INTRODUCTION
The Romans
When a cultural group overruns a large area or territory and spreads its ideas and philosophy, we might see changes in the state of human health as a consequence. The Romans conquered a huge empire and altered the lives of its subjects for centuries. Using changes in the ancient parasites present in the regions under Roman control, this study investigates whether Romanization can be seen to have altered the balance of disease experienced by the people who lived in Europe and the Mediterranean region 2000 years ago.
The city of Rome was traditionally founded in 753 BC, and in 509 BC, the Roman Republic was founded (Lintott, Reference Lintott2000). In 31 BC, Augustus became the first Roman Emperor following a series of civil wars (Kershaw, Reference Kershaw2013). Imperial Rome reached its greatest extent in the 3rd century AD, when the empire covered all the lands from coastal North Africa, the Near East, southern and western Europe and much of Britain (Fig. 1). While the Western Roman Empire fell in 476 AD with the conquest of Rome, the Eastern Empire with its capital of Constantinople lasted until 1453 AD as the Byzantine Empire (Brownworth, Reference Brownworth2009; Stathakopoulos, Reference Stathakopoulos2014).

Fig. 1. Map of the Roman Empire at the time of Emperor Trajan, around 117 AD.
The Roman Empire was a place of great mobility, both voluntary and forced. Soldiers, government officials, merchants and slaves moved all over the empire, and Rome itself was a major draw for the ambitious (Handley, Reference Handley2011). We might potentially find evidence for spread of people with infections if we were to find parasites, endemic in one part of the empire, in a region where their life cycle would make an endemic focus of disease impossible. While the process of ‘Romanization’ is debated, the Romans were well-known for bringing their ideas about literature, engineering, culture, cuisine, religion and hygiene wherever they ruled (Pitts and Versluys, Reference Pitts and Versluys2015). It is clear from modern research and theoretical modelling that good sanitation should have improved the health of past populations compared with those who have no sanitation (Mitchell, Reference Mitchell and Mitchell2015a ). The spread of Roman knowledge such as clean fresh water in aqueducts, under floor heating for houses, elaborate bathhouses for washing, flushing toilets, civic drains and sewers could in theory have improved the health of the inhabitants (Hobson, Reference Hobson2009; Yegül, Reference Yegül2010; Jansen et al. Reference Jansen, Koloski-Ostrow and Moorman2011; Koloski-Ostrow, Reference Koloski-Ostrow2015). In this paper, we will look at the evidence for parasites in Roman times, and by comparing with the situation before and after the Romans we will assess if these forms of sanitation seem to have led to a health benefit to those living under Roman rule.
How to study ancient parasites (palaeoparasitology)
The eggs of most intestinal helminths can be preserved for thousands of years in the right conditions, due to their tough chitinous walls. Human feces in archaeological contexts can be recovered from latrine soil, coprolites (preserved pieces of human feces) and the pelvic soil from burials, where the intestinal contents would have decomposed after death (Reinhard et al. Reference Reinhard, Confalonieri, Ferreira, Herrmann and Araújo1986; Warnock and Reinhard, Reference Warnock and Reinhard1992; Araújo et al. Reference Araújo, Reinhard and Ferreira2015; Rácz et al. Reference Rácz, Pucu de Aráujo, Jensen, Mostek, Morrow, Van Hove, Bianucci, Willems, Heller, Aráujo and Reinhard2015). While parasite eggs may also be found in ancient rubbish tips, pits or wells, it can sometimes be difficult to differentiate whether these eggs originated from humans rather than animals. For example, whipworm (Trichuris trichiura) in humans is indistinguishable on light microscopy from Trichuris suis in pigs. In such cases, archaeological context and the presence of other parasites unique to animals can help us to determine which origin is more likely (Da Rocha et al. Reference Da Rocha, Harter, Le Bailly, Araújo, Ferreira, Serra-Freire and Bouchet2006). If coprolites are being analysed, then fecal bile acids and sterols can help identify whether they are from humans, carnivore or herbivore animals (Shillito et al. Reference Shillito, Bull, Matthew, Almond, Williams and Evershed2011; Baeten et al. Reference Baeten, Marinova, De Laet, Degryse, De Vos and Waelkens2012). The ancient DNA of parasites can also be studied to differentiate species that appear similar on microscopy (Leles et al. Reference Leles, Araújo, Ferreira, Vicente and Iñiguez2008; Oh et al. Reference Oh, Seo, Chai, Lee, Kim, Park and Shin2010). However, this has yet to be undertaken on Roman period parasites.
Identifying ancient parasite eggs does require an understanding of how certain eggs tend to change over archaeological time, known as taphonomy. Roundworm eggs may lose the mammillated outer coat, whipworm may lose the polar plugs and fish tapeworm may lose the operculum. The eggs of some parasite species such as hookworm (Ancylostoma duodenale) and pinworm (Enterobius vermicularis) are particularly fragile and may be easily deformed or lost completely from archaeological samples (Reinhard and Pucu de Araújo, Reference Reinhard, Pucu de Araújo and Zuckerman2014). Research comparing microscopy with genetic techniques suggests that microscopy can underestimate past prevalence for some species, since positive genetic results may occur even when fragile eggs are no longer visible (Jaeger and Iñiguez, Reference Jaeger and Iniguez2014). Evidence for species with fragile eggs are most likely to survive in dried coprolites, but while these are commonly found in the Americas they are very rare in Europe. This means that species with a fragile wall may appear to have been rare or absent in Roman times, when they may in fact have been present.
