Introduction
The ore deposits of Eastern Liguria, Northern Apennines, Italy, are well-known among mineralogists for the occurrence of several rare species from the Mn ore deposits hosted in middle Jurassic metacherts of the Graveglia Valley, Genoa Province (Cabella et al., Reference Cabella, Lucchetti and Marescotti1998; Bindi et al., Reference Bindi, Carbone, Belmonte, Cabella and Bracco2013; Biagioni et al., Reference Biagioni, Belmonte, Carbone, Cabella, Zaccarini and Balestra2019a) and the Cerchiara Mine, La Spezia Province (Kampf et al., Reference Kampf, Roberts, Venance, Carbone, Belmonte, Dunning and Walstrom2013; Kolitsch et al., Reference Kolitsch, Merlino, Belmonte, Carbone, Cabella, Lucchetti and Ciriotti2018). In addition, Cu–Fe–Ni–Co sulfide ore deposits are also hosted in ophiolitic rocks of the Northern Apennines and the Sestri–Voltaggio Zone, where several types of Volcanic Massive Sulfide (VMS) deposits occur in mafic–ultramafic rocks at different stratigraphic positions within the ophiolitic sequences (Ferrario and Garuti, Reference Ferrario and Garuti1980; Zaccarini and Garuti, Reference Zaccarini and Garuti2008; Schwarzenbach et al., Reference Schwarzenbach, Früh-Green, Bernasconi, Alt, Shanks III, Gaggero and Crispini2012). Among them, stockwork-vein and seafloor stratiform orebodies are associated with serpentinised mantle peridotites and serpentinite breccias (e.g. Ferrario and Garuti, Reference Ferrario and Garuti1980), like in the Monte Ramazzo–Lagoscuro area, where a pyrrhotite-dominated mineralisation had been actively exploited since the beginning of the 19th Century (Rolandi, Reference Rolandi1974; Pipino, Reference Pipino1977). The primary mineralisation underwent subsequent fluid–rock interaction, hydrothermal mobilisation, and multi-stage alteration processes which led, on the one hand, to sulfide reconcentration and recrystallisation along tectonic structures, on the other hand to metal reworking and the formation of secondary phases, such as (Ni,Co)-bearing oxy-hydroxides (asbolane and heterogenite), carbonates (kolwezite, Co-rich malachite and spherocobaltite) and silicates (pecoraite). This peculiar mineral assemblage occurring in the Monte Ramazzo–Lagoscuro ore deposit is quite different from other hydrothermal sulfide mineralisation described so far in ophiolites from the Eastern Liguria, where (Ni,Co)-enrichment occurs mostly in pyrite and/or accessory minerals like millerite, siegenite and pentlandite (e.g. Cortesogno et al., Reference Cortesogno, Lucchetti and Penco1977; Schwarzenbach et al., Reference Schwarzenbach, Früh-Green, Bernasconi, Alt, Shanks III, Gaggero and Crispini2012; Moroni et al., Reference Moroni, Rossetti, Naitza, Magnani, Ruggieri, Aquino, Tartarotti, Franklin, Ferrari, Castelli, Oggiano and Secchi2019).
During the examination of secondary minerals from the Monte Ramazzo mining complex, blue acicular crystals from the Lagoscuro mine were encountered. Crystallographic studies and chemical analyses showed this phase to be a new mineral species, which we describe herein and name isselite. The mineral and its name have been approved by the International Mineralogical Association (IMA) Commission on New Minerals, Nomenclature and Classification (CNMNC) (IMA2018-139, Biagioni et al., Reference Biagioni, Belmonte, Carbone, Cabella, Demitri, Perchiazzi, Kampf and Bosi2019b). Holotype material for isselite is deposited in the mineralogical collection of the Museo di Storia Naturale, Università di Pisa, Via Roma 79, Calci, Pisa, Italy, under catalogue number 19904, and of the Dipartimento di Scienze della Terra, dell'Ambiente e della Vita (DISTAV), Università di Genova, Corso Europa 26, Genova, Italy, under catalogue number MO484. Cotype material is preserved in the collections of the Natural History Museum of Los Angeles County, 900 Exposition Boulevard, Los Angeles, CA 90007, USA, catalogue number 67195. The new mineral is dedicated to Arturo Issel (1842–1922) for his pioneering work on the geology, palaeontology and mineralogy of Italy, with a special reference to Liguria (Issel, Reference Issel1892). He was professor of Geology and Mineralogy at the University of Genoa beginning in 1866 and Director of the Institute of Geology and the Geological Museum until 1917. Arturo Issel was the author of important scientific monographs on a variety of topics (not only geology and palaeontology, but also anthropology and ethnology) and contributed to several geological maps of Liguria.
