Staphylococcal mastitis in sheep was first studied towards the end of the 19th century by the French veterinarian and microbiologist Edmund Nocard, who described the experience of a practicing veterinarian of the time as follows: ‘This disease is rightly considered to be the plague of the cheese-making flocks; it is not rare to see one tenth of the animals affected by the terrible evil; those, which recover, are invariably lost for milk-production. All the treatments have been tried equally unsuccessfully; this, what the shepherds have found the best to save the animal, is to cut open the udder in different directions from the start of the disease and to treat the wounds with any detergent lotion. The majority of veterinarians of the country consider this disease as a simple mastitis, caused by the milk engorgement and the knocks given on the mammary gland by the milkers; but the owners refuse to believe in these possible causes of the disease and the majority of them thinks that this mastitis is related to anthrax. This opinion should be rejected, because the animals never exhibit, during their life or after their death, the signs or lesions of anthrax; microscopic examination of the blood has never allowed me to see bacteria …’ (Nocard, Reference Nocard1887).
Nocard (Reference Nocard1887) described staphylococcal mastitis from pathological and microbiological examinations. He isolated the causative microorganism from the milk of an affected ewe and described its morphological, cultural and biochemical characteristics. Also, he studied the effects of experimental intramammary inoculation of mammary secretion from an affected mammary gland, by means of which he successfully reproduced a pathological condition similar to the natural disease. Thereafter, Bridre (Reference Bridre1907) undertook a systematic investigation of the disease in dairy flocks in France. He found that the incidence of the disease was approximately 5%, with a mortality of 20%. Further, he attempted to protect sheep by means of immunisation.
In general, the onset and outcome of staphylococcal infections are dependent on the combination of the virulence of the invading isolate and the defence abilities of the host. Protection of a host against staphylococci is to a large degree dependent upon (a) the integrity of the skin and mucous membranes, which form an important barrier to entrance of staphylococci into the body, and (b) the number and functionality of leucocytes, which are important for phagocytosing and destroying the invading bacteria (Murray et al., Reference Murray, Pearson, Coombs, Flexman, Golledge, Speers, Dyer, McLellan, Reilly, Bell, Bowen and Christiansen2008). Moreover, recent work has also presented a role for anti-staphylococcal antibodies, which seem to clear staphylococcal isolates more effectively (Vasileiou, Reference Vasileiou2019, Vasileiou et al., Reference Vasileiou, Chatzopoulos, Cripps, Ioannidi, Gougoulis, CHouzouris, Lianou, Calvo Gonzalez-Valerio, Guix Vallverdu, Argyros, Cesio, Font, Mavrogianni, Petinaki and Fthenakis2019a).
Staphylococcal infections are usually preceded by colonisation and are characterised by intense tissue inflammation. They occur when the bacteria enter through skin or mucous membrane breakdowns (e.g. injuries, ulcerations, surgical incisions). The invading bacteria multiply locally and produce enzymes and toxins, leading in tissue destruction, influx of polymorphonuclear cells, severe tissue damage and abscess formation, which characterise the staphylococcal infections (Lowy, Reference Lowy1998). By means of this mechanism and in accord with the characteristics of staphylococci, local infections occur initially at the point of entry. Thereafter, more severe infections, consequently to bacterial dissemination in tissues or haematogenously can occur in other areas of the body of the host.
Staphylococci have been isolated from various sites of the body of healthy sheep (online Supplementary Table S1). In sheep, the most important problem caused by staphylococci is mastitis, clinical or subclinical. Moreover, the bacteria have been implicated also in various other disorders (online Supplementary Table S1), the significance of which varies. Some of these occur frequently, e.g. impetigo or abscesses; some sporadically, e.g. vaginal infections or abortion; whilst others have been described only experimentally, e.g. osteomyelitis or rhinosinusitis.
In dairy sheep flocks, mammary infections have an obvious financial significance (Selvaggi et al., Reference Selvaggi, D’ Alessandro and Dario2017), due to the reduction in milk yield, the downgrading of milk quality and the rejection of milk consequently to antibiotic administration. Mammary infections are important also in meat production flocks, as reduced milk yield of ewes has been shown to lead to suboptimal growth of their lambs (Fthenakis and Jones, Reference Fthenakis and Jones1990a). Other costs associated with the disease include those for replacement animals and the relevant veterinary expenses. Mastitis is also a great welfare concern (European Food Safety Authority, 2014).
The objective of this review is to appraise the importance and significance of staphylococci in mastitis in ewes. Although staphylococci are important as causal agents for the disease, there is a lack of a systematic review of relevant knowledge.
Methodology
The review includes primarily references published in journals cited in the Web of Knowledge database (http://www.webofknowledge.com); papers published in these journals have been refereed. Various search terms have been employed to identify relevant publications (e.g. ‘sheep’, ‘goat*’, ‘mastitis’, ‘somatic cell count*’, ‘milk’, ‘staphylococcus’, ‘teat’, ‘carriage’, ‘carrier’). Subsequently, the full papers have been retrieved through the websites of the respective journals. Citations of other material are scarce; these include a few references to book chapters and doctoral theses (i.e. material that has been reviewed) and one reference to edited conference material. References cited in the review appear at the end of the review, and additional supporting references are included in the online Supplementary File.
The microorganism
Classification – taxonomy
Taxonomically, the genus Staphylococcus (S.) is classified in the bacterial family Staphylococcaceae (order: Bacillales, class: Bacilli, phylum: Firmicutes, domain: Bacteria). Currently, the genus includes more than 50 species, with many having subspecies. Apart from speciation, various other taxonomic schemes have been proposed for staphylococci, which are based on phenotypic characteristics or molecular findings.
A well-known approach refers to the reaction of staphylococcal species to the coagulase test, which identifies whether a staphylococcal isolate produces the exoenzyme coagulase: staphylococcal species are classified as coagulase-positive or coagulase-negative, with a third class, coagulase-variable, having been established recently. Another approach refers to natural susceptibility of the species to novobiocin: staphylococcal species are classified as novobiocin-sensitive or novobiocin-resistant. Further, clustering into groups based on results of 16s rRNA sequencing has also been performed and 11 groups have been distinguished (Table 1).
