Introduction
We are often faced with demanding cognitive and physical tasks. Completing effortful tasks can leave us feeling drained and exhausted, leading to a dampened mood (Broderick, Reference Broderick2005; Erber & Erber, Reference Erber and Erber1994; Erber & Tesser, Reference Erber and Tesser1992; Kron, Schul, Cohen, & Hassin, Reference Kron, Schul, Cohen and Hassin2010). Imagine that you need to work through a pile of papers by the end of the day. Despite your positive mood in the morning, you will probably feel exhausted afterwards and it might take long to return to the positive feeling you had before. Accordingly, we experience a wide range of mood states that encompasses a spectrum from positive to negative moods every day (Clark & Watson, Reference Clark and Watson1988; Thayer, Reference Thayer1997). In contrast to temporary alterations in mood, persistent negative mood that is present in affective disorders incurs a debilitating individual burden (Ferrari et al., Reference Ferrari, Charlson, Norman, Patten, Freedman, Murray and Whiteford2013) and high socio-economic costs (König, König, & Konnopka, Reference König, König and Konnopka2019). Thus, it is crucial to identify potential causes of mood alterations and to investigate ways to alleviate pathologically relevant mood disturbances.
As illustrated by going to work and completing assignments, one factor that affects our moods is cognitive and physical tasks that require effort to complete. On the one hand, exerting cognitive effort after positive and negative mood induction leads to a dampening of the induced mood (Broderick, Reference Broderick2005; Erber & Erber, Reference Erber and Erber1994; Erber & Tesser, Reference Erber and Tesser1992; Kron et al., Reference Kron, Schul, Cohen and Hassin2010). Additional mood regulation processes have been observed to mitigate induced negative mood states with a concurrent increase in a positive mood (Erber & Erber, Reference Erber and Erber2000; Forkosh & Drake, Reference Forkosh and Drake2017; Kim & Kanfer, Reference Kim and Kanfer2009; Sirois, Reference Sirois2014). On the other hand, exerting physical effort may increase or decrease mood depending on how much effort was exerted. Higher training intensities can lead to an increase in negative mood immediately following training (Morgan, Brown, Raglin, O'Connor, & Ellickson, Reference Morgan, Brown, Raglin, O'Connor and Ellickson1987; Oliveira, Slama, Deslandes, Furtado, & Santos, Reference Oliveira, Slama, Deslandes, Furtado and Santos2013; Selmi et al., Reference Selmi, Haddad, Majed, Khalifa, Hamza and Chamari2018), in part due to unpleasant interoceptive cues for high exercise intensity (Ekkekakis, Reference Ekkekakis2009). Nevertheless, effort exertion may lead to long-term improvements in mood and symptoms of depression, demonstrating the relevance of post-acute recovery (Brosse, Sheets, Lett, & Blumenthal, Reference Brosse, Sheets, Lett and Blumenthal2002; Schuch et al., Reference Schuch, Vancampfort, Richards, Rosenbaum, Ward and Stubbs2016; Stathopoulou, Powers, Berry, Smits, & Otto, Reference Stathopoulou, Powers, Berry, Smits and Otto2006). Taken together, these findings point to task exertion as a core modulator of affect, potentially leading to aberrant mood states if the mood does not recover quickly after exertion.
Since prolonged negative mood is a key symptom of major depression and related mental disorders [(Diagnostic & statistical manual of mental disorders: DSM-5, 2013) and ICD-10 (World Health Organization, 2004)], treatments of affective disorders often target improvements in mood (Sin & Lyubomirsky, Reference Sin and Lyubomirsky2009). Current treatment for depression is heterogeneous and can include medication, behavioral therapies, or brain stimulation techniques such as deep brain stimulation (Delaloye & Holtzheimer, Reference Delaloye and Holtzheimer2014) or vagus nerve stimulation (VNS) (Cimpianu, Strube, Falkai, Palm, & Hasan, Reference Cimpianu, Strube, Falkai, Palm and Hasan2017; Howland, Reference Howland2014). When VNS is applied invasively, the device is usually implanted in the chest wall with wires stimulating the vagus nerve at the neck (George et al., Reference George, Nahas, Molloy, Speer, Oliver, Li and Ballenger2000). Previous studies have reported improvements in mood states and depressive symptoms after VNS (Elger, Hoppe, Falkai, Rush, & Elger, Reference Elger, Hoppe, Falkai, Rush and Elger2000; George et al., Reference George, Rush, Marangell, Sackeim, Brannan, Davis and Goodnick2005; Reference George, Ward, Ninan, Pollack, Nahas, Anderson and Ballenger2008; Rush et al., Reference Rush, Marangell, Sackeim, George, Brannan, Davis and Cooke2005; Schlaepfer et al., Reference Schlaepfer, Frick, Zobel, Maier, Heuser, Bajbouj and Hasdemir2008) which increased with the duration of use (Berry et al., Reference Berry, Broglio, Bunker, Jayewardene, Olin and Rush2013). Given the invasive nature of VNS, a non-invasive alternative is needed as it would greatly expand its potential use as an adjunct treatment (Ventureyra, Reference Ventureyra2000). Indeed, transcutaneous auricular VNS (taVNS) was developed as a non-invasive variant of VNS where the vagus nerve is stimulated through the skin of the auricle (Fallgatter et al., Reference Fallgatter, Neuhauser, Herrmann, Ehlis, Wagener, Scheuerpflug and Riederer2003). Preliminary studies have shown that non-invasive taVNS improves emotion recognition (Koenig et al., Reference Koenig, Parzer, Haigis, Liebemann, Jung, Resch and Kaess2019), mood (Kraus et al., Reference Kraus, Hösl, Kiess, Schanze, Kornhuber and Forster2007) and depressive symptoms (Liu et al., Reference Liu, Fang, Wang, Rong, Hong, Fan and Kong2016; Tu et al., Reference Tu, Fang, Cao, Wang, Park, Jorgenson and Kong2018; Wu et al., Reference Wu, Liu, Fu, Chen, Cui, Lu and Tang2018) similar to invasive VNS. Such improvements could be due to alterations in brain activity in the prefrontal cortex and the limbic system after invasive (Nahas et al., Reference Nahas, Teneback, Chae, Mu, Molnar, Kozel and George2007; Pardo et al., Reference Pardo, Sheikh, Schwindt, Lee, Kuskowski, Surerus and Rittberg2008) and non-invasive VNS (Badran et al., Reference Badran, Dowdle, Mithoefer, LaBate, Coatsworth, Brown and George2018; Yakunina, Kim, & Nam, Reference Yakunina, Kim and Nam2017). Since these mesocorticolimbic structures are characteristically dysregulated in depression (Anand et al., Reference Anand, Li, Wang, Wu, Gao, Bukhari and Lowe2005; Groenewold, Opmeer, de Jonge, Aleman, & Costafreda, Reference Groenewold, Opmeer, de Jonge, Aleman and Costafreda2013; Iseger, van Bueren, Kenemans, Gevirtz, & Arns, Reference Iseger, van Bueren, Kenemans, Gevirtz and Arns2020) and critically involved in cost-benefit decision-making (Husain & Roiser, Reference Husain and Roiser2018; Kroemer et al., Reference Kroemer, Guevara, Ciocanea Teodorescu, Wuttig, Kobiella and Smolka2014; Reference Kroemer, Burrasch, Hellrung, Studer and Knecht2016), taVNS may provide a means to improve mood, particularly after effort exertion.
