Postprandial lipaemia, characterised by increased concentrations of TAG-rich chylomicron and remnant particles, exerts a significant and often unappreciated impact on CVD risk( Reference Nordestgaard and Freiberg 1 ). Chylomicrons are synthesised within the small intestinal enterocyte, with a lipid composition highly reflective of the previous meal ingestion( Reference Bysted, Hølmer and Lund 2 , Reference Summers, Barnes and Fielding 3 ). Chylomicrons tend to increase in size with increasing quantities of ingested lipids, but are also larger when composed of proportionally more longer-chain and less saturated TAG( Reference Perez-Martinez, Ordovas and Garcia-Rios 4 , Reference Jackson, Zampelas and Knapper 5 ). Although larger chylomicrons are cleared more slowly than smaller particles, the total number of particles competing for removal has been shown to be the rate-limiting factor in postprandial chylomicron and remnant clearance( Reference Martins, Mortimer and Miller 6 ), with chylomicrons being the preferential lipolytic substrate over remnants( Reference Bjorkegren, Packard and Hamsten 7 , Reference Karpe, Olivecrona and Hamsten 8 ).
Older individuals experience greater postprandial lipaemia( Reference Cohn, McNamara and Cohn 9 – Reference Krasinski, Cohn and Schaefer 15 ). The current evidence suggests that ageing is associated with an increased TAG content of chylomicrons( Reference Cardinault, Tyssandier and Grolier 14 , Reference Krasinski, Cohn and Schaefer 15 ). Further, measurements of postprandial chylomicron dynamics in older adults have been inconsistent and are frequently reliant on proxy measures such as fat-soluble vitamin appearance( Reference Borel, Mekki and Boirie 12 – Reference Krasinski, Cohn and Schaefer 15 ) or apoB-48:TAG ratios( Reference Silva, Kelly and Jones 16 ). Direct measurement of chylomicron particle size with ageing is lacking and this can be quantified by dynamic light scattering methods( Reference Ruf and Gould 17 ). As chylomicron size dictates lipolytic clearance, age-related differences in chylomicron composition and size could contribute to the reported impaired chylomicron remnant clearance and hypertriacylglycerolaemia in elderly adults( Reference Krasinski, Cohn and Schaefer 15 ).
Fat meal loads used in previous studies to induce postprandial lipaemia have varied widely and have often been limited to simple fat boluses that are poorly representative of a typical meal( Reference Mihas, Kolovou and Mikhailidis 18 ). As macronutrients exert differential metabolic responses when ingested alone( Reference Carrel, Egli and Tran 19 ) or in unison( Reference Carrel, Egli and Tran 19 , Reference Kameyama, Maruyama and Matsui 20 ), it is of considerable interest to understand the metabolic response to representative mixed meals. High-fat and high-carbohydrate meals representative of a typical Western diet have increasingly been used to study postprandial lipaemia in healthy( Reference Deopurkar, Ghanim and Friedman 21 – Reference Ghanim, Abuaysheh and Sia 23 ) and metabolically compromised populations( Reference Thomsen, Storm and Holst 24 , Reference Devaraj, Wang-Polagruto and Polagruto 25 ). Thus, in this study, direct measurement of the effect of age on chylomicron dynamics and composition following a high-fat (and high-carbohydrate) meal, representative of a Western diet, was conducted. Analysis was performed to examine chylomicron particle size, apoB concentration and fatty acid composition of both the chylomicron TAG and the phospholipid (PL) fractions in a healthy younger and older adult population. We hypothesised that an exaggerated postprandial lipaemic response in older adults would be due to the formation of larger chylomicrons containing greater TAG that would delay clearance. We further aimed to describe the fatty acid composition of both the chylomicron TAG and the PL fraction in older adults.