Table 1. Clustering of staphylococcal species into 11 groups based on results of 16s rRNA sequencing
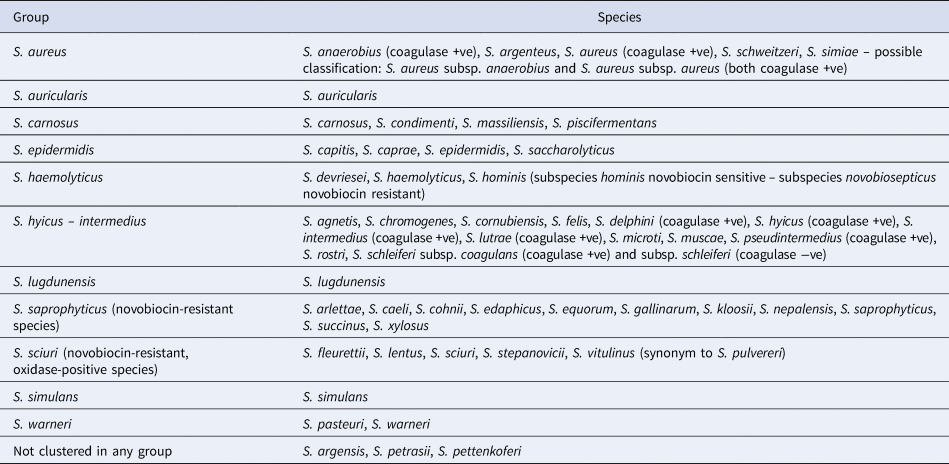
Note. Reports regarding other species, e.g. S. leei (coagulase +ve), S. lyticans, S. pseudolugdunensis, have also been published, but their valid taxonomic status has not yet been confirmed.
Source: Euzeby (Reference Euzeby1997).
Isolation and identification
The procedure of isolation of staphylococci starts with culture of the clinical samples, which for mastitis are, usually, milk samples collected from ewes under examination or from the bulk milk tank. Less often, other types of samples may need to be processed, e.g. swabs from mammary lesions or udder skin, or material from the teat duct or mammary tissue samples (Fragkou et al., Reference Fragkou, Boscos and Fthenakis2014).
Enrichment of samples is not necessary. They can be inoculated directly onto conventional (e.g. blood agar) or selective (e.g. Chapman's medium) media. The plates should be incubated at 35–37 °C for up to 48 h (Fragkou et al., Reference Fragkou, Boscos and Fthenakis2014, Vasileiou et al., Reference Vasileiou, Cripps, Ioannidi, Chatzopoulos, Gougoulis, Sarrou, Orfanou, Politis, Calvo Gonzalez-Valerio, Argyros, Mavrogianni, Petinaki and Fthenakis2018b). If no apparent growth has occurred the media can be reincubated for a further 24 h. Due to the tolerance of staphylococci in increased salt concentrations (as high as 10%), mannitol salt agar is an appropriate selective medium (e.g. Chapman's medium) for growth and isolation. Chromogenic agar can be used for the isolation of S. aureus (Ariza-Miguel et al., Reference Ariza-Miguel, Oniciuc, Sanz, Fernandez-Natal, Hernandez and Rodriguez-Lazaro2015). False negative results may always occur with culture technologies. A prolonged transportation time or inappropriate maintenance of samples can trigger bacterial survival mechanisms, e.g. slime production and biofilm formation. In such cases, the bacteria do not produce obvious colonies when transferred onto agar plates (Skrlin, Reference Skrlin2016). Further, in long-standing infections, staphylococci may not grow on solid media (Ehrlich et al., Reference Ehrlich, Demeo, Palmer, Sauber, Altman, Altman, Sotereanos, Conti, Baratz, Maale, Hu, Post, Nistico, Kreft, Hall-Stoodley, Costerton, Stoodley, Ehrlich, DeMeo, Costerton and Winkler2014).
Evaluation of the appearance of colonies is the first step in identification of staphylococci. Staphylococcal species usually form distinctive colonies on sheep blood agar, being smooth and butyrous, with a low convex profile and an entire edge (Winn and Koneman, Reference Winn and Koneman2006). Most Staphylococcus aureus isolates are pale light yellow colonies, due to staphyloxanthin, a carotenoid pigment with a notable role in pathogenicity of the bacteria (Lan et al., Reference Lan, Cheng, Dunman, Missiakas and He2010). Other species, most often, produce white-coloured colonies.
Microscopically, Gram-staining confirms a Gram-positive microorganism. Staphylococcal colonies are differentiated from other Gram-positive bacteria (e.g. streptococci, enterococci) by the catalase test, which detects cytochrome oxidase enzymes, staphylococci show a positive reaction in the test. This is followed by the coagulase test, which serves to distinguish coagulase-positive isolates (S. anaerobius, S. aureus, S. delphini, S. hyicus, S. intermedius, S. lutrae, S. pseudintermedius and S. schleiferi subsp. coagulans).
Thereafter, identification to species level can be by commercial assays which use modified carbohydrate fermentation tests or adaptations of standard bacteriological identification biochemical tests (Donay et al., Reference Donay, Mathieu, Fernandes, Pregermain, Bruel, Wargnier, Casin, Weill, Lagrange and Herrmann2004), or by molecular identification techniques, e.g. the Polymerase Chain Reaction (PCR) or Matrix-Assisted Laser Desorption/Ionisation-Time of Flight (MALDI-TOF). The latter analyses the protein profile of bacterial cells (Katsafadou et al., Reference Katsafadou, Tsangaris, Billinis and Fthenakis2015).
MALDI-TOF technology allows a greater number of bacterial isolates to be rapidly and accurately identified to species level (Cameron et al., Reference Cameron, Perry, Middleton, Chaffer, Lewis and Keefe2018, Hulland et al., Reference Hulland, Dufour and Munoz2018). This is particularly relevant for coagulase-negative staphylococci, the identification of which to species level is often omitted and thus these organisms are cumulatively reported to the genus level only.
Virulence factors
Pathogenicity of staphylococcal infections is based on the virulence factors of the bacteria that allow them to survive within the host and cause relevant damage. The bacteria carry a wide array of potential virulence factors (Table 2), being capable of (i) adhering to host tissues, (ii) avoiding, overcoming or invading the host immune system and (iii) releasing harmful toxins or enzymes (Sahuquillo Arce et al., Reference Sahuquillo Arce, Yarad Auad, Hernández Cabezas and Méndez-Vilas2013). During infections, the bacteria produce the respective virulence determinants in a sequence tightly coordinated by relevant regulatory systems. The expression of both virulence factors and regulatory mechanisms is controlled by specific virulence genes. Factors contributing to the pathogenicity of staphylococci can be classified as (i) bacterial cell surface components (adherence factors) and (ii) secreted variants.