Furthermore, little research has been done on the lateralization effects of taVNS in humans. While animal studies show that VNS-induced effects on reward and dopamine release may be lateralized (Han et al., Reference Han, Tellez, Perkins, Perez, Qu, Ferreira and de Araujo2018), most studies conducted in humans use only left-sided stimulation due to possible effects of right-sided stimulation on the cardiac cycle. However, recent studies suggest that there are no detrimental effects of right auricle stimulation in humans (De Couck et al., Reference De Couck, Cserjesi, Caers, Zijlstra, Widjaja, Wolf and Gidron2017; Redgrave et al., Reference Redgrave, Day, Leung, Laud, Ali, Lindert and Majid2018). Therefore, investigating the lateralization effects of taVNS in humans would be of high therapeutic value. Despite emerging evidence for beneficial effects of taVNS on mood especially when used chronically (Hein et al., Reference Hein, Nowak, Kiess, Biermann, Bayerlein, Kornhuber and Kraus2013; Rong et al., Reference Rong, Liu, Wang, Liu, Fang, Zhao and Kong2016), studies on the acute effects of taVNS on mood remain surprisingly scarce (Kraus et al., Reference Kraus, Hösl, Kiess, Schanze, Kornhuber and Forster2007). Moreover, none of the studies includes a challenge that temporarily reduces mood. To close this gap, we investigated the effects of acute left- and right-sided taVNS v. sham on mood after the exertion of effort and after a mood recovery period. To measure mood states, we used the Positive and Negative Affect Schedule (Watson, Clark, & Tellegen, Reference Watson, Clark and Tellegen1988) before, during, and after stimulation. Based on previous results, we hypothesized that taVNS enhances positive mood and attenuates negative mood compared to sham stimulation.
Methods
Participants
Eighty-five volunteers participated in the study. Participants were recruited via internal university mailing lists, flyers, and advertisement on websites. To be included in the analysis, each participant had to complete two sessions: one after stimulation of the cymba conchae and one after a sham stimulation at the earlobe. Thus, we had to exclude three participants who did not finish the second experimental session, leading to a sample size of N = 82 with complete mood ratings. As reported before (Neuser et al., Reference Neuser, Teckentrup, Kühnel, Hallschmid, Walter and Kroemer2020), one additional participant had to be excluded from the correlation analysis involving taVNS-induced changes in motivation because of an incorrectly assigned maximum of the button press frequency for the effort task precluding comparisons of the two sessions. Out of the 82 participants, 42 completed the study during left-sided taVNS, whereas 40 completed it during right-sided taVNS. All participants went through a telephone screening to ensure that they were healthy, spoke German, and were right-handed (47 women; M Age = 24.6 years ± 3.5; M BMI = 23.1 kg/m2 ± 3.01; 17.9–30.9). The study was approved by the local ethics committee and was conducted in accordance with the ethical code of the World Medical Association (Declaration of Helsinki). Participants provided written informed consent at the beginning of Session 1 and received either monetary compensation (32€ fixed amount) or course credit for their participation after completing the second session. Moreover, they received money and breakfast depending on their performance during the tasks.
Experimental procedure
Primary analyses of the Effort Allocation Task focusing on motivational effects of taVNS have first been reported in Neuser et al. (Reference Neuser, Teckentrup, Kühnel, Hallschmid, Walter and Kroemer2020). In the present work, we report results on the effects of taVNS on mood which was a secondary outcome of the study.
Experimental sessions were conducted in a randomized, single-blind crossover (taVNS/sham) design (Fig. 1). Participants received either left- or right-sided taVNS and sham stimulation in their two experimental sessions. Assignment to left-sided v. right-sided stimulation was not randomized since we first collected data with stimulation on the left side. Participants were required to fast overnight. Each session began in the morning and lasted about 2.5 h. After measuring physiological parameters such as pulse and weight as well as assessing information about last food intake, the participants were instructed that they would be collecting energy and money points throughout the session depending on their performance in the following tasks. Earned energy points would be converted into participants' breakfast consisting of cereal and milk, scaled according to the number of points they won. Water was provided ad libitum during the experiment.