In order to detect parasite eggs from soil sediments we need to transform solid soil to a liquid suspension, known as disaggregation (Reinhard et al. Reference Reinhard, Confalonieri, Ferreira, Herrmann and Araújo1986; Anastasiou and Mitchell, Reference Anastasiou and Mitchell2013a ). Then a number of techniques are available to concentrate the eggs, including flotation, sedimentation and microsieves. This concentrate can be mixed with glycerol and viewed with light microscopy. In a coprolite it is possible to count the number of eggs per gram of soil to estimate the degree of parasitism, but in latrines the egg concentration may be effected as much by the amount of rubbish thrown into the latrine as how concentrated the eggs were in the feces of the various users of the facilities. In consequence, in this paper we will look at the geographic distribution of parasite species rather than egg concentrations in order to evaluate change in parasite infection.
The fragile cysts of intestinal amoeba such as Entamoeba histolytica, Giardia duodenalis and Cryptosporidium parvum do not survive well in archaeological soils, and when deformed they are hard to detect with microscopy. A more effective way to detect them has been with enzyme-linked immunosorbent assay (ELISA). This technique employs antibodies that react to the proteins unique to these organisms, regardless of whether the cysts are deformed or broken (Gonçalves et al. Reference Gonçalves, Araújo, Duarte, Pereira da Silva, Reinhard, Bouchet and Ferreira2002, Reference Gonçalves, da Silva, de Andrade, Reinhard, Da Rocha, Le Bailly, Bouchet, Ferreira and Araújo2004; Frías et al. Reference Frías, Leles and Araújo2013). Ectoparasites can be detected by fine sieving of soil, and also looking for them on hair combs, mummies or ancient textiles (Mumcuoglu, Reference Mumcuoglu, Raoult and Drancourt2008; Arriaza et al. Reference Arriaza, Standen, Reinhard, Araújo, Heukelbach and Dittmar2013; Forbes et al. Reference Forbes, Dussault and Bain2013). Mummies also have the potential to preserve the ancient DNA of endoparasites, the proteins secreted by these parasites when alive, and possibly the host antibodies made to fight the infection (Anastasiou and Mitchell, Reference Anastasiou and Mitchell2013b ).
Some endoparasites have the potential to cause anaemia in their human hosts, and we can look for this when we study the skeletal remains of past populations. Examples of parasite infections that often trigger anaemia include malaria, hookworm and schistosomiasis (Garcia, Reference Garcia2009). Anaemia can manifest itself in the skeleton with pitting in the roof of the orbits (cribra orbitalia), on the cranial vault of the skull (porotic hyperostosis) and thickening of the cranial vault (Polo-Cerdá et al. Reference Polo-Cerdá, Miquel-Feucht, Villalaín-Blanco, La Verghetta and Capasso2001; Walker et al. Reference Walker, Bathurst, Richman, Gjerdrum and Andrushko2009). One study investigated the prevalence of these changes in a site close to Imperial Rome (1st–3rd century AD) and compared it with a 7th century AD population nearby (Salvadei et al. Reference Salvadei, Ricci and Manzi2001). Cribra orbitalia was present in 60–80% of children at both sites, highlighting how common anaemia was in Roman period Italy.
PARASITES PRIOR TO THE ROMAN PERIOD
In order to appreciate the consequences of Romanization following the expansion of the Roman Republic and the subsequent Roman Empire, we need to determine which parasites were present in those people living in the region before the Romans.
In Europe, the inhabitants were hunter-gatherers until around 4000 BC and then started to develop farming in the Neolithic period (4000–2800 BC). The development of metal tools led to the Bronze Age (2800–500 BC) and then Iron Age (700–100 BC). The overlap in dates is due to the gradual spread of these technologies across the continent. In the Neolithic, the intestinal parasites identified were beef or pork tapeworm (Taenia asiatica, Taenia saginata or Taenia solium), bile duct fluke (Opisthorchis sp.), Capillaria sp., E. histolytica dysentery, Fasciola genus liver fluke (Fasciola hepatica), fish tapeworm (Diphyllobothrium sp.), giant kidney worm (Dioctophymidae), hookworm (Ancylostomids), lancet liver fluke (Dicrocoelium sp.), roundworm and whipworm (Bouchet et al. Reference Bouchet, Harter and Le Bailly2003; Anastasiou, Reference Anastasiou and Mitchell2015). It can be seen that there was a mixture of zoonotic parasites from eating raw or undercooked wild animals (beef/pork tapeworm, bile duct fluke, capillariosis, fish tapeworm, giant kidney worm, lancet liver fluke) and parasites transmitted by the fecal contamination in villages (Entamoeba dysentery, hookworm, roundworm, whipworm).
In Bronze and Iron Age Europe the parasites found were E. histolytica that can cause dysentery, roundworm, whipworm, lancet liver fluke and so far just two examples of fish tapeworm (Bouchet et al. Reference Bouchet, Harter and Le Bailly2003; Le Bailly and Bouchet, Reference Le Bailly and Bouchet2013; Anastasiou, Reference Anastasiou and Mitchell2015). We do have to consider that fewer Bronze and Iron Age archaeological sites have been studied for parasites than is the case for earlier Neolithic or later Roman and medieval periods. However, we do nevertheless have parasites identified from sites in 7 different European countries in the Bronze and Iron Age (Austria, Britain, Czech Republic, Denmark, France, Germany, Poland), so this evidence should reflect the species present reasonably well. It does appear that over time, the number of species affecting people in Europe seems to have decreased from the Neolithic to the Bronze and Iron Age. There seems to have been a shift away from zoonotic parasites contracted from eating raw or undercooked animals, to parasites transmitted by poor personal hygiene (dysentery, roundworm, whipworm). Whether these wild animals were no longer being caught and eaten in the Bronze and Iron Age, or whether the meat was just being cooked better (so killing the parasites), is not entirely clear.
