Introduction
This paper reports on the continuing work by our team of mammalogists and parasitologists who participated in the Mongolian Vertebrate Parasite Project (MVPP). The field work of the MVPP first started in 1999 and funding from the United States National Science Foundation was secured to start the main expeditionary field research in the summer of 2009 with fieldwork continuing through 2012. The overall goal of the MVPP was to discover, describe and document the distribution of vertebrates and their parasites in the south-central and south-western areas of the Gobi Desert/steppe-grasslands and eastern Altai Mountains in Mongolia (see summary in Tinnin et al., Reference Tinnin, Gardner and Ganzorig2008).
During our NSF-funded field expeditions to Mongolia (1999–2012), several cestodes were recovered at necropsy from individuals of Ellobius tancrei Blasius, 1884 (Rodentia: Arvicolinae) (Fig. 1) which is the only subterranean species of the sub-family Arvicolinae thus far recorded in Mongolia (Wilson et al., Reference Wilson, Lacher and Mittermeier2017). In addition to the mole-voles, also occurring in isolated populations throughout the area are Myospalax aspalax Pallas, 1776 and Myospalax psilurus Minle-Edwards, 1874, subterranean rodents of the family Spalacidae. These rodents occupy suitable habitats in north-central and north-eastern Mongolia, respectively (Batsaikhan et al., Reference Batsaikhan, Samiya, Shar, Lhagvasuren and King2014). Only a single helminth parasite, Ascarops strongylina (Rudolphi, 1819) species has been reported from M. psilurus collected from eastern Mongolia (Ganzorig et al., Reference Ganzorig, Batsaikhan, Samiya, Morishima, Oku and Kamiya1999).

Fig. 1. Digital image of the symbiotype host Ellobius tancrei Blasius 1844 collected at Baitag Bogd, Hovd province, Mongolia.
At the current time, five species of Ellobius Fischer, 1814 have been recorded from the Palearctic region with species occupying suitable habitat as far west as the southern Ukraine and as far east as south-central Mongolia and north-central China (Wilson et al., Reference Wilson, Lacher and Mittermeier2017). Current knowledge of the mammal fauna of Mongolia shows that only a single species of Ellobius is known (Musser and Carleton, Reference Musser, Carleton, Wilson and Reeder2005; Tinnin et al., Reference Tinnin, Ganzorig and Gardner2011). The distribution of E. tancrei within Mongolia extends from the south-eastern Gobi-steppe region through the central steppe grasslands and the Khangai mountains through the north-western regions of the country. Habitat types consist of relatively hard but friable soils in mountain steppe, dry steppe and semi-desert zones (Batsaikhan et al., Reference Batsaikhan, Samiya, Shar, Lhagvasuren and King2014).
Interestingly, before our expeditionary work in Mongolia, cestodes of the genera Echinococcus, Taenia, Hymenolepis, Mesocestoides, Nomadolepis and Catenotaenia had been reported from the species of Ellobius only by Tokobaev (Reference Tokobaev1960) from the Kyrgyz Republic, by Zanina and Tokobaev (Reference Zanina and Tokobaev1962) from Tajikistan and by Alfonso et al. (Reference Alfonso, Knapp, Tête, Umhang, Rieffel, van Kesteren, Ziadinov, Craig, Torgerson and Giraudoux2015) from Sary Mogol, Alay valley, Kyrgyzstan. In 2015, work by a field party from the Museum of Southwestern Biology (MSB) reported A. microtis from Microtus gregalis (Pallas, 1779), A. gulyaevi Makarikov et al. Reference Makarikov, Galbreath and Hoberg2013 from Myodes rufocanus (Sundevall, 1846) and A. macrocirrosa Makarikov et al. Reference Makarikov, Gulyaev and Kontrimavichus2011 from Myodes rutilus (Pallas, 1779), all were collected from Uvs province in north-western Mongolia (Haas et al., Reference Haas, Hoberg, Cook, Henttonen, Makarikov, Gallagher, Dokuchaev and Galbreath2020), which makes the current report the fourth occurrence record of Arostrilepis tapeworm species found from Mongolia.
The most important morphological synapomorphies for species included in the genus Arostrilepis (Cestoda: Hymenolepididae) are: cirrus with long spines, lack of any kind of both a rostellum or apical organ, oblong eggs with embryos pointed or tapered on the ends and delicate minute embryonic hooks (see definitions of hymenolepidid eggs in Ubelaker, Reference Ubelaker and Arai1980). Species of Arostrilepis have a Holarctic distribution, currently with 15 species reported to occur in 27 species of mammals between latitudes 36°S and 75°N (see Fig. 2). At the present time, arvicoline rodents serve as definitive hosts for more than 80% of the species of these cestodes (Makarikov et al., Reference Makarikov, Galbreath, Eckerlin and Hoberg2020), and the number is increasing.