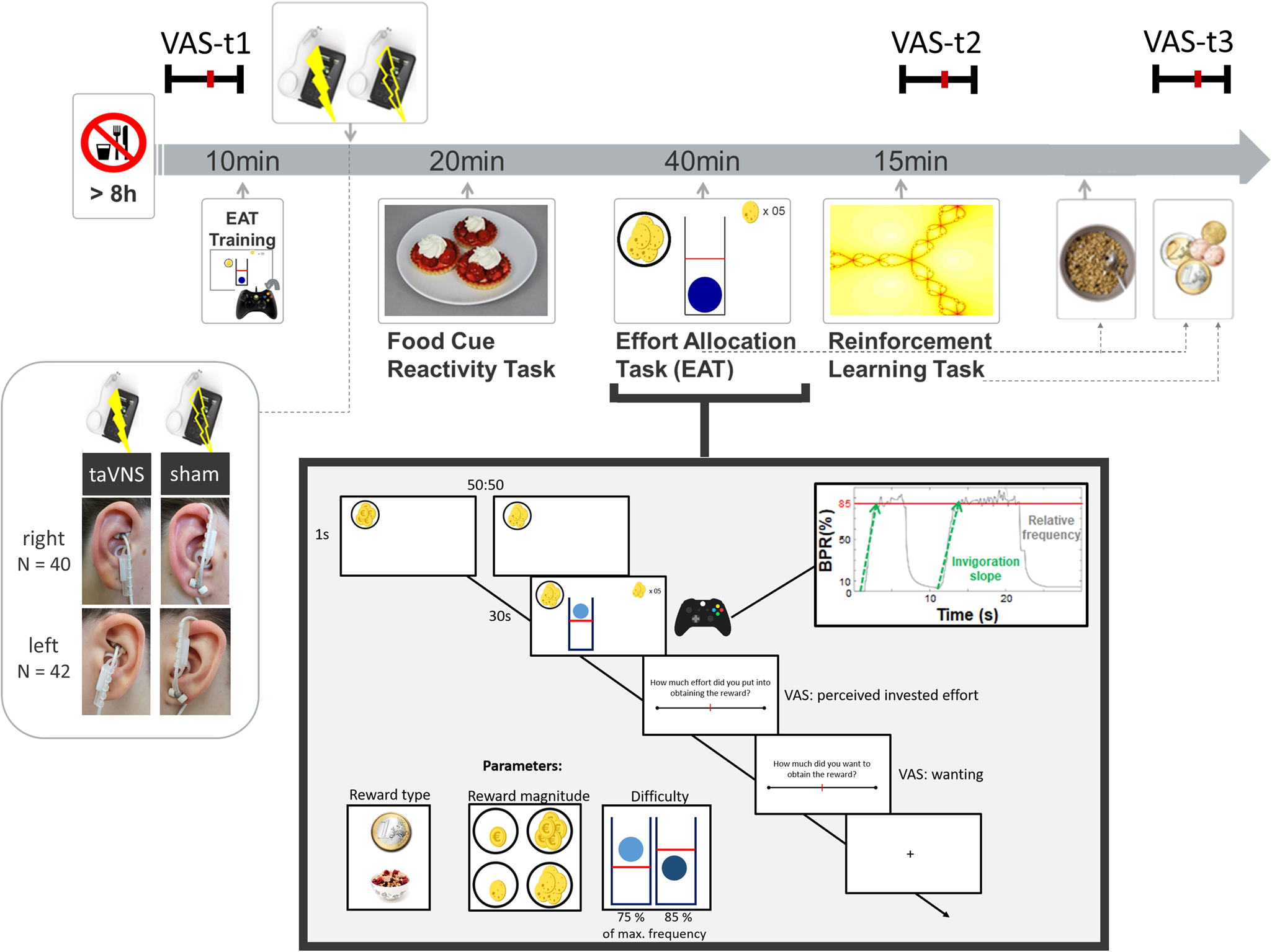
Fig. 1. Experimental procedure and schematic summary of the task block. The timeline shows the experimental procedure with stimulation beginning before a block of three tasks. State measurements were taken before stimulation, during stimulation as well as after the tasks, and after stimulation using visual analog scales (VAS). The food cue reactivity task required quick ratings of wanting and liking. The effort allocation task required physical effort to obtain rewards by pressing a button vigorously. The reinforcement learning task required cognitive effort to track which actions following a cue maximize reward and minimize punishments.
Next, participants responded to state questions based on the PANAS (Watson et al., Reference Watson, Clark and Tellegen1988) using visual analog scales (VAS). A practice session of the effort task followed where the maximum frequency of the button press was recorded to adjust the task to each participant's individual ability.
Afterwards, the taVNS electrode was placed on the ear. The first group of participants received stimulation on the left ear (N = 42), the second group on the right ear (N = 40). Strips of surgical tape secured the electrode and the cable in place. For every session and stimulation condition, the stimulation strength was individually assessed using VAS ratings of pain to match the subjective experience. Stimulation was initiated at an amplitude of 0.1 mA and increased by the experimenter by 0.1–0.2 mA at a time. Participants rated the sensation after every increment until ratings corresponded to a ‘mild pricking’. The stimulation remained active at this level.
Participants then completed a food-cue reactivity task (~20 min) as well as the effort task (~40 min), which tested the willingness to expend physical effort in return for rewards. Since the default stimulation protocol of the NEMOS taVNS device alternates between 30 s of on and off phases, the experimenter manually switched on the stimulation phases of the device at the onset of the effort phases of the task. Thereafter, participants' pulse was measured again. Moreover, participants completed a reinforcement learning task (Kühnel et al., Reference Kühnel, Teckentrup, Neuser, Huys, Burrasch, Walter and Kroemer2020), which required cognitive effort (~15 min).