In the Middle East, the ancient parasite evidence before the time of the Romans is more complicated to interpret. This is because human civilization started in the region, where farming, herding and early town and city formation began perhaps 5000 years before they did in Europe (Harris, Reference Harris1996; Zeder, Reference Zeder2011). Major civilizations developed in Mesopotamia and Egypt several millennia before the expansion of the Roman Empire, and so they were already experiencing the effects of higher population density, the challenges of sanitation and long distance trade with the potential spread of infectious diseases. Intestinal parasites found in the region before Roman times include Fasciola genus liver fluke, fish tapeworm, lancet liver fluke, pinworm, roundworm, schistosomiasis (Schistosomiasis haematobium and Schistosomiasis mansoni), Taenia genus tapeworm, threadworm (Strongyloides stercoralis) and whipworm. Mummification in Egypt also allows the identification of endoparasites not preserved by the skeletonized burials of northern Europe, such as the species that cause dracunculiasis, filariasis, leishmaniasis, malaria, toxoplasmosis and trichinosis (Anastasiou, Reference Anastasiou and Mitchell2015). It is clear that there was a very broad range of parasites present in the Middle East and North Africa region prior to the arrival of the Romans. It is quite likely this resulted from the higher population density from existing civilizations, the warm climate and the fact that many parasites of humans originated in Africa (Kliks, Reference Kliks1990; Mitchell, Reference Mitchell2013).
INTESTINAL PARASITES IN THE ROMAN PERIOD
As the geographical borders of Roman influence varied over time, we will only include evidence from Roman contexts, rather than merely regions that were at some point governed by the Romans. Parasites have been identified from Roman period excavations undertaken in 10 modern countries: Austria, Belgium, Britain, Egypt, France, Germany, Israel, Italy, The Netherlands and Poland. The sites in Italy itself include Rome, Pompeii and Parma. Others are from cesspits, sewer drains, rubbish pits, bog bodies and mummified burials from across Europe and the Mediterranean region.
Human whipworms were found in 8 countries (Table 1), and based on current evidence they seem to have been the most widely spread intestinal parasite in the Roman Empire (Jansen and Over, Reference Jansen and Over1962, Reference Jansen, Over and Corradetti1966; Pike, Reference Pike, Brothwell and Sandison1968; Wilson and Rackham, Reference Wilson, Rackham and Buckland1976; Jones and Hutchinson, Reference Jones, Hutchinson and McCarthy1991; Rousset et al. Reference Rousset, Heron and Metrot1996; Aspöck et al. Reference Aspöck, Auer and Picher1999; Heirbaut et al. Reference Heirbaut, Jones, Wheeler, Jansen, Koloski-Ostrow and Moormann2011; Searcey et al. Reference Searcey, Reinhard, Egarter-Vigl, Maixner, Piombino-Mascali, Zink, van der Sanden, Gardner and Bianucci2013). This is a fecal oral parasite spread by the contamination of food with feces (Garcia, Reference Garcia2009, 332). It could have been spread by the use of unwashed hands to prepare food or by the use of human feces as crop fertilizer.
Table 1. Endoparasites found in the Roman period
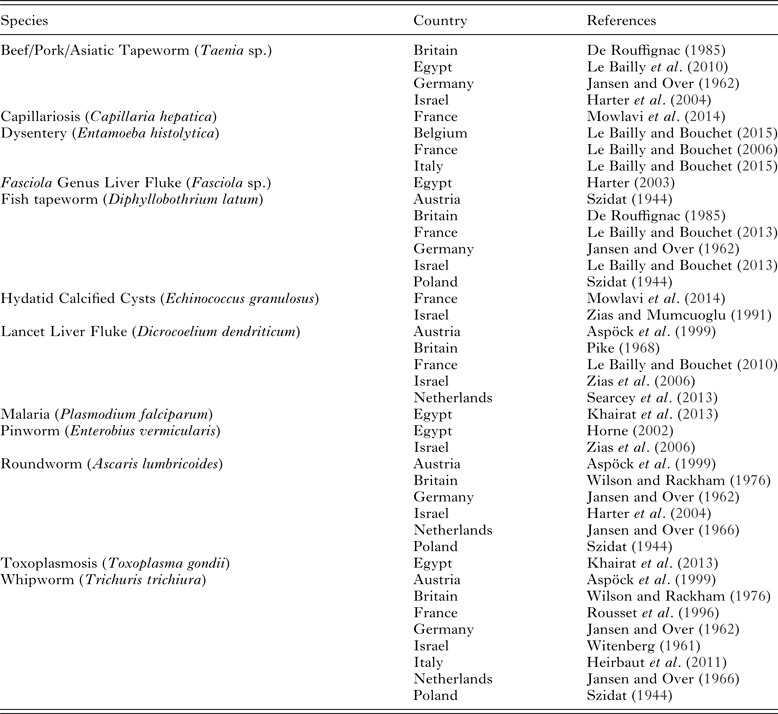
Note: (1) not all publications include an image of the eggs described, and in those cases it is possible that the claims may not be accurate. (2) Not all published examples of parasites are included in this table, but one example from each country has been included to show the geographic spread of different species.