Fig. 2. Map of Mongolia showing collection localities of four species of Arostrilepis from arvicoline rodents through the western part of the country. Note that collections were made from several dozen separate localities spanning the western half of Mongolia and these four records represent the only places where the cestodes were found.
The first species known from this group was Arostrilepis horrida (Linstow, 1901) which was initially described as Taenia horrida Linstow, 1901 from Rattus norvegicus (Berkenhout, 1769) (Rodentia: Muridae) from the Lower Saxony (Niedersachsen) area of Germany (Linstow, 1901) and subsequently placed in the genus Hymenolepis Weinland, 1858. Makarikov et al. (Reference Makarikov, Gardner and Hoberg2012) described two new species of Arostrilepis from disparate rodent groups including Peromyscus Gloger, 1841, a member of the widespread new-world family Cricetidae and from a species of Thomomys Wied-Neuwied, 1839 of the mostly Nearctic Geomyidae. More recently, from the Appalachian mountains area of eastern North America, Makarikov et al. (Reference Makarikov, Galbreath, Eckerlin and Hoberg2020) reported two new species of Arostrilepis, including one from jumping mice, Napaeozapus insignis (Miller, 1891), of the Holarctic family Dipodidae, a heretofore unreported host group for these cestodes, and the other from a southern red-backed vole, Myodes gapperi (Vigors, 1830).
Almost all tapeworms have complex life-histories that require multiple hosts with specific biological, physiological and linked ecological processes that must be perfectly aligned for them to infect these hosts successfully and then, to be transmitted to the next trophic level. Unfortunately, very little is known about the natural life-cycles of the species of Arostrilepis even though a proven intermediate host of at least one species includes springtails (Hexapoda: Collembola) (see Smirnova and Kontrimavichus, Reference Smirnova and Kontrimavichus1977). Springtails are speciose, globally diverse, omnivorous, free-living animals that live in moist habitats, and relatively recently, an experimental study on the development of metacestode stages of A. microtis in springtails has been published (Ishigenova et al., Reference Ishigenova, Berezina and Makarikov2018). Even with these new data, general information about the natural intermediate hosts, life-cycles and ecology of most species of Arostrilepis is sparse at this time, and much more ecological information is needed to discover the intermediate hosts used by these cestodes throughout their ranges. The current paper is a continuation of the process of the documentation part of the DAMA protocol that was described by Brooks et al. (Reference Brooks, Hoberg, Gardner, Boeger, Galbreath, Herczeg, Mejía-Madrid, Racz and Dursahinhan2014).
Materials and methods
In the field in Mongolia, mole-voles were captured using Victor MaCabee™ gopher traps (Victor Pest Management LLC, NY, USA) that were placed in active burrow systems that had evidence of freshly expulsed and mounded soil; these traps euthanize the animal instantly when the vole enters the trap. Our experience shows that the voles appear to work or burrow almost continuously over a 24 h period, so traps were checked at various intervals during both day and night. Elevations from where mole-voles were collected in Mongolia range from about 800 m above sea level (masl) at the lowest in the south-eastern grasslands of the Gobi up to approximately 3000 (masl) in the south and west of the country (Batsaikhan and Tinnin, Reference Batsaikhan and Tinnin2016).
At time of collection, each individual mammal was placed in a single use plastic bag and transported to the field-lab where each specimen was provided a field collection number, standard measurements were taken and tissues were preserved for future study following Yates et al. (Reference Yates, Jones, Cook, Wilson, Cole, Nichols, Rudram and Foster1996) and Galbreath et al. (Reference Galbreath, Hoberg, Cook, Armien, Bell, Campbell, Dunnum, Dursahinhan, Eckerlin, Gardner, Greiman, Henttonen, Jimenez, Koehler, Nyamsuren, Tkach, Torres-Perez, Tsvetkova and Hope2019). Specimens were then necropsied, and internal parasites were collected and processed by standard methods following Gardner and Jiménez-Ruiz (Reference Gardner, Jiménez-Ruiz, Kunz and Parsons2009).