Occurrence and physical properties
Isselite occurs as a secondary phase in the Fe–Ni–Co sulfide mineralisation formerly exploited at the Lagoscuro mine (44°28′35''N, 8°51′35″E), Ceranesi, Genoa Province, Liguria, Italy. This mine, which belongs to the Monte Ramazzo mining complex, operated from 1815 to 1825, mainly for magnesium sulfate (Pipino, Reference Pipino1977). The Co-rich pyrrhotite + pentlandite ± sphalerite sulfide ore, hosted within serpentinite and serpentinite breccia in contact with basaltic rocks belonging to the Figogna Unit in the Ligurian Alps (Galli, Reference Galli1954 and Reference Galli1963; Capponi and Crispini, Reference Capponi and Crispini2008), underwent multi-stage alteration processes and hydrothermal mobilisation, which developed a mineral assemblage formed by violarite + valleriite + andradite ± magnetite, along with other Ni–Co secondary minerals (e.g. pecoraite, heterogenite and asbolane) and hydrated Mg–Cu(–Ni) carbonates and sulfates such as malachite, brochantite, langite, posnjakite and hydromagnesite. The transformation of pentlandite to violarite in the sulfide ore seems to constrain the first stage of hydrothermal alteration to T < 210°C under strongly acidic to neutral (i.e. 1 < pH < 6) and reducing (i.e. log$f_{O_2}$(g) < –35) conditions (Tenailleau et al., Reference Tenailleau, Pring, Etschmann, Brugger, Grguric and Putnis2006; Xia et al., Reference Xia, Brugger, Chen, Ngothai, O'Neill, Putnis and Pring2009).
Isselite was identified in only a few specimens as sprays of blue acicular crystals, striated parallel to [100] and tabular on {001}, up to 0.1 mm long (Fig. 1). Observed forms are {001}, {010}, {103} and {10$\bar{3}$}. The streak is light blue. Isselite is vitreous and transparent. The mineral is brittle, with an irregular fracture and good cleavage on {001} and {100}. Hardness was not measured, owing to the small crystal size. The density measured by sink–float in methylene iodine – toluene is 3.00(2) g/cm3, compared with a density of 2.946 g/cm3, calculated on the basis of the empirical formula and the unit-cell volume refined from single-crystal X-ray diffraction data at room temperature.
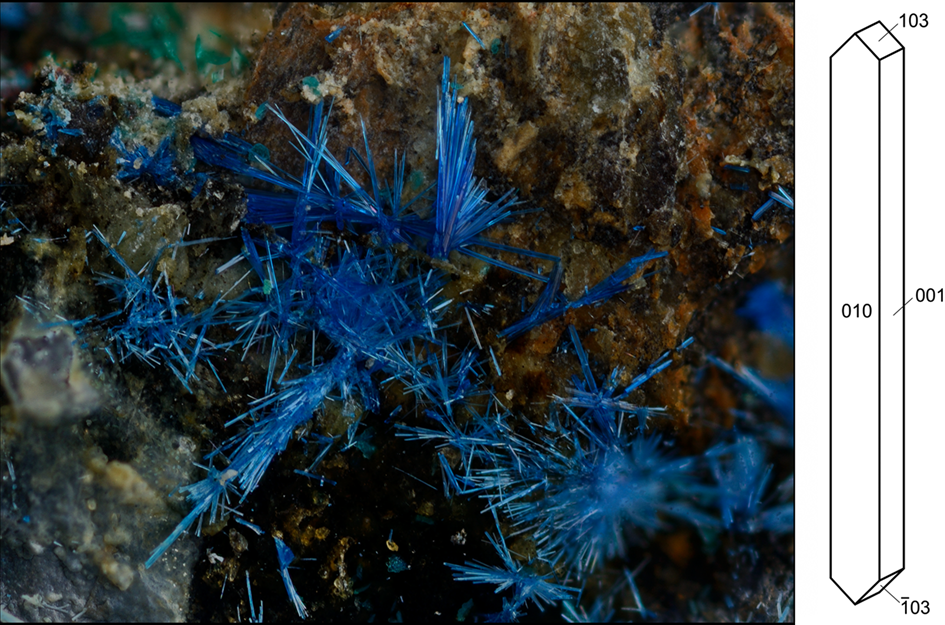
Fig. 1. Isselite, sprays of acicular blue crystals. Field of view: 2 mm. Monte Ramazzo mining complex, Genoa. Photo M. Esposito. The crystal morphology is shown to the right.