Human roundworm was the next most widely found parasite species in Roman sites, being identified in 6 countries (Jansen and Over, Reference Jansen and Over1962, Reference Jansen, Over and Corradetti1966; Pike, Reference Pike, Brothwell and Sandison1968; Wilson and Rackham, Reference Wilson, Rackham and Buckland1976; Jones and Hutchinson, Reference Jones, Hutchinson and McCarthy1991; Rousset et al. Reference Rousset, Heron and Metrot1996; Aspöck et al. Reference Aspöck, Auer and Picher1999; Searcey et al. Reference Searcey, Reinhard, Egarter-Vigl, Maixner, Piombino-Mascali, Zink, van der Sanden, Gardner and Bianucci2013). Just as was the case for whipworm, roundworm is spread by the fecal contamination of food, and so limited hand hygiene and the manuring of crops with human feces would have facilitated its spread.
The fish tapeworms were also found in 6 countries, namely Austria, Britain, France, Germany, Israel and Poland (Szidat, Reference Szidat1944; Jansen and Over, Reference Jansen and Over1962; De Rouffignac, Reference De Rouffignac1985; Le Bailly and Bouchet, Reference Le Bailly and Bouchet2013). They are spread by eating fish of species that spend at least part of their life cycle in fresh water such as rivers or lakes. The parasite eggs of this species will not develop in sea water. If the fish is cooked, this will kill the parasite. Hence, fish tapeworm is typically found in regions where raw, smoked or pickled fish is eaten rather than cooked fish (Garcia, Reference Garcia2009, 354).
Beef/pork/Asiatic tapeworms of humans and dog tapeworm (Echinococcus sp.) eggs all appear similar on microscopy so, these can only be identified to species level using ancient DNA analysis. Eggs have been recovered from Roman Period sites in Britain, Egypt, Germany and Israel (Jansen and Over, Reference Jansen and Over1962; De Rouffignac, Reference De Rouffignac1985; Harter et al. Reference Harter, Bouchet, Mumcuoglu and Zias2004; Le Bailly et al. Reference Le Bailly, Mouze, Rocha, Heim, Lichtenberg, Dunand and Bouchet2010).From human feces, its presence indicates that raw or undercooked beef, pork or similar large farm animals were being eaten by the population.
Lancet liver flukes have been found in Austria, Britain, France, Israel and the Netherlands (Pike, Reference Pike, Brothwell and Sandison1968; Aspök et al. Reference Aspöck, Auer and Picher1999; Le Bailly and Bouchet, Reference Le Bailly and Bouchet2010; Searcey et al. Reference Searcey, Reinhard, Egarter-Vigl, Maixner, Piombino-Mascali, Zink, van der Sanden, Gardner and Bianucci2013). It is generally an infection of animals such as cattle, so if undercooked cow liver is eaten, the eggs of this parasite may end up in human feces, an example of false parasitism. However, Dicrocoelium genus parasites can potentially infect humans and may cause diarrhoea and abdominal bloating. A good example confirming genuine infection of a person in Roman times is the mummy known as Zweeloo woman, dating from 78 to 233 AD (calibrated radiocarbon date). This bog body from the Netherlands was found to have whipworm and roundworm eggs in her intestines, but the eggs of Dicrocoelium dendriticum in the preserved liver (Searcey et al. Reference Searcey, Reinhard, Egarter-Vigl, Maixner, Piombino-Mascali, Zink, van der Sanden, Gardner and Bianucci2013).
Entamoeba histolytica infection has been detected using ELISA in Roman period samples from latrines and cesspits in Belgium, France and Italy (Gonçalves et al. Reference Gonçalves, da Silva, de Andrade, Reinhard, Da Rocha, Le Bailly, Bouchet, Ferreira and Araújo2004; Le Bailly and Bouchet, Reference Le Bailly and Bouchet2006, Reference Le Bailly, Bouchet and Mitchell2015). Entamoeba histolytica is spread by the drinking of water contaminated by human feces, and can cause outbreaks of dysentery, with bloody diarrhoea, abdominal pain and fevers (Garcia, Reference Garcia2009, 256).
Hydatid disease (Echinococcus granulosus) can be identified in archaeological samples from the calcified cysts found in the abdomen or thorax of human burials. If humans inadvertently consume the eggs of this dog tapeworm, the hydatid cysts develop in the organs of the body. Good examples from the Roman period include calcified hydatid cysts in the burial of a 3rd–4th century AD adolescent at Amiens in France, and from 1st century AD Jerusalem (Zias and Mumcuoglu, Reference Zias and Mumcuoglu1991; Mowlavi et al. Reference Mowlavi, Kacki, Dupouy-Camet, Mobedi, Makki, Harandi and Naddaf2014).
Pinworm eggs have been found in the mummified remains of a teenage girl and an adult male from the Dakleh Oasis in Egypt (30BC–395 AD), and also in latrine soil from the Essenes sect at Qumran in Israel (100 BC–100 AD) (Horne, Reference Horne2002; Zias et al. Reference Zias, Tabor and Harter-Lailheugue2006). It is spread by fecal contamination of hands or by airborne transmission, and causes intense anal itching at night.
Fasciola genus liver fluke eggs have only been found in one Roman period archaeological context, in Egypt (Harter, Reference Harter2003). The liver fluke is contracted by humans who eat unwashed water plants grown in locations where farm animals such as sheep and cattle drop their dung (Garcia, Reference Garcia2009, 368).
