements are mostly located on the higher, largely flood-free Lower Terraces, preferably directly at the edge along the floodplain. In the northern part of the Lower Rhine, the older Holocene terraces, which are only slightly higher, also served as settlement sites.
Holocene floodplain terraces at the Lower Rhine
The Holocene floodplain of the Lower Rhine consists of land areas of different ages (Auenterrassen, sensu Schirmer, Reference Schirmer1983, Reference Schirmer1995), which were primarily formed by reworking of clastic sediments as a result of meandering (Figs. 2 and 3). The palaeomeanders mainly form a loop terrace pattern, which is typical for a river type with predominantly high sinuosity meanders (Schirmer, Reference Schirmer1995; Schirmer et al., Reference Schirmer, Bos, Dambeck, Hinderer, Preston, Schulte, Schwalb, Wessels, Herget and Dikau2005). During meander migration activities, older deposits (Lower Terraces or older Holocene Terraces) were reworked and relocated whereby the sediments on the undercut bank of the meander were eroded and deposited at the point bar inside of the meander (Fig. 4). This sediment rearrangement ends when the meander bend breaks through (neck- or chute-cutoff) or is artificially cut-off. The new shortened river course deepens and a floodplain terrace remains behind in the interior of the abandoned meander. In addition, channels of a more anabranching section of a river with lower sinuosity can also shift, leaving behind new land (seam terraces, see Fig. 3). As a result, the Holocene floodplain consists of a complex mosaic of terraces, which can be differentiated from each other morphologically, lithologically, pedologically and by cross-cutting relations. Their age can be estimated by archaeological remains or archaeobotanical data (pollen analysis or dendrochronology) and by geochronological dating (14C, OSL) (Schirmer, Reference Schirmer1983, Reference Schirmer1995; Klostermann, Reference Klostermann1989, Reference Klostermann1992; Zhou, Reference Zhou2000; Shala, Reference Shala2001; Berendsen & Stouthamer, Reference Berendsen and Stouthamer2001; Erkens, Reference Erkens2009). In Fig. 2, the existing geological surveys and dates of the Holocene floodplain terraces were compiled, their morphology verified (by using Digital Elevation Models) and, if necessary, re-dated.
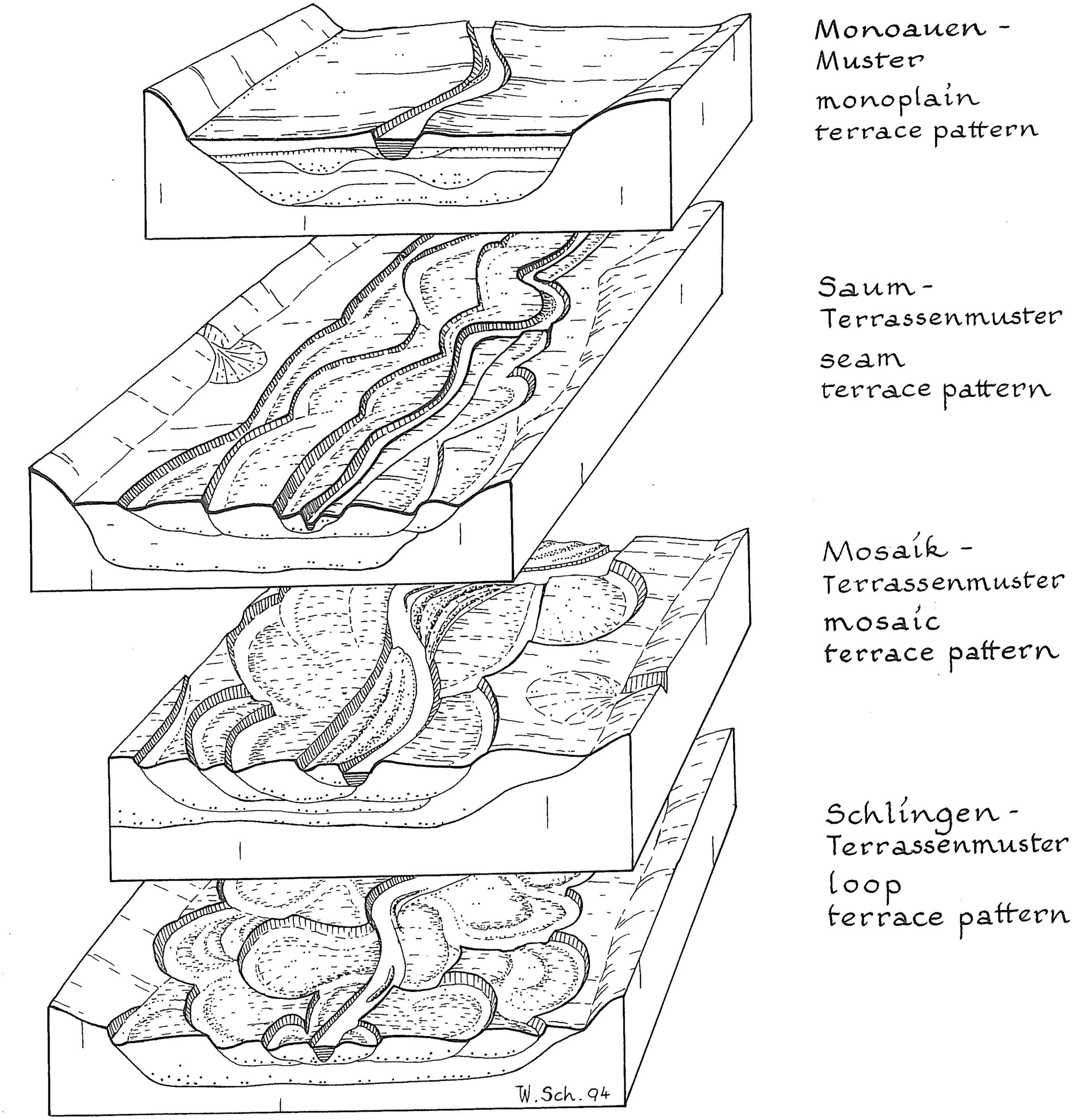
Fig. 3. Terrace patterns of Holocene valley bottoms in Middle Europe (Schirmer, Reference Schirmer1995: 32 and Schirmer et al., Reference Schirmer, Bos, Dambeck, Hinderer, Preston, Schulte, Schwalb, Wessels, Herget and Dikau2005: 203).