After completing the task block, participants entered state VAS ratings comprising the PANAS items for the second time. Then, participants had the taVNS electrode removed and received their breakfast and a snack according to the energy points earned in the effort allocation task. They were instructed that this was their food reward and that they could eat as much as they liked. Next, participants completed state VAS ratings comprising the PANAS items for the third time. At the end of the session, participants received their monetary reward as earned in the Effort Allocation Task and the Reinforcement Learning Task. The second visit followed the same standardized protocol and was conducted at approximately the same time within a week, usually within 3–4 days.
Mood ratings
For assessing participants' mood, we used the PANAS items (Watson et al., Reference Watson, Clark and Tellegen1988) presented with VAS on a scale of 0 (lowest rating) to 100 (highest rating; cf. Kobiella et al. Reference Kobiella, Ulshofer, Vollmert, Vollstadt-Klein, Buhler, Esslinger and Smolka2011; Renner, Schwarz, Peters, & Huibers, Reference Renner, Schwarz, Peters and Huibers2014). Although the PANAS originally uses a five-point Likert-type scale, using VAS has been shown to prevent memory-dependent evaluation effects when presenting the scale repeatedly over time (Renner et al., Reference Renner, Schwarz, Peters and Huibers2014). Since we wanted to assess taVNS-induced changes relative to baseline mood ratings, we used VAS to better capture changes within each session. Presenting positive affect can be described as a state of high energy and enthusiasm, whereas negative affect comprises different aversive feelings such as anger, guilt, or nervousness. Participants responded to state questions of the PANAS at three time points: before starting the tasks and stimulation (baseline VAS), after completing the task block during the stimulation (stimulation VAS), and finally about 20 min after completing the tasks and the stimulation period and after receiving the earned rewards including breakfast (post-stimulation VAS). The questionnaire was presented on a computer screen as VAS using the joystick on an Xbox 360 controller (Microsoft Corporation, Redmond, WA).
Effort allocation task
In the effort allocation task, participants had to collect food and money tokens throughout the task by exerting effort (i.e. repeatedly pressing the right trigger button with the right index finger). The task was adapted from Meyniel, Sergent, Rigoux, Daunizeau, & Pessiglione (Reference Meyniel, Sergent, Rigoux, Daunizeau and Pessiglione2013) and used frequency of button presses instead of grip force to measure physical effort (Neuser et al., Reference Neuser, Teckentrup, Kühnel, Hallschmid, Walter and Kroemer2020). Trials varied in the type of reward, which was always indicated by money or food symbols on the screen. Moreover, we varied the magnitude of the prospective reward (low v. high) as well as the difficulty (easy v. hard) to obtain the rewards. After every effort phase of a trial, participants were presented sequentially with two VAS questions inquiring about the exertion for and the wanting of the reward at stake.
taVNS device
To stimulate the auricular branch of the vagus nerve, we used the NEMOS® stimulation device (cerbomed GmbH, Erlangen, Germany). These devices have been previously used in clinical trials (Bauer et al., Reference Bauer, Baier, Baumgartner, Bohlmann, Fauser, Graf and Hamer2016; Kreuzer et al., Reference Kreuzer, Landgrebe, Husser, Resch, Schecklmann, Geisreiter and Langguth2012) and proof-of-principle neuroimaging studies (Frangos, Ellrich, & Komisaruk, Reference Frangos, Ellrich and Komisaruk2015). The stimulation protocol for the NEMOS is preset to a biphasic impulse frequency of 25 Hz with a stimulation duration of 30 s, followed by a 30 s off phase. The electrical current is transmitted by a titanium electrode placed at the cymba conchae (taVNS) or earlobe (sham) of the ear (Frangos et al., Reference Frangos, Ellrich and Komisaruk2015). To match the subjective experience of the stimulation, the intensity was determined for each participant and each condition individually to correspond to mild pricking (Kühnel et al., Reference Kühnel, Teckentrup, Neuser, Huys, Burrasch, Walter and Kroemer2020; Neuser et al., Reference Neuser, Teckentrup, Kühnel, Hallschmid, Walter and Kroemer2020; Teckentrup et al., Reference Teckentrup, Neubert, Santiago, Hallschmid, Walter and Kroemer2020). In contrast to sham conditions that do not apply any stimulation or pause after an initial stimulation that is used for other brain stimulation techniques, this electrode placement ensures comparable sensory effects and effective blinding. Therefore, it is currently considered as ‘best practice’ for sham control of taVNS (Farmer et al., Reference Farmer, Strzelczyk, Finisguerra, Gourine, Gharabaghi, Hasan and Koenig2020; Frangos et al., Reference Frangos, Ellrich and Komisaruk2015; Frangos & Komisaruk, Reference Frangos and Komisaruk2017; Kraus et al., Reference Kraus, Hösl, Kiess, Schanze, Kornhuber and Forster2007, Reference Kraus, Kiess, Hösl, Terekhin, Kornhuber and Forster2013; Yakunina et al., Reference Yakunina, Kim and Nam2017).
Data analysis
Statistical modeling of mood ratings
Although VAS scales are commonly used to measure mood, there are several potential pitfalls that have to be addressed in the analysis (Heller, Manuguerra, & Chow, Reference Heller, Manuguerra and Chow2016; Vautier, Reference Vautier2011). For example, change scores after an intervention are often strongly correlated with baseline levels and this statistical dependence must be modeled accordingly (Vickers & Altman, Reference Vickers and Altman2001). Therefore, we used linear mixed-effects as well as hierarchical Bayesian analyses to analyze taVNS-induced changes in mood based on all collected ratings. Compared to ANOVAs, linear mixed-effects analyses are advantageous because they enable the estimation of ‘fixed’ effects of the stimulation and other design elements (‘immutable’ differences that are shared across the group), ‘random’ effects (persistent differences of individuals from a group average), and noise (non-reproducible differences due to measurement error). A repeated-measures ANOVA can be regarded as a simple and restricted mixed-effects model (Raudenbush & Bryk, Reference Raudenbush and Bryk2002). Compared to mixed-effects analyses, hierarchical Bayesian models are advantageous because they provide several crucial extensions (Gelman & Hill, Reference Gelman and Hill2006). First, it is possible to use informed priors to improve the estimation and precision of a model (Bürkner, Reference Bürkner2017; Gelman & Hill, Reference Gelman and Hill2006). For example, a useful constraint is to restrict the range of the VAS ratings from 0 to 100. Second, it is possible to sample the posterior distribution of a parameter (Bürkner, Reference Bürkner2017). This posterior distribution represents the updated beliefs about the parameters after having seen the data in terms of a probability distribution (Gelman & Hill, Reference Gelman and Hill2006). In contrast, linear mixed-effects analyses estimate differences in parameters compared to null hypotheses based on distributional statistics of the data (Raudenbush & Bryk, Reference Raudenbush and Bryk2002). Third, by sampling the posterior distribution, we can estimate the likelihood of the alternative hypothesis, including the likelihood of the desired effect size such as taVNS-induced changes in mood, instead of estimating the probability of observing a change of a given magnitude if the null hypothesis were true.