Capillariosis (Capillaria hepatica) was identified in a burial from France (Mowlavi et al. Reference Mowlavi, Kacki, Dupouy-Camet, Mobedi, Makki, Harandi and Naddaf2014). Often capillaria eggs in the feces represent false parasitism, when uncooked animal organs had been consumed and passed unchanged in the feces. However, this example was of capillaria eggs embedded in the calcified wall of a hydatid cyst. This demonstrates genuine infection of the individual with capillariosis while they were alive.
Malaria (Plasmodium falciparum) and toxoplasmosis (Toxoplasma gondii) have been identified from their preserved ancient DNA in an Egyptian mummy dating from the third intermediate to Roman Period (Khairat et al. Reference Khairat, Ball, Chang, Bianucci, Nerlich, Trautmann, Ismail, Shanab, Karim, Gad and Pusch2013). Malaria is spread by the bite of mosquitos and infection causes fevers, enlarged liver and spleen, and may cause death especially in children (Garcia, Reference Garcia2009, 302). Toxoplasmosis is generally contracted by humans who are in close contact with cats. Since cats were cult animals in ancient Egypt with many being mummified and used as religious offerings (Ikram, Reference Ikram2005), it is possible this exposure to cats put the inhabitants of Egypt at added risk of contracting this parasite compared with other parts of the Roman Empire.
ECTOPARASITES IN THE ROMAN PERIOD
Head lice (Pediculus humanus capitis), body lice (Pediculus humanus corporis), pubic lice (Phthirus pubis), fleas (Pulex irritans) and bed bugs (Cimex lectularius) have been identified at a range of archaeological sites across the world and across time periods (Mumcuoglu, Reference Mumcuoglu, Raoult and Drancourt2008). Ectoparasites from Roman period settlements have been preserved in combs, textiles, tombs and waterlogged soils. While they have not been identified in as many countries as the eggs of intestinal helminths, this seems to reflect the location and interests of those who have been searching for them, and probably does not necessarily indicate their absence from the rest of the Roman Empire.
Head lice and their eggs have been recovered from Roman period wooden combs from the Essene religious sect at Qumran (1st century BC–1st century AD) and the Jewish fortress of Masada (1st century AD), Israel (Mumcuoglu and Zias, Reference Mumcuoglu and Zias1988). Body lice have also been noted on textiles from Masada dating to the time of Jewish revolt against the Romans (66–73 AD) (Mumcuoglu et al. Reference Mumcuoglu, Zias, Tarshis, Lavi and Stiebe2003).
In Britain, large numbers of human fleas have been recovered from sediment layers in Roman Period York (Hall and Kenward, Reference Hall, Kenward and Mitchell2015). A pubic louse was recovered from a waterlogged Roman pit dating from the late 1st to mid-2nd century AD Carlisle (Kenward, Reference Kenward1999). The probable remains of a bed bug were found at Roman Alcester in Warwickshire (Osborne, Reference Osborne1971). One study has assessed the number of ectoparasites in sediments in the same town to compare the Roman period with later times. In York, the concentration of ectoparasites in Roman, Viking and medieval layers were similar across the centuries. For example, human head and body lice were found in over 50 archaeological layers and fleas were also routinely detected (Kenward and Hall Reference Kenward and Hall1995, 488-91 and 700-3; Hall and Kenward, Reference Hall, Kenward and Mitchell2015, 114). This suggests that the Roman habit of washing at the public baths does not seem to have decreased their risk of contracting ectoparasites, compared with Vikings and medieval people who did not use public baths in the same way.
Ectoparasites are also known to spread bacterial infectious diseases amongst humans. For example, the body louse can transmit louse-borne relapsing fever (Borrelia recurrentis), epidemic typhus (Rikkettsia prowazekii) and trench fever (Bartonella qintana) (Gunn and Pitt, Reference Gunn and Pitt2012, 157). The ancient DNA of these organisms has been extracted from human skeletal remains and from the lice themselves from post-Roman archaeological sites in the past (Raoult et al. Reference Raoult, Dutour, Houhamdi, Jankauskas, Fournier, Ardagna, Drancourt, Signoli, Dang La, Macia and Aboudharam2006; Tran et al. Reference Tran, Forestier, Drancourt, Raoult and Aboudharam2011a ). It is therefore quite plausible that some Roman period epidemics could have been caused by these bacterial diseases spread by ectoparasites.
Rodent fleas such as Xenopsylla cheopis are thought to have spread bubonic plague (Yersinia pestis) in recent epidemics among humans. When rats die from plague infection, it has been noted that fleas have to transfer to feed from humans rather than starve themselves, so spreading the disease (Schotthoefer et al. Reference Schotthoefer, Bearden, Holmes, Vetter, Montenieri, Williams, Graham, Woods, Eisen and Gage2011; Chouikha and Hinnebusch, Reference Chouikha and Hinnebusch2012; Bobrov et al. Reference Bobrov, Kirillina, Vadyvaloo, Koestler, Hinz, Mack, Waters and Perry2015). It is not entirely clear which ectoparasites were responsible for spreading plague in past pandemics, since the medieval Black Death still spread across areas such as Scandinavia where rats have been shown to have been either absent or very rare (Hufthammer and Walløe, Reference Hufthammer and Walløe2013). The first pandemic of bubonic plague is known as the plague of Justinian, and took place from 541 to 543 AD. It started in the Eastern Roman Empire and then spread across the rest of Europe and the Mediterranean region (Little, Reference Little2011). Ancient DNA analysis of human skeletal remains from mass graves dating to this event has determined the genome sequence of the Y. pestis strain responsible for the pandemic (Harbeck et al. Reference Harbeck, Seifert, Hänsch, Wagner, Birdsell, Parise, Wiechmann, Grupe, Thomas, Keim, Zöller, Bramanti, Riehm and Scholz2013; Wagner et al. Reference Wagner, Klunk, Harbeck, Devault, Waglechner, Sahl, Enk, Birdsel, Kuch, Lumibao, Poinar, Pearson, Fourment, Golding, Riehm, Earn, DeWitte, Rouillard, Grupe, Wiechmann, Bliska, Keim, Scholz, Holmes and Poinar2014). This has demonstrated that the strain was much earlier on the evolutionary tree than modern plague strains, highlighting considerable evolution of the bacterium over the last 1500 years.