Fig. 4. Block diagram of the Holocene floodplain. (1 = oldest, 3 = youngest floodplain terrace) (modified after Schirmer, Reference Schirmer1995: 29 and Schirmer et al., Reference Schirmer, Bos, Dambeck, Hinderer, Preston, Schulte, Schwalb, Wessels, Herget and Dikau2005: 200).
The Rhine was forming pronounced meanders with a high sinuosity during Prehistoric times. Beginning in the later Iron Age and increasingly during ancient and historical times, the river also divided into channels at bars and islands (anabranching), so that a mixture of meanders and branched river sections arose (Klostermann, Reference Klostermann1989: 92–105, Reference Klostermann1992: 175, Schirmer, Reference Schirmer1991, Reference Schirmer1995). The channel then widened and the channel base rose. This tendency towards anabranching is assumed to be caused by an increase in sediment load due to increasing land clearing since the Iron Age (Schirmer, Reference Schirmer1991; Schirmer et al., Reference Schirmer, Bos, Dambeck, Hinderer, Preston, Schulte, Schwalb, Wessels, Herget and Dikau2005; Hoffmann et al., Reference Hoffmann, Erkens, Gerlach, Klostermann and Lang2009; Erkens et al., Reference Erkens, Hoffmann, Gerlach and Klostermann2011). However, despite these changes in river dynamics, the river continues to be meandering (Schirmer, Reference Schirmer1995: 46).
Focusing on the Roman era, three clustered floodplain terraces could be mapped (Fig. 2):
-
1. Pre-Roman floodplain terraces (prehistoric terraces), which were already established and – if a few decimetres higher – could serve as settlement areas in Roman times and thus had a usage potential comparable to that of the Lower Terraces.
-
2. Areas formed in Roman times by coeval meanders/river branches. Depending on the quality of the archaeological data, transitional areas such as “pre-Roman to Roman” or “Roman to post-Roman” areas could also be mapped.
-
3. Post-Roman floodplain terraces. Their formation destroyed existing older land surfaces including all archaeological sites. In situ archaeological finds from Roman and older times no longer exist there, but more or less relocated finds may be found embedded in the gravel.
Hydrological state of the Roman Rhine
The characteristics of the floodplain and the palaeomeanders already gave first indications of the possible condition of the Rhine in Roman times as being wider, flatter and more branched. Thomas Roggenkamp (Reference Roggenkamp2016) and Roggenkamp & Herget (Reference Roggenkamp and Herget2016) were able to provide more specific hydrological data. On the base of written sources from Antiquity as well as from archaeological and archaeobotanical data, he modelled Roman Age water levels and average discharge (m3/s) rates of the Middle and Lower Rhine. He showed that the discharge of the river in Roman times was about half of the present one. Compared to today’s water level, the one of the Lower Rhine was 2 m lower in Roman times. This might be explained by the still largely undisturbed existence of fen peats on the Upper Rhine Plain and the more extensive woodland cover in the catchment area, retaining large amounts of water (Roggenkamp, Reference Roggenkamp2016; Roggenkamp & Herget, Reference Roggenkamp and Herget2016). To what extent climatic fluctuations may also have been involved in the low water level is yet not solved. According to climate reconstructions, the period of Roman expansion was characterised by a relatively warm and dry “climatic optimum” (Schönwiese, Reference Schönwiese2003), but the point in time this “optimum” ended, whether during the course of Late Antiquity or earlier, is still debated (Roggenkamp, Reference Roggenkamp2016: 45 f.). According to some authors, a significant drop in temperature should have started as early as the middle of the 2nd century (Klostermann Reference Klostermann, Müller, Schalles and Zieling2008a, b; McCormick et al., Reference McCormick, Büntgen, Cane, Cook, Harper, Huybers, Litt, Manning, Mayewski, More, Nicolussi and Tegel2012: 174 ff.). In contrast, Roggenkamp, based on the documentation of the remains of the Constantinian bridge between Cologne (CCAA) and its bridgehead Deutz (built in AD 310), was able to show that the Rhine continued to maintain the above-mentioned run-off deficit at the beginning of the 4th century, i.e. at a time when the climate should already have been significantly cooler (Roggenkamp, Reference Roggenkamp2016: 174; Roggenkamp & Herget, Reference Roggenkamp and Herget2016). There is even no evidence that larger floods, such as those documented for the Rhine-Maas delta during the middle Roman Age (as between AD 185 and 282, Jansma, Reference Jansma2020), led to meander cut-offs in the Lower Rhine (see below).
This reconstruction of the hydrological conditions in the Roman age strengthens the assumptions made on the basis of the floodplain terrace geology and leads to an image of the Lower Rhine that differs significantly from the present one with its historical river regulations: a relatively shallow, broad river that is often split up into multiple channels by bars and islands. Indeed, at around AD 98, Tacitus describes the Lower Rhine (up to the channel division at the Batavian Island in the Rhine Delta) (Verhagen et al., Reference Verhagen, Kluiving, Anker, van Leeuwen and Prins2017: fig. 2, 9; Polak & Kooistra, Reference Polak and Kooistra2013: 390) as a watercourse (uno alveo) interspersed with small islands (modicas insulas circumveniens): Tac. ann. 2.6.
With regard to the function of the Lower Rhine as a military and economic waterway, Roggenkamp (Reference Roggenkamp2016) concluded that the low water level must have been a serious navigational problem, persisting into the Middle Ages and early modern times. According to Jasmund (Reference Jasmund1898: 21), 25 Rhine islands existed in the Kleve district alone (the Lower Rhine from Moers to the Dutch border) in the 18th century, dividing the river into many branches. The river channel regulations carried out since then had the explicit aim of narrowing and thus deepening the stream bed (Jasmund, Reference Jasmund1901).
Results and Interpretation
A key site for the Late Antiquity vegetation history: Tricensima, a silted up defensive trench on the Lower Terrace
To answer the question of when the silting up of Rhine meanders began, the Late Antiquity vegetation history became of paramount importance. The database for this period, however, was very poor for the Lower Rhine. Detailed pollen diagrams were available only from the Maas floodplain (Teunissen, Reference Teunissen1990). For the German side, there was one pollen diagram that was unambiguously dated by archaeological and radiometric methods and stemmed from outside the floodplain on the Lower Terrace of the Rhine: that from the defensive trench of the Tricensima of the CUT (Kalis et al., Reference Kalis, Karg, Meurers-Balke, Teunissen-van Oorschot, Müller, Schalles and Zieling2008). This high-resolution diagram enabled us – together with data from the Maas Valley – to recognise pollen spectra from Late Antiquity in the archive data (diagrams and counting protocols) from the Rhine floodplain and to develop a relative chronology.
The Tricensima was a Roman fortress that, according to the archaeological finds and features, was built at the end of the 3rd or beginning of the 4th century in the middle of the urban area of the CUT (see Fig. 5). At around AD 400, the fortress was abandoned and the settlement moved to the Xanten cathedral hill, further south (Otten & Ristow, Reference Otten, Ristow, Müller, Schalles and Zieling2008; Zerl et al., Reference Zerl, Meurers-Balke and Kalis2021). The function and construction of the Tricensima is archaeologically well described. The fortress was enclosed by a defence trench of approximately 3.5 m depth. The trench was cleared regularly until the moment the fortress lost its military function, around the end of the 4th / beginning of the 5th century. From that moment on, the filling of the ditch began (minerogenic filling and terrestrialisation = organic growth, Mueller-Dombois & Ellenberg, Reference Mueller-Dombois and Ellenberg1974). It started with a sandy gyttja, followed by fen peat and then by different levelling layers. Nowadays, it is completely filled, but only its bottom layer of about 30 cm (gyttja and peat) contains pollen and other plant remains in excellent preservation. During an excavation by the University of Nijmegen, a pollen profile was taken and later palynologically analysed at the Archaeobotanical Laboratory of the University of Cologne (Fig. 6). The pollen diagram reveals the vegetation history from the final phase of the Roman presence until the beginning of the early medieval re-settlement. It clearly shows how the Late Antiquity crisis is reflected in the pollen spectra. The chronology is corroborated by three 14C dates (Table 2). The lowermost date, from the sandy gyttja, 1702 ± 50 BP, calibrated AD 330–412, limits, in accordance with the archaeological data, the start of the silting to the first decade of the 5th century (Zerl et al., Reference Zerl, Meurers-Balke and Kalis2021).