Therefore, in addition to frequentist p values estimated by the linear mixed-effects model, we also estimated a corresponding hierarchical Bayesian model. Model 1 + predicted the outcome positive mood ratings of all individual items using the factors run (1: baseline VAS, 2: stimulation VAS, 3: post-stimulation VAS) and stimulation as well as their interaction. To account for inter-individual differences in taVNS-induced changes, the intercept and slopes were modeled as random effects. Model 1- predicted the outcome negative mood ratings of all individual items using the same set of predictors (for prior settings and R code used to fit the models, see SI).
Estimation of invigoration and maintenance of effort as a motivational index
For isolating invigoration and maintenance of effort as motivational indices, we segmented the behavioral data into work and rest segments as reported in a previous publication (Neuser et al., Reference Neuser, Teckentrup, Kühnel, Hallschmid, Walter and Kroemer2020). Invigoration of effort was computed by estimating the slope of the transition between the relative frequency of button presses during a rest segment and their initial plateau during the subsequent work segment based on the MATLAB findpeaks function. Maintenance of effort was computed as the average relative frequency of button press over a given trial, which is equivalent to the area under the curve. As previously reported (Neuser et al., Reference Neuser, Teckentrup, Kühnel, Hallschmid, Walter and Kroemer2020), taVNS effects were calculated using two univariate mixed-effects models. Briefly, either invigoration or maintenance of effort was predicted based on stimulation (taVNS, sham), reward type (food, money), reward magnitude (low, high), difficulty (easy, hard; all dummy coded), the interaction between reward magnitude and difficulty as well as interactions between stimulation and all other terms. At the participant level, stimulation order and stimulation side (mean-centered) were included. To account for deviations from fixed group effects, random slopes and intercepts were modeled for all predictors.
Statistical threshold and software
For our analyses, we used a two-tailed α ⩽ 0.05. Mixed-effects analyses were conducted with HLM v7 (effort invigoration and maintenance (Raudenbush, Bryk, Cheong, Congdon, & du Toit, Reference Raudenbush, Bryk, Cheong, Congdon and du Toit2011)) and lmerTest in R (positive and negative mood (Kuznetsova, Brockhoff, & Christensen, Reference Kuznetsova, Brockhoff and Christensen2017)). Hierarchical Bayesian analyses were conducted with brms in R (Bürkner, Reference Bürkner2017). To determine the evidence for the alternative hypothesis (i.e. taVNS improves mood) provided by our results, we calculated corresponding Bayes Factors (BF). For the correlation with taVNS-induced changes in motivation, we used a stretched beta prior = 0.7 (to reflect that large correlations are less likely a priori) as implemented in JASP v0.9 (JASP Team, 2019). We processed data with MATLAB vR2019a and SPSS v26 and plotted results with R v3.6.0 (R Core Team, 2019), including bayesplot (Gabry & Mahr, Reference Gabry and Mahr2019) and JASP.
Results
taVNS increases positive mood after the stimulation phase
To estimate taVNS-induced changes in mood, we first used a linear mixed-effects model predicting positive or negative mood as outcomes based on the factors run and stimulation as well as their interaction. To account for inter-individual differences in taVNS-induced changes, the intercept and slopes were modeled as random effects. As expected, the demanding tasks conducted during the stimulation phase led to a decrease in a positive mood (fixed effect of stimulation VAS, Run 2: b = −7.50, t = 4.81, p < 0.001) that had only partly recovered 20 min after the stimulation (fixed effect of post-stimulation VAS, Run 3: b = −3.79, t = 2.51, p = 0.014). However, there was no increase in negative mood during the stimulation phase (fixed effect of stimulation VAS, Run 2: b = 0.77, t = 0.79, p = 0.43) and it even improved slightly 20 min after the stimulation (fixed effect of post-stimulation VAS, Run 3: b = −1.93, t = 2.27, p = 0.026).
In line with the randomization of stimulation conditions, there was no main effect of taVNS on positive and negative mood at baseline (ps > 0.48). Crucially, we observed a significant taVNS-induced improvement of positive mood in the post-stimulation VAS (interaction Run 3 × Stimulation: b = 4.30, t = 2.44, p = 0.017, Fig. 2b), but not in the stimulation VAS (interaction Run 2 × Stimulation: b = 1.12, t = 0.63, p = 0.53). Likewise, we observed a taVNS-induced improvement of negative mood in the post-stimulation VAS, but this effect was not significant, likely due to the restricted range of negative mood ratings in healthy participants (interaction Run 3 × Stimulation: b = −1.25, t = 1.05, p = 0.30, Fig. 2a).