Isselite is optically biaxial (–), with indices of refraction α = 1.599(2), β = 1.633(2) and γ = 1.647(2) (determined in white light). The measured 2V of 63.6(5)°, obtained using extinction data analysed with EXCALIBR (Gunter et al., Reference Gunter, Bandli, Bloss, Evans, Su and Weaver2004), compares well with the calculated 2V of 64.2°. Dispersion is moderate, with r > v. The optical orientation is X = b, Y = c and Z = a. Isselite is pleochroic, with X = light blue, Y = blue, Z = blue; X << Z < Y. The compatibility index, calculated using the Gladstone–Dale relationship (Mandarino, Reference Mandarino1979, Reference Mandarino1981), is 0.003 (superior), using the empirical formula and the calculated density; it is 0.021 (excellent) using the empirical formula and the measured density.
Isselite is a late-stage, secondary mineral that crystallised from a low-temperature, aqueous solution. In holotype material, it is associated closely with brochantite and posnjakite. Other associated minerals are pyrrhotite, pentlandite, allophane, chrysocolla and langite. The source of the Cu in isselite may be valleriite, along with minor chalcopyrite, covellite and digenite. These sulfides are widespread in the Monte Ramazzo–Lagoscuro ore deposit, though not in close association with isselite in the samples studied.
Chemical and spectroscopic analyses
Preliminary energy-dispersive spectroscopy (EDS) chemical analyses showed that isselite contains Cu, Ni, Co and S as the only elements with Z > 8. Quantitative chemical analyses were performed using a CAMECA SX50 electron microprobe (Istituto di Geologia Ambientale e Geoingegneria, CNR, Rome) operating in wave-length dispersive mode. Operating conditions were: accelerating voltage 15 kV, beam current 15 nA, and beam diameter 5 μm. Standards (element, emission line) were: periclase (MgKα), baryte (SKα), synthetic Co (CoKα), synthetic Ni (NiKα), synthetic Cu (CuKα) and synthetic Zn (ZnKα). Iron and Pb were sought but found to be below detection limits. The small amount of available material precluded the determination of H2O by direct measurement; consequently, the H2O content was inferred from the crystal structure analysis. Its presence is confirmed by Raman spectroscopy (see below).
The electron microprobe analysis results are given in Table 1. The empirical formula of isselite, based on Σ(Mg,Co,Ni,Cu,Zn) = 6 atoms per formula unit, is (Cu4.80Ni0.94Co0.11Zn0.10Mg0.06)Σ6.00(S1.06O4.19)(OH)10⋅5H2O. The ideal formula of isselite is Cu6(SO4)(OH)10⋅5H2O, which requires SO3 10.86, CuO 64.71, H2O 24.43, total 100.00.
Table 1. Electron microprobe analysis of isselite (average of six spot analyses – in wt.%).

S.D. – standard deviation
Micro-Raman spectra were obtained on an unpolished sample of isselite in nearly back-scattered geometry with a Jobin-Yvon Horiba XploRA Plus apparatus, equipped with a motorised x–y stage and an Olympus BX41 microscope with a 10× objective (Dipartimento di Scienze della Terra, Università di Pisa). The 532 nm line of a solid-state laser was used. The minimum lateral and depth resolution was set to a few μm. The system was calibrated using the 520.6 cm–1 Raman band of silicon before each experimental session. Spectra were collected through multiple acquisitions with single counting times of 60 s. Back-scattered radiation was analysed with a 1200 gr/mm grating monochromator. The Raman spectrum of isselite is shown in Fig. 2. The strongest band occurs at 984 cm–1 and can be related to the symmetrical stretching ν1 of the SO4 group. The bands at 386, 440 and 495 cm–1 can be interpreted as due to the symmetrical bending ν2 of the SO4 group, whereas the weak band at 630 cm–1 could be related to the antisymmetrical bending ν4. Finally, the band at 250 cm–1 may be attributed to lattice modes. The broad band at 3484 cm–1 can be attributed to the O–H stretching modes. No evident bending of H–O–H bonds, in the typical range between 1595–1700 cm–1 (e.g. Kolesov, Reference Kolesov2006), was observed. The interpretation of the spectral bands is in agreement with Frost et al. (Reference Frost, Williams, Martens, Leverett and Kloprogge2004).

Fig. 2. Raman spectrum of isselite in the range 200–1200 cm–1 (a) and 2700–4000 cm–1 (b).
Crystallography
The powder X-ray diffraction pattern of isselite was obtained using a 114.6 mm diameter Gandolfi camera, with Ni-filtered CuKα radiation. The observed powder X-ray diffraction pattern is compared with the calculated one (obtained using the software PowderCell; Kraus and Nolze, Reference Kraus and Nolze1996) in Table 2. Unit-cell parameters, refined from the powder data on the basis of 22 unequivocally indexed reflections through the software UnitCell (Holland and Redfern, Reference Holland and Redfern1997), are a = 6.789(1), b = 5.923(1), c = 20.651(3) Å, V = 830.4(2) Å3 and Z = 2.