Fig. 5. Area around Alpen, Wesel, Xanten and the mouth of the Lippe. (a) Relief map (LIDAR DEM) with structures of the Lower German Limes (camps, roads, CUT). (b) Holocene floodplain terraces and data points (outlined dots = explanation in text). 1 = Xanten: Colonia Ulpia Traiana (CUT) and Tricensima in the center. 2 = Xanten Fürstenberg: Castra Vetera I. 3 = Xanten: Castra Vetera II (presumed, gravel pit finds). 4 = Alpen: temporary camps. 5 = Alpen-Drüpt: auxiliary fort (south, partially eroded) and large temporary camps (north). 6 = Wesel-Flüren: temporary camps. 7 = Wesel Aue, site of prehistoric finds in the year 1936 (bronze axe and arm rings, Hallstatt B 1000–800 BC) in alluvial loam (modified after Gerlach et al., Reference Gerlach, Meurers-Balke and Kalis2020, GIS: Irmela Herzog, Reiner Lubberich LVR-ABR, Database: Geobasis NRW, LIDAR DEM 10, Limes sites after Polak et al., Reference Polak, Bödecker, Berger, Zandstra and Leene2020).

Fig. 6. Pollen diagram Xanten Tricensima. 1 = fillings. 2 = peat, peat-lined gyttja. 3 = fine sandy gyttja. 4 = humous sand (modified after Kalis et al., Reference Kalis, Karg, Meurers-Balke, Teunissen-van Oorschot, Müller, Schalles and Zieling2008, graphics by H. Schluse Univ. Köln).
Table 2. 14 C data (Xanten and Wesel) mentioned in the text.

The lower spectra show the vegetation during the final phase of the Tricensima, with tree pollen characterised by the pioneer species birch (Betula), alder (Alnus glutinosa) and willow (Salix), pointing at bush encroachment at the abandoned urban territory. Herb pollen comprising ruderal and grassland species is likely to have originated partly from the pioneer vegetation on the rubble of the town, too. It was not until the second half of the 4th century that open land indicators declined rapidly and the increasing tree pollen spectrum shows all features of natural reforestation. In the ditch itself, a willow bush developed. In the wider surroundings firstly birch (Betula) and hazel (Corylus avellana) spread, followed by oak (Quercus), beech (Fagus sylvatica), elm (Ulmus), lime (Tilia) and ash (Fraxinus excelsior). Hornbeam (Carpinus betulus) could establish itself here for the first time in the woodlands. From the 5th century on the Lower Rhine area was widely forested. However, the area was not entirely uninhabitated because pollen grains of cereals appear in the pollen spectrum from around AD 450 onwards, indicating agriculture. The cereal pollen curve increases around 600 together with that of grass pollen, indicating the Early Medieval resettlement in the area around the town of Xanten.
Although the Late Antiquity/Early Medieval vegetation development as shown in the Tricensima pollen diagram represents the Lower Terrace, it can serve as a blueprint and be transferred to the immediate adjacent floodplain of the Rhine. It was the main base for the relative chronological classification of the palynological results from the various other data sources and archives.
Kalkar-Burginatium, a quietly silted-up oxbow-lake
A largely undisturbed organically rich silting-up sequence was encountered in a 10 m deep borehole (BUR 1) at the tip of a former Rhine meander in front of the Burginatium Roman cavalry camp (near Kalkar) (Fig. 7). This palaeomeander is drained today by the Leybach. The borehole profile starts with the underlying sandy-gravelly sediments which were deposited by the active river. After the meander had been cut-off, an oxbow lake remained, which silted up with fine sandy, silty and clayey sediments. Silting-up began at a depth of 7.6 m and from there on upwards pollen samples were taken and analysed (Gerlach & Meurers-Balke, Reference Gerlach, Meurers-Balke and Kennecke2014; Gerlach et al., Reference Gerlach, Fischer, Meurers-Balke, Mirschenz, Röpke, Hadler, Willershäuser, Vött, Mirschenz, Gerlach and Bemmann2019) (Figs. 8 and 9).

Fig. 7. Area around Kalkar. (a) Relief map (LIDAR DEM) with structures of the Lower Germania Limes (camps, roads). (b) Holocene floodplain terraces and data points (outlined dots = explanation in text). 1 = Till-Moyland (Steincheshof): auxiliary fort. 2 = Kalkarberg: sanctuary. 3 = Kalkar-Burginatium: cavalry fort Burginatium (fleet base?). 4 = Kalkar-Hönnepel: auxiliary fort (presumed, gravel pit finds). 5 = Xanten-Vynen: auxiliary fort. 6 = Uedem-Hochwald: temporary camps (GIS: Irmela Herzog, Reiner Lubberich LVR-ABR, Database: Geobasis NRW, LIDAR DEM 10, Limes sites after Polak et al., Reference Polak, Bödecker, Berger, Zandstra and Leene2020).

Fig. 8. Kalkar-Burginatium, core lines (BUR 1) (No. = pollen samples from the organically rich silting-up sediments) (photo: Timo Willershäuser).

Fig. 9. Pollen diagram Kalkar-Burginatum, borehole BUR 1. 1 = clayey, fine sandy silt. 2 = anthropogenic mixed layer (gravelly-loamy sand, pieces of bricks and charcoal). 3 = fine gravelly sand (modified after Gerlach & Meurers-Balke, Reference Gerlach, Meurers-Balke and Kennecke2014, graphics by H. Schluse, Univ. Köln).
A pre-Roman age was expected, because the preservation of the remains of the army camp at the tip of the bend were once seen as evidence that the Rhine must have already left this meander in pre-Roman times (Hoppe, Reference Hoppe1970: 22). But the pollen spectra show a different picture. The lowest sample at 7.5 m was deposited at a time when beech (Fagus sylvatica) and hornbeam (Carpinus betulus) were growing in the area. Characteristic pollen grains of Roman agriculture, however, were missing. Alder (Alnus glutinosa) and fern values between 15 and 20% indicate that the shore areas were covered with bushes. All this suggests that silting-up began at its earliest in Late Antiquity.
During the early siltation phase (between 7 m and 3 m depth), floods of the Rhine initially still regularly reached the oxbow lake, with long-distance water-transported pollen types (Picea abies, Abies alba) being washed in. Spruce and fir have their nearest natural occurrence in the Black Forest, Vosges, Alpine foothills and in the Fichtelgebirge. The upper four pollen samples differ significantly from the lower ones. In particular, pollen of grassland species indicates the presence of extended meadow and pasture land in the vicinity. Since pollen grains of spruce and fir are missing, the influx of Rhine floods must have been prevented. The construction of dykes, which shut off the meander bend from the Rhine floods, began in Medieval times. Water and riparian plant species were able to spread in the calm water of the oxbow lake and terrestrialisation began. This process must have ended before the 19th century, because the large-scale afforestation with conifers in the region is not recorded in the pollen spectra.
The pollen analytical dating of the beginning of the silting-up of this meander in Late Antiquity shows that the Roman army camp, which existed from Augustine times until the end of the Roman military presence in Germania Inferior in the 5th century AD, was located on an active Rhine meander. But its position directly on the undercut bank of the active river did not remain without consequences. The northeastern corner of the camp was damaged and repaired, probably after demolition by cut-bank erosion at the tip of the meander (Fig. 10, Berkel et al., Reference Berkel, Bödecker, Brüggler, Otte, Mirschenz, Gerlach and Bemmann2019). The remains of wood and the accumulation of basalt stones found below the fort in the former undercut bank (preserved in the wetland environment of the Leybach) might even indicate the existence of a former bank protective wall (Gerlach et al., Reference Gerlach, Meurers-Balke and Herchenbach2016a).