Methods
Subject selection
In total, thirty healthy, community-dwelling subjects (n 7 young females, n 8 young males, n 9 older females, n 6 older males) from the Auckland region were recruited through newspaper advertisements and from the university community to participate in the study. Eligible subjects were required to have a BMI between 18 and 30 kg/m2 and be between the ages of 20–25 and 60–75 years. Individuals with a history of CVD or metabolic disease/conditions including diabetes or thyroid conditions or who used medications that may interfere with study end points (i.e. anti-inflammatory drugs, statin drugs) were not eligible for participation. All subjects reported that they were non-smokers. This study was conducted according to the guidelines laid down in the Declaration of Helsinki, and all procedures involving human subjects were approved by the University of Auckland Human Participants and Ethics Committee (ref no. 8026). Written informed consent was obtained from all subjects. This study was registered prospectively at Australian New Zealand Clinical Trials Registry at anzctr.org.au (ID: ACTRN12612000515897).
Study procedures
The present study was a cross-sectional, postprandial trial using a high-fat breakfast, chosen as a standard test meal with high fat (62·2 g) and protein content, also representative of a high-fat restaurant breakfast meal( Reference Ghanim, Abuaysheh and Sia 23 ) (Table 1). Subjects were asked to abstain from vigorous physical activity, high-fat foods, anti-inflammatory medications and nutritional supplements the day before their visit. Subjects arrived fasted on the morning of their visit; anthropometric data were collected before a catheter was inserted into an antecubital vein and a baseline sample (time 0 h) was obtained followed by consumption of the test breakfast. Subject physical activity was limited to sedentary activities within the clinical room of the study facility. Blood samples were collected after the meal into serum- and EDTA-containing blood collection tubes (Becton Dickinson) every hour for 5 h. Serum tubes were left to clot at room temperature for 15 min before all the tubes were centrifuged at 1500 g for 15 min at 4°C for serum and plasma separation. An aliquot of plasma was maintained at 4°C for chylomicron-rich fraction (CMRF) separation within 6 h, and the remaining plasma was collected in pyrogen-free microtubes and stored at −20°C until analysis.
Table 1 Macronutrient and fatty acid composition of the breakfast meal (Mean values with their standard errors)

* Meal consisting of two sausage egg muffin sandwiches containing English muffin (wheat), egg, sausage patty (beef), cheese slice and rapeseed oil, with two hash browns containing potatoes and rapeseed oil. Values presented are based on nutrient panel data obtained from the website of the fast food restaurant.
† n 3.
‡ Fatty acids with a mass proportion <0·5 g/100 g are pooled to others category.
Chylomicron-rich fraction isolation
The CMRF, containing chylomicrons and their large remnants, was separated in 4·7-ml OptiSeal tubes (Beckman Coulter) in an Optima MAX-XP ultracentrifuge using a TLA-110 rotor (Beckman Coulter), adapted from Oikawa et al.( Reference Oikawa, Mizunuma and Iwasaki 26 ). Density-gradient saline solutions were prepared with NaCl and 0·005 % EDTA (Sigma-Aldrich) according to Naito( Reference Naito 27 ), and separation protocols were based on the studies of Kupke & Wörz-Zeugner( Reference Kupke and Wörz-Zeugner 28 ). In brief, 3·5 ml plasma was overlaid with 1·2 ml saline solution (d=1·006 g/ml) and centrifuged at 117 000 g for 10 min. The visible top layer was aspirated into microtubes and corrected to a final collection volume of 1·4 ml using saline solution. This provided a standardised dilution factor of the collected CMRF volume relative to initial plasma volume. The CMRF was stored in pyrogen-free microtubes at −80°C until use.
Chylomicron-rich fraction particle size analysis
CMRF particle size was measured using a NanoZetasizer S (Malvern Instruments) by dynamic light scattering. If more than one size peak was observed, the mean peak data for the peak within the range of large TAG-rich lipoproteins (TRL) were used (>70 nm) based on the lower range of mean diameters of small chylomicrons (Sf 400–1000), as reported by Fraser( Reference Fraser 29 ).
Biochemical analysis
Concentrations of plasma glucose, cholesterol, LDL, HDL, TAG, NEFA and CMRF TAG, apoB, serum alanine transaminase (ALT) and serum aspartate transaminase (AST) were measured using a Hitachi 902 autoanalyser (Hitachi High Technologies Corporation) by enzymatic colorimetric assay (Roche). Plasma insulin concentration was measured using an Abbott AxSYM system (Abbott Laboratories) by microparticle enzyme immunoassay.