Table 2. Virulence factors involved in the pathogenetic role of a typical S. aureus isolate
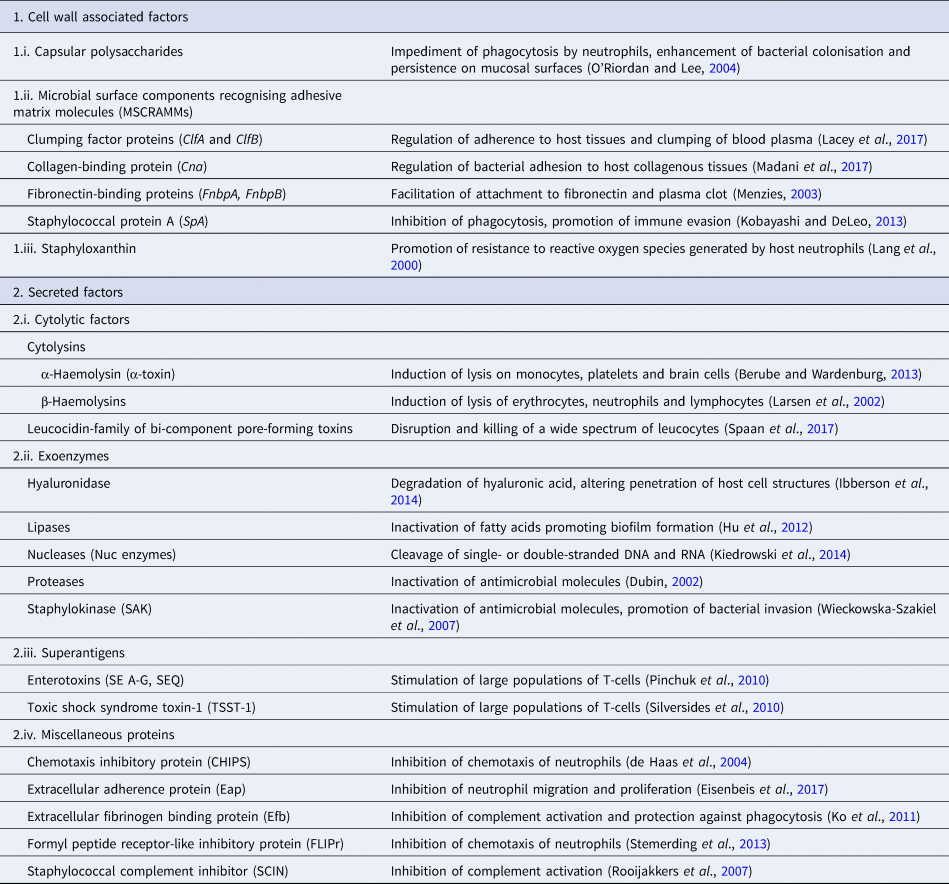
Adherence factors include various proteins which mainly act during the early phase of infection. Their principal function is to facilitate attachment of the bacteria to the host cell surface, simultaneously leading to the cascade host immune response evasion (Foster and Höök, Reference Foster and Höök1998). Various capsular polysaccharides, located on the bacterial cell wall, are involved in the inhibition of phagocytosis by neutrophils, while teichoic acids are implicated in bacterial adherence to the mucosal surface. Most of these are microbial surface components recognising adhesive matrix molecules including fibrinogen, fibronectin and collagens. Staphylococcal protein A (SpA), fibronectin-binding protein A and fibronectin-binding protein B (FnbpA and FnbpB), collagen-binding protein and clumping factor A and B proteins have also been determined to play a keynote role in the virulence of staphylococci (Palmqvist et al., Reference Palmqvist, Foster, Tarkowski and Josefsson2002, Heying et al., Reference Heying, van de Gevel, Que, Moreillon and Beekhuizen2007).
Slime production by staphylococcal isolates contributes to biofilm formation by these bacteria and plays an important role in the pathogenesis of staphylococcal infections and mastitis specifically (Schönborn et al., Reference Schönborn, Wente, Paduch and Krömker2017), particularly at the early stage of the infection, when the bacteria adhere to the mammary epithelial cells. Genes icaA, icaB, icaC, icaD are responsible for production of icaADBC-encoded polysaccharide intercellular adhesion and ica-independent chemically diverse slime (Cramton et al., Reference Cramton, Gerke, Schnell, Nichols and Götz1999, Reference Cramton, Ulrich, Götz and Doring2001). More specifically, the icaA gene product is a transmembrane protein which, for optimal action, requires presence of the icaD gene product. The icaC gene encodes a product contributing to chain formulation of N-acetyl-glucosamine oligomers produced by the icaAD combination (Gerke et al., Reference Gerke, Kraft, Sussmuth, Schweitzer and Götz1998); this product is also considered to be involved in translocation of the polysaccharide on cell surface (Gerke et al., Reference Gerke, Kraft, Sussmuth, Schweitzer and Götz1998). Τhe icaB-encoded protein is considered responsible for the bacterial evasion process from the phagocytosis process (Cerca et al., Reference Cerca, Jefferson, Maira-Litrán, Pier, Kelly-Quintos, Goldmann, Azeredo and Pier2007). Moreover, the clfa gene encodes a surface protein, demonstrated as clumping factor A, which has a crucial role in bacterial initial attachment and evasion of host immune responses (Stutz et al., Reference Stutz, Stephan and Tasara2011). The bap gene also encodes an important surface protein, termed ‘biofilm associated protein’; beyond its contribution to initial bacterial attachment, it has also been considered that this protein is capable to induce a polysaccharide intercellular adhesion/poly-N-acetyl glucosamine-independent slime production process, especially on abiotic surfaces (Latasa et al., Reference Latasa, Solano, Penadés and Lasa2006). Finally, the eno gene encodes a laminin-binding surface protein (Carneiro et al., Reference Carneiro, Postol, Nomizo, Reis and Brentani2004).
Secreted virulence factors are mainly present during the late phase of infection and usually have a more distinct role in microbial pathogenicity (Otto, Reference Otto2013). Based on their principal activity, secreted virulence determinants are further classified in four categories: (i) super-antigens, (ii) cytolytic toxins, (iii) exoenzymes and (iv) miscellaneous proteins. Tissue shock syndrome toxin-1 (TSST-1) and enterotoxins are the most prominent superantigens, usually causing clinical conditions of increased severity. Cytolytic toxins (i.e. α-haemolysin, β-haemolysin, γ-haemolysin, toxins of the leucocidin family) are capable of forming pores in the host cell wall, causing the osmotic leakage of cell content and, therefore, lysis of the cell; cytolysis provides the required nutrients for further growth of the bacteria. The extracellular enzymes are produced by most staphylococcal isolates and aim to inactivate host antimicrobial mechanisms, allowing bacterial dissemination. These exoenzymes include various lipases, nucleases and proteases (i.e. hyaluronidase, serine, cysteine, staphylokinase). Finally, many other proteins have been shown to have a further impact on the host immune system; staphylococcal complement inhibitor protein, extracellular fibrinogen binding protein, chemotaxis inhibitory protein and formyl peptide receptor-like protein-1 inhibitory protein are the ones most frequently detected (Otto, Reference Otto2013).
Synthesis of these factors occurs during the two growth phases of the bacteria. During the early phase, cell wall-associated factors facilitate tissue attachment and evasion of host defence system, allowing staphylococci to accumulate and, possibly, also form a biofilm. and the late phase, during which bacteria secrete a spectrum of exoproteins including proteinases, haemolysins and super-antigens, whilst, at the same time, cell wall-associated factors are downregulated, leading to enhancement of the biofilm and bacterial dissemination within the mammary gland (Novick, Reference Novick2003). The production of the various virulence factors of the bacteria is controlled by various mechanisms, following the general strategy of the microbial adhesion, invasion and proliferation. The function of regulation systems is in response of bacterial cell density (quorum-sensing) and environmental factors (e.g. nutrient availability, pH, temperature, oxygen tension). Moreover, it is also noteworthy that a virulence determinant may be under the influence of several regulatory systems that act synergistically to ensure the appropriate conditions for bacterial survival (Wang and Muir, Reference Wang and Muir2016).