Fig. 10. Kalkar Burginatium. Auxiliary Fort: ground plan, excavation sections and course of the antique riverbank. Phase 1 = wall (Early Roman period). Phase 2 = reinforcement of the wall (?). Phase 3 = demolition of the fort corner (Late Antique?). 2–8 = various erosion events and fillings. 9 = Leybach: Basalt and Tuff fragments, wood remains. 10 = Leybach sediments (alluvial loam) (modified after Berkel et al., Reference Berkel, Bödecker, Brüggler, Otte, Mirschenz, Gerlach and Bemmann2019, GIS and graphics by Harald Berkel LVR-ABR).
The Roman Rhine in front of the CUT, bank demolitions and bank protection
The Rhine bend in front of the Colonia Ulpia Traiana (CUT), which today is used by a brook called Pistley, is, in its archaeological context, one of the best studied. This abandoned bend, just opposite the Roman town gates, was examined by 150 boreholes (985 m of cores), from which 86 pollen samples and 50 samples of plant macro remains were taken and studied (Knörzer et al., Reference Knörzer, Leichtle, Meurers-Balke and Neidhöfer1994) (see Fig. 5, 1). In addition, archaeological excavations were carried out (Leih, Reference Leih, Müller, Schalles and Zieling2008; Selke, Reference Selke, Mirschenz, Gerlach and Bemmann2019). In this river channel, however, no quiet, undisturbed silting up process as in the case of Burginatium could be observed. Instead there was a complicated sequence of layers caused by natural bank erosion and anthropogenic fillings. The palynological investigations were initially focused on the fine-grained sediments, which were assumed to represent the Roman silting-up history of the channel. The underlying gravel and sands were considered to be pre-Roman according to the “abandoned channel paradigm” (see above, Knörzer et al., Reference Knörzer, Leichtle, Meurers-Balke and Neidhöfer1994). A further re-examination of the cores, however, revealed that these “pre-Roman” gravel layers (previously seen as sediments of the Lower Terrace) also contained Roman finds like brick fragments, ceramics and broken glass (Charlier & Leih, Reference Charlier and Leih1996). This contradiction led to a re-interpretation of the pollen analytical results of the silted-up sediments. Some fine-grained layers with pollen spectra characterised by high pollen values of Pinus, Picea, Abies and hornbeam (Carpinus) in absence of beech (Fagus), which could be attributed to the Eemian Interglacial played a key role. The presence of those Pleistocene deposits in several boreholes originally led to the conclusion that the Roman deposits must of course be above these layers.
In borehole H2, shown as an example (Fig. 11), the pollen spectra below the Eemian sediments were seen as an admixture of late-glacial and interglacial provenance, due to the presence of helophytic species, like buckthorn (Hippophae), rockrose (Helianthemum) and dwarf birch (Betula nana). But, apart from the fragments of bone, ceramics and bricks, there are also pollen analytical indications of a “Roman input”, namely pollen of Cerealia-type and flax (Linum usitatissimum). These had already been noticed during the initial examination, but were regarded as contamination due to coring procedures of the boreholes.

Fig. 11. Pollen diagram Xanten CUT, borehole H 2. 1 = alluvial loam, gravel, pieces of bricks and charcoal, etc. 2 = anthropogenic mixed layers (clay, sand, gravel, building rubble, organic waste, pieces of bricks and charcoal, etc.), loamy silt bands. 3 = silty clay. 4 = gravely sand, silt streaks, bricks and pieces of charcoal. 5 = sandy gravel, pieces of bricks and charcoal, ceramics, glass (modified after Gerlach et al., Reference Gerlach, Herchenbach and Meurers-Balke2016b, graphics by H. Schluse, Univ. Köln).
Such an admixture could occur by undercutting the steep slope of the meander, causing bank demolition (Knighton, Reference Knighton1998: 113ff). Rotnicki and Borowka (Reference Rotnicki and Borowka1984: 223) described such a situation where lumps of cohesive material were torn off from the steep bank in channel-fill deposits of a palaeomeander (Prosna river, Poland). In a borehole, such allochthonous impurities can hardly be distinguished from an autochthonous layer, which can lead to misleading interpretations of the borehole profile. As far as we know, this source of error is hardly taken into account in drilling investigations. According to the borehole profiles and palynological data, the outer, erosive bank of the river bend in front of the CUT was affected by land erosion on two levels:
-
i. Near the surface the bank was undercut and the lumps of humic topsoil (floodplain loam with artefacts and other household waste such as plant remains and charcoal) were torn down.
-
ii. At the same time, cohesive in situ layers from the Pleistocene (Eemian and glacial layers), hidden in the deeper underground near the erosive base, were torn off and the loamy material including preserved pollen were embedded in the Holocene fluvial sediments (2–3 m below the in situ Eemian layer). The incorporation of such Pleistocene loam clods and sharp-edged, fragile artefacts (such as broken glass) suggests that the material was more or less immediately embedded in the river gravel and hardly transported. The resulting difficulties in dating, in conjunction with the “abandoned channel paradigm”, led to the former misinterpretation.
Mapping the boreholes with gravels containing either Roman artefacts and/or Pleistocene plant remains allows an almost metre-accurate mapping of the erosion front and basis caused by Roman-age fluvial demolition events at the outer bank of the Rhine in front of the CUT (Fig. 12). It is unclear whether the erosion was a longer process or a single event. The town wall facing the Rhine front, with its suspiciuous kink, seems to take an undermined undercut slope into account.