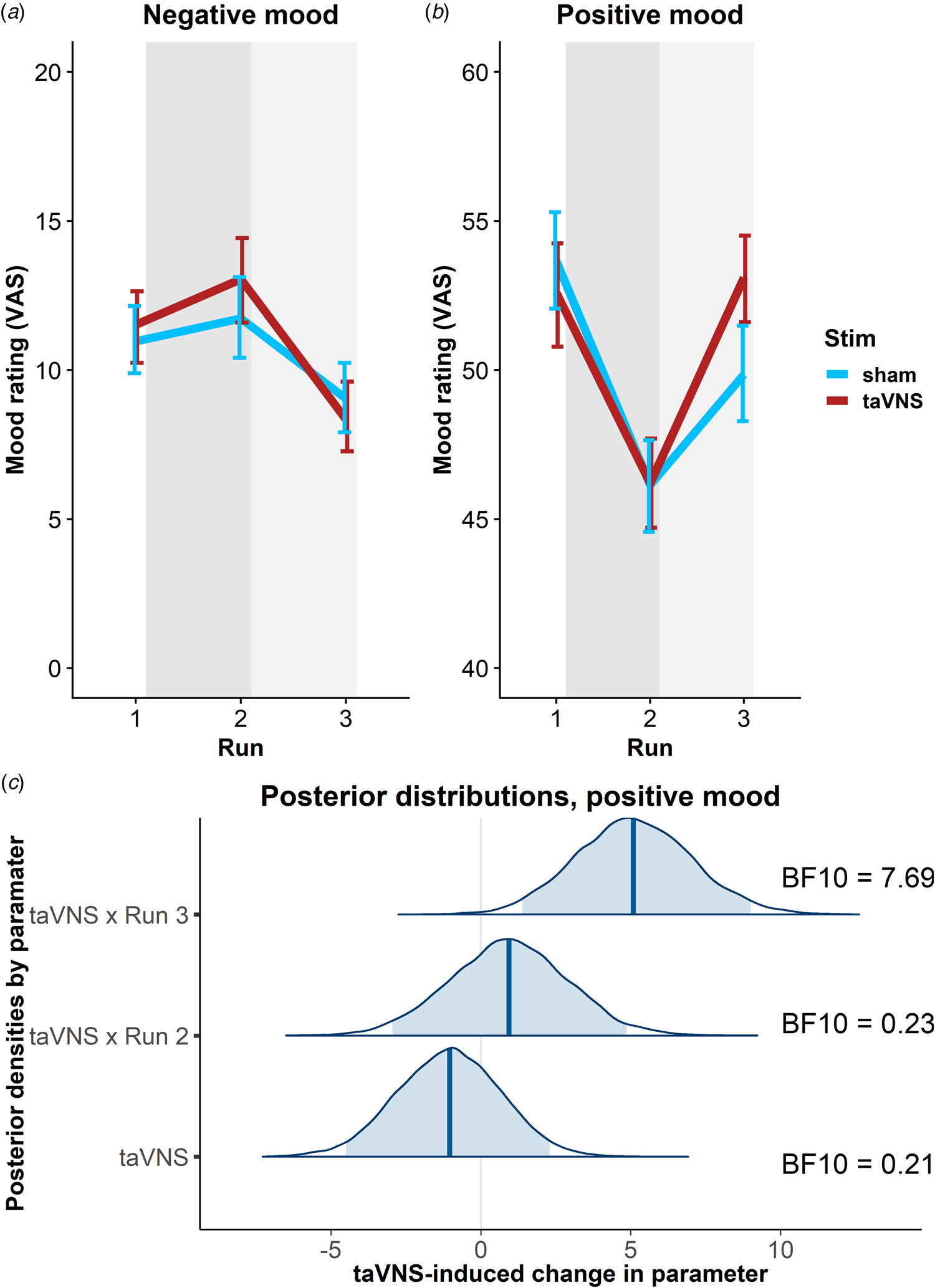
Fig. 2. Transcutaneous auricular vagus nerve stimulation (taVNS) increases positive mood in the post-stimulation visual analog scales (Run 3). (a) No significant changes in a negative mood. (b): Significant increase in positive mood 20 min post-stimulation (light gray shading), but not during stimulation (gray shading). Error bars denote 95% confidence intervals derived via bootstrapping. (c): Posterior distribution of the taVNS-induced change in a positive mood (95% credible interval in light blue, median in dark blue). BF10 = Bayes factor for the alternative hypothesis.
The mixed-effects results were corroborated by the hierarchical Bayesian analysis. Crucially, the Bayesian model provided a higher estimate of the taVNS-induced increase in a positive mood with a credible interval that clearly exceeded zero [interaction Run 3 × Stimulation: b = 5.11, e = 1.95, 95% CI (1.39–9.01), Fig. 2c]. Consequently, the BF provided strong support for a taVNS-induced increase in the post-stimulation VAS (BF10 = 7.69), while there was strong support for the null hypothesis at baseline (BF10 = 0.21) and in the stimulation VAS (BF10 = 0.23). Moreover, contrasting taVNS-induced changes in the stimulation VAS v. the post-stimulation VAS revealed a conclusive posterior probability, p(H1R3 > H1R2|D) = 0.99, that the effect was larger during mood recovery after the stimulation had ended. In contrast, due to the restricted range of negative mood ratings in many participants, the hierarchical Bayesian model failed to converge.
Baseline mood moderates taVNS-induced improvements in motivation
Since the exertion of effort during the task block had a strong effect on mood, we assessed whether taVNS-induced changes in mood and effort were associated. We have previously shown that effort exerted during the task can be dissociated into invigoration and maintenance of effort, and taVNS only increased invigoration (Neuser et al., Reference Neuser, Teckentrup, Kühnel, Hallschmid, Walter and Kroemer2020). Notably, lower baseline scores of positive mood were associated with stronger taVNS-induced increases in invigoration, r = −0.42, 95% CI (−0.57 to −0.21), BF10 = 249 (Fig. 3), but not maintenance of effort, r = −0.11, 95% CI (−0.31 to 0.11), BF10 = 0.27. However, taVNS-induced changes in mood in the stimulation VAS as well as the post-stimulation VAS were not significantly correlated with changes in invigoration or maintenance of effort (rs < 0.2, BF10 < 0.4). This suggests that initial mood states moderate subsequent improvements in motivation while taVNS-induced improvements in affect and motivation are only weakly associated.