HOW DID THE ROMANS CHANGE PARASITISM ACROSS THE EMPIRE?
If we are going to assess the impact of Romanization across Europe, the Middle East and North Africa, then it is important to compare the evidence for parasites before, during and after the Romans. We have to be careful how we do this, to ensure the conclusions are not misleading due to limitations in the evidence. Some parasites eggs are more fragile than others (such as hookworm and pinworm) and so could have been present in the past despite lack of surviving evidence. In consequence, I will not make claims about the meaning of the presence or absence of these species here. Similarly, some time periods may have been better studied in the past and this might give us more evidence for parasites at the time. In the geographic region under study, there have extremely few studies of parasites in the Paleolithic period, a moderate number in the Neolithic, fewer in the Bronze and Iron Age, a moderate number in the Roman period and a large number in the medieval period. This must be borne in mind when comparing change between the Bronze and Iron Ages with the Roman period, in particular. However, I would argue that we actually have a fairly good idea of the parasites present in the Bronze and Iron Ages for the region that would later become the Roman Empire. There is evidence for the species present in what is now Austria, Britain, Denmark, Egypt, France, Germany, Israel and Poland, and in some countries a number of different Bronze and Iron Age sites have been analysed (Aspöck et al. Reference Aspöck, Auer and Picher1999; Anastasiou, Reference Anastasiou and Mitchell2015; Anastasiou and Mitchell, Reference Anastasiou, Mitchell and Mitchell2015). This means that if tough-walled parasite species are absent across all or many of these excavations, it is quite likely to indicate that the species was either rare or absent at that time.
Before the Romans, the Neolithic farmers of Europe and Mediterranean region could be infected with a combination of zoonotic endoparasites such as fish tapeworm, Lancet liver fluke, Fasciola genus liver fluke, bile duct fluke, capillariosis and also parasite species specifically evolved to infect humans such as roundworm, whipworm, hookworm, beef/pork tapeworm and Entamoeba dysentery protozoa. However, by the time of the Bronze and Iron Age there had already been a shift away from zoonotic parasites so that parasites spread by poor sanitation dominated. Interestingly, this is mirrored by modern nomadic hunter-gatherer societies who settle and become farmers (Dounias and Froment, Reference Dounias and Froment2006). Hence, at the time the Romans expanded their empire the dominant parasites in Europe were roundworm, whipworm and Entamoeba protozoa that cause dysentery. Interestingly, Lancet liver fluke was also fairly widespread, suggesting that ants were either accidentally or deliberately consumed. The picture in the Middle East and North Africa at the arrival of the Romans is more complex as ancient civilizations already existed there. In these regions, the range of endemic species was much greater than was the case for Europe.
At the time of the Romans, we see the most widespread and dominant species were roundworm and whipworm. The Romans are credited with spreading the concept of hygienic latrines across the empire, with contoured seats, flushing systems, personal hygiene with a sponge on a stick and hand washing (Hobson, Reference Hobson2009; Jansen et al. Reference Heirbaut, Jones, Wheeler, Jansen, Koloski-Ostrow and Moormann2011; Antoniou and Angelakis, Reference Antoniou, Angelakis and Mitchell2015; Koloski-Ostrow, Reference Koloski-Ostrow2015). They introduced the culture of person cleanliness with the regular washing in heated public baths (Yegül, Reference Yegül2010). The Romans also introduced legislation whereby human waste from towns and cities would be collected in carts and taken out to the countryside (Taylor, Reference Taylor and Mitchell2015). Modern research has shown that clean drinking water, latrine use and hand washing all decrease the prevalence of geohelminths such as whipworm and roundworm (Zeigelbauer et al. Reference Zeigelbauer, Speich, Mäusezahl, Bos, Keiser and Utzinger2012). In view of this, we might expect the prevalence of fecal oral parasites such as whipworm and roundworm to drop in Roman times rather than increase. However, this was not the case and we must ask ourselves why.
One possibility is that the eggs of roundworms and whipworms were spread via the warm communal waters of the Roman bathhouses. In some baths the water was only changed intermittently, and could acquire a scum on the surface from human dirt and cosmetics. Clearly, not all Roman baths were as clean as they might have been (Fagan, Reference Fagan and Jansen2000; Yegül, Reference Yegül2010). Another possible explanation is that the Romans were known to have used the human feces collected from towns to fertilize crops growing in the fields (Jones, Reference Jones2012; Taylor, Reference Taylor and Mitchell2015). This has been shown in modern research to increase crop yields (Heinonen-Tanski and van-Wijk-Sebesma, Reference Heinonen-Tanski and van Wijk-Sebesma2005). However, unless the feces are composted for many months before being added to the fields, this can result in the spread of viable parasite eggs to the plants grown (Phuc et al. Reference Phuc, Konradsen, Phuong, Cam and Dalsgaard2006; Jensen et al. Reference Jensen, Phuc, Knudsen, Dalsgaard and Konradsen2008). Hence, it may be the use of human feces as crop fertilizer under the Romans that led to an increase in roundworm and whipworm despite their use of sanitation technologies.