Fatty acid composition analysis of meals and chylomicron-rich fraction TAG and phospholipids
Meals were homogenised using a domestic blender with 600 g water. Samples were aliquoted into 50-ml centrifuge tubes, frozen and stored at −80°C until use. Fatty acid extraction, methylation and GC of meal and CMRF samples were carried out according to protocols described previously( Reference Folch, Lees and Sloane Stanley 30 – Reference Christie 32 ). In short, an internal standard mixture of triheptadecanoin (Sigma-Aldrich) and dinonadecanoylphosphatidylcholine (Sigma-Aldrich) was added to the isolated CMRF. Subsequently, 1·5 ml methanol, 3 ml chloroform and 0·8 ml 0·88 % KCl in water were added and the blend was thoroughly vortexed after each addition. Samples were centrifuged at 2000 g for 3 min to separate the layers, and the lower chloroform-rich layer was removed and the upper layer was extracted again( Reference Folch, Lees and Sloane Stanley 30 ). Food lipids were extracted just as CMRF after further homogenisation with an Ultra Turrax (IKA). CMRF TAG and PL were isolated from the extracted lipid mixture with solid-phase extraction based on Sep-Pak® Vac 1 cc silica columns (Waters)( Reference Hamilton and Comai 31 ).
Fatty acid methyl esters (FAME) were prepared using the sodium methoxide method. In short, the lipids were suspended into 1 ml dry diethyl ether; subsequently, 25 μl methyl acetate and 25 μl sodium methoxide were added and the blend was incubated for 5 min while shaken at times. The reaction was stopped with 6 μl acetic acid. Samples were centrifuged at 2000 g for 5 min, after which the supernatant was removed and gently evaporated to dryness. The resulting FAME were transferred to 100 μl inserts in hexane( Reference Christie 32 ). The FAME were analysed using a gas chromatograph (Shimadzu GC-2010) equipped with an AOC-20i auto injector, a flame ionisation detector (Shimadzu Corporation) and a wall-coated open tubular column DB-23 (60 m×0·25 mm i.d., liquid film 0·25 μm; Agilent Technologies, J.W. Scientific). Splitless/split injection was used and the split was opened after 1 min. Supelco 37 Component FAME Mix (Supelco), 68D (Nu-Chek-Prep) and GLC-490 (Nu-Chek-Prep) were used as external standards.
Calculations
The homoeostatic model assessment of insulin resistance (HOMA-IR) was calculated from fasting glucose and insulin concentrations using the equation from the study by Matthews et al.( Reference Matthews, Hosker and Rudenski 33 ). Maximum concentrations (C max) were identified as the greatest value in an individual’s data set, with maximum peak times (T max) identified as the corresponding nominal sampling time. Arithmetic means were used for group C max comparisons, whereas median values were used for group T max comparisons. Plasma fatty acids were pooled for mathematical analysis into SFA, MUFA, PUFA and dairy-derived odd-chain fatty acids.
Statistical analyses
Statistical analyses were carried out using Statistical Package for the Social Sciences version 21 (SPSS; IBM Corporation). Sample size was estimated with a power analysis for a superiority design based on data from a previous study on lipaemia in the elderly( Reference Puga, Meyer and Everman 11 ). To achieve a significance level of P≤0·05 with 80 % power to detect a between-subject difference of 4·77 mmol/l per h for TAG incremental AUC (iAUC), a sample size of 30 was necessary. Data are presented as means values with their standard errors except T max, which is presented as the medians and interquartile ranges. iAUC was calculated after subtracting fasting values. Baseline subject characteristics, iAUC and C max were compared using unpaired Student’s t test. T max was compared using the Mann–Whitney U test using mean ranks. Two-factor repeated-measures ANOVA (time compared within-subject and age compared between subjects) followed by the Sidak-adjusted post hoc test were used for all multiple comparisons between different groups. Where Mauchly’s sphericity test failed, the Huynh–Feldt correction was applied. α was set at P<0·05. Heat maps were created using R software version 2.15.2 using the packages gplots (heatmap.2), RColorBrewer and colorRamps (R Development Core Team).