Table 2. X-ray powder diffraction (d in Å) data for isselite*.
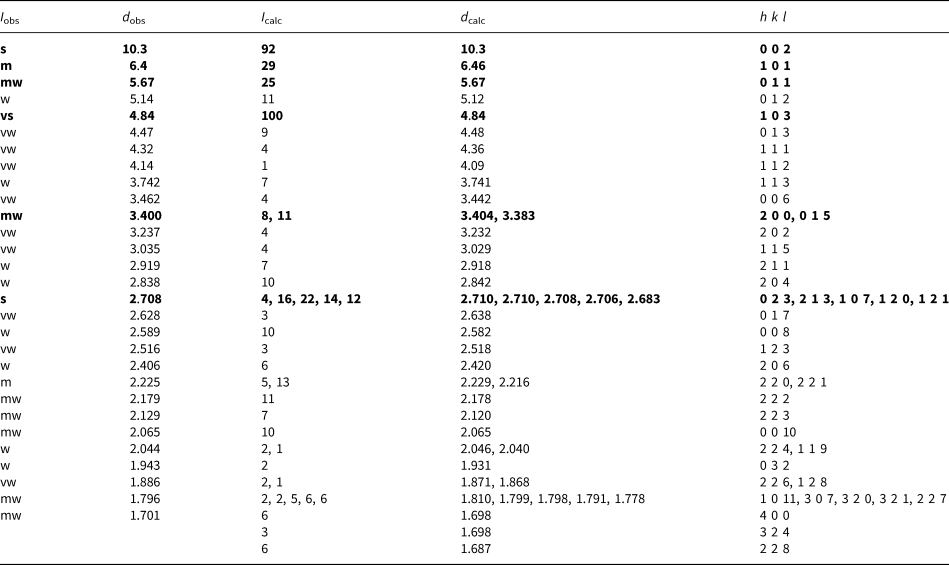
* Notes: the d hkl values were calculated on the basis of the unit cell refined by using single-crystal data. Intensities were calculated on the basis of the structural model reported in Table 4. Observed intensities were visually estimated: vs = very strong; s = strong; m = medium; mw = medium–weak; w = weak; vw = very weak. Only reflections with I calc > 5 are listed, if not observed. The strongest reflections are shown in bold.
The single-crystal X-ray diffraction study was carried out at the XRD1 beamline, ELETTRA synchrotron facility (Lausi et al., Reference Lausi, Polentarutti, Onesti, Plaisier, Busetto, Bais, Barba, Cassetta, Campi, Lamba, Pifferi, Mande, Sarma, Sharma and Paolucci2015). A monochromatic wavelength of 0.59040 Å was used on a 50 μm × 50 μm beam size, using a Dectris Pilatus 2M hybrid pixel area detector at a distance of 85 mm. A total of 500 frames was collected using φ scan mode in Δφ = 0.5° slices, with an exposure time of 1 s per frame. The diffraction data, collected at room temperature, were indexed, integrated, scaled, and corrected for the Lorentz-polarisation factor using the software XDS (Kabsch, Reference Kabsch2010). The refined unit-cell parameters are a = 6.8070(14), b = 5.8970(12), c = 20.653(4) Å and V = 829.0(3) Å3. The a:b:c ratio calculated from unit-cell parameters is 1.1543:1:3.5023.
The statistical tests on the distribution of |E| values (|E 2 – 1| = 0.703) suggest the acentric nature of isselite. The examination of the systematic absences indicated the possible space groups P21nm and Pmn21. Only the choice of this latter space group allowed the solution of the crystal structure through direct methods using Shelxs-97 (Sheldrick, Reference Sheldrick1997). After having located the heavier elements, the structure was completed through successive difference-Fourier maps; owing to the quality of available material, H atoms were not located. After several cycles of anisotropic refinement, performed using Shelxl-2018 (Sheldrick, Reference Sheldrick2015) and using neutral scattering curves taken from the International Tables for Crystallography (Wilson, Reference Wilson1992), the refinement converged to R 1 = 0.0669 for 2964 unique reflections with F o > 4σ(F o) and 140 refined parameters. The occurrence of high electron density residuals (up to 6.19 e –/Å3) is related to the less-than-ideal quality of the available crystals of isselite. An attenuation of the residuals can be obtained by truncating the intensity data to low angular values. For instance, the truncation of the dataset to 30° in 2θ results in a highest residual of 2.3 e –/Å3. However, the ratio between observed reflections and least-square parameters significantly decreases (from ~21 to ~ 4.5). An attempt at truncating the dataset at 2θ = 50° resulted in a slightly better R value (0.0625 for 2269 reflections) and slightly lower residuals (up to 5.4 e –/Å3). However, the estimated standard uncertainties on bond lengths slightly increased. For this reason, the full dataset is here presented, with the awareness of the non ideal quality of the structure refinement (in particular as regards the high residuals) but supported by the crystal-chemical soundness of the proposed structural model.