Fish tapeworms were found to be surprisingly widespread in the Roman period. This is in contrast to Bronze and Iron Age Europe, where fish tapeworm eggs have only been in France and Germany (Le Bailly and Bouchet, Reference Le Bailly and Bouchet2013). Bearing in mind there are Bronze and Iron Age sites where parasites have been identified from 7 different countries, the identification of fish tapeworm in just 2 countries suggests the parasite was relatively uncommon at that time. In the Roman period it was identified in 6 countries, which was as many as roundworm. Roundworm and whipworm are perceived by modern researchers to have been more ubiquitous in past farming populations than all other intestinal helminths (Reinhard et al. Reference Reinhard, Ferreira, Bouchet, Sianto, Dutra, Iñiguez, Leles, Le Bailly, Fugassa, Pucu and Araújo2013; Reinhard and Pucu de Araújo, Reference Reinhard, Pucu de Araújo and Zuckerman2014). However, this study has shown for the first time quite how widespread fish tapeworm was at the time of the Romans. It is unlikely that the increase in its detection in Roman period samples is due to chance. It is notable that five of the 6 countries where fish tapeworm was identified were in northern Europe. In northern Europe it was common in the past to smoke, dry, salt or pickle fish to preserve it, and it was often eaten uncooked (Hoffman, Reference Hoffman2003; Hagen, Reference Hagen2006, 275–276). The culture of eating uncooked fish may be one element as to why the parasite was quite widespread at this time, but does not explain why there appears to have been an increase between the Bronze/Iron Age and the Roman Period. We must ask ourselves which aspect of Romanization in Europe seems to have triggered this apparent rise in fish tapeworm.
One possibility that could explain a change in fish tapeworm distribution in the Roman period is the Roman enthusiasm for fish sauce, known as garum, liquamen or muria (Cool, Reference Cool2006, 58). It was not only used as a culinary ingredient, but also as a medicine (Curtis, Reference Curtis1984). Garum was a fermented sauce made from pieces of fish, herbs, salt and flavourings. This sauce was not cooked, but allowed to ferment in the sun (Curtis, Reference Curtis1983). Garum seems to have its origins in the Mediterranean region, but over time was made in northern Europe where fish tapeworm was much more common. Archaeological examples of garum in Roman pottery vessels have been recovered at many sites, and the bones of fish from these pots include both sea fish and fresh water fish (Van Neer et al. Reference Van Neer, Wouters, Ervynck and Maes2005; Locker, Reference Locker2007). Garum was traded right across the empire, ensuring that people living in regions that were not endemic for fish tapeworm would still have been at risk of contracting the parasite (Curtis, Reference Curtis1988; Haley, Reference Haley1990). It is quite possible that this fermented fish sauce may have acted as a vector for the spread of fish tapeworm and hence contributed to the rise in the archaeological evidence for fish tapeworm in the Roman Empire.
We can see that in Roman times we still have evidence for genuine parasitism of humans by zoonotic parasites that generally affect animals rather than humans today. The Zweeloo woman bog body was found to have infection by Lancet liver fluke in her liver at the time of death (Searcey et al. Reference Searcey, Reinhard, Egarter-Vigl, Maixner, Piombino-Mascali, Zink, van der Sanden, Gardner and Bianucci2013). An individual from 3rd to 4th century Amiens in France was found to have the eggs of C. hepatica entombed in the calcified walls of hydatid cysts, so demonstrating a case of true infective capillariosis during their life (Mowlavi et al. Reference Mowlavi, Kacki, Dupouy-Camet, Mobedi, Makki, Harandi and Naddaf2014). This illustrates how certain parasites that rarely infect humans today may have caused active infection in past peoples living a less hygienic life style than in modern times.
In Roman times, we have parasite species such as beef and pork tapeworm spread by the consumption of farm animals. We also see the appearance of echinococcosis from dog tapeworm, compatible with the close contact between humans and dogs kept for herding animals or as pets (O'Connor, Reference O'Connor2008). In Egypt, the reverence for cats may have facilitated the spread of toxoplasmosis in Roman times there.
It is also interesting to consider the absence of certain parasites in the Roman Empire. We might expect to have found the eggs of S. haematobium or S. mansoni in Roman latrines in Europe as soldiers, officials and merchants moved between the Middle East and the rest of the empire. There is good archaeological evidence for such migrations from analysis of skull shape, stable isotopes in teeth and written Roman texts (Leach et al. Reference Leach, Lewis, Chenery, Müldner and Eckardt2009). Schistosomiasis has been identified in Syria and Egypt well before the Roman Period (Anastasiou et al. Reference Anastasiou, Lorentz, Stein and Mitchell2014; Anastasiou and Mitchell, Reference Anastasiou, Mitchell and Mitchell2015). Both S. haematobium and S. mansoni have been recovered from medieval latrines in France dating from 1450 to 1550 AD, indicating the migration of travellers between France and areas endemic for schistosomiasis (Bouchet and Paicheler, Reference Bouchet and Paicheler1995; Bouchet et al. Reference Bouchet, Harter, Paicheler, Araújo and Ferreira2002). However, such evidence for migration with parasites has yet to be identified at Roman period sites.