In vitro studies have shown that regulation mechanisms can be classified into two major groups: (i) the two-component regulatory systems and (ii) the global transcriptional regulators (Cheung et al., Reference Cheung, Bayer, Zhang, Gresham and Xiong2004). At nucleotide level, the two-component regulatory systems include the staphylococcal accessory element (sae locus) and the accessory gene regulator (agr locus). The sae locus codes the expression of several virulence determinants, mainly contributing to bacterial adhesion and host immune evasion. The agr-coded genes seem to promote the expression of bacterial exoproteins (e.g. TSST-1, enterotoxins, serine proteinase), simultaneously reducing the synthesis of cell-wall associated proteins (e.g. SpA, FnbpA, FnbB). This system was initially described in S. aureus, in which four distinct allelic variants, agrA/Β/C/D, have been sequenced. Subsequently, presence of homologous agr-like loci in other staphylococcal species have been detected (Dufour et al., Reference Dufour, Gillet, Bes, Lina, Vandenesch, Floret, Etienne and Richet2002). Most S. aureus isolates have a third significant group of virulence genes regulators, usually referred as sigma factors (σ).
Mechanisms of resistance to antibacterial agents
A major attribute of most staphylococcal species (including S. aureus) is their extended capacity to develop, fairly rapidly, resistance to antimicrobial agents. Nowadays, multi-drug resistant staphylococci (e.g. S. aureus, S. epidermidis, S. intermedius) are commonly recovered from human or animal clinical samples (Mediavilla et al., Reference Mediavilla, Chen, Mathema and Kreiswirth2012).
Antimicrobial tolerance or resistance is linked to the genetic background of each individual isolate and develops through mutations and rearrangements within the staphylococcal genome or by acquisition of resistance determinants. The wide spectrum of staphylococcal genetic variants and the increased antibiotic pressure contribute significantly in antibiotic resistance formation. Most resistance determinants of staphylococcal genetic material are in highly volatile areas (e.g. genomic islands, plasmids) promoting the occurrence of mutations leading to antibiotic resistance (Foster, Reference Foster2017). Further, during co-infections, genetic transfer may also take place and essential resistant components may easily be transferred horizontally. Finally, genetic transfer of resistant genetic elements between bacteria belonging to different species has also been recorded (Haaber et al., Reference Haaber, Penadés and Ingmer2017).
In general, mechanisms of resistance against antibiotics developed by staphylococci are: (i) relevant modulations of cell wall permeability, (ii) enzymatic inactivation of the antimicrobial agent, (iii) modification of the antibiotic target and (iv) activation of relevant bacterial efflux pumps (McCallum et al., Reference McCallum, Berger-Bächi and Senn2010). Depending on specific antimicrobial agents, one or more of these mechanisms may be involved (Pantosti et al., Reference Pantosti, Sanchini and Monaco2007).
β-Lactams and glycopeptides are antimicrobial agents that inhibit the formation of the staphylococcal cell wall. The penicillin-binding protein (PBP) 1 and PBP 2 are the target of β-lactams, which inhibit the function of PBPs and, therefore, the formation of an intact cell wall. To overcome the effects of β-lactams, methicillin-resistant S. aureus (MRSA) isolates produce a fifth additional PBP named PBP 2a, encoded by the mecA gene, which has reduced affinity with β-lactams and remains active in the presence of β-lactams (Lovering et al., Reference Lovering, Gretes, Safadi, Danel, De Castro, Page and Strynadka2012). Glycopeptides (e.g. vancomycin) attach to the dipeptide D-ala/D-Ala and inhibit the function of PBP. S. aureus isolates with intermediate susceptibility to vancomycin have a remarkably modified architecture on their cell wall, lacking most of the crucial targets of glycopeptides (McGuinness et al., Reference McGuinness, Malachowa and DeLeo2017). Regarding resistance to tetracyclines, the main mechanism is associated with the energy-dependent efflux of tetracycline encoded by the tetA(K) and tetA(L) genes (Khosravi et al., Reference Khosravi, Jenabi and Montazeri2017); recent findings have indicated an association between presence of genes encoding for tetracycline-resistance and for biofilm-formation in staphylococcal isolates (Vasileiou et al., Reference Vasileiou, Sarrou, Papagiannitsis, Chatzopoulos, Malli, Mavrogianni, Petinaki and Fthenakis2019b). With regards to acquisition of resistance to aminoglycosides, resistant isolates are capable to release cytoplasmic aminoglycoside modifying enzymes, which inhibit ribosome binding. Finally, resistance to macrolides and to lincosamides is mainly due to ribosomal modification encoded by the erm genes.
Implication of staphylococci in mastitis in ewes
Implication in clinical mastitis
S. aureus is the primary causal agent of clinical mastitis in ewes. The incidence risk is probably less than 7% across a lactation (Bergonier and Berthelot, Reference Bergonier and Berthelot2003, Arsenault et al., Reference Arsenault, Dubreuil, Higgins and Belanger2008). Field reports have indicated that staphylococci have been isolated from up to 70% of cases of clinical mastitis in field investigations in dairy production flocks (Bergonier and Berthelot, Reference Bergonier and Berthelot2003, Mork et al., Reference Mork, Waage, Tollersrud, Kvitle and Sviland2007). In meat production flocks, staphylococci have been reported to be less frequent, but still responsible for up to 40% of cases of the disease (Arsenault et al., Reference Arsenault, Dubreuil, Higgins and Belanger2008). Other coagulase-positive staphylococcal species associated with clinical mastitis in ewes include S. anaerobius, S. hyicus, S. intermedius and S. schleiferi (Table 3).
Table 3. Selected references regarding implication of non-S. aureus staphylococcal strains in mastitis or mammary carriage in ewes

Coagulase-negative staphylococci have been isolated from cases of clinical mastitis, although much less frequently than S. aureus. These include S. epidermidis, S. saprophyticus, S. simulans, S. xylosus and S. warneri (Table 3). Further, Fthenakis and Jones (Reference Fthenakis and Jones1990b) have reported experimentally induced clinical mastitis caused by S. chromogenes.
