Introduction
Hydrology, topography, and the spatiotemporal dynamics of canopy gaps are among the main abiotic factors that determine the composition, structure, and richness of palm communities at both local and landscape scales (Eiserhardt et al. Reference Eiserhardt, Svenning, Kissling and Balslev2011). These factors strongly influence the functional response of the plants (Gratani Reference Gratani2014, Gross et al. Reference Gross, Robson, Lavorel, Albert, Le Bagousse-Pinguet and Guillemin2008, Ishii & Asano Reference Ishii and Asano2010, Pearcy Reference Pearcy, Pugnaire and Valladares2007, Salgado-Negret et al. Reference Salgado-Negret, Canessa, Valladares, Armesto and Pérez2015).
Hydrology determines the availability or amount of excess water in the soil. Elongation of shoots is a strategy to avoid anoxic stress by keeping the aerial parts of plants above the water level. This has been identified as a functional response of plants to survive in flooded areas (Blom & Voesenek Reference Blom and Voesenek1996, Voesenek & Bailey-Serres Reference Voesenek and Bailey-Serres2015, Vormisto et al. Reference Vormisto, Tuomisto and Oksanen2004). There are few published works on the functional response of palms to flooding (Balslev et al. Reference Balslev, Kahn, Millan, Svenning, Kristiansen, Borchsenius, Pedersen and Eiserhardt2011). Compared with palms growing in well-drained soils, palms that grow in floodplain forests (FPs) have different tolerances and preferences (Clark et al. Reference Clark, Clark, Sandoval and Castro1995); nevertheless, it is not clear how functional traits vary in response to these environmental traits. Palms may also be specific to particular microhabitats within these habitat types, and fine-scale palm distributions may depend on heterogeneity in terms of soil moisture and drainage (Svenning Reference Svenning1999, Reference Svenning2001).
The density and size of tree-fall gaps importantly affect forest dynamics because they generate variation in light availability and temperature (Kern et al. Reference Kern, Montgomery, Reich and Strong2014). The gradient in light availability gives rise to increased richness (Muscolo et al. Reference Muscolo, Bagnato, Sidari and Mercurio2014) and causes physiological and morphological responses in some understory species (Gratani Reference Gratani2014, Ishii & Asano Reference Ishii and Asano2010, Pearcy Reference Pearcy, Pugnaire and Valladares2007) depending on their tolerance to shade and the amount of increase in light after the formation of a gap. For palms, it has been suggested that large arborescent species depend on tree-fall gaps for recruitment (De Granville Reference De Granville1991, Kahn Reference Kahn1986). However, some species, such as Euterpe edulis, do not grow in open areas, such as large gaps; this phenomenon has been attributed to growth, survival and hydraulic traits, because photoinhibition and hydraulic limitations can affect growth rates (Gatti et al. Reference Gatti, Campanello, Villagra, Montti and Goldstein2014).
Topography is another environmental factor with important effects on plant growth and vegetation development (Den Dubbelden & Knops Reference Den Dubbelden and Knops1993). Soil on slopes experiences a relatively high degree of runoff, and such habitats favor species that evade wet and flooded areas (Méndez-Toribio et al. Reference Méndez-Toribio, Ibarra-Manríquez, Navarrete-Segueda and Paz2017). The local distributions of many palm species are significantly influenced by topography (Clark et al. Reference Clark, Clark, Sandoval and Castro1995, Kahn Reference Kahn1986, Svenning Reference Svenning1999). However, palm species distribution patterns vary from one place to another because topography has no direct influence on itself but rather functions through its correlations with other environmental variables, such as drainage, formation of catenas, exposure of originally different sediment layers, and forest architecture (Vormisto et al. Reference Vormisto, Tuomisto and Oksanen2004).
The ecology and evolution of palms is strongly linked to interspecific variation in the growth, reproduction, and morphology of stems, leaves, inflorescences, fruits, and seeds (Kissling et al. Reference Kissling, Balslev, Baker, Dransfield, Göldel, Lim, Onstein and Svenning2019). Moreover, the functional traits of the dominant species in a community determine ecosystem processes (Conti & Díaz Reference Conti and Díaz2013, Finegan et al. Reference Finegan, Peña-Claros, De Oliveira, Ascarrunz, Bret-Harte, Carreño-Rocabado, Casanoves, Díaz, Eguiguren Velepucha and Fernandez2015, Hobbie Reference Hobbie2015). Palms constitute a noticeable structural and functional component in the Amazon Forest (Gentry Reference Gentry1988); compared with other common species, they are well represented among trees and can reach very high population densities (Ter Steege et al. Reference Ter Steege, Pitman, Sabatier, Baraloto, Salomão, Guevara, Phillips, Castilho, Magnusson and Molino2013), making them an ideal group for evaluating ecological responses because they are diverse, they are gregarious, and they are locally abundant (Terborgh & Andresen Reference Terborgh and Andresen1998).