Results
Subject characteristics
A total of thirty subjects completed the study (n 15 younger, n 15 older). There were no significant differences between the two age groups for BMI or fasting measurements of plasma glucose, plasma insulin, HOMA-IR, plasma or CMRF TAG or CMRF apoB content or particle size (Table 2). Fasting plasma lipid profiles were within the normal range for both age groups, although total, LDL and HDL-cholesterol levels were all higher in older subjects (P<0·001, P=0·002 and P<0·001, respectively). Liver function measured by serum ALT and AST were within the normal range for all participants; however, the older participants displayed lower concentrations for each (P=0·004 and P=0·001, respectively).
Table 2 Baseline subject characteristics and fasting plasma, serum and chylomicron-rich fraction lipid profile of older and younger subjects (Mean values with their standard errors)
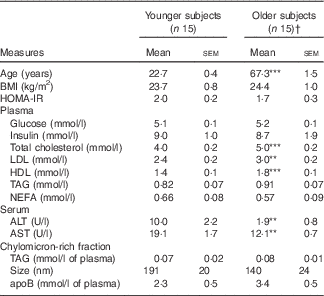
HOMA-IR, homoeostatic model assessment of insulin resistance; ALT, alanine transaminase; AST, aspartate transaminase.
† Significance was determined by unpaired Student’s t test: *** P<0·001, ** P<0·01 compared with younger subjects.
Plasma glucose, insulin and lipid responses
Postprandial glucose (Fig. 1(a)) and insulin (Fig. 1(b)) responses to the meal did not differ between younger and older subjects. The older group had a delayed median time to achieve peak plasma TAG concentrations relative to the younger group (T max 3 (IQR 3–4) v. 2 (IQR 1·5–3) h, respectively; P<0·001), reaching peak TAG concentration (C max) after 3 h (P<0·001 v. baseline). The younger subjects reached maximum peak concentration at 2 h (P=0·02 v. baseline). The plasma TAG in the younger subjects also returned to baseline by 4 h; however, in the older group, plasma TAG remained significantly elevated at 5 h post-meal (Fig. 1(c)). As such, we observed a prolonged TAG response in older subjects. This pattern of response did not impact on the iAUC (0–5 h) response between the younger and the older groups (103·6 (sem 21·6) v. 102·5 (sem 19·1) mmol/l, respectively; P=0·97; data not shown). Marked suppression of circulating plasma NEFA was observed following the meal, with no difference between younger and older subjects (Fig. 1(d)). Postprandial total plasma cholesterol concentrations decreased in the older but not in the younger participants (Table 3; time–age interaction; P<0·001).

Fig. 1 Postprandial plasma concentrations of glucose (a), insulin (b), TAG (c) and NEFA (d) in older () and younger (
) subjects. Values are means (n 15/group), with their standard errors. There were no differences in glucose, insulin or NEFA responses between older and younger subjects. There were significant differences over time in the TAG response between older and younger subjects (age×time interaction of P<0·01, two-factor repeated-measures ANOVA). *** P<0·001 change from baseline in all subjects; † P<0·01, change from baseline in older subjects; ‡ P<0·01 change from baseline in younger subjects; § P<0·01 change from 5 h in younger subjects (Sidak-corrected post hocs).
Table 3 Postprandial total cholesterol in older and younger subjects (Mean values with their standard errors)

† Significance was determined by two-factor repeated-measure ANOVA with Sidak-corrected post hoc analysis: ** P<0·01, *** P<0·001 compared with baseline within age group; § P<0·01 compared with 1 h. All time point comparisons between younger and older subjects were significant to P<0·01.