Implication in subclinical mastitis
Subclinical mastitis is more common than clinical disease. Criteria employed for definition of subclinical mastitis are important in determining its prevalence; for example, in a field investigation, in which strict criteria had been used, prevalence of subclinical mastitis was 26% (Vasileiou et al., Reference Vasileiou, Cripps, Ioannidi, Chatzopoulos, Gougoulis, Sarrou, Orfanou, Politis, Calvo Gonzalez-Valerio, Argyros, Mavrogianni, Petinaki and Fthenakis2018b, Reference Vasileiou, Gougoulis, Riggio, Ioannidi, Chatzopoulos, Mavrogianni, Petinaki and Fthenakis2018c), whilst in other field studies, with less strict criteria, prevalence was found to be as high as 40%, although the accuracy of detection of cases has been questioned (Vasileiou et al., Reference Vasileiou, Cripps, Ioannidi, Chatzopoulos, Gougoulis, Sarrou, Orfanou, Politis, Calvo Gonzalez-Valerio, Argyros, Mavrogianni, Petinaki and Fthenakis2018b). It is also noteworthy to cite a report of epidemic occurrence of subclinical mastitis in a flock, where prevalence of the disease has been reported to be 94%, with all cases caused by coagulase-negative staphylococci (Fthenakis et al., Reference Fthenakis, Leontides, Skoufos, Taitzoglou and Tzora2004).
A clear consensus exists in the literature that coagulase-negative staphylococcal species are the primary aetiological agents of subclinical mastitis and can constitute up to 70% of bacterial isolates from cases of subclinical disease (Gelasakis et al., Reference Gelasakis, Mavrogianni, Petridis, Vasileiou and Fthenakis2015). The more frequent staphylococcal species recovered from cases of subclinical mastitis were S. chromogenes, S. epidermidis, S. simulans and S. xylosus. Other species recovered as aetiological agents of the disease less often include S. auricularis, S. capitis, S. caprae, S. cohnii, S. equorum, S. haemolyticus, S. hominis, S. lentus, S. saprophyticus, S. sciuri, S. schleiferi, S. warneri (Table 3).
S. aureus has also been recovered as the causative agent of subclinical mastitis, but much less frequently than from cases of clinical disease (Vasileiou et al., Reference Vasileiou, Cripps, Ioannidi, Chatzopoulos, Gougoulis, Sarrou, Orfanou, Politis, Calvo Gonzalez-Valerio, Argyros, Mavrogianni, Petinaki and Fthenakis2018b). Sporadically, S. aureus may account for as many as 40% of isolates from cases of clinical mastitis (Al-Majali and Jawabreh, Reference Al-Majali and Jawabreh2003).
Mammary carriage
The term mammary ‘carriage’ (or ‘carrier state’) (Verhoeven et al., Reference Verhoeven, Gagnaire, Botelho-Nevers, Grattard, Carricajo, Lucht, Pozzetto and Berthelot2014) describes the presence of bacteria in the udder with no increased somatic cell numbers (i.e. in the absence of inflammation). The term refers to bacterial flora present in the teat duct or teat cistern (Fragkou et al., Reference Fragkou, Mavrogianni, Cripps, Gougoulis and Fthenakis2007, Mavrogianni et al., Reference Mavrogianni, Cripps and Fthenakis2007). By using culture-independent methods of microbial identification (e.g. MALDI-TOF), recent work performed in cows has suggested the existence of a microbiota in the teat and the lactiferous ducts; a possible source of these bacteria can be the intestine, as it has been found that bacteria from the gut microbiota reach the mammary gland within leucocytes (e.g. dendritic cells, macrophages, lymphocytes) (Rainard, Reference Rainard2017). Vasileiou et al. (Reference Vasileiou, Cripps, Ioannidi, Chatzopoulos, Gougoulis, Sarrou, Orfanou, Politis, Calvo Gonzalez-Valerio, Argyros, Mavrogianni, Petinaki and Fthenakis2018b) have indicated that the prevalence of staphylococcal mammary carriage in ewes was 6.5%. The significance of mammary carriage is that the microorganisms might become pathogenic under the effect of various factors, which decrease defensive efficacy of hosts or promote pathogenicity of bacteria. In a previous experimental study, Fragkou et al. (Reference Fragkou, Mavrogianni, Cripps, Gougoulis and Fthenakis2007) have shown that, under the effect of factors reducing efficacy of teat defences, staphylococcal carriage could multiply, ascend to the mammary parenchyma and, ultimately, cause clinical mastitis.
Cases of false positive mammary carriage refer to isolation of bacteria at the very early stage of infection before an inflammatory reaction would be elicited (Fthenakis and Jones, Reference Fthenakis and Jones1990b), as well as in cases of contamination of milk samples during collection. Nevertheless, with experienced staff and strict maintenance of aseptic sampling, contamination should be minimal; for example, Rovai et al. (Reference Rovai, Caja, Salama, Jubert, Lázaro, Lázaro and Leitner2014), in a field investigation, found that only 2% of milk samples from ewes were contaminated.
Characteristics of isolates with regards to potential virulence factors
Typing of S. aureus from cases of clinical mastitis has produced varying results regarding the presence of agr locus. Vautor et al. (Reference Vautor, Carsenti-Dellamonica, Sabah, Mancini, Pepin and Dellamonica2007) indicated that 70% of isolates belonged to the agr group III and 13% to the agr group I. In contrast, de Almeida et al. (Reference de Almeida, de Almeida, de Mendonça and Mamizuka2013) indicated that isolates from clinical mastitis belonged mostly to the agr group I (38%) and less often to the agr group II (19%), whilst isolates from subclinical mastitis were equally distributed to these two groups (38% in each). Finally, Bar-Gal et al. (Reference Bar-Gal, Blum, Hadas, Ehricht, Monecke and Leitner2015) reported that all S. aureus isolates from mastitis, clinical or subclinical, belonged to the agr group I.
Further, there is disagreement regarding the significance of biofilm-forming staphylococci from cases of mastitis in ewes varying from 0% (Azara et al., Reference Azara, Longheu, Sanna and Tola2017) to 26% (Vautor et al., Reference Vautor, Carsenti-Dellamonica, Sabah, Mancini, Pepin and Dellamonica2007) to 40–43% (Ergun et al., Reference Ergun, Aslantas, Kirecci, Ozturk, Ceylan and Boyar2012) to 91–98% (Tel et al., Reference Tel, Aslantas, Keskin, Yilmaz and Demir2012) of relevant isolates found to form biofilms. However, phenotypic results of biofilm formation depend upon the criteria employed for assessment and, moreover, isolates may not always express a biofilm-forming phenotype, despite carrying genes associated with slime production and biofilm-formation (Vasileiou et al., Reference Vasileiou, Chatzopoulos, Gougoulis, Sarrou, Katsafadou, Spyrou, Mavrogianni, Petinaki and Fthenakis2018a). The prevalence of subclinical mastitis caused specifically by biofilm-forming staphylococci (independently of species) was found to be 15.5%, with hand-milking recognised as the most important risk factor (Vasileiou et al., Reference Vasileiou, Chatzopoulos, Gougoulis, Sarrou, Katsafadou, Spyrou, Mavrogianni, Petinaki and Fthenakis2018a).