This article investigates the relationships between local environmental variables and functional traits of palms in the Colombian Amazon, with the goal of contributing to the knowledge of the functional response and strategies of neotropical groups of high ecological importance, such as palms. The question asked is as follows: How are the functional composition of the palm community and the functional traits of palm species related to environmental characteristics (soil moisture, slope and gaps) in a locality in the northeastern region of the Colombian Amazon?
Methods
Study area
The study was performed along the Guaviare River in the eastern region of the Colombian Amazon in the departments of Guainía and Vichada (Figure 1), where three forest types are recognized. One type is terra firme forests, which are never flooded and generally have infertile and well-drained soils, with high local diversity. The second type is FPs, which are usually flooded annually. The third type is forests on terraces that are never or only very rarely flooded, and these include several ancient never-flooded terraces derived from rivers where they used to flow (Balslev et al. Reference Balslev, Copete, Pedersen, Bernal, Galeano, Duque, Berrio, Sanchez and Myster2017, Villota Reference Villota2005). The data collected along the Guaviare River form part of a larger dataset covering 546 transects in palm communities covering the western region of the Amazon (Balslev et al. Reference Balslev, Copete, Pedersen, Bernal, Galeano, Duque, Berrio, Sanchez and Myster2017, Reference Balslev, Kristiansen and Muscarella2019).

Figure 1. Study area along the Guaviare River in the Colombian Amazon. The triangles represent the position of 29 transects (500×5 m) in which all adult individuals of 25 species of palms were identified and counted.
Data collection
We established 29 transects of 500×5 m each, which were subdivided into 100 subunits of 5×5 m. The transects were established in the terra firme forests (11), in floodplains (14), and on terraces (4). In each transect, all adult palm individuals were registered, and on the basis of their size, it was determined whether they had reached the reproductive stage. The palm species were documented with voucher specimens under Rodrigo Bernal’s numbers 4442–4541 deposited at the Colombian National Herbarium in Bogotá (COL), with duplicates at the Aarhus University Herbarium (AAU). The specimens can be seen on the Aarhus University Herbarium database (https://www.aubot.dk/search_form.php). Further details of the collection and census methodology can be found in the study of Balslev et al. (Reference Balslev, Navarrete, Paniagua-Zambrana, Pedersen, Eiserhardt and Kristiansen2010, Reference Balslev, Kristiansen and Muscarella2019).
In each transect, the following environmental variables were recorded: (1) Presence/absence of gaps in each subunit of 5 × 5 m, with the subunits with gaps subsequently counted to obtain a value between 0 and 100 per transect; (2) moisture on the soil surface in subunits of 5×5 m within each transect, recorded as 0 or 1 for dry soil or muddy soil and/or stagnant water, respectively; with the subunits with values of 1 subsequently counted to obtain a value between 0 and 100 per transect; and (3) slope measured in degrees (0–90°) in each subunit of 5×5 m, which were averaged to obtain a single value per transect.
Palm functional traits were determined based on the literature (Galeano & Bernal Reference Galeano and Bernal2010, Henderson Reference Henderson2011) and by examining specimens deposited in the Herbario Nacional Colombiano (COL). Nine functional traits were recorded for each palm species (Table 1): lifeform (LF; cespitose = ces, solitary = sol), growth form (GF; acaulescent = aca, erect = ere, climbing = cli), maximum stem height (StH), maximum leaf number (LN), maximum petiole length (PeL), maximum leaf rachis length (RL), maximum fruit diameter (FD), seed number per fruit (SN), and breeding system (BS, dioecious = dio, monoecious = mon) (Supplement 1).
Table 1. Functional traits and abundances of 25 palm species registered in 29 transects, each covering 500×5 m, equalling 0.25 ha each or a total of 7.25 ha for all transects. The species names are according to the World Flora Online (worldfloraonline.org).