Details of the data collection and crystal-structure refinement are given in Table 3. Atom coordinates and equivalent isotropic displacement parameters are reported in Table 4, whereas Table 5 gives selected bond distances. Bond-valence calculations, shown in Table 6, were performed using the bond parameters of Brese and O'Keeffe (Reference Brese and O'Keeffe1991). The crystallographic information file has been deposited with the Principal Editor of Mineralogical Magazine and is available as Supplementary material (see below).
Table 3. Crystal data and summary of parameters describing data collection and refinement for isselite.

Table 4. Sites, Wyckoff positions, atom coordinates and equivalent isotropic displacement parameters (Å2) for isselite.

Wyk. = Wyckoff position
Table 5. Selected bond distances (in Å) for isselite.

Table 6. Bond-valence sums (BVS – in valence units) for isselite*.

* Left and right superscripts indicate the number of equivalent bonds involving anions and cations, respectively.
Crystal structure description
General organisation
The crystal structure of isselite (Fig. 3) includes five independent Cu sites, one S site, and fourteen O positions. It can be described as formed by {001} zig-zag layers of CuΦ4–6 polyhedra, where Φ = (OH or H2O). Sulfate groups occur in the interlayer, together with one H2O group. No H positions were located from the structure determination; the assignments of OH and H2O groups were based on the bond-valence analysis (see Table 6).

Fig. 3. Crystal structure of isselite as seen down b. Symbols: green polyhedra = Cu sites and yellow polyhedra = S site. Circles: red = O2–, blue = OH– and light blue = H2O.
Cation coordination and site occupancies
In isselite, the Cu sites display different kinds of coordination (Fig. 4). The Cu(2) and Cu(3) sites show the typically distorted (4 + 2) octahedral coordination of Cu2+, related to the Jahn-Teller effect (e.g. Burns and Hawthorne, Reference Burns and Hawthorne1996). The average value of the four shorter distances is 2.013 Å for both sites, somewhat longer than the equatorial bond length of 1.97 Å reported by Eby and Hawthorne (Reference Eby and Hawthorne1993). The two apical Cu–O distances have average values of 2.237 and 2.197 Å at the Cu(2) and Cu(3) sites, respectively, shorter than the average value of 2.44 Å reported by Eby and Hawthorne (Reference Eby and Hawthorne1993). The smaller than typical octahedral distortions observed for the Cu(2) and Cu(3) sites suggest that minor Ni and Co detected in isselite are located at these two sites. Taking into account the nature of the ligands, Cu(2) and Cu(3) octahedra can be defined both as Cu(OH)5(H2O) polyhedra. The Cu(1) and Cu(5) sites have a square pyramidal coordination, with four equatorial bonds ranging between 1.941 and 1.965 Å (average value of 1.954 Å); the fifth bond is at a longer distance, i.e. 2.571 Å [Cu(5)–Ow(13)] and 2.623 Å [Cu(1)–Ow(12)]. Taking into account the ligand nature, the cations at the Cu(1) and Cu(5) sites can be related to the Cu(OH)4(H2O) polyhedra.