Chylomicron-rich fraction dynamics
CMRF particle size increased after the meal (P=0·01; main effect of time, Fig. 2(a)) and was smaller for the older compared with the younger participants (P=0·011, main effect of age). Owing to variation in time to peak size between individuals, median group maximum peak CMRF particle size and T max were compared. Overall median peak time was 3 h post-meal (T max 3 (IQR 2–3·5) v. 2 (IQR 1–4) h, younger v. older, respectively; P=0·467). Maximum CMRF particle size tended to be smaller in the older subjects (C max 321 (sem 29) and 390 (sem 22) nm, older v. younger, respectively; P=0·072). As CMRF particle size was not different at baseline between age groups, post hoc analysis was conducted to determine age-group postprandial changes in size, despite no time–age interaction. The older group’s CMRF particle size remained constant after the meal, whereas the younger group’s CMRF particle size increased, resulting in an age difference in size at 2–3 h (P=0·022 and P=0·007, respectively). Postprandial CMRF TAG concentration was not different between older and younger subjects (P=0·894; Fig. 2(b)).

Fig. 2 Postprandial chylomicron-rich fraction (CMRF) particle size (a), TAG concentration (b) and apoB concentration (c) in older () and younger (
) subjects. Values are means (n 15/group), with their standard errors in nm for particle size, mmol/l of plasma for TAG and g/l of plasma for apoB. There were no differences in CMRF TAG response concentrations between older and younger subjects. There were significant main age differences in the particle size and apoB responses between older and younger subjects (age effect marked (†) of P<0·05 each, two-factor repeated-measures ANOVA). * P<0·05 change from baseline in all subjects; ** P<0·01 change from baseline in all subjects (Sidak-corrected post hocs).
Changes in CMRF particle numbers were assessed by apoB concentrations. Older subjects had higher apoB concentrations than younger subjects (mean postprandial concentration 0–5 h of 5·3 (sem 0·4) v. 4·2 (sem 0·4)×10−2 g/l, respectively; P=0·035; Fig. 2(c)). Overall, apoB concentrations peaked at 2 h post-meal (P=0·001) returning to comparable baseline concentrations by 5 h.
Chylomicron-rich fraction TAG and phospholipid fatty acid composition
Comparison of chylomicron-rich fraction TAG composition between ages
Older subjects had greater proportions of MUFA in their CMRF TAG (5·6 % greater proportion in older v. younger subjects; P=0·014; Fig. 3). In addition, 4 h after the meal, older subjects had a lower proportion of PUFA in their CMRF TAG (9·4 % lower proportion in older v. younger subjects; P=0·004). Older participants’ CMRF TAG tended to have lower proportions of SFA at 2 h (7·0 % lower proportion in older v. younger subjects; P=0·079), a trend that was lost by 4 h.

Fig. 3 Heat map of distribution changes of chylomicron-rich fraction SFA, MUFA and PUFA after the meal of older and younger subjects. Values are presented as mean per cent changes relative to younger-subject 2-h mass proportion for each saturation class. , 0 % change from younger 2 h;
, negative percentage changes;
, positive percentage changes.
These differences in MUFA and PUFA CMRF TAG composition were attributable to differences in the major fatty acids – oleic acid and linoleic acid. The older group’s CMRF TAG had relatively more oleic acid (18 : 1n-9; P=0·033; Fig. 4(a)). Although 18 : 2n-6 (linoleic acid) was found in equal proportions in both groups’ CMRF TAG at 2 h, by 4 h the older subjects had lower proportions (P=0·003). The older group had lower proportions of 18 : 0 (stearic acid) at 2 h compared with younger participants (P=0·009), whereas these age differences were not apparent after 4 h. Older subjects had lower proportions of 15 : 1 at 2 h compared with the younger group (P=0·037).

Fig. 4 Major chylomicron-rich fraction (CMRF) TAG (a) and phospholipid (b) fatty acid composition of younger subjects at 2 h () and 4 h (
) and older subjects at 2 h (
) and 4 h (
) as mass proportions (g/100 g). Data marked (†) differ with age, and data marked (‡) differ with time; P<0·05 for age and time by two-factor repeated-measures ANOVA. Age×time interaction post hocs (Sidak-corrected within each acid) are indicated as * P<0·05, ** P<0·01 between younger and older subjects at specified time points.
Pooling of the dairy-derived odd-chain fatty acids (15 : 0, 15 : 1, 17 : 0, 17 : 1) showed higher proportions in the CMRF TAG of older subjects (P<0·001). In both groups, CMRF TAG composition changed over the postprandial period. From 2 to 4 h after the meal, proportions of CMRF TAG 20 : 4n-6 (arachidonic acid) and 22 : 6n-3 (DHA) increased (P=0·002 and P=0·042, respectively).