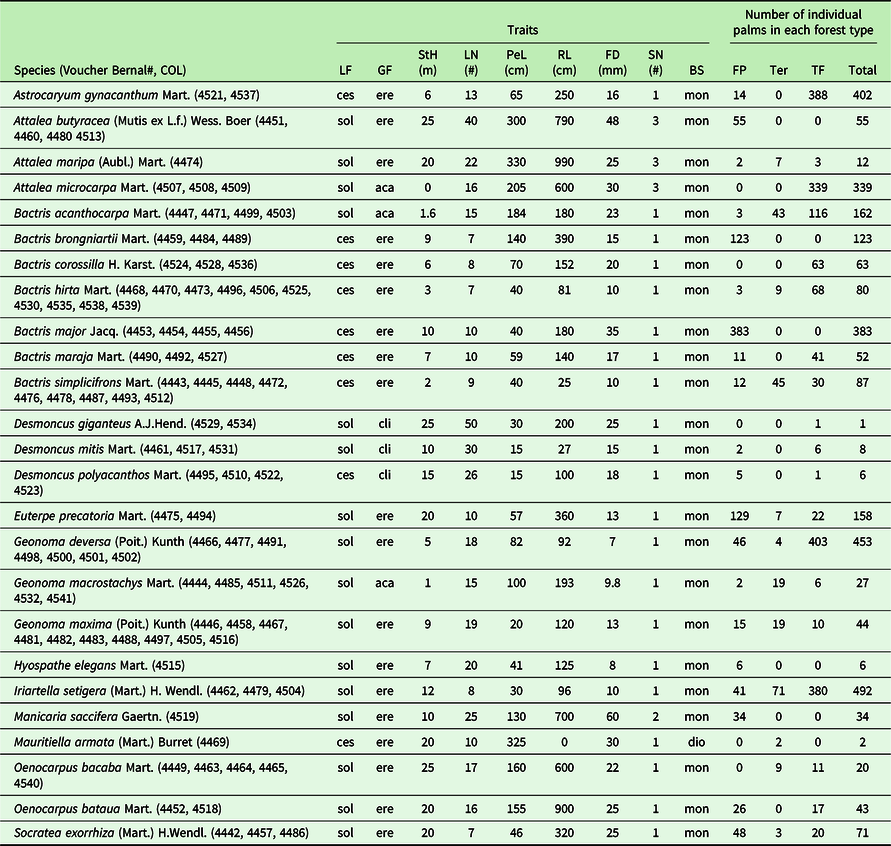
Functional traits: LF=lifeform (cespitose=ces, solitary=sol), GF=growth form (acaulescent=aca, erect=ere, climbing=cli), StH= maximum stem height, LN= maximum leaf number, PeL= maximum petiole length, RL= maximum leaf rachis length, FD=maximum fruit diameter, SN=seed number, BS=breeding system (dioecious=dio, monoecious=mon). Forest type: TF=terra firme, FP=floodplain and Ter=terrace.
Data analysis
The following continuous traits were categorized to show their distribution and frequencies among the forest types: maximum stem height (StH; short=0−8 m, medium=9−17 m, tall=18–25 m); maximum leaf number (LN; low=7−21, medium=22−35, high=36−50); maximum petiole length (PeL; short=45−120 cm; medium=121−225 cm; long=226−330 cm); maximum leaf rachis length (RL; =0−263 cm, medium=264−526 cm, long=527−750 cm); maximum fruit diameter (FD; small=7−24 mm, medium=25−42 mm, large=43−60 mm); and seed number per fruit (SN; 1, 2 or 3 seeds). With the field data, three tables were constructed: matrix Q (q x m) = 9 functional traits of 25 palm species (Table 1); matrix R (n x p) = environmental variables of 29 transects; and matrix L (n x q) = palm abundance in the 29 transects (Supplement 2).
The functional trait composition was calculated from the community-weighted means of traits (CWM) using the formula of Garnier et al. (Reference Garnier, Lavorel, Ansquer, Castro, Cruz, Dolezal, Eriksson, Fortunel, Freitas and Golodets2007). The CWM was obtained from the combination of the abundance (L) and trait (Q) matrices. The CWM calculates a trait value for each transect (Kleyer et al. Reference Kleyer, Dray, Bello, Lepš, Pakeman, Strauss, Thuiller and Lavorel2012) from the mean trait value, weighted by the abundance of all the species present in that transect (for continuous traits), or the weighted proportion of species (for categorical traits). The CWM corresponds to the weighted mean of the trait in the community (Díaz et al. Reference Díaz, Lavorel, De Bello, Quétier, Grigulis and Robson2007, Lavorel et al. Reference Lavorel, Grigulis, McIntyre, Williams, Garden, Dorrough, Berman, Quétier, Thébault and Bonis2008).