For the study of the morphological characters of these cestodes, we followed the methods outlined by Gardner and Jiménez-Ruiz (Reference Gardner, Jiménez-Ruiz, Kunz and Parsons2009) with modifications as follows. Tapeworm strobilae discovered at necropsy were relaxed for 20 min in stream water immediately after extraction from the host intestine. They were subsequently killed and fixed in a hot 10% aqueous formalin solution, placed in a Wheaton™ snap cap vial (Fischer Scientific, Pittsburgh, PA, USA), a label was added indicating host, locality, fixative and other data, sealed and transported to the HWML where they were stored in the same solution until study.
In the laboratory, whole mounts were stained with Semichon's acetic carmine, dehydrated in an ethanol series, cleared in cedarwood oil or terpineol, washed briefly in xylene and mounted permanently on microscope slides in Damar gum under a No. 1 cover glass.
For molecular investigation, a part of each individual specimen was removed before fixation and stored for future molecular analysis. Specifically, several gravid, but not terminal, proglottids were removed and either snap-frozen in liquid nitrogen or preserved in 95% ethanol and were subsequently frozen at −80°C in the Parasite Genomic Research Facility in the Manter Laboratory. Note that the terminal gravid proglottids should not be used for molecular study because it is necessary to examine the terminal proglottids on complete specimens to determine the anapolytic or apolytic state. It is also important to know how many proglottids were removed from individual specimens to afford an accurate count of total number of proglottids on a strobila.
All parasite specimens studied were deposited in the HWML, and museum catalogue numbers were assigned to each specimen. Specimens were studied with a Zeiss Axiophot™ microscope (Carl Zeiss Microscopy, LLC, White Plains, NY, USA) using both bright-field and Normarsky™ illumination. Images were prepared using Zeiss AxioVision™ (V4.6.3.0) software (Carl Zeiss Microscopy) and Adobe Photoshop CS5™ (Adobe LLC, Damascus, OR, USA). Line drawings (see Fig. 3) were prepared directly from images captured with the Zeiss microscope using the layers function in Photoshop and lines were traced using a stylus equipped Wacom-Intuos™ tablet (Wacom Technology Corporation, Portland, OR, USA). Measurements of organs in mature segments were taken from the last five mature segments, which are the segments immediately anterior to the segment in which eggs begin to appear in the developing uterus. To ensure normality of measurements and to enable the analysis in TNT, all mensural values were log-transformed in either SAS 9.4 or Microsoft Excel. In the description, all measurements are given in micrometres unless otherwise indicated.

Fig. 3. Total evidence phylogenetic tree of all known species of Arostrilepis. The tree was constructed using the phylogenetics program TNT 1.5 (Goloboff and Catalano, Reference Goloboff and Catalano2016). Data used to construct the tree included morphological characters (both qualitative and quantitative) and 516 base pairs of DNA from cytochrome-b. Hymenolepis diminuta was used as an outgroup.
For analysis of DNA sequence from an individual cestode, frozen material of specimens stored in the HWML Parasite Genomic Research Facility (PGRF) was used. Non-terminal posterior proglottid tissue samples were used for DNA extraction using Qiagen™ DNeasy Tissue Kits® (Qiagen, California, USA). Cytochrome-b (cytb) was amplified by PCR using the forward primer HYM01 (5′ATTGTGGTTYTGTTGAATGC) and reverse primers HYM08 (5′GGGATTTTTACTA CCACTCTTGTGC), HYM22 (5′ AAGAAACCGAAAACAATGGAT) and HYMLEM02 (5′CCCACAATAGCAAAYCCCAARCATACATG) (Makarikov et al., Reference Makarikov, Galbreath and Hoberg2013). All existing cytb sequences stored in GenBank for Arostrilepis were downloaded and aligned with Clustal Omega to estimate the extent of evolutionary divergence using genetic distances with the cytochrome-b sequences among Arostrilepis species for which molecular data are available, we included 516 positions from the dataset, and we analysed the matrix of DNA sequences (which included 11 species: see Table 1) with MEGA X (Kumar et al., Reference Kumar, Stecher, Li, Knyaz and Tamura2018; Nei and Kumar, Reference Nei and Kumar2000). Genetic distance analyses were conducted using the Maximum Composite Likelihood model (Tamura et al., Reference Tamura, Nei and Kumar2004) and rate variation among nucleotide sites was modelled with a gamma distribution (shape parameter = 1). For this part of the analysis, all positions containing gaps and missing data were eliminated (complete deletion option).