Comparison of chylomicron-rich fraction phospholipid composition between ages
In both older and younger subjects, CMRF PL composition changed over the postprandial period. At 4 h, CMRF PL contained relatively more PUFA (P=0·034), less SFA (P=0·042) and tended to contain more MUFA (P=0·052) than at 2 h (Fig. 3). This difference in the later postprandial period was characterised by relatively more linoleic acid (18 : 2n-6; P=0·023) than at 2 h (Fig. 4(b)).
The CMRF PL of the older subjects contained relatively more MUFA (8·7 % greater proportion in older v. younger subjects; P=0·038), less PUFA (3·3 % lower proportion in older v. younger subjects; P=0·032) and tended to contain less SFA (3·2 % lower proportion in older v. younger subjects; P=0·085; Fig. 3) relative to younger subjects. Arachidonic acid was found in lower concentrations in older subjects (P=0·018).
Similar to the CMRF TAG, the CMRF PL in the older group contained greater proportions of oleic acid (18 : 1n-9; P=0·028). Elaidic acid (18 : 1n-9trans at 2 h; P=0·001) and 18 : 2n-6trans (P=0·012) were also more abundant in the CMRF PL of older participants; this was accompanied by relatively higher levels of the dairy-derived odd-chain fatty acids (P=0·001), particularly 15:0 and 15:1 (P=0·018 and P=0·003, respectively). Furthermore, the older subjects had greater proportions of the PUFA docosapentaenoic acid (22 : 5n-3; P=0·013) and tended to have relatively more EPA (P=0·058).
Discussion
Following a high-fat meal, the older participants of the present study had smaller chylomicron particles with a greater proportion of oleic acid and a lower PUFA content over a 5-h postprandial period. This study highlights that there are subtle changes in the dynamics governing lipid absorption, which occur along with differences in relative absorption and/or clearance of specific fatty acids, predominately oleic acid. Furthermore, our data do not support either altered appearance or delayed chylomicron clearance as a major regulator of the exaggerated lipaemia, typically present in older individuals. Rather, this study shows that the major contributant to the heightened postprandial TAG response is in the non-chylomicron fraction and includes other TAG-rich particles including VLDL and chylomicron remnants( Reference Puga, Meyer and Everman 11 ).
Consistent with previous reports, our data demonstrate prolonged postprandial triacylglycerolaemia in older adults( Reference Cohn, McNamara and Cohn 9 – Reference Krasinski, Cohn and Schaefer 15 ). However, within this literature, a few studies have addressed the dynamics and role of chylomicron structure and composition in this hypertriacylglycerolaemic response. In the present study, the concentration of CMRF TAG was not different between older and younger participants. Previous studies reporting differences in chylomicron TAG( Reference Borel, Mekki and Boirie 13 – Reference Krasinski, Cohn and Schaefer 15 ) have shown greater TAG content in large, rather than small, TRL in the elderly( Reference Borel, Mekki and Boirie 12 ), plus a greater postprandial NEFA spillover from exogenous TRL( Reference Puga, Meyer and Everman 11 ). Neither mechanism was demonstrated in the present study. Rather, our data implicate alterations of small TRL (endogenous VLDL and small chylomicron remnants) production and clearance as a major determinant of the sustained postprandial hypertriacylglycerolaemia in the elderly. It must be noted that there were no age differences in BMI, HOMA-IR, fasting TAG and NEFA or indications of hepatic dysfunction in this present study. Previously, these factors have been shown to adversely affect postprandial chylomicron dynamics( Reference Puga, Meyer and Everman 11 ). Furthermore, our findings are presented in the context of a mixed breakfast meal, rather than in response to the ingestion of isolated lipid loads.