Fig. 12. 3 D reconstruction of the Roman riverbank in front of the CUT (around AD 130) on the basis of 150 boreholes (red dots). According to the quay remains, the Roman mean water level was at 15.3 m a.s.l. (von Petrikovits, Reference von Petrikovits1952). Dashed line= undercut bank of the Roman river bend. Excavation sections with important riverbank features: 1 = quay (von Petrikovits, Reference von Petrikovits1952). 2 = ship house/ship ramp (Selke, Reference Selke, Mirschenz, Gerlach and Bemmann2019). 3 = bank protection construction “Packwerk” (Gerlach et al., Reference Gerlach, Meurers-Balke and Herchenbach2016a, Selke, Reference Selke, Mirschenz, Gerlach and Bemmann2019) (modified after Gerlach et al, Reference Gerlach, Herchenbach and Meurers-Balke2016b, GIS and graphics Sonja Groten LVR-ABR).
In any case, the Romans saw the need for protective measures, because sections of the undercut bank were secured by a massive bank protection (Fig. 13a, b) (Gerlach et al., Reference Gerlach, Herchenbach and Meurers-Balke2016b; Gerlach et al., Reference Gerlach, Fischer, Meurers-Balke, Mirschenz, Röpke, Hadler, Willershäuser, Vött, Mirschenz, Gerlach and Bemmann2019; Selke, Reference Selke, Mirschenz, Gerlach and Bemmann2019). It is a so-called “Packwerk”, an artificial earthen construction consolidated by posts, wattle, fascines, and diagonally hammered-down piles, as it was and still is used in nature oriented hydraulic engineering. The embankment was built before AD 90 (archaeological data) and reinforced with rows of oak posts between AD 130 and 150 (dendrochronological data) (Selke, Reference Selke, Mirschenz, Gerlach and Bemmann2019). This construction was discovered and documented in 1993 (LVR-Archäologischer Park Xanten, archive no. 93/16) but at that time it was still interpreted as part of the harbour (Leih, Reference Leih, Müller, Schalles and Zieling2008). The soil material of the Packwerk, which served as “Senkerde” (soil material to weigh down the wooden construction and fascines underwater), contained many finds: on the one hand terrestrial soil material with mixed artefacts from the settlement (Gerlach et al., Reference Gerlach, Fischer, Meurers-Balke, Mirschenz, Röpke, Hadler, Willershäuser, Vött, Mirschenz, Gerlach and Bemmann2019; Selke, Reference Selke, Mirschenz, Gerlach and Bemmann2019; Leih, Reference Leih, Müller, Schalles and Zieling2008), and on the other hand many plant remains. The latter (pollen und macro remains) were analysed in the Archaeobotanical Laboratory of the University of Cologne. The archaeobotanical analysis revealed a wealth of plants species, partly crop plants (e.g. remains from cereal processing) as waste from the nearby settlement, partly, however, species from river bank vegetation. The species assemblage was so numerous (more than 200 taxa) that specific plant communities could be identified: reeds interlocked with mud bank vegetation on nutrient-rich shores, which fall dry in summer. It is striking that such vegetation is not distributed on undercut banks but on the slip-off slopes on the opposite side of the river. In retrospect, a previously unexplainable pattern in the soil layers of the “Packwerk” could now also be explained (Fig. 13b). This pattern resulted from spade clods of loam with adhering above-surface plant remains of identifiable plant communities. Apparently these clods were dug out sods. They were called “caespites” in Roman times and primarily used by the Roman military as a universal building material. Pliny the Elder (AD 23/4-79) even explicitly describes the use of caespites as protection against the impact of rivers (Plin. n. hist. 35. 169). Here, too, caespites were used in the matrix of the bank protection construction, the “Packwerk” of the CUT. Moreover, even the provenance of the sods could be ascertained, thanks to their plant species content: they were dug out from the surface of a nearby point bar, possibly because of their quality as building material (Gerlach et al., Reference Gerlach, Meurers-Balke and Herchenbach2016a). In addition, humous topsoil with artefacts and terrestrial plant remains was used, although it can no longer be established whether this pertained to household waste layers or removed settlement soil (Leih, Reference Leih, Müller, Schalles and Zieling2008; Selke, Reference Selke, Mirschenz, Gerlach and Bemmann2019). A final renewal of the wooden construction took place between AD 130 and 150 (Selke, Reference Selke, Mirschenz, Gerlach and Bemmann2019: 285).

Fig. 13. Roman bank protection constructions. (a) Xanten CUT bank protection (“Packwerk”): square timber posts (right) and smaller piles in organic layers (dark) and light colored “Senkerde” (Gerlach et al., Reference Gerlach, Meurers-Balke and Herchenbach2016a, photo: LVR-APX by Bernd Münster). (b) Xanten CUT bank protection: detail from the earthen construction (below): the individual loamy spade clods (caespites) can still be recognised by the light stripes that correspond to the former humus top soil (Gerlach et al., Reference Gerlach, Meurers-Balke and Herchenbach2016a, photo: LVR-APX by Bernd Münster). (c) Moers-Asberg: rows of piles and soil infill (Gerlach et al., Reference Gerlach, Meurers-Balke and Herchenbach2016a, photo: LVR-ABR by Clive Bridger, NI 1993/0008). (d) Krefeld-Gellep: rows of piles with basalt stone fillings (Meurers-Balke et al., Reference Meurers-Balke, Herchenbach, Gerlach, Siepen, Kronsbein and Siepen2015: 239, fig. 1, photo: Stadtarchäologie Krefeld).

Fig. 14. Pollen diagram Wesel Kreishaus/county administration office (GD NRW 4305-19). 1 = clayey silt. 2 = alternation of sand and silt strata (graphics by H. Schluse, Univ. Köln).
Judging from descriptions in earlier excavation reports, similar bank protection constructions, in part with stone deposits instead of “Senkerde”, have apparently also been found at Krefeld-Gellep (Meurers-Balke et al., Reference Meurers-Balke, Herchenbach, Gerlach, Siepen, Kronsbein and Siepen2015), Moers-Asberg (dendrochronologically dated around AD 60–90) (Bridger, Reference Bridger1994; Gerlach et al., Reference Gerlach, Röpke, Kels and Meurers-Balke2017) and Burginatium (s. above, Gerlach et al., Reference Gerlach, Meurers-Balke and Herchenbach2016a), however, with far less archaeological and archaeobotanical evidence (see Fig. 13c, d). More archaeological evidence of Roman bank protection structures has been found further downstream along the Delta-Rhine, the so called Old Rhine. The excavations near the former farmstead “de Balije” in De Meern (Utrecht-Leidsche Rijn; Langeveld et al., Reference Langeveld, Luksen-IJtsma and Graafstal2010) produced many good examples of constructions protecting the limes road against the currents of the Roman Rhine. The protective measures include many elements that could also be identified on the Lower Rhine: wooden structures with backing of sods, stones, silt, posts, wattle, etc.
In the end, this bank protection found in Xanten, of which the extent, predecessors and expansion phases are not yet known, proofed to be so effective that the Roman city wall remained undamaged although the active Rhine stream remained in front of the CUT until Late Antiquity.
In the H2 borehole, plant-bearing deposits with Roman artefacts and plant remains lay on top of the “Eemian-age” loam clods (Fig. 11). However, as the pollen values of beech (Fagus sylvatica) and hornbeam (Carpinus betulus) show, these layers were not deposited in Roman times but in Late Antiquity, when these tree species already had established themselves in the woodlands of the Lower Rhine area. This means that the silting up of the abandoned channel began not until then. At the same time, woodland, rich in alder (Alnus glutinosa), began to spread along the river banks. The time around which this process began could be determined by radiometric dating. About 2 km downstream from the CUT, G. Erkens (Reference Erkens2009: 94 ff.; Erkens et al., Reference Erkens, Hoffmann, Gerlach and Klostermann2011) was able to recover terrestrial plant material at the bottom of the silted-up sediments of the abandoned Rhine channel (called the Pistley). The sediment began with an undisturbed terrestrialisation. Here, fragments of female catkins, a leaf fragment and bud scales of Alnus glutinosa, and a fruit of Rumex spec. (identification J.A.A. Bos) were recovered and selected for dating. The result was 1570 ± 50 BP, which when calibrated (Oxcal 4.4, IntCal 20) gives an age between AD 433 and 550 (1 sigma) (Table 2).
After Late Antiquity, there is a sedimentation gap in the CUT-profiles. The upper 4 m of the H2 core comprise sediments that were deposited only from Late Medieval up to Early Modern times. This is evidenced by the cultivation of rye (Secale cereale) and buckwheat (Fagopyrum esculentum) (e.g. Bunnik, Reference Bunnik1995) in the area, as testified by corresponding pollen records. The high pollen values of grasses and grassland species show that the floodplain and the surroundings of the former CUT now served as meadows and pastures for animal husbandry and the abandoned channel as a watering pool (Gerlach et al., Reference Gerlach, Herchenbach and Meurers-Balke2016b).
The Wesel-Flüren meander, a reactivation of a prehistoric meander loop
In addition to our own investigations and sampling activities, numerous borehole and pollen analytical data generated by the Geological Survey NRW as part of the geological mapping of the Rhine floodplain and its surroundings were made available to us (85 borehole or profile localities). While evaluating the pollen analytical data, we were surprised by the abundance of Late Antiquity pollen spectra (40 out of 85 localities). A clustering occurred in boreholes and excavated profiles in the area around Wesel and Alpen at the so called Wesel-Flüren and the Alpen/Büderich meanders (Jansen, Reference Jansen2001) (Fig. 5).
For the Wesel-Flüren meander pollen analytical results of six boreholes and one profile from a construction pit were available. From the latter comes a detailed pollen diagram analysed by H.-W. Rehagen 1982 (unpublished, Geological Survey NRW) (Fig. 14).