Table 1. Estimates of genetic distance among the species of the genus Arostrilepis using 12 cytochrome-b gene sequences with a total of 516 positions using MEGA-X (Kumar et al., Reference Kumar, Stecher, Li, Knyaz and Tamura2018)

Evolutionary divergence was estimated using the Maximum Composite Likelihood model (Tamura et al., Reference Tamura, Nei and Kumar2004). GenBank accession numbers are provided after each species name.
For the phylogenetic analysis of the total evidence data matrix (see Supplementary material), we used the options: new technology search and implicit enumeration in the program TNT, version 1.5 (available for free use via sponsorship of the Willi Hennig Society) (see Goloboff and Catalano, Reference Goloboff and Catalano2016). To insure that we used as many data as possible for the phylogenetic estimations, we employed a total evidence approach (Kluge, Reference Kluge1989) to develop and analyse our dataset which consisted of morphological continuous-mensural, discrete-qualitative (Weaver et al., Reference Weaver, Monks and Gardner2016) and DNA sequence characters.
All mammal specimens collected during the MVPP, including individuals of Ellobius obtained during this study, have been deposited in the Division of Mammals, MSB, University of New Mexico, Albuquerque, New Mexico. All parasite samples were deposited in the Harold W. Manter Laboratory of Parasitology, University of Nebraska State Museum, Lincoln, Nebraska as voucher and type specimens and all frozen parasite specimens are stored in the freezers of the HWML-PGRF. Genetic data for the parasites studied herein were deposited in GenBank, and tissues of these parasites are stored in the freezers of the parasite genomic research facility in the Manter Laboratory. The current paper is a continuation of the process of the documentation part of the DAMA protocol that was described by Brooks et al. (Reference Brooks, Hoberg, Gardner, Boeger, Galbreath, Herczeg, Mejía-Madrid, Racz and Dursahinhan2014).
Results
The collection locality of the specimens used in this study was the northeast foothills of Baitag Bogd mountain, part of the high Asian Altai Mountain range, located in Hovd province in south-western Mongolia (see Fig. 2). During our work in Mongolia, 103 individuals of E. tancrei were collected and we found two infected with an undescribed species of cestode from a single locality.
Following is the description of a new species of Arostrilepis. All measurements are given in micrometres unless otherwise noted. N is the number of individual morphological characters measured. In the following description, the range of minimum to maximum measurement values is given, and the mean value is separated by a comma followed by standard deviation in parentheses. Measurements of organs in mature segments were taken from the last mature segment and the four segments anteriad, the last mature segment was that one segment immediately anterior to that in which eggs were observed in the developing uterus.
Description
Arostrilepis batsaikhani n. sp.
Four specimens were studied for the following description, not all characters visible in each specimen (see Fig. 4A–F); therefore, the number of characters measured is noted as n. Scolex unarmed (see Fig. 4A), n = 4, 151–272, 208 (44) long by n = 4, 170–386, 276 (102) wide. Suckers, n = 8, 106–206, 155 (33) long by n = 8, 68–176, 125 (44) wide. Neck, n = 4, 98–118, 1094 (78) long by n = 4, 108–275, 191 (68) wide. Apical organ (rostellum) not present. Strobila craspidote, n = 3, 97–143, 123.6 (24) mm long by n = 4, 2–3126, 2.53 (0.46) mm in maximum width. Minimum number of proglottids is 1350 (excluding 10 proglottids taken for gene sequencing). Mature proglottid, n = 30, 154–255, 198 (31) long by n = 30, 1116–1682, 1355 (164) wide (see Fig. 4D). Gravid proglottids n = 15, 292–447, 370 (45) long by n = 15, 2872–3125, 3003 (91) wide (see Fig. 4E). Proglottids wider than long. Specimens used in this description appear to be anapolytic (see Fig. 4F). In the holotype specimen, it is clear that the eggs have passed out of the last segments of the strobila before the spent segments have detached. However, in the four other individuals, this character was not observed because the posterior segments were cut-off for molecular analysis. Strobilar margins serrate, with intersegmental boundaries well defined in both mature and gravid proglottids.

Fig. 4. (A–F) Line drawings and images of Arostrilepis batsaikhani n. sp. (A) Scolex, showing suckers and lack of apical organ. (B) Drawing of embryo removed from the egg shell showing larval hooks. (C) Digital image of egg showing diagnostic embryophore with bilaterally attenuated shape. (D) Line drawing of the last mature proglottid, dorsal view. (E) Digital image of gravid proglottids showing uterus filled with eggs in sub-terminal proglottids. (F) Digital image of sub-terminal proglottids filled with eggs and terminal proglottid with lack of eggs.