Examination of staphylococci by Multi Locus Sequence Typing also revealed varying and inconsistent findings. Porrero et al. (Reference Porrero, Hasman, Vela, Fernandez-Garayzabal, Dominguez and Aarestrup2012) examined S. aureus strains from clinical mastitis and found that they belonged to types ST9, ST133, ST1739 and ST2011. de Almeida et al. (Reference de Almeida, de Almeida, de Mendonça and Mamizuka2013) reported that S. aureus strains from clinical mastitis belonged to types ST750, ST1728, ST1729 and ST1730, whilst those from subclinical mastitis to types ST750, ST1728 and ST1729. Finally, Bar-Gal et al. (Reference Bar-Gal, Blum, Hadas, Ehricht, Monecke and Leitner2015) reported that S. aureus strains from clinical or subclinical mastitis belonged to types ST133 and ST522. In general, S. aureus ST133 is most often isolated from samples of sheep origin (McMillan et al., Reference McMillan, Moore, McAuley, Fegan and Fox2016).
In studies which had investigated production of virulence factors by staphylococci (S. aureus or coagulase-negative isolates) from cases of mastitis, various factors or genes encoding for shock syndrome toxin-1 (Orden et al., Reference Orden, Goyache, Hernandez, Domenech, Suarez and Gomez Lucia1992b, Scherrer et al., Reference Scherrer, Corti, Muehlherr, Zweifel and Stephen2004), leucocidin (Burriel and Dagnall, Reference Burriel and Dagnall1997), Panton-Valentine toxin (Unal and Cinar, Reference Unal and Cinar2012), exfoliative toxins (Mariutti et al., Reference Mariutti, Souza, Ullah, Caruso, de Moraes, Zanphorlin, Tartaglia, Seyffert, Azevedo, Le Loir, Murakami and Arni2015), haemolysins (Azara et al., Reference Azara, Longheu, Sanna and Tola2017) and autolysin (Azara et al., Reference Azara, Longheu, Sanna and Tola2017) have been detected. The presence of these factors was, in general, more prevalent in S. aureus than in coagulase-negative isolates, which can explain the increased pathogenicity of the former.
Resistance to antibacterial agents of isolates from cases of mastitis in ewes
There is one rule for the effective treatment of mastitis: the combination of speed and efficacy. As soon as mastitis is diagnosed, effective antimicrobial agents should be administered (Mavrogianni et al., Reference Mavrogianni, Menzies, Fragkou and Fthenakis2011). Ideally, and to preserve susceptibility of pathogens to available drugs, treatment should be a narrow spectrum antibiotic known to be effective against staphylococci.
Recent evidence from around Europe does not indicate significant problems of resistance to antibiotics commonly used for cases of mastitis in sheep. Vautor et al. (Reference Vautor, Carsenti-Dellamonica, Sabah, Mancini, Pepin and Dellamonica2007) reported only sporadic resistance in S. aureus isolated in France. Onni et al. (Reference Onni, Sanna, Larsen and Tola2011), in Italy, also found limited resistance in S. epidermidis, except to penicillin, for which the resistance rate was 38%. Similar results have been observed in Turkey, where in coagulase-negative isolates from subclinical mastitis only resistance to β-lactams was noteworthy (43%), whilst there was much smaller frequency of resistance to tetracycline (11%) and even less to other agents (Ergun et al., Reference Ergun, Aslantas, Kirecci, Ozturk, Ceylan and Boyar2012). Further work in Turkey corroborated those findings, the rate of resistance to penicillin was 27% and to tetracycline 8% (Unal et al., Reference Unal, Askar, Macun, Sakarya, Altun and Yildirim2012). Martins et al. (Reference Martins, Faccioli, Bonesso, Fernandes, Oliveira, Dantas, Zafalon and Cunha2017) published similar results; 17% of isolates were resistant to penicillin and 11% to tetracycline. Finally, evidence from Greece was consistent with the above, as the frequency of resistant isolates was <25% for all the antimicrobial agents evaluated (Vasileiou et al., Reference Vasileiou, Sarrou, Papagiannitsis, Chatzopoulos, Malli, Mavrogianni, Petinaki and Fthenakis2019b). Different findings have been reported by Azara et al. (Reference Azara, Longheu, Sanna and Tola2017), who found greater resistance to tetracycline (50%) of S. aureus from clinical mastitis. In contrast to the above results, in Brazil, Franca et al. (Reference Franca, Peixoto, Cavalcante, Melo, Oliveira, Veschi, Mota and Costa2012) determined a higher resistance to amoxicillin, erythromycin, lincomycin, streptomycin and tetracycline (>35% of staphylococcal isolates tested).
Whilst the above results are indicative of the possibility for effective treatment of clinical mastitis, in vitro results of antimicrobial susceptibility do not always correspond with results of in vivo administration of an antibacterial agent. Various reasons may account for this discrepancy, e.g. pharmacokinetic limitations during clinical application, inappropriate treatment regime or iatrogenic infection occurring during treatment (Mavrogianni et al., Reference Mavrogianni, Menzies, Fragkou and Fthenakis2011).
Staphylococcal mastitis in sheep
Sources of infection
Staphylococci usually disseminate into the mammary gland from the hands of milkers (Marco Melero, Reference Marco Melero1994, Vasileiou et al., Reference Vasileiou, Chatzopoulos, Gougoulis, Sarrou, Katsafadou, Spyrou, Mavrogianni, Petinaki and Fthenakis2018a). The use of bare hands during milking contributes to transfer of bacteria to the teat, when the duct is open.
Other potential sources of bacteria include the nasopharynx of sucking lambs (Gougoulis et al., Reference Gougoulis, Kyriazakis, Tzora, Taitzoglou, Skoufos and Fthenakis2008), staphylococci already present in the teat duct (Fragkou et al., Reference Fragkou, Mavrogianni, Cripps, Gougoulis and Fthenakis2007) and staphylococci present on the udder skin (Mavrogianni et al., Reference Mavrogianni, Cripps and Fthenakis2007). Albenzio et al. (Reference Albenzio, Taibi, Caroprese, De Rosa, Muscio and Sevi2003) considered that lambs' mouths and milkers' hands were the major sources of ewe udder and milk contamination, whilst few staphylococci and streptococci were isolated from the teat cups. During suckling, the teat orifice comes into contact with the wall of the buccal cavity of lambs. As Laukova and Marounek (Reference Laukova and Marounek1992) have isolated staphylococci form the upper alimentary tract of lambs, it is evident that the bacteria can transmit to the teat during sucking As the lower part of the teat comes into contact with the pharynx of the lamb (Titchen, Reference Titchen1977) the bacteria subsequently enter the duct; perhaps; the tongue of the lamb may ‘push’ the bacteria upwards into the duct. Isolation of the microorganism after only a short (1 min) sucking activity indicates the speed by which the whole process can take place (Gougoulis et al., Reference Gougoulis, Kyriazakis, Tzora, Taitzoglou, Skoufos and Fthenakis2008). Staphylococci have been found as resident flora of the teat duct of healthy ewes (Fragkou et al., Reference Fragkou, Mavrogianni, Cripps, Gougoulis and Fthenakis2007, Mavrogianni et al., Reference Mavrogianni, Cripps and Fthenakis2007). When the microbial equilibrium is disrupted for any reason, it is possible that pathogenicity of the flora isolates increases, leading to invasion of the mammary parenchyma and disease. Further, physicochemical changes occurring of udder skin, e.g. in response to bad weather conditions or harsh teat disinfectants, may create chapping increasing the susceptibility of the mammary gland. In the epidermis, drying results in a lower lipid content thus less antibacterial fatty acids, bacteriostatic salts and proteins, as well as immunoglobulins (Noble and Somerville, Reference Noble, Somerville and Noble1974). Additionally, the reduced hydration of chapped skin, alters the skin microflora, consequently decreasing resistance to bacterial colonisation (Fox et al., Reference Fox, Nagy, Hillers, Cronrath and Ratkowsky1991). Chapping removes the acid mantle and increases teat surface area, due to excoriations and fissuring, thus providing additional surface for bacterial attachment. All these increase bacterial populations on the udder skin, thus increasing risk of infection of the mammary parenchyma (Mavrogianni et al., Reference Mavrogianni, Cripps and Fthenakis2007).