Redundancy analysis (CWM-RDA) was used to establish the relationships between functional composition (CWM) and environmental factors (Kleyer et al. Reference Kleyer, Dray, Bello, Lepš, Pakeman, Strauss, Thuiller and Lavorel2012). The categorical variable ‘forest type’ was included in the analysis as a passive variable. Additionally, RLQ (Dolédec et al. Reference Dolédec, Chessel, Ter Braak and Champely1996), fourth corner (Dray et al. Reference Dray, Choler, Dolédec, Peres-Neto, Thuiller, Pavoine and Ter Braak2014), and Pearson correlation (Sokal & Rohlf Reference Sokal and Rohlf2000) analyses were used. All analyses were performed using R statistical software (R Core Team 2016) and the Vegan and Ade-4 packages (Dray & Dufour Reference Dray and Dufour2007).
To test if the observed trait–environment relationships are potentially driven by phylogenetic covariation with other traits, we related our data to the most comprehensive palm phylogeny to date (Faurby et al. Reference Faurby, Eiserhardt, Baker and Svenning2016), using the version of the phylogeny that includes full taxonomic constraints. We visually explored the distribution of the traits across the phylogenetic tree, calculated phylogenetic signal, and statistically related mean trait values across communities to phylogenetic eigenvectors. These analyses were performed in R using the phangorn (Schliep Reference Schliep2011), picante (Kembel et al. Reference Kembel, Cowan, Helmus, Cornwell, Morlon, Ackerly, Blomberg and Webb2010), and PCPS (Debastiani & Duarte Reference Debastiani and Duarte2014) packages.
Results
We recorded 3123 mature individuals from 25 palm species in the 29 transects that together encompassed a total of 7.25 ha (Table 1). The most diverse genus was Bactris, with seven species, followed by Attalea, Desmoncus, and Geonoma, each with three species. The most abundant species was Iriartella setigera, with 492 individuals, followed by Geonoma deversa and Astrocaryum gynacanthum, with 453 and 402 individuals, respectively. Astrocaryum jauari, Bactris bidentula, and Astrocaryum acaule were identified and collected in the area, but they were not included in the analysis because they were collected only outside the transects.
Most individuals (62%) were solitary. The cespitose palms were evenly distributed between the floodplains and terra firme forests, while the solitary palms were more frequent in the terra firme forests (Figure 2A). Most individuals (83%) had erect growth forms. Notably, 99% of the acaulescent palms grew in the terra firme and terrace forests (Figure 2B).

Figure 2. Relative frequency distribution of functional traits in a palm community in floodplain (FP), terrace (Ter), and terra firme forest types along the Guaviare River in the northeastern region of the Colombian Amazon.
The highest proportion of individuals had short stems (52%), particularly in the terra firme forests, whereas tall stems were more frequent in the FPs (Figure 2C). Most individuals had few leaves (96%). The individuals with high and medium numbers of leaves were in the FP (Figure 2D). Of all individuals, 75% had short petioles (Figure 2E). Moreover, most individuals had short leaf rachises (73%); medium-rachis individuals and species were predominant in the FPs, and long-rachis individuals were better represented in the terra firme forests (Figure 2F).
Most individuals (70%) had small fruits, and all large-fruited palms grew in the FPs (Figure 2G). Most individuals (86%) had one seed in each fruit. Individuals with three seeds were best represented in the terra firme forests, while all individuals with two seeds were in the FPs (Figure 2H). Most of the individuals palm were monoecious (99.9%); the only two dioecious individuals were in the terrace forests (Figure 2I).
Environmental variables and palm functional traits
Redundancy analysis (CWM-RDA) showed that the two first axes of the environmental variables (gaps, moisture, slope, and FT) explained 22% (P = 0.002) of the variation in the functional composition (Figure 3). Water in the soil surface (moisture) and the FPs was associated with positive values of axis 1, while the slope (slope) and terra firme forests (TF) were associated with the negative values of these axes. The presence of gaps (Gap) and terrace forests (Ter) were associated with positive values of axis 2 (Figure 3).