Genital pores unilateral, dextral. Cirrus n = 24, 29–58, 45 (8) long by n = 24, 12–21, 16 (3) wide. Antiporal side of cirrus sac does not overlap osmoregulatory canals. The cirrus sac piriform, n = 22, 102–170, 134 (19) long by n = 22, 36–43, 36 (4) wide. Cirrus spines n = 50, 4–5, 4 (0.2). Internal seminal vesicle n = 20, 48–109, 69 (14) long by n = 20, 28–50, 39 (6) wide. External seminal vesicle n = 20, 150–255, 202 (24) long by n = 20, 53–98, 71 (10) wide. Testes relatively large equal in size, a single testis may extend from anterior to posterior margins of segment, n = 40, 138–249, 180 (29) long by n = 40, 123–209, 161 (23.06) wide. Ovary n = 30, 116–260, 177 (47) long by n = 30, 282–499, 378 (60) wide, arranged linearly with two on antiporal side of ovary and one on poral side of ovary. In rare cases, all three testes may occur on antiporal side of ovary. Vitelline gland n = 30, 58–119, 90 (16) long by n = 30, 97–212, 146 (31) wide. Seminal receptacle n = 30, 256–505, 349 (67) long by n = 30, 29–69, 45 (11) wide. Uterus saccular, developing as a transverse tube beginning centrally and slightly anterior in segment and extending laterally as it develops in its saccular shape. Developing uterus crossing both dorsal and ventral excretory canals laterally. Uterus passing dorsally to the dorsal and ventral excretory canals. Also, in developing segments when uterus first appears it extends to the level of the distal end of the cirrus. As eggs begin to appear and mature, the uterus expands, eventually filling the whole segment well-overlapping the lateral excretory canals on both sides of the strobila. Eggs with relatively smooth eggshells (Fig. 4C), n = 40, 22–35, 28 (3) long by n = 40, 39–54, 47 (3) wide. Developing eggs appear before the uterus reaches lateral margins of segments. Embryophore n = 40, 9–13, 10 (1) long by n = 40, 21–35, 30 (3) wide. Oncosphere size n = 40, 6–9, 7 (1) long by n = 40, 8–15, 10 (3) wide. Hooks in onchosphere embryo delicate (Fig. 4B), lengths of hooks n = 31, 6–7, 6 (0.2). From each egg, morphological characters of the hooks are as follows: lateral hook pairs dimorphic consisting of one small or more delicate hooks and one more robust hook with a larger guard and slightly thicker blade. Middle pair of hooks both delicate not dimorphic.
Taxonomic summary
Symbiotype (see Frey et al., Reference Frey, Duszynski, Gannon, Yates and Gardner1992): Ellobius tancrei Blasius, 1884, MSB, Division of Mammals, catalogue no. MSB267768, field collection number NK224165, collector: Terry R. Haverkost, collector number TRH1552.
Date of collection: 16 July, 2012.
Holotype: Harold W. Manter Laboratory: HWML216390.
Paratypes: HWML216391, HWML216392, HWML216393, HWML216394.
Site of infection: Small intestine, duodenum.
Type locality: Buduun Hargait Gol, North side of Baitag Bogd, Bulgan soum, Hovd province, Mongolia (45°15′47.1240″S, 90°57′06.0840″W, 1992 m – taken with GPS).
Etymology: This species was named in honour of Batsaikhan Nyamsuren, Professor of Zoology, National University of Mongolia, in recognition of his years of enduring commitment to research in biodiversity and conservation biology in Mongolia.
Prevalence at type locality: 2/10 = 20%.
Infection intensity: Four individuals in one host.
GenBank accession number: MW645238.
Life cycle: The life cycle of this species is unknown.
Differential diagnosis
At the current time, 15 species of Arostrilepis are known only from rodents in the Northern Hemisphere. Arostrilepis batsaikhani n. sp. represents the 16th species in this genus and shares a common ancestor with A. microtis, a species with a wide host and geographic range, having been reported from both Microtus oeconomus (Pallas, 1776) and M. agrestis (Linnaeus, 1761) in the Palearctic. Previous studies have shown that the genetic distance for Arostrilepis tapeworms recognized as distinct species based on Cytb locus ranges from 4 to 15% (Makarikov et al., Reference Makarikov, Galbreath and Hoberg2013). Arostrilepis batsaikhani n. sp. can be recognized as distinct from A. microtis by a genetic distance value of 4.1% and the following morphological characters: having larger testes, smaller cirrus sac, cylindrical cirrus and larger cirrus spines (see Table 2).