Giannakopoulos et al. (Reference Giannakopoulos, Vasileiou, Gougoulis, Cripps, Ioannidi, Chatzopoulos, Billinis, Mavrogianni, Petinaki and Fthenakis2019) have modelled for the first time areas of high risk for the development of staphylococcal mastitis, taking into account data regarding prevalence of the disease in the field and environmental and locality conditions, using the ecological niche modelling approach. Whilst the work referred to Greece, there is the possibility to extent the findings to cover other parts of the world.
Course of the disease
The general principles of mammary defences apply for staphylococcal infections. After successful ascent of staphylococci into the teat cistern and the mammary parenchyma, leucocytes constitute the main line of defence against staphylococci. These are developed immediately upon bacterial entry into the mammary gland, 2–4 h after that and 3–4 d later, respectively (Fthenakis and Jones, Reference Fthenakis and Jones1990b, Fragkou et al., Reference Fragkou, Dagleish, Papaioannou, Cripps, Boscos, Ververidis, Orfanou, Solomakos, Finlayson, Govaris, Kyriazakis and Fthenakis2010). An increased diameter of mammary vessels, a greater blood volume of blood passing through the gland increase soon after infection, and an increased permeability of the blood–milk barrier, by various mechanisms (e.g. by modulating claudins at the tight mammary junctions), lead to the transportation of an increased amount of blood constituents into the mammary gland (Barbagianni, Reference Barbagianni2016). Thus, the inflammatory response is developed and sustained. Neutrophils phagocytose bacteria and perform intracellular killing by the rapid release of reactive oxygen species: superoxide radicals and hydrogen peroxide (‘respiratory burst’) (Van Oostveldt et al., Reference Van Oostveldt, Paape, Dosogne and Burvenich2002). Neutrophils also release various antibacterial proteins, e.g. cathelicidins, which become available at the mammary parenchyma (Katsafadou et al., Reference Katsafadou, Tsangaris, Anagnostopoulos, Billinis, Barbagianni, Vasileiou, Spanis, Mavrogianni and Fthenakis2019).
Neutrophils can also kill staphylococci extracellularly, by means of extracellular traps, which have been described for the first time in sheep by Pisanu et al. (Reference Pisanu, Cubeddu, Pagnozzi, Rocca, Cacciotto, Alberti, Marogna, Uzzau and Addis2015). These represent a mesh of DNA, histones, antimicrobial proteins and proteinases that entrap and inactivate the invading staphylococci with no direct contact or engulfment necessary (Phillipson and Kubes, Reference Phillipson and Kubes2011).
Interleucins play an important role in the host response, as they regulate the influx of leucocytes in milk after bacterial invasion. Albenzio et al. (Reference Albenzio, Caroprese, Santillo, Ruggieri, Ciliberti and Sevi2012) have reported the relationships between bacteria, leucocytes and interleucins produced after bacterial invasion (TNF-α, IL-8, IL-1β, IL-10, IL-12). Concentrations of TNF-α and IL-12 were higher after invasion of pathogenic bacteria (including staphylococci); IL-8 was associated with somatic cell counts and pathogenic bacteria. Regarding IL-1β, Albenzio et al. (Reference Albenzio, Caroprese, Santillo, Ruggieri, Ciliberti and Sevi2012) have not observed any differences after intramammary staphylococcal challenge, whilst other authors have (Winter and Colditz, Reference Winter and Colditz2002, Winter et al., Reference Winter, Schilcher, Fuchs and Colditz2003).
Production of virulence factors by the invading staphylococci leads, principally, to destruction of mammary epithelial cells and leucocytes, as well as blood vessels within the mammary parenchyma and consequentially results in intramammary haemorrhages (Fthenakis and Jones, Reference Fthenakis and Jones1990b). Further, biofilm formation allows rapid diffusion of the bacteria, which thus become difficult to control. Occasionally, pathogens that had not been eliminated by the mammary defences pass into the blood circulation. Further, staphylococci can survive in leucocytes, especially if these are not fully functioning, e.g. due to lack of energy in the immediately post-partum period (Fthenakis et al., Reference Fthenakis, Mavrogianni, Gallidis and Papadopoulos2015). This could lead to bacterial accumulations and abscessation within the mammary parenchyma, whence a recrudescence of the infection can ensue (Fthenakis and Jones, Reference Fthenakis and Jones1990b).
The various virulence factors in mastitis-associated staphylococci have not been detected consistently in all isolates tested and there are significant differences even within the various species of the microorganism. This underlines the importance of the host defences in the development and outcome of an intramammary infection. Potential development of clinical mastitis or limitation of the infection to subclinical mastitis depends upon the combination of virulence factors of the invading bacteria and the efficacy of the defence mechanisms of the host. There is now evidence confirming that cellular defences are the determining host factor that may limit a virulent isolate to only cause subclinical mastitis (Barbagianni et al., Reference Barbagianni, Mavrogianni, Katsafadou, Spanos, Tsioli, Galatos, Nakou, Valasi, Gouletsou and Fthenakis2015, Mavrogianni et al., Reference Mavrogianni, Papadopoulos, Gougoulis, Gallidis, Ptochos, Fragkou, Orfanou and Fthenakis2017). Cell defences are non-specific, acting against all invading bacteria, including staphylococci. A factor that can affect efficacy of cell defences is the breed of sheep (Riggio and Portolano, Reference Riggio and Portolano2015). Potentially, subclinical mastitis can convert to clinical, if defences of the host are impaired. Complete recovery requires a fully functioning defence system of the animals, coupled with an effective course of treatment. Other possible outcomes include the development of a long-standing (‘chronic’) infection with periodic flare-ups of clinical disease, the necrosis of mammary parenchyma, leading to partial or extensive tissue slough-off, the formation of intramammary abscesses with presence of staphylococci therein and the recrudescence of disease. The role of humoral defences has not been studied in depth. Nevertheless, in a recent study, anti-staphylococcal antibodies detected after vaccination of ewes have been found to contribute to improved clearance of staphylococci, but not to fully prevent establishment of the infection (Vasileiou et al., Reference Vasileiou, Chatzopoulos, Cripps, Ioannidi, Gougoulis, CHouzouris, Lianou, Calvo Gonzalez-Valerio, Guix Vallverdu, Argyros, Cesio, Font, Mavrogianni, Petinaki and Fthenakis2019a).