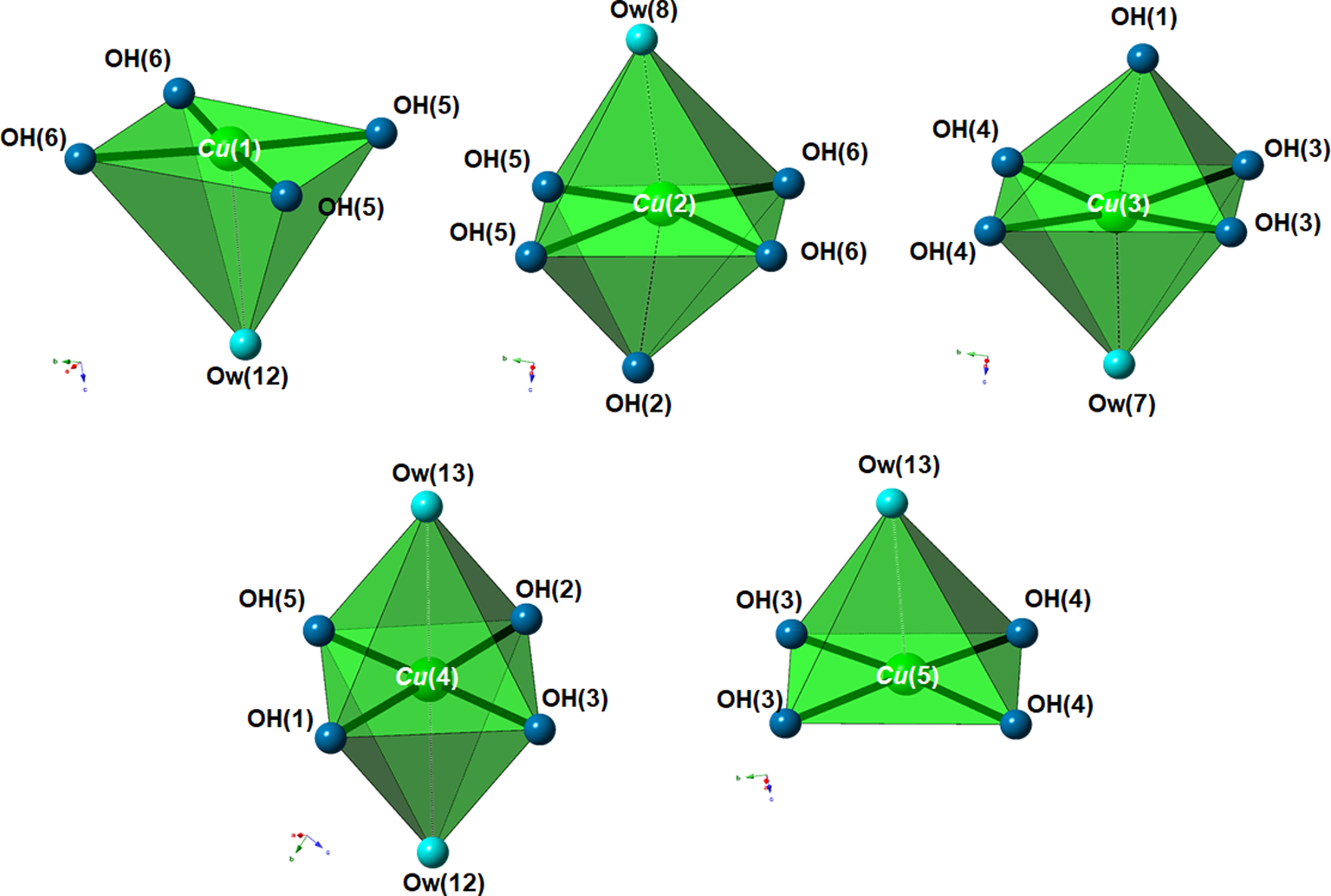
Fig. 4. Coordination environments of Cu2+ in the crystal structure of isselite. The colour scheme is the same as in Fig. 3.
A square-planar coordination is shown by the Cu(4) site, having four ligands at an average distance of 1.944 Å, in agreement with the ideal mean value of 1.94 Å for square-planar Cu2+ (Eby and Hawthorne, Reference Eby and Hawthorne1993). Taking into account the two very long distances Cu(4)–Ow(12) and Cu(4)–Ow(13), ≈2.9 Å, a very distorted octahedron Cu(OH)4(H2O)2 can be identified, instead of a square-planar group Cu(OH)4.
Bond-valence sums (BVS) at the Cu sites range between 1.98 and 2.13 valence units (vu), in agreement with the occurrence of Cu in the divalent state. The higher values occur at the Cu(2) and Cu(3) sites and can be related to the admixture of minor Ni2+ and very small amounts of Co2+, Zn2+ and Mg2+.
The SO4 group is hosted in the interlayer and is bonded to the {001} Cu layers through hydrogen bonds, as revealed by the occurrence of several underbonded O in the bond-valence calculation (Table 6) and the occurrence of short O⋅⋅⋅O distances (Table 7). The BVS at the S site is 6.48 vu, considerably greater than expected for S6+. In this regard, it should be noted that the position of O(11) seems to be affected by disorder, as indicated by a U eq value larger than those of other O atoms belonging to the SO4 group.
Table 7. O⋅⋅⋅O distances (in Å) and corresponding bond strengths (in valence units, vu).

*Calculated using the relationships of Ferraris and Ivaldi (Reference Ferraris and Ivaldi1988).