Fig. 3. Lower positive mood at baseline is associated with greater improvements in invigoration induced by transcutaneous auricular vagus nerve stimulation. (a) Scatter plot of positive mood ratings at baseline and taVNS-induced changes in invigoration during the acute stimulation phase. (b) Evidence for the alternative and the null hypothesis given the observed association. BF10 = Bayes factor for the alternative hypothesis.
taVNS-induced improvements in mood are independent of stimulation side
To assess whether the reported results are robust across stimulation sides (left or right), sex, or order, we ran control analyses in the sample with complete data. Bayesian two-sample t tests showed unanimous support for the null hypothesis that there are no differences in mood or changes in mood due to the stimulation side (Fig. 4a), sex, or order (BF10 ⩽ 0.66, ps ⩾ 0.12). Moreover, controlling for these three variables increased the association between baseline mood scores and taVNS-induced invigoration of effort (β corrected = −0.49 v. β uncorrected = −0.42). These results suggest that the reported mood effects are independent of the stimulation side and robust across the potential confounds order and sex. Lastly, we explored whether taVNS-induced effects are evident across positive VAS items or driven by a more specific change. A visual inspection of the single-item residuals indicated only weak specificity suggesting that the changes in mood are best explained by a general change in a positive mood (Fig. 4b).

Fig. 4. Changes in positive mood ratings during the post-stimulation effects are invariant across stimulation sides and items. (a) Changes in positive mood relative to baseline are depicted for each participant (dots) and as group density. Changes are comparable for transcutaneous auricular vagus nerve stimulation (taVNS) applied to the left and the right side. (b) Changes in positive mood relative to baseline are largely invariant across items indicating a general effect of taVNS on mood.
Discussion
The vagus nerve plays a vital role in the regulation of affect by providing interoceptive feedback. Whereas effects of chronic VNS on mood have been often reported (Albert et al., Reference Albert, Maina, Aguglia, Vitalucci, Bogetto, Fronda and Lanotte2015; Elger et al., Reference Elger, Hoppe, Falkai, Rush and Elger2000; Schlaepfer et al., Reference Schlaepfer, Frick, Zobel, Maier, Heuser, Bajbouj and Hasdemir2008), effects of acute non-invasive taVNS on mood have been scarcely investigated, particularly after a mood challenge. Here, we used an effortful task block to perturb mood and concurrently applied taVNS for 90 min. Using linear mixed-effects and hierarchical Bayesian models, we found that taVNS facilitated mood recovery in the post-stimulation VAS, but not in the stimulation VAS directly after the task block. Furthermore, we showed that lower positive baseline mood correlated with taVNS-induced increases in the invigoration of effort (Neuser et al., Reference Neuser, Teckentrup, Kühnel, Hallschmid, Walter and Kroemer2020). Crucially, taVNS-induced effects on mood did not depend on the side of stimulation, sex of the participants, or order of the sessions. Collectively, our study provides strong evidence for post-acute effects of taVNS on mood recovery after an effort challenge as well as for a moderating role of affect on motivational effects elicited by acute taVNS during an effort challenge. We conclude that taVNS could be a promising tool to improve mood regulation in response to challenges which might indicate its potential as an adjunct therapy.
Non-invasive stimulation of the vagus nerve boosted positive mood in the post-stimulation VAS, approximately 20 min after stimulation and completion of effortful tasks. The facilitating effect on mood is in line with previously reported mood-enhancing effects of VNS (Howland, Reference Howland2014). However, we identified a crucial temporal dissociation in acute effects of taVNS that was previously unknown. While the positive mood was considerably reduced directly after the tasks (Broderick, Reference Broderick2005; Erber & Erber, Reference Erber and Erber1994; Erber & Tesser, Reference Erber and Tesser1992; Kron et al., Reference Kron, Schul, Cohen and Hassin2010) this drop in positive mood was not attenuated by taVNS. In contrast, we observed strong evidence for a faster mood recovery from an effort-induced reduction in positive affect after taVNS. A potential explanation that taVNS primarily facilitates mood recovery might be a reduced stress response induced by the tasks, as the vagus nerve has been consistently shown to play an important inhibitory role in regulating allostatic systems (Balzarotti, Biassoni, Colombo, & Ciceri, Reference Balzarotti, Biassoni, Colombo and Ciceri2017; Crowley et al., Reference Crowley, McKinley, Burg, Schwartz, Ryff, Weinstein and Sloan2011; Smeets, Reference Smeets2010; Thayer & Sternberg, Reference Thayer and Sternberg2006; Weber et al., Reference Weber, Thayer, Rudat, Wirtz, Zimmermann-Viehoff, Thomas and Deter2010). For example, the low vagal tone has been associated with an impaired recovery from stress (Porges, Reference Porges2007; Weber et al., Reference Weber, Thayer, Rudat, Wirtz, Zimmermann-Viehoff, Thomas and Deter2010) and increases in vagal tone, which are speculated to be induced by taVNS, may facilitate recovery from task exertion. Notably, impaired or delayed recovery from disturbances of homeostasis have been proposed as critical factors in the development of mood disorders, suggesting that a faster recovery from stress is beneficial (Brosschot, Pieper, & Thayer, Reference Brosschot, Pieper and Thayer2005; McEwen, Reference McEwen1998). Alternatively, taVNS may increase the relative contribution of interoceptive signals forwarded via the NTS in inference processes that play a role in regulating affect (Allen, Levy, Parr, & Friston, Reference Allen, Levy, Parr and Friston2019). Put simply, taVNS may speed up recovery by facilitating the subjective inference that one's body is in an improved state after a straining episode. Another possibility is that taVNS changes the retrospective evaluation of preceding effort for rewards once they have been received and experienced during a consummatory phase such as the breakfast, our participants received prior to the third mood rating. This could be due to a modulatory effect on nigrostriatal circuits involved in cost/benefit decision-making (de Araujo, Schatzker, & Small, Reference de Araujo, Schatzker and Small2020; Neuser et al., Reference Neuser, Teckentrup, Kühnel, Hallschmid, Walter and Kroemer2020). To summarize, a taVNS-induced increase in vagal tone might alter the subjective evaluation of the preceding effort during a post-stimulation phase by modulating interoceptive feedback, which may ultimately facilitate mood recovery (Porges, Reference Porges1992).