Vaccination against the disease
As part of udder health management, various measures can be implemented for prevention of mastitis. Most of these have a broad spectrum of efficacy and are relevant for prevention of staphylococcal mastitis as well. Such measures include various management practices, e.g. application of post-milking teat disinfection and correct use and maintenance of milking systems.
Vaccination is a targeted means for prevention of staphylococcal mastitis. In the past, inactivated vaccines with whole staphylococcal cells and/or toxoids have been developed against mastitis (Watson, Reference Watson1988), offering mainly reduction in severity of clinical signs (not reduction in incidence risk). A vaccine with inactivated S. aureus–S. simulans whole cells and S. aureus exopolysaccharide antigens within liposomes reduced the incidence risk of the disease (Amorena et al., Reference Amorena, Baselga and Albizu1994). More recently, Perez et al. (Reference Perez, Prenafeta, Valle, Penades, Rota, Solano, Marco, Grillo, Lasa, Irache, Maira-Litran, Jimenez-Barbero, Costa, Pier, de Andres and Amorena2009) described the induction of antibodies against the poly-N-acetyl β−1,6 glucosamine exopolysaccharide, the main component of the extracellular matrix of staphylococcal biofilms. A vaccine has been produced which includes a S. aureus bacterin containing bacterial cells surrounded by components of the biofilm matrix. The vaccine elicits an exopolysaccharide specific antibody response offering some protection against S. aureus mastitis (Prenafeta et al., Reference Prenafeta, March, Foix, Casals and Costa2010) and has contributed to prevention of slime production by staphylococcal isolates, thus limiting their growth and dissemination within and outside the mammary gland. Vasileiou et al. (Reference Vasileiou, Chatzopoulos, Cripps, Ioannidi, Gougoulis, CHouzouris, Lianou, Calvo Gonzalez-Valerio, Guix Vallverdu, Argyros, Cesio, Font, Mavrogianni, Petinaki and Fthenakis2019a) described the efficacy of this vaccine in reducing the incidence risk of staphylococcal mastitis in an extensive field study, offering protection against S. aureus and coagulase-negative staphylococci. Autogenous staphylococcal vaccines are of limited use in specific flocks and do not have a wide applicability. There are no reports of their efficacy, as they are produced for ‘tailor-made’ administration in flocks with perceived problem of staphylococcal mastitis, without first being evaluated for efficacy.
Public health considerations
Staphylococcal enterotoxins are in a family of over 20 exotoxins, which are related and have a sequence homology (Pinchuk et al., Reference Pinchuk, Beswick and Reyes2010). The enterotoxins can cause significant diseases in people, among these food poisoning and toxic shock syndrome. Enterotoxins are mainly produced by S. aureus, although other staphylococcal species may also show enterotoxigenic properties. Staphylococcal food poisoning is an intoxication occurring consequently to consumption of foods containing sufficient amounts of one (or more) pre-formed enterotoxins (Angeles Argudin et al., Reference Angeles Argudin, Carmen Mendoza and Rosario Rodicio2010). Hence, it becomes evident that if milk collected from sheep was subjected to appropriate thermal processing leading to staphylococcal killing there would be no real danger of enterotoxin poisoning from dairy products from that milk. In contrast, the danger would increase sharply with consumption of unprocessed milk, given the frequency of staphylococcal mammary infections, especially as production of enterotoxin has been detected in S. aureus isolated from intramammary infections (Orden et al., Reference Orden, Cid, Blanco, Quiteria, Gomez Lucia and de la Fuente1992a, Reference Orden, Goyache, Hernandez, Domenech, Suarez and Gomez Lucia1992b) or the farm milk tank (Scherrer et al., Reference Scherrer, Corti, Muehlherr, Zweifel and Stephen2004).
For these same reasons, dairy products have not been considered to be implicated in the dissemination of antimicrobial resistance to consumers of milk. Nevertheless, recent studies have documented that cell-free genetic material from staphylococci resistant to antimicrobial agents, not destroyed during thermal processing of milk, could be transferred to humans (Wang et al., Reference Wang, McEntire, Zhang, Li and Doyle2012, Schwarz et al., Reference Schwarz, Loeffler and Kadlec2017). Given that resistance genes could be incorporated in other bacterial species (e.g. Streptococcus spp., Acinetobacter spp.), which are part of the normal bacterial flora of humans, dissemination of resistance genes can occur, potentially making staphylococci ‘stores’ of resistant genes and dairy products a means for their transfer.
Concluding remarks
Staphylococci are the most frequent mastitis pathogens in ewes. Extensive research has clarified various facets of their pathogenicity for the mammary gland of sheep. Some of that knowledge has been based in relevant work performed in cattle. However, the situation with cattle and sheep differs significantly, not only in terms of differences between the animal species but also between management practices in the respective industries. In relation to ovine mastitis, there are still areas, which have not been fully clarified, whilst further questions have arisen from the findings of recent work.
The identification of a supposed microbiota within the mammary gland has implications in the interpretation of the results of bacteriological testing of milk samples from cases of mastitis; much more work is needed to confirm any, or the absence of a, pathogenic role of bacteria. The microbiota has implications for the immune response of the mammary gland as these bacteria may contribute to a defensive role of the gland.
The recent detection of anti-staphylococcal antibodies in cases of mastitis raises questions regarding their significance in contributing to immunological enhancement of the defence response.
Further, the identification of biofilm-forming staphylococci (independently of species) as causal agents of mastitis, i.e. as an independent disease entity beyond staphylococcal species, raises issues regarding the dynamics of infection by these organisms. Their presence in milking parlours and relevant factors contributing in their dissemination have not been studied.
Finally, the identification, in dairy products, of cell-free genetic material of strains resistant to antimicrobial agents adds another public health concern. Pasteurised milk had previously been considered of low risk for the transfer of resistance genes, but the new findings indicate a further route for dissemination of such genes. This puts further pressure in maintaining udder health and limiting staphylococcal involvement as a mammary pathogen, with a view to minimising public health concerns.
Supplementary material
The supplementary material for this article can be found at https://doi.org/10.1017/S0022029919000591.