We can get an indication of how parasite infection changed after the Roman Period by comparing excavations of levels from different time periods at the same sites. In Italy, whipworm was the only species of helminth egg recovered from Roman Period Parma. This is in contrast to the 6 species found in the same town during the medieval period (roundworm, whipworm, lancet liver fluke, fish tapeworm, Taenia/Echinococcus sp. and Capillaria sp.) (Bosi et al. Reference Bosi, Mazzanti, Florenzano, N'siala, Pederzoli, Rinaldi, Torri and Mercuri2011; Florenzano et al. Reference Florenzano, Mercuri, Pederzoli, Torri, Bosi, Olmi, Rinaldi and Mazzanti2012). This increase in species found in the same time over this 1000-year period gives an indication of how parasite infections do appear to have been rather different in Roman times compared with later periods. The change may reflect the increasing population size of medieval towns compared with most classical towns in Europe outside Rome itself, as larger populations generally sustain infectious diseases better than small populations. In the medieval period fish tapeworm was even more common than in the Roman Empire, and this may reflect the spread of Christianity and the eating of fish on Fridays and other religious fast days in medieval times (Yeh et al. Reference Yeh, Pluskowski, Kalējs and Mitchell2014; Mitchell, Reference Mitchell2015b ).
MEDICAL HISTORY OF ROMAN PARASITES
Having demonstrated that a range of endoparasites and ectoparasites infected people living in the Roman Empire, it makes sense to consider what they thought of their parasites, and whether they may have tried to treat them medically.
The core philosophy underpinning Roman beliefs about medicine originated in classical Greece, largely from the Hippocratic corpus of texts. Hippocrates was a medical practitioner born on the island of Kos in the Aegean Sea. He lived during the 5th and 4th centuries BC and the texts he wrote were augmented by those of his students and laid out a new way of thinking about how disease originated. Hippocrates argued that a state of disease could be triggered by an imbalance in the proportions of the four humours (black bile, yellow bile, blood and phlegm) or a corruption of one of these humours (Totelin, Reference Totelin2009; Mann, Reference Mann2012). The key texts that mention intestinal helminths were in the three works entitled Prognostics, Epidemics and Coan Prognoses (Hippocrates, Reference Hippocrates and Littré1839; Trompoukis et al. Reference Trompoukis, German and Falagas2007).
The leading Roman period medical practitioner was Aelius Galenus, who lived from c.130 AD to c.210AD. Galen was a physician to the Roman Emperors Marcus Aurelius (ruled 161–180 AD), Commodus (ruled 180–192 AD) and Septimus Severus (ruled 193–211 AD), and wrote a large collection of medical texts (Johnston, Reference Johnston2006; Hankinson, Reference Hankinson2008; Mattern, Reference Mattern2013). He followed the humoural theory of Hippocrates, and also adopted his basic classification of helminths. Galen described three groups of intestinal worms, named lumbrici lati, teretes and ascarides. We do have to be careful when using ancient texts to come to a modern diagnosis of past disease, as there are many factors that might complicate a seemingly obvious description (Mitchell, Reference Mitchell2011). However, from these descriptions it has been proposed that these terms probably equate to Taenia genus tapeworms, roundworm and pinworm, respectively (Jirsa and Winiwarter, Reference Jirsa and Winiwarter2010). Galen believed that helminths were formed from spontaneous generation in putrified matter under the effect of heat. He recommended treatment with dietary modification, bloodletting and medicines that were believed to have a cooling and drying effect, in order to return the humoural balance to normal. Galen's beliefs as to the origin and treatment of intestinal parasites were to become the accepted view in Europe and the Middle East for over 1000 years, until the time of the Renaissance and the Enlightenment.
While medical practitioners attempted to treat certain types of parasite with medicines, we have archaeological evidence that people also tried to physically remove head lice with special combs. In Roman Period Judea (Israel), two-sided combs of wood, bone or ivory have been excavated. On one side the teeth of the comb were well spaced, presumably in order to comb the hair. On the other side the teeth were placed very close together, in order to remove the head lice and eggs. Indeed both lice and their eggs have been noted on microscopy, caught between the fine teeth (Mumcuoglu and Zias, Reference Mumcuoglu and Zias1988).
Concluding remarks
We can see that 12 species of endoparasite and 5 species of ectoparasite have been identified in Roman Period archaeological sites. Roman technology such as flushing latrines, clean piped water, sewers and drains, hand washing and bath houses does not seem to have protected them from contracting either intestinal parasites or ectoparasites. Using human feces as crop fertilizer may explain why roundworms and whipworms were so common in the Roman period. Certain ectoparasites may well have spread infectious diseases, and one likely example to have been spread by flea bite was the Plague of Justinian, now known to have been an early strain of bubonic plague.
One unexpected finding from this analysis was that fish tapeworms seem to have been fairly widespread in Roman Period Europe, in contrast to the evidence from the Bronze and Iron Age. After considering possible explanations, it seems plausible that the Roman enthusiasm for the fermented fish sauce known as garum may have acted as a method where fish tapeworm eggs could have been transported large distances across the empire and then be consumed without being subjected to cooking.
We have presented archaeological evidence for genuine infection by parasites that rarely cause disease in people living today. Examples of lancet liver fluke and capillariosis that were not false parasitism suggest that the way of life under the Romans may have facilitated the invasion of human tissues by these organisms more easily than do modern lifestyles.
Roman doctors believed intestinal worms were formed by spontaneous generation from putrefied matter under the effect of heat. They tried to treat them by rebalancing the four bodily humours with dietary modification, bloodletting and medicines. There is also evidence for special delousing combs to strip head lice from the hair, and delousing may have been a daily routine for many people living across the Roman Empire.
FINANCIAL SUPPORT
This research received no specific grant from any funding agency, commercial or not-for-profit sectors.