Hydrogen bonds
The short O⋅⋅⋅O distances are given in Table 7, confirming the importance of H bonds in the crystal structure of isselite, in agreement with the undersaturation of all O atoms. Taking into account the results of BVS given in Table 6, three distinct groups of O atoms can be found: (1) O atoms having BVS close to ~1.6 vu; (2) O atoms with BVS of ~1.2–1.3 vu; and (3) O atoms showing BVS of ~ 0.2 vu. These three different O groups correspond to O2–, (OH)– and H2O groups, respectively.
Oxygen atoms belonging to the isolated SO4 groups, that is O(9), O(10) and O(11), are acceptors of H bonds. Oxygen atom hosted at O(9) is an acceptor of two H bonds from two symmetry-related (OH)– groups hosted at OH(5); the oxygen atom at O(10) is an acceptor of H bonds from two symmetry related (OH)– groups hosted at OH(6) and from an H2O group occurring at the Ow(14) site. Finally, O(11) is involved in a more complex H-bond system. Indeed, O(11) is an acceptor of H bonds from OH(4) and it is at H-bond distances with Ow(7), Ow(8), and Ow(14). In addition, Ow(14) is an acceptor of H bonds from two symmetry-related OH(3) groups, whereas a still more complex situation involves the H2O groups hosted at Ow(7) and Ow(8). These two H2O groups are bonded to the Cu atoms hosted at Cu(3) and Cu(2) sites, respectively. Both are at short O⋅⋅⋅O distances with O(11). In addition, the distance Ow(7)⋅⋅⋅Ow(8), 2.85 Å, suggests another H-bond contact. Owing to the lack of knowledge about the H positions and the occurrence of different possible configurations, the actual H-bond system involving these atoms has to be considered only speculative and it will not be detailed here. As a matter of fact, the possibility of different configurations involving the oxygen atom hosted at O(11) could be the reason for its relatively high U eq value. Its position could be an average position between different H-bond configurations. Moreover, this uncertainty could affect the BVS of S, as discussed briefly above.
Oxygen atoms hosted at OH(4) and OH(6) are both donor (in the H bonds described above) and acceptor from H2O groups hosted at Ow(12) and Ow(13), respectively. It is worth noting that Ow(12) and Ow(13) act as donor to two symmetry-related OH(4) and OH(6), respectively, whereas they are acceptors of H bonds from OH(2) and OH(1), respectively.
Discussion
Isselite and relations with other Cu sulfates
Fifteen different mineral species are currently known in the system CuO–SO3–H2O (Table 8). From a chemical point of view, the most simple species are those having the chemical formula Cu(SO4)⋅nH2O (0 < n < 7): chalcocyanite (n = 0), poitevinite (n = 1), bonattite (n = 3), chalcanthite (n = 5) and boothite (n = 7). Their crystal structures are reviewed by Eby and Hawthorne (Reference Eby and Hawthorne1993). Chalcocyanite typically occurs in fumarolic environments. Indeed, it was first described from Vesuvius, Italy, by Scacchi (Reference Scacchi1873), along with another anhydrous copper oxy-sulfate, dolerophanite, Cu2O(SO4). The other hydrated copper sulfates listed above are usually related to the weathering of Cu ores, although in some case they can be found also as sublimates in fumaroles (e.g. Balassone et al., Reference Balassone, Petti, Mondillo, Panikorovskii, de Gennaro, Cappelletti, Altomare, Corriero, Cangiano and D'Orazio2019).
Table 8. Natural Cu sulfates in the system CuO–SO3–H2O.

1MDO1; 2MDO2.
The remaining species, commonly associated with weathered Cu ores (e.g. Zittlau et al., Reference Zittlau, Shi, Boerio-Gates, Woodfield and Majzlan2013), are represented by the two basic copper sulfates antlerite and brochantite and seven hydrated basic copper sulfates. Isselite belongs to this latter group of copper sulfates, showing the presence of (OH)– and H2O groups. In particular, it is chemically related to redgillite, Cu6(SO4)(OH)10(H2O) (Pluth et al., Reference Pluth, Steele, Kampf and Green2005) and montetrisaite, Cu6(SO4)(OH)10(H2O)2 (Orlandi and Bonaccorsi, Reference Orlandi and Bonaccorsi2009). However, notwithstanding the chemical similarities, the crystal structure of isselite is different from those shown by redgillite and montetrisaite (Fig. 5). In addition, isselite could be the Cu analogue of guarinoite, Zn6(SO4)(OH)10⋅5H2O (Sarp, Reference Sarp1993). However, Mills et al. (Reference Mills, Missen and Favreau2019) noted that guarinoite might have been misidentified in its original description and is more likely to be Na(Zn,Co)4(SO4)(OH)6Cl⋅5-6H2O and equivalent to Co-rich gordaite-2H.