Fig. 15. Scheme of translation and rotation of a meander-bend (modified after Ghinassi et al., Reference Ghinassi, Nemec, Aldinucci, Nehyba, Özaksoy and Fidolini2014).
This pollen diagram has some striking features in common with that from the Tricensima: there is an overlap in the peak in pollen values of birch (Betula) at around AD 400 (according to the chronology of the Tricensima diagram) and the peak of hazel (Corylus avellana) and alder (Alnus glutinosa) around AD 500. But while the Tricensima diagram continues from here on to younger time periods, the opposite occurs for Wesel-Flüren. It breaks off at around AD 500 but extends further back in time. It shows a peak in pollen values of anthropogenic indicators which could reflect the revival of economic activities during the reign of Emperor Constantine (306–337 AD), the time also the Tricensima was built. That means that the silting-up of this meander probably began in the second half of the 3rd century.
At the lower part of the pollen diagram, the onset of Late Antiquity pollen spectra starts with the continuous representation of beech (Fagus sylvatica) and hornbeam (Carpinus betulus). The relative abundance of so-called anthropogenic indicators and grass pollen suggests that the area was used for animal husbandry. A decline in pollen values of settlement indicators and the increase in pollen of the pioneer tree species birch (Betula) and hazel (Corylus avellana) indicate abandoned areas that were increasingly covered with shrubs. At the same time, parts of the wet meadows were given up leading to the spread of willow (Salix) and alder (Alnus glutinosa) in these places. Nevertheless – as the continuous pollen curve of ribwort plantain (Plantago lanceolata) indicates – grassland continued to exist in the area. The same applies to arable farming, which manifests itself in two cereal-pollen peaks, meaning that farming was still practised somewhere nearby.
Based on the chronology of the Tricensima diagram, the upper pollen spectra of the Wesel diagram are from the 5th century, i.e. before the extensive woodland regeneration.
Even if the pollen analytical data from the other boreholes of the meander are not as detailed as the one presented above, it can be supposed that the beginning of the silting-up of the Wesel-Flüren meander can also be dated into the second half of the 3rd century.
It has been debated whether this palaeomeander originally was the bed of the River Lippe in Roman times, essentially putting the mouth of the Lippe significantly further to the west, roughly opposite the army camps Vetera I and II (von Petrikovits, Reference von Petrikovits1959: 91). The location of the mouth of the River Lippe has always been an important question in Roman military history, since the Roman’s march to the east took place on and along this river. But it could be shown that the Lippe still drains into the Rhine at the same location as it did in Roman times (Klostermann, Reference Klostermann1991; Bremer, Reference Bremer2001). For the adjacent Wesel-Flüren meander, it was assumed that it was either already no longer a flowing palaeomeander in Roman times (Klostermann, Reference Klostermann1991) or a tributary branch of the Rhine with a weak flow (von Detten, Reference von Detten and Kühlborn1995). The pollen diagram clearly supports the latter view. The Wesel-Flüren river branch was primarily fed by Rhine water, evidenced by pollen finds of spruce (Picea abies) and fir (Abies alba) coming from the Rhine catchment’s area by long-distance water-transport (see above). The River Lippe, on the contrary, did not flow through the distribution areas of either of these conifers.
According to the strata lists of the Geological Survey NRW, it is known that medium and coarse sands dominate the fluvial sediments underneath the pollen-bearing silt layers in the Wesel-Flüren meander (see supplements). The relatively small grain size for fluvial Rhine sediments suggests that only a tributary branch could have flown here, because the main Rhine stream used to deposit more gravely sediments. From this evidence, it can be deduced that the side channel used an already existing older loop of the Rhine. In the past, the older meander had created new land at the inside during its migrating process (erosion and aggradation). When the formation of this floodplain terrace ended with the meander cut-off, the last channel remained as an oxbow lake. From this point on, the floodplain was only reached by floods depositing floodplain sediments. The archaeological finds in the alluvial sediments, e.g. bronze axe and arm rings discovered in 1936 before the graveling (see Fig. 5, 7) (BODEON: NI 1936/0008: Hallstatt B, around 1000–800 BC), indicate a period in which an oxbow lake existed and alluvial silt and clay accumulated in it before the Late Bronze Age (Gerlach & Groten, Reference Gerlach and Groten2020).
A question is arising now: how could this meander loop, abandoned since prehistory (i.e. earlier than the deposits from the Late Bronze Age), become reactivated in Roman times before Late Antiquity? This can only have happen by force. As mentioned above, cut-off meanders are quickly separated at their entrance from the new main channel by accumulating sand and gravel plug bars with their exits being sealed in a second step. One possibility of a forced reactivation is that severe flooding and/or drifting ice might have overcome these sediment barriers. Another possibility, and one that could explain a constant water flow as suggested by the sandy river sediments underneath the silt layers of the Wesel-Flüren meander, might have been a man-made barrier breach, maintained over a prolonged period of time for continuous water supply. The Roman military would no doubt have been able to take such measures. This may be concluded from an inscription found in Herwen-De Bijland (NL) near the bifurcation, which mentions a groyne or dam by means of which water was directed into the northern arm of the Rhine in the first decade of the 1st century AD. This might possibly relate to the Drusus dam. Unfortunately, this embankment has not been found so far (e.g. Verhagen et al., Reference Verhagen, Kluiving, Anker, van Leeuwen and Prins2017).
Herget (Reference Herget2000) and Herget et al. (Reference Herget, Bremer, Coch, Dix, Eggensetin and Ewald2005) assume that the Romans transformed the relatively shallow branched river Lippe, being the march route to the east at the end of the 1st century BC, into a single-bed river with a sufficient water level for shipping with planned cut-offs. Building such complex hydrologic structures was only worth the effort at strategically important spots. One of these was located at the mouth of the Lippe near the Wesel-Flüren tributary (see above; Bremer, Reference Bremer2001). The special status of this area is apparent from the presence of the many temporary army camps on the right bank of the Rhine. They would have been easily reached via the reactivated Wesel-Flüren oxbow lake (Fig. 5, 6). If we assume an artificial water supply from the Rhine, river flow guidance constructions (e.g. a groyne) would have to have existed at the upstream beginning of the former meander bend. They then lost their function in the second half of the 3rd century so that the channel finally silted up (again). It is difficult, however, to prove this hypothesis of a Roman water engineering work, since the Wesel floodplain has recently been deeply transformed by gravel quarrying on a large scale. There are only a few areas left, especially in the area of the silted palaeomeander, that can still be investigated in the future.
Alpen/Büderich meander, a Late Antiquity meander migration path
The main channel of the Rhine in Roman times was situated opposite the Flüren meander in the area of Alpen/Büderich. In contrast to the Wesel-Flüren meander, the drilling logs of the Geological Survey NRW show a significantly deeper erosion base as well as gravely deposits (sand and gravel) underneath the silted-up layers (see supplements). The Alpen bend is, however, not the remnant of one single meander (like the Wesel-Flüren meander), but a so-called meander path (“Mäanderweg” after Hoppe, Reference Hoppe1970: 37). The wide bend was formed by the downstream migration of a meander as a result of translation and rotation (Fig. 15) (Knighton, Reference Knighton1998; Ghinassi et al., Reference Ghinassi, Nemec, Aldinucci, Nehyba, Özaksoy and Fidolini2014). The undercut bank erosion of all successive stages together did dissect a semi-arch out of an older, prehistoric Holocene floodplain terrace. The morphological result is a ”seam channel“ (“Nahtrinne”) between two Holocene terraces in the sense of Schirmer (Reference Schirmer1995); Schirmer et al. (Reference Schirmer, Bos, Dambeck, Hinderer, Preston, Schulte, Schwalb, Wessels, Herget and Dikau2005) (see Fig. 4)
The auxiliary fort of Alpen-Drüpt (1st- around 3rd century) was likely built directly on the undercut bank of the meander that existed at the time of its construction (Bödecker et al., Reference Bödecker, Brüggler, Hunke, Otte, Mirschenz, Gerlach and Bemmann2019) (Fig. 5, 5). To what extent the two large marching camps were positioned on subsequent meander stages must remain speculative. The only thing for sure is that the auxiliary fort was partially cut-off by the river at a later stage – due to the absence or demolition of existing bank protection structures. This can hardly be clarified because of a lack of data. A borehole was specifically drilled at this site, but it did not recover any suitable sample for pollen. Further downstream, however, a whole chain of boreholes from the Geological Survey NRW follows the edge of the Alpen meander path. From each core, pollen samples from the bottom of silted-up sediments were analysed (Figs. 5 and 16). The beginning of the silting-up of all respective stages could be dated palynologically to Late Antiquity. This means that by this time the migrating meanders had evidently already moved downstream, away from the military fort and the marching camps. The destruction of the auxiliary fort therefore must have taken place before the hydrographical change. A closer look at the pollen spectra of the boreholes shows a sequence of stages from a vegetation succession. It starts with the pioneer tree species birch and willow, followed by hazel and continues with the increase of semi natural shadow tolerant tree species like lime and beech (Fig. 16).