In this study, the older participants had a maximum chylomicron particle size, on average 18 % smaller and 27 % more numerous compared with the younger group. Importantly, chylomicron sizes were measured directly through dynamic light scattering, a technique used previously for chylomicron and lipoprotein size analyses( Reference Ruf and Gould 17 , Reference Sakurai, Trirongjitmoah and Nishibata 34 ), although rarely in the context of postprandial dynamics( Reference Vors, Pineau and Gabert 35 ). These smaller and more numerous TRL are a reported feature of insulin resistance( Reference Mekki, Christofilis and Charbonnier 36 ) and certain types of dyslipidaemia( Reference Sakai, Uchida and Ohashi 37 ). Smaller and more abundant TRL may be due to intestinal over-production of apoB-48( Reference Iqbal and Hussain 38 , Reference Duez, Pavlic and Lewis 39 ) and/or failed suppression of postprandial large VLDL production( Reference Chen, Swami and Skowronski 40 ). However, in this study, no age difference in fasting insulin sensitivity, as measured by HOMA-IR, were observed. Thus, the mechanisms contributing to the increased concentrations of smaller and more numerous chylomicron particles were not determined in the present study.
Differences in chylomicron particle size and number may have important implications for chylomicron clearance and potentially for atherosclerotic risk in these older subjects. Smaller chylomicron particle size has been shown to retard clearance rate( Reference Quarfordt and Goodman 41 ); however, more recent investigations have shown that this may be an artifact of unmatched number of particles( Reference Martins, Mortimer and Miller 6 ), indicating that larger numbers, often corresponding to smaller size, are cleared more slowly. Smaller and greater circulating numbers of chylomicron remnants, due to their ability to penetrate the arterial intima, have been shown to increase atherosclerotic risk( Reference Nordestgaard and Freiberg 1 ). However, our results suggest that the large CMRF, although containing relatively smaller particles, is cleared efficiently, whereas the small TRL fraction may persist.
In addition to the alterations in the size and number of circulating chylomicrons, differences in the lipid composition of both the TAG and the PL fractions were measured. The older individuals had greater proportions of MUFA, particularly oleic acid, in chylomicron TAG, with a corresponding lowering of the proportion of PUFA. These compositional differences were also present in the PL fraction. Our findings correspond with reports of age-related differences in adipose and circulating TAG composition with age-related declines in SFA( Reference Schafer, Overvad and Thorling 42 ), linoleic acid (18 : 2n-6)( Reference Bolton-Smith, Woodward and Tavendale 43 ) and total PUFA contents( Reference Holman, Smythe and Johnson 44 ), along with increases in MUFA content( Reference Schafer, Overvad and Thorling 42 ). Similarly, our findings of lower chylomicron PL PUFA are similar to what has been previously demonstrated in elderly populations( Reference Holman, Smythe and Johnson 44 – Reference Crowe, Skeaff and Green 46 ). Habitual diet or health status has been suggested to impact these age-related compositional differences( Reference Bolton-Smith, Woodward and Tavendale 43 , Reference Asciutti-Moura, Guilland and Fuchs 45 ). Indeed, the post-meal plasma fatty acid composition has previously been shown to correspond poorly with meal fatty acid composition, particularly regarding plasma MUFA content in older subjects, suggesting an important impact of habitual diet( Reference Burdge, Powell and Calder 47 ). In contrast, meal composition has been reported to significantly impact postprandial TAG in young subjects( Reference Koutsari, Zagana and Tzoras 48 ), supporting a difference in post-meal fatty acid metabolism between old and young individuals. Furthermore, as our postprandial findings match longer-term evaluations of circulating fatty acids in elderly populations, the metabolic handling of fatty acids may contribute to age-related fatty acid differences in the elderly.