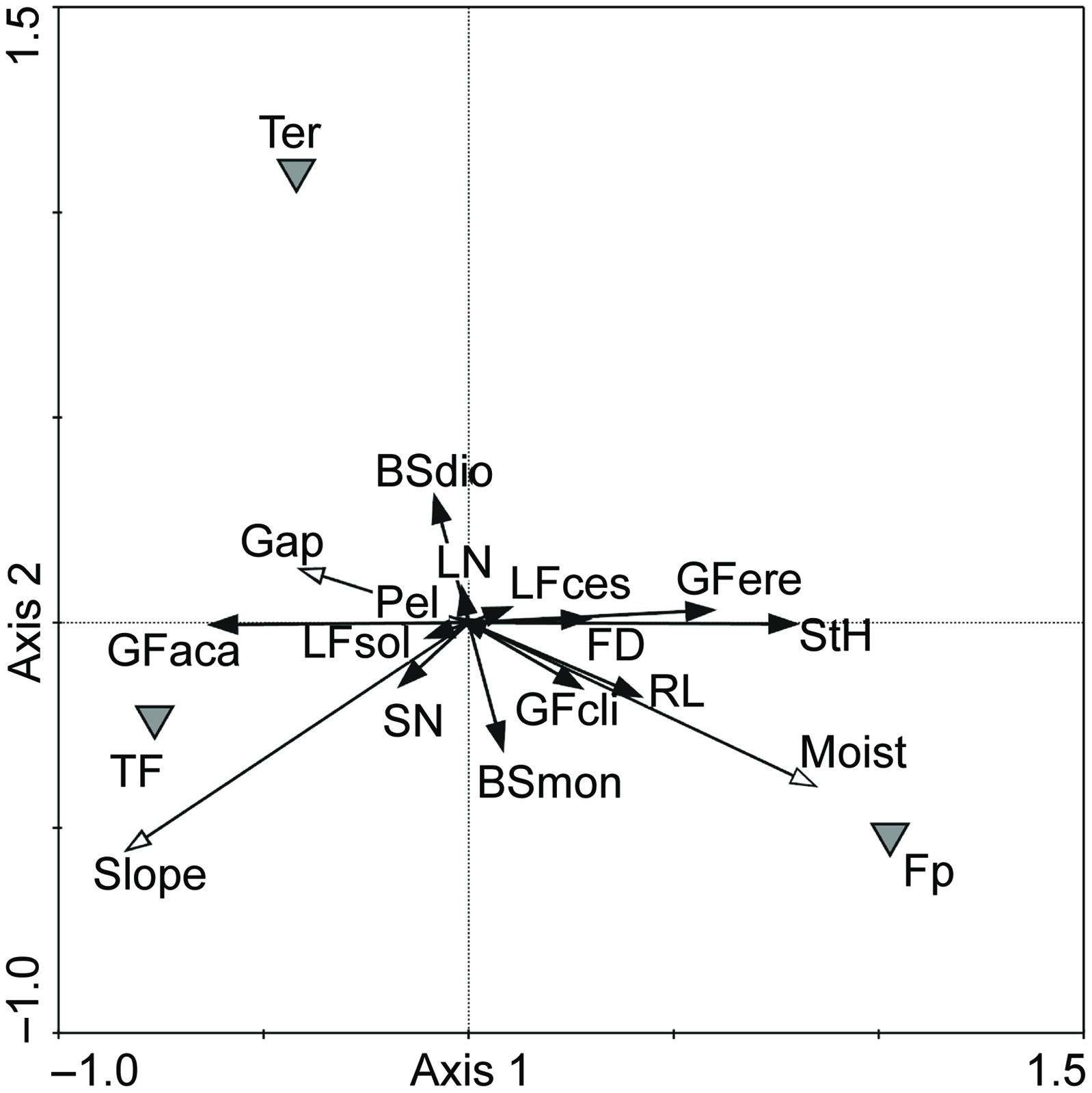
Figure 3. Ordination obtained by redundancy analysis (RDA) of the functional trait composition (CWM) in the palm community along the Guaviare River in the Colombian Amazon as a function of environmental variables. The white-tipped arrows represent environmental variables: Gap=presence of tree-fall gaps, Slope=inclination, Moist=soil moisture. The gray triangles are the forest types: TF=terra firme forests, Fp=floodplain forests and Ter=terrace forests. The black-tipped arrows represent traits: LF=lifeform (cespitose=ces, solitary=sol), GF=growth form (acaulescent=aca, erect=ere, climbing=cli), StH= maximum stem height, LN= maximum leaf number, RL= maximum leaf rachis length, PeL= maximum petiole length, FD=maximum fruit diameter, SN=seed number, BS=breeding system (dioecious=dio, monoecious=mon).
StH and FD were clearly associated with positive values of axis 1, while the number of seeds per fruit (SN) and the acaulescent growth form (GFaca) were related to negative values in the CWM-RDA. In general, the species with the relatively long petioles are present in greater numbers in sites with high numbers of forest gaps, while tall, large-leaved and climbing species occur mainly in sites with high moisture (Figure 3).
The RLQ analysis showed that species traits, environmental factors, and abundance were correlated (P <0.001). Most of the variation was captured by the first axis of the RLQ (81%, Supplement 3). In the FP transects, the maximum height of the stem (StH) and the soil moisture were associated with positive values of this axis. Species of the Socratea, Oenocarpus, and Euterpe genera (all tall palms) were associated with axis 1, whereas Attalea and Manicaria species were associated with negative values of axis 2. Likewise, tree-fall gaps and terrace forests were associated with the positive values of axis 2, where mainly species of the genera Geonoma and Bactris were abundant (medium to low number of palms) (Supplement 3).