Fig. 16. Pollen diagrams Alpen: vegetation sequence along the meander (graphics by H. Schluse, Univ. Köln).
The pollen spectrum 4305-32 is characterised by high pollen values of birch (Betula) and willow (Salix). These pioneer trees spread on fallows, resulting from the abandonment of agricultural land. The trees already produce pollen and seeds about 5–10 years after sprouting. The spectrum has many features in common with the birch peak in the Tricensima pollen diagram, suggesting that the silting-up of this meander loop began around AD 400 (according to the Tricensima chronology).
The silting-up of the next downstream meander, pollen spectrum 4305-26, begins in a succession phase in which, in addition to birch, hazel (Corylus avellana) became an important pollen producer. Hazel spreads slower but is able to compete better than birch. Willow has at this stage largely disappeared. Willows are relatively short-lived shrubs, with a life span of around 30 years, and are easily outcompeted, too. Compared with the Tricensima pollen diagram, this pollen spectrum must have been formed only some tens of years later.
The pollen spectra at the start of the silting-up in the next three downstream samples (4305-31, 4305-33 and 4305-55) show that hazel pollen values reached their maximum. Hazel bushes covered the landscape and gave shadow tolerant trees the possibility to spread and subsequently flower. Especially pollen values of lime (Tilia) rise at this stage. Lime starts flowering 20–25 years after sprouting. In the pollen diagram of the Tricensima, this pollen spectrum is represented between about AD 440 and ca. 580.
The silting-up of the two most downstream courses of the Rhine (diagrams 4305-56 and 4305-69) began, according to the pollen spectra, when there was a raised amount of flowering beeches (Fagus sylvatica). Beech starts to flower about 50–80 years after sprouting. It had by now become the dominant tree in the woodlands of the Lower Rhine area. Unfortunately, this phase of woodland succession is not well represented in the Tricensima pollen diagram. Other pollen diagrams in the Rhineland show, however, that beech reached its maximum distribution between around AD 500 and 600 (Kalis & Meurers-Balke, Reference Kalis, Meurers-Balke, Horn, Hellenkemper, Isenberg and Kunow2005).
The successive fine-grained siltation and bank sediments show pollen spectra reflecting a vegetation development that could have taken place in a span of approximately just 200 years. During this time the river bend migrated around 2.8 km downstream. Correlated with the vegetation history, this happened between around AD 400 and 600, which documents the dynamics of the Late Antiquity Rhine.
Discussion
River dynamics and Romans
In this study, we have looked at the temporal indicators for palaeomeander infilling in the proximity of Roman army camps and settlements in the Lower Rhine area. It is the first time that a systematic study was undertaken to check the long held hypothesis that Roman sites were located on oxbow lakes instead of the active river to prevent damage to ships and infrastructure. The dating method used was predominantly the relative-chronological classification of palynological samples from the beginning of siltation in the palaeomeanders. In addition to new samples, extensive archival data could be used for this purpose.
The new and surprisingly consistent result is that at all the locations examined so far the silting took place in the Late Antiquity. In addition to the locations presented here, the army camps of Moers-Asberg, Krefeld-Gellep and Dormagen along the southern Lower Rhine and the meander along Qualburg and Rindern (see Fig. 2) close to Kleve follow the same pattern (Gerlach et al., Reference Gerlach, Röpke, Kels and Meurers-Balke2017; Meurers-Balke et al., Reference Meurers-Balke, Herchenbach, Gerlach, Siepen, Kronsbein and Siepen2015; Gerlach et al., Reference Gerlach, Fischer, Meurers-Balke, Mirschenz, Röpke, Hadler, Willershäuser, Vött, Mirschenz, Gerlach and Bemmann2019). All these sites have in common that their respective river bends became oxbow lakes in Late Antiquity. That means that all sites were previously, during Roman times, located on the undercut bank of the active river (Table 3). It can be assumed that such a location was deliberately chosen.
Table 3. Roman sites on former meanders of the Rhine, which silted up since Late Antiquity.