Fig. 5. Comparison between the crystal structure of isselite (a) and those of montetrisaite (b) and redgillite (c). The colour scheme is the same as in Fig. 3.
Nickel partitioning in isselite
After the first identification of isselite on the type specimen from the Lagoscuro mine, this mineral was identified, through powder X-ray diffraction, also on a sample from the Monte Ramazzo mine, as thin flattened acicular crystals associated with brochantite. Only EDS chemical data are available for this new occurrence; however, these data clearly indicated the occurrence of Ni, whereas Co and other elements are below the detection limit. The Ni/(Ni + Cu) atomic ratio is close to 0.19, similar to the value observed in the type material, where the Ni/(Mg + Co + Ni + Cu + Zn) atomic ratio is 0.16. In both cases, the ideal composition is close to NiCu5(SO4)(OH)10⋅5H2O.
The similar scattering factors of Ni (Z = 28) and Cu (Z = 29) precluded the reliable description of the distribution of Ni and Cu atoms among different cation sites. Nickel and Cu are ordered in some minerals. Gillardite and the related mineral paratacamite-(Ni), both ideally Cu3Ni(OH)6Cl2, are atacamite-group minerals where Ni is partitioned in the octahedrally coordinated sites not showing the Jahn-Teller effect, whereas Cu2+ is hosted at the distorted octahedrally coordinated sites (Clissold et al., Reference Clissold, Leverett, Williams, Hibbs and Nickel2007; Sciberras et al., Reference Sciberras, Leverett, Williams, Hibbs, Downes, Welch and Kampf2013). Similarly, Ni2+ is preferentially partitioned in the less distorted octahedrally-coordinated Me2 site of the crystal structure of glaukosphaerite, (Cu,Ni)2(CO3)(OH)2, whereas the Me1 site shows the typical 4 + 2 distorted octahedral coordination of Cu2+ (Perchiazzi and Merlino, Reference Perchiazzi and Merlino2006). Another example is represented by hloušekite. This mineral, having the ideal formula NiCu4(AsO4)2(AsO3OH)2(H2O)9, is a member of the lindackerite supergroup and Ni is hosted at a mixed (Ni,Co) site not showing the typical distortion of Cu2+-centred polyhedra (Plášil et al., Reference Plášil, Sejkora, Škoda, Novák, Kasatkin, Škácha, Veselovský, Fejfarová and Ondruš2014).
In isselite, the Cu(2) and Cu(3) sites have octahedral coordinations, showing the typical (4 + 2) distortion due to the Jahn–Teller effect for Cu2+. As discussed above, these sites have slightly longer equatorial bonds and shorter apical distances with respect to those reported by Eby and Hawthorne (Reference Eby and Hawthorne1993) for a typical Cu2+-centred octahedron. This could suggest the substitution of Cu2+ by Ni2+ at these two positions. However, as there is no certainty about the quantification of Cu–Ni site distribution, for classification purposes the Cu(2) and Cu(3) sites could be merged, as occurs in the nomenclature of other minerals (e.g. M1 + M2 + M3 in amphibole; Hawthorne et al., Reference Hawthorne, Oberti, Harlow, Maresch, Martin, Schumacher and Welch2012). In accord with the IMA–CNMNC guidelines (Bosi et al., Reference Bosi, Hatert, Hålenius, Pasero, Miyawaki and Mills2019), the end-member formula of isselite can be considered Ni-free. However, the actual role of Ni in stabilising this phase deserves further study.
Conclusion
Isselite is a new secondary Cu mineral showing a novel crystal structure and a complex hydrogen-bond system. Its Ni content, observed at both of its occurrences, opens the question about the role of this element in favouring the crystallisation of this new mineral species. It is likely that isselite could be more common than thought as an alteration product of Cu–Ni ores.
Along with ramazzoite (Kampf et al., Reference Kampf, Rossman, Ma, Belmonte, Biagioni, Castellaro and Chiappino2018), isselite is the second new mineral discovered in the assemblages from the Monte Ramazzo mining complex, stressing the necessity for further investigation of this locality. In particular, a deep understanding of the geochemical relations between the Cu–Fe–Ni–Co primary mineralisation and their secondary alteration products would shed light on the possible role of hydrothermal fluids in Ni vs. Co selective mobilisation and enrichment as well as on the degree of hydrothermal alteration of the Monte-Ramazzo–Lagoscuro ore deposit.
Supplementary material
To view supplementary material for this article, please visit https://doi.org/10.1180/mgm.2020.50.
Acknowledgements
Marco Esposito and Fabrizio Castellaro are acknowledged for providing the first specimen of isselite and the photo used in this paper. The comments of three anonymous reviewers improved the quality of the original manuscript.