Individual trait-environment relationships
The acaulescent growth form was positively correlated with slope and terra firme forests and negatively correlated with moisture and FPs. The StH was positively correlated with soil moisture and FPs and negatively correlated with slope and terra firme forests. We found functional dominance of tall palms in FPs with high soil moisture and functional dominance of small palms in terra firme and sloping forests (Figure 4).
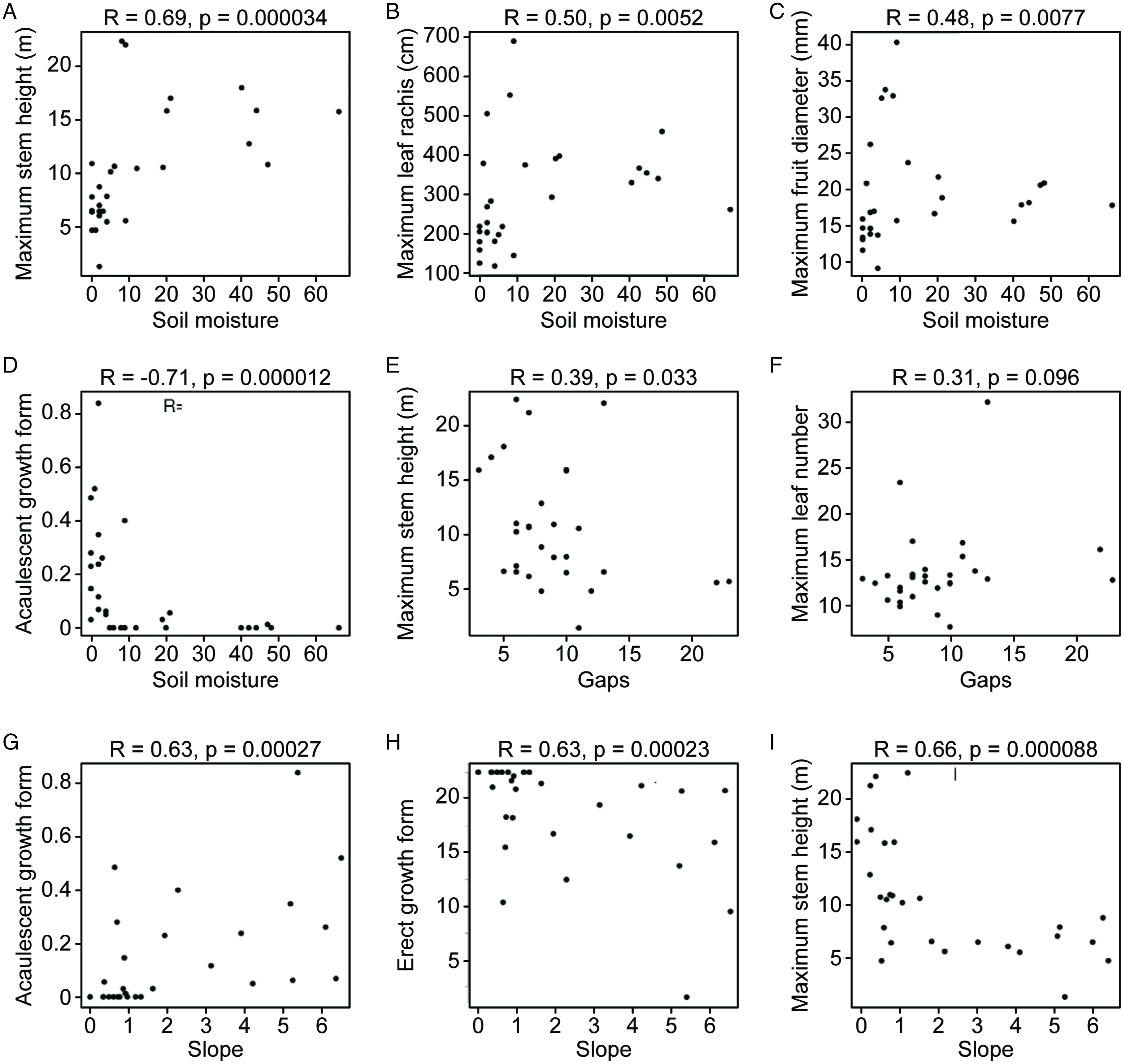
Figure 4. Scatter plots of palm functional traits and environmental variables that showed significant Spearman correlations in a palm community in the Amazon rainforest of Colombia, as studied in 29 transects of 500×5 m.