There are likely to be several mechanisms exerting influence on the differences in chylomicron fatty acid composition between the younger and the older subjects following the consumption of an identical meal, including possible differences in fatty acid absorption and clearance from TRL. Enterocyte fatty acid metabolism may be involved as chylomicron incorporation of fat-soluble vitamins has suggested age-related differences in postprandial absorption( Reference Borel, Mekki and Boirie 12 , Reference Cardinault, Tyssandier and Grolier 14 ). The greater proportions of MUFA observed in the older group may indicate enhanced intestinal absorption of oleic acid. This is supported by animal models indicating that uptake of oleic acid( Reference Hollander and Dadufalza 49 ), linoleic acid( Reference Hollander, Dadufalza and Sletten 50 ) and SFA( Reference Keelan, Walker and Thomson 51 ) are increased in aged rats, fitting with our data. In addition, chylomicron TAG are known to contain proportions of fatty acids originating from enterocyte storage pools, containing fatty acids from the previous meal( Reference Ravikumar, Carey and Snaar 52 , Reference Chavezjauregui, Mattes and Parks 53 ) as well as early postprandial contributions from NEFA( Reference Ravikumar, Carey and Snaar 52 ). It has been suggested that in insulin-resistant states the intestines may store greater quantities of fat, contributing to aberrant apoB production( Reference Matikainen and Taskinen 54 ). It is unknown whether differences in intestinal enterocyte storage pools between age groups may have contributed to postprandial fatty acid compositional differences. However, it is probable that the predominant mediator is exchange with either circulating free or lipoprotein-derived PL and TAG or the presence of endogenous large TRL. Indeed, the postprandial reductions in total cholesterol we observed in the older group suggest increased inter-lipoprotein transfer; however, measures of this activity such as cholesterol ester transfer activity were not assessed. Furthermore, the origins of altered proportions of fatty acids in any endogenous lipoproteins, such as greater MUFA content, remain unknown.
The postprandial age-related differences in older participants’ chylomicron composition may have metabolic implications outside of transient effects on postprandial lipaemia. As older people incorporate ingested TAG at a greater rate into small TRL rather than chylomicrons( Reference Puga, Meyer and Everman 11 ), any differences in fatty acid uptake will be amplified with the increased residence time of these remnants( Reference Cohn, McNamara and Cohn 9 , Reference Krasinski, Cohn and Schaefer 15 ). In addition, the increased surface area:volume ratio of chylomicrons in the older group implies proportionally more PL, magnifying the impact of these compositional differences. Specifically, enhanced uptake of oleic acid could have implications for uptake of other fatty acids, as long-chain fatty acid uptake into the enterocyte is competitive( Reference Davidson, Magun and Glickman 55 ), and could explain the lower proportions of PUFA found in older subjects’ chylomicron TAG. Furthermore, the fatty acid composition differences reported in these older adults have been identified as features in individuals with metabolic dysfunction; lower serum linoleic acid has been associated with an increased risk of developing impaired fasting glucose or type 2 diabetes mellitus in middle-aged men( Reference Laaksonen, Lakka and Lakka 56 ). In addition, as we show that some age-related compositional differences are dependent on postprandial sampling time, differences in rates of uptake or clearance may contribute to these age-related differences, highlighting a downfall of compositional profile analysis from a single time point when temporal metabolic differences exist between populations.
Conclusions
The exaggerated postprandial lipaemia typical of older individuals is not characterised by elevated chylomicron TAG. This study demonstrated that in an older healthy cohort there is no impairment of large chylomicron clearance. Despite this preservation of a chylomicron response to a high-fat meal, the resultant chylomicrons are smaller and more numerous in the elderly, containing greater proportions of MUFA and lower proportions of PUFA. The implications of these fundamental differences in chylomicron dynamics and composition are unknown in the context of CVD risk and warrant further investigation.
Acknowledgements
The authors thank the study participants, the support of the Maurice & Agnes Paykel Clinical Research Unit, particularly Janene Biggs, Ben Albert, Christine Brennan, Vic Shao-Chih Chiang and Wonjoo Lee and the lipid analysis assistance of Karlo Villa.
This study was funded by the Liggins Institute Trust through project no. 3701462. The Liggins Institute Trust had no role in the design, analysis or writing of this article.
A. M. M. carried out laboratory analysis and data interpretation and drafted the manuscript. A. N. carried out lipidomic analysis. A. M. M., S. P. and C. A. P. were involved in the coordination, management and implementation of the clinical trial. J. F. M. provided laboratory supervision. J. F. M. and K. M. L. critically evaluated the manuscript. K. M. L. supervised lipidomic analysis. D. C.-S. formulated the research question, initiated and supervised all aspects of the study. All the authors approved the final version of the manuscript for submission.
The authors declare that there are no conflicts of interest.