For the Rhine Delta, Marieke van Dinter (Reference van Dinter2017 and van Dinter et al., Reference van Dinter, Cohen, Hoek, Stouthamer, Jansma and Middelkoop2017) and Harm Jan Pierik (Reference Pierik2017 and Pierik & van Lanen, Reference Pierik and van Lanen2019) were able to show that typical river challenges, such as floods, bank demolitions, or shifting streams, were hazards accepted by the Roman army in trade for direct access to mooring facilities and the waterway. Considering that the troops were primarily supplied by ship, this shows how essential a direct location on the river was for the military.
This need for direct access to the river was certainly not limited to the Rhine Delta but extended upstream, towards the Lower Rhine. The water level required for permanent landing structures, like a quay system, was only guaranteed all year round on the steep undercut-bank, considering the hydrological status of the Roman river with its flat beds divided by islands (Roggenkamp, Reference Roggenkamp2016; Roggenkamp & Herget, Reference Roggenkamp and Herget2016). In contrast, on the shallow banks of point-bar slopes fluctuations in the water level lead to considerable lateral shifts of the river bank, meaning that ships constantly have to moor in different places. Such places were thus avoided and the disadvantages of the opposite undercut-bank side accepted. Because undercut banks were the areas most strongly affected by fluvial erosion protective measures had to be taken against the migrating tendencies of the meander channels to avoid demolition of land and settlements, including potential harbour facilities. The danger of erosion was real.
Several examples of damage caused by the erosion events have been documented archaeologically and geoarchaeologically:
-
i. Bank demolition in front of the CUT,
-
ii. The partial destruction of the Alpen-Drüpt army camp,
-
iii. Demolition damage at the Burginatium army camp.
Obviously, bank protection structures in themselves are evidence of (further impending) bank demolition:
-
i. At the CUT the best example so far of a Roman bank construction (“Packwerk”) at the Lower Rhine could be excavated. The use of caespites shows that such work was carried out by the military. Archaeological evidence points to a construction before AD 90, Dendrochronological data proves a reinforcement with rows of oak posts in the period AD 130-–150 (Selke, Reference Selke, Mirschenz, Gerlach and Bemmann2019).
-
ii. At Moers-Asberg and Krefeld-Gellep two further bank protection constructions could be identified retrospectively based on earlier excavation reports (Gerlach et al., Reference Gerlach, Meurers-Balke and Herchenbach2016a). The bank protection at Moers-Asberg can be dated dendrochronologically to the period AD 60-–90 (Bridger, Reference Bridger1994).
-
iii. At Burginatium the interpretation of wood and stone remains as bank protection in front of the army camp must remain more hypothetical.
Another Roman-age hydraulic engineering project on the Rhine was likely undertaken in the meander of Wesel-Flüren. An artificial river diversion is assumed there due to the fact that a former, prehistoric palaeomeander was reactivated in Roman times. This could be explained as an artificial and planned intervention considering the high strategic importance of that area near the mouth of the River Lippe (Bremer, Reference Bremer2001; Herget et al., Reference Herget, Bremer, Coch, Dix, Eggensetin and Ewald2005). Such a measure would be comparable to the Drusus Dam (known from a Roman inscription), a groyne that is assumed to have been built at Herwen-De Bijland to divert water into a northern arm of the Rhine (Verhagen et al., Reference Verhagen, Kluiving, Anker, van Leeuwen and Prins2017).
The possibilities of excavating further Roman hydraulic engineering constructions are, unfortunately, limited. On the one hand, a large part of the Holocene floodplain has been reworked since then (see post-Roman floodplain terraces on Fig. 2), on the other hand the wooden remains are deep underground in the gravel and may be preserved underwater. It can be assumed that there were far more Roman hydraulic engineering works than we know so far, certainly not only at the undercut banks but also at potential breakthrough positions. Identifying these will be a challenge for gravel pit archaeology in the future.
Conclusion
Due to the Roman infrastructure along the frontier, there must have been a high level of interest in consolidating and optimising the “wet Limes” by means of bank protection structures. We assume that those bank protection structures were often – but not always – so effective that the Rhine actually remained stable in most places during the heyday of the Roman era. Although Jansma (Reference Jansma2020) found on the basis of dendroclimatological data that the period between 185 and 282 AD was the most flood intensive of the first millennium in the Dutch Rhine-Meuse delta, no corresponding enhanced fluvial dynamic as meander cut-offs can be detected for this period at the Lower Rhine.
A shift in the course of the river and thus a silting up of the Roman meanders took place predominantly in Late Antiquity. It is customary to associate intensified river activity with climatic detioration. We assume, on the contrary, that this increase in meander cut-offs in Late Antiquity (from the end of the 3rd century onwards) does not necessarily have to be a climate-induced phenomenon. Rather, we would like to direct the attention to an alternative option that hitherto has been barely considered: In Late Antiquity, the climatically induced river dynamics possibly increased, however, anthropogenic modification of the river course also decreased. Previously, the Romans had been able to keep the course of the Lower Rhine largely stable by bank protection constructions. Based on the results of the pollen analytical studies, we assume that in times of unrest and crises from the end of the 3rd century onwards the bank protection structures were no longer maintained properly. The Rhine was able to regain its more natural morphodynamic behaviour. Meander cut-offs and renewed meander shifts changed the landscape drastically and the former main course of the Rhine and thus the Roman frontier literally dissolved.
Supplementary material
To view supplementary material for this article, please visit https://doi.org/10.1017/njg.2022.11
Acknowledgements
These investigations were part of the “Rhein-Limes-Projekt” of the LVR-Amt für Bodendenkmalpflege im Rheinland, funded by the ministry of “Heimat, Kommunales, Bau und Gleichstellung” of the federal state of Nordrhein-Westfalen (NRW) and part of the Special Research Programme SPP 1630: “Häfen von der Römischen Kaiserzeit bis zum Mittelalter” (Harbours from the Roman Period to the Middle Ages), subproject “Der Rhein als europäische Verkehrsachse” (The Rhine as a European transportation route) funded by the German Research Foundation (DFG).
The Geologischer Dienst NRW (many thanks especially to Christoph Hartkopf-Fröder and Rüdiger Stritzke) provided us with extensive data (pollen diagrams, strata logs, mapping documents). With the data acquisition and their evaluation, we were greatly supported by Julia Gerz, Sonja Groten, Ruthild Kropp, Reiner Lubberich, Alex Thieme and Niklas Wener. We would also like to thank Thomas Becker, Steve Bödecker, Harald Berkel, Clive Bridger, Marion Brüggler, Peter Fischer, Hanna Hadler, Michael Herchenbach, Irmela Herzog, Kerstin Kraus, Jürgen Kunow, Manuela Mirschenz, Sabine Leih, Julia Obladen-Kauder, Thomas Roggenkamp, Julia Rücker, Andreas Vött, Claus Weber and Timo Willershäuser for valuable discussions and suggestions. Above all, we would like to thank all the field archaeologists whose excavation results are at the beginning of all further interpretations.
We also thank Denise Kupferschmidt for her linguistic improvement of the manuscript and two anonymous reviewers and the Editor Harm Jan Pierik for valuable comments.