Leaf size was positively correlated with soil moisture and FPs and negatively correlated with terrace forests. The FD was strongly and positively correlated with FPs and negatively correlated with terra firme forests (Table 2). The seed number was not strongly correlated with any environmental variable measured.
Table 2. Spearman’s rank correlations between environmental variables and functional composition (CWM) of a palm community in the Colombian Amazon.
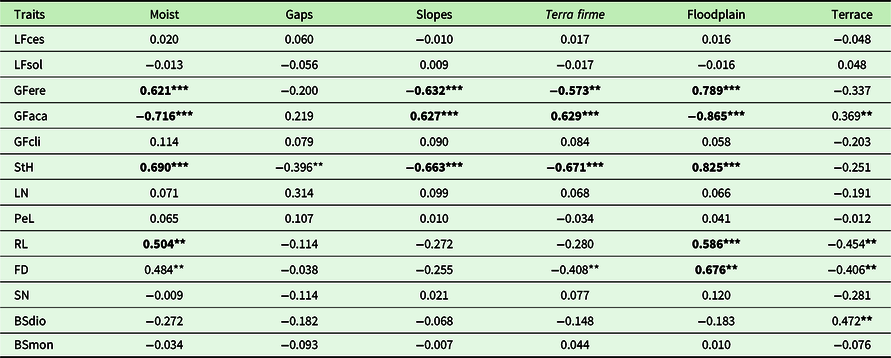
N=29 transects. ***, P< 0.001; **, P< 0.05. Environmental variables: moist=soil moisture, gaps=presence of tree-fall gaps, slope=inclination; traits: LF=lifeform (cespitose=ces, solitary=sol), GF=growth form (acaulescent=aca, erect=ere, climbing=cli), StH= maximum stem height, LN= maximum leaf number, PeL= maximum petiole length, RL= maximum leaf rachis length, FD=maximum fruit diameter, SN=seed number, BS=breeding system (dioecious=dio, monoecious=mon).
Phylogenetic patterns
All traits except fruit diameter and acaulescent growth form showed phylogenetic signal significantly larger than random in a significant number of the phylogenies sampled from the posterior distribution of Faurby et al. (Reference Faurby, Eiserhardt, Baker and Svenning2016) (Supplement 4). Cespitose life form, climbing habit, seed number, and leaf dimensions (rachis and PeL) showed particularly strong phylogenetic signal. However, when testing for phylogenetic patterns in the distribution of traits across communities, only cespitose habit and – to a much lesser degree – PeL deviated from a phylogenetically random distribution (Supplement 4).
Discussion
Relationship between functional traits and environmental variables
Our results are in agreement with the plant ecology strategy scheme, which states that leaf morphology, stem height, and seed size (LHS) capture the main axes of plant responses to competition, stress, and disturbance (Westoby Reference Westoby1998). Tall and large-leaved palms were associated with moist and flooded areas, and small and acaulescent palms were associated with terra firme forests and sloping areas (Figure 3). This suggests that hydrology is the main environmental filter of functional composition, as has been documented in other studies of palm functional traits in the Amazonia region (Kristiansen et al. Reference Kristiansen, Svenning, Grández, Salo and Balslev2009, Pereira De Souza Reference Pereira De Souza2019). Hydrology segregates tall canopy palms with a competitive and avoidance strategy (Voesenek et al. Reference Voesenek, Rijnders, Peeters, Van de Steeg and De Kroon2004) from small understory palms, which are mainly stress tolerant (Grime Reference Grime1974).
We found that slope was strongly correlated with acaulescent growth (Table 2). Previous studies of palms have shown that the effects of topography on the distribution and abundance of palms depend on species and varies between sites (Svenning Reference Svenning1999). It was suggested that topography has no direct influence on species but that slope is correlated with other environmental variables such as drainage, which does have an effect (Vormisto et al. Reference Vormisto, Tuomisto and Oksanen2004). This is the most plausible explanation of our results.