We further found that baseline levels of positive mood moderated taVNS-induced effects on motivation in the effort task (Neuser et al., Reference Neuser, Teckentrup, Kühnel, Hallschmid, Walter and Kroemer2020). More specifically, when a positive mood was low at baseline, taVNS showed a significantly stronger impact on invigoration. A comparable dependence of taVNS effects on baseline positive mood state has been shown by Steenbergen, Colzato, and Maraver (Reference Steenbergen, Colzato and Maraver2020) on delay discounting. A possible explanation for a modulatory role of baseline mood is that people with low baseline mood are less likely to participate in effortful, potentially mood-worsening tasks (Taquet, Quoidbach, Gross, Saunders, & Goodwin, Reference Taquet, Quoidbach, Gross, Saunders and Goodwin2020), whereas elated mood states typically facilitate motivation (Young & Nusslock, Reference Young and Nusslock2016). Therefore, the potential of taVNS to improve motivation could be strongest for low baseline levels of positive mood. Alternatively, as previously hypothesized (Steenbergen et al., Reference Steenbergen, Colzato and Maraver2020), low baseline mood could be indicative of a low task-relevant arousal state which may improve more strongly by taVNS. In conclusion, taVNS may increase the motivation to engage in effort particularly in a dampened initial mood state by encouraging participants to work harder for rewards even of a lower value. In turn, this effect may help quickly restore mood once participants can reap the fruit of their labor.
Despite the conclusive evidence for improvements in mood during the post-stimulation phase, there are limitations of our study that should be addressed in future research. First, we found no effects on negative mood. This is likely due to the restricted range of baseline negative mood as well as a lack of increase in negative mood in response to the tasks in our sample as the hierarchical Bayesian model failed to converge with random effects for participants. Thus, to better resolve effects on negative mood, this study should be extended either by including a more aversive task block to induce increases in a negative mood or conducting it in a sample of patients with affective disorders such as major depression as the negative mood is a key symptom of the disorder (Diagnostic & statistical manual of mental disorders : DSM-5, 2013). Second, although we assessed mood at three time points providing a more fine-grained resolution compared to previous studies, more frequent mood ratings could help determine the exact mechanism of taVNS on mood. Third, we provided evidence that acute taVNS boosts mood recovery after effort exertion, but we did not observe an association between taVNS-induced changes in mood and taVNS-induced changes in effort parameters. However, this might be due to differential modulatory effects of individual tasks since the exertion of cognitive tasks can interfere with one's ability to regulate mood (Kohn et al., Reference Kohn, Toygar, Weidenfeld, Berthold-Losleben, Chechko, Orfanos and Habel2015), whereas physical effort can support mood regulation and improve mood (Berger & Motl, Reference Berger and Motl2000). Thus, systematically evaluating taVNS effects on mood recovery after different task types would provide a more refined understanding of taVNS-induced mood recovery. Lastly, we recognize that gastrointestinal parameters and taVNS effects on mood may be associated since we observed an effect of taVNS on mood following food intake after an overnight fast. To this end, recording an electrogastrogram in future research might shed light on the interplay of gastrointestinal motility and mood.
To summarize, the mood is a crucial moderator of everyday engagement with tasks, yet it has not been shown if non-invasive taVNS can swiftly enhance mood. Here, we showed that acute taVNS improves positive mood recovery in a post-stimulation phase, but not during the stimulation when the strain of the effortful tasks was most pronounced. Moreover, participants showed a stronger taVNS-induced increase of invigoration if they had lower baseline levels of positive mood. Collectively, our results highlight the importance of vagal afferent signaling in mood homeostasis highlighting a previously unanticipated role for taVNS in specifically boosting mood recovery after exertion. To conclude, these findings are highly relevant in light of the etiology of affective disorders that are characterized by prolonged periods of negative mood after stress or effort (McEwen, Reference McEwen1998). Therefore, our results might pave the way for integrating non-invasive taVNS as an adjunct therapy into the current affective treatment repertoire.
Supplementary material
The supplementary material for this article can be found at https://doi.org/10.1017/S0033291720005073
Acknowledgements
We thank Monja P. Neuser, Caroline Burrasch, Franziska Müller, Sandra Neubert, Moritz Herkner, and Leonie Osthof for help with data acquisition. The study was supported by the University of Tübingen, Faculty of Medicine fortune grant #2453-0-0. NBK received support from the Daimler and Benz Foundation, grant 32-04/19, the Else Kröner-Fresenius Stiftung, grant 2017_A67, and the Durham-Tübingen Seedcorn fund, grant DFG ZUK 63.
Author contributions
NBK was responsible for the study concept and design. MF collected data under supervision by NBK. NBK conceived the method and MF & NBK processed the data and performed the data analysis. MF, VT, WL, FK, AK, and NBK wrote the manuscript. All authors contributed to the interpretation of findings, provided critical revision of the manuscript for important intellectual content, and approved the final version for publication.
Conflict of interest
None.
Ethical standards
The authors assert that all procedures contributing to this work comply with the ethical standards of the relevant national and institutional committees on human experimentation and with the Helsinki Declaration of 1975, as revised in 2008.