The strong correlation found between StH and flooded and moist environments (FP and moist) (Figure 4) suggests that being a tall palm is a functional response to prevent leaves and leaf buds from becoming submerged, making survival and growth in flooded zones possible. Therefore, it is an evasion strategy to avoid anoxic effects caused by water (Garssen et al. Reference Garssen, Baattrup-Pedersen, Voesenek, Verhoeven and Soons2015, Gross et al. Reference Gross, Robson, Lavorel, Albert, Le Bagousse-Pinguet and Guillemin2008, Mommer et al. Reference Mommer, Lenssen, Huber, Visser and De Kroon2006, Voesenek et al. Reference Voesenek, Rijnders, Peeters, Van de Steeg and De Kroon2004). Other studies have demonstrated strong dominance of tall palms in FPs and a low representation of medium and small palms in these environments (Balslev et al. Reference Balslev, Kahn, Millan, Svenning, Kristiansen, Borchsenius, Pedersen and Eiserhardt2011, Kahn & De Castro Reference Kahn and De Castro1985). On a local scale in Peru, the composition of species is determined by moisture at the soil surface (Normand et al. Reference Normand, Vormisto, Svenning, Grandez and Balslev2006); the results of this work show that these variables also determine the functional composition (Figure 4).
A proportional increase in petiole length with respect to leaf size reduces the overlap between leaves of adjacent Geonoma cuneata and Asterogyne martiana palms (Figure 3) (Chazdon Reference Chazdon1985, Reference Chazdon1986). Therefore, we interpret long petioles in the presence of gaps as the ability of the palms to compete for light, since the length of the petiole increases the diameter of the crown, allowing the palm to optimize the uptake of resources and grow rapidly. In this sense, it has been proposed that canopy palms can reach their adult state only in the presence of gaps because their light requirements increase with ontogeny (Kahn Reference Kahn1986, Salm et al. Reference Salm, Jalles-Filho and Schuck-Paim2005), adapting to the opportunity for growth between disturbances through an acquisitive strategy (Reich et al. Reference Reich, Wright, Cavender-Bares, Craine, Oleksyn, Westoby and Walters2003). However, additional studies are needed to corroborate this relationship given the low statistical support we found (Table 1), and the finding that the distribution of petiole length across communities is phylogenetically structured (Supplement 4), indicating that the observed patterns may be driven by phylogenetic co-variation with other traits.
Long-leaved palm species are well represented in flooded habitats. This trait might represent an adaptation to floodplains to capture more light, as the canopy is more open in flooded environments (Kahn & De Castro Reference Kahn and De Castro1985). However, the abundance of palms with long leaves may be due to the abundance of members of the genus Astrocaryum in FPs, and the correlation could be driven by a phylogenetic artifact (Kahn & De Castro Reference Kahn and De Castro1985). On the other hand, leaf size may have an allometric correlation with plant size. Knowing the possible constraining effects of allometry, ontogeny, and phylogeny on leaf size may help us interpret the adaptive significance of this trait (Cornelissen Reference Cornelissen1999).
We found a clear relationship between FD and moist and flooded environments (Table 2). Generally, fruit diameter is related to the capability to colonize new habitats and to recruit in resource-limiting environments (Salgado-Negret & Paz Reference Salgado-Negret, Paz and Salgado-Negret2016). Likewise, it has been documented that seed and fruit size determine the success of the establishment of plants because a relatively large seed mass increases the ability of seedlings to survive drought (Baskin & Baskin Reference Baskin and Baskin2014). In particular, some studies in varzea and igapo forests in the central region of the Brazilian Amazonia have shown that large seeds are adapted to areas with high levels of flooding by promoting rapid seedling establishment (Parolin et al. Reference Parolin, Ferreira and Junk2003). Seed size can affect germination by other means, but evidence that corroborates further relationships between large fruits and FPs is currently limited.
Conclusion
Soil moisture and flood regimes are the main environmental factors that affect the functional trait composition of this Amazonian rainforest palm community. Avoidance strategies allow palms to keep their aerial parts above the water level, preventing anoxic stress under waterlogged conditions. Acaulescence and small palm size are stress tolerance mechanisms on sloping and dry terrain (terra firme) under shady conditions, and palms with these traits do not tolerate flooded areas. Hydrology is one of the main determinants of the functional trait composition of neotropical palms on a local scale, and habitats with prolonged floods have communities of palms in which species are adapted to the stressful ecophysiological conditions that flooding creates.
Acknowledgements
WT thanks the program ‘Socios para la Conservación de la Amazonia Colombiana’ for partially financing his Master at Pontificia Universidad Javeriana. Rodrigo Bernal is thanked for help in organizing the fieldwork and identifying the palms.
Financial support
This work was supported by the Independent Research Fund Denmark (HB grant number 9040-00136B). WLE considers this work a contribution to his VILLUM Young Investigator project ‘Explaining the hyperdiversity of Tropical rainforests using the Tree of Life (TropiToL)’ funded by VILLUM FONDEN (grant 00025354).
Conflict of interest
None
Ethical approval
None
Supplementary material
To view supplementary material for this article, please visit https://doi.org/10.1017/S0266467421000493