Table 2. Species of Arostrilepis and their morphological characters (measurements in micrometres except where otherwise indicated)
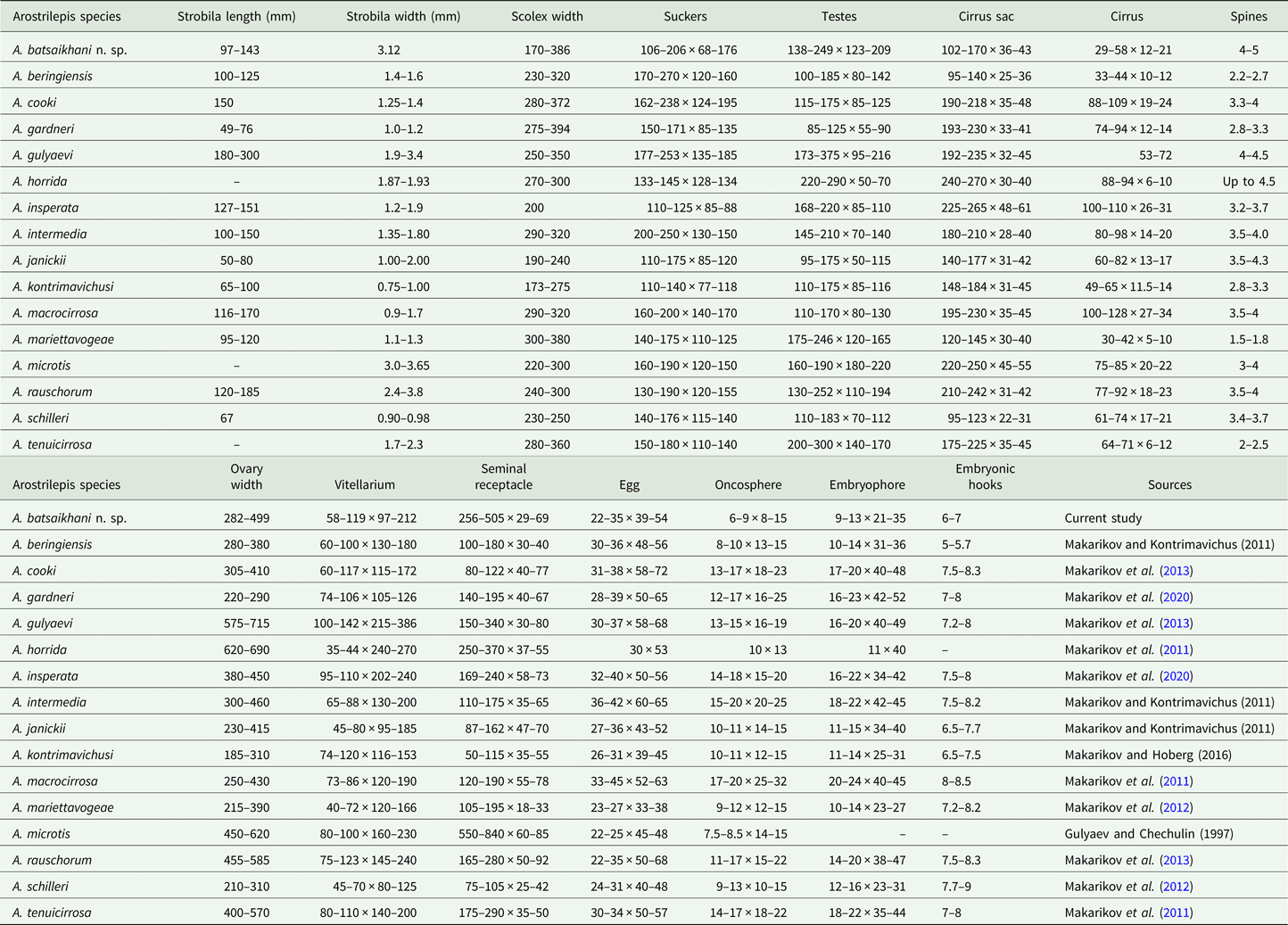
Table shows character length by width for the summary of each measurement.
Further, A. batsaikhani can be recognized as distinct from other species in the genus by the following morphological characters: the arrangement of testes for A. batsaikhani is strictly linear, which distinguishes A. batsaikhani from all described species of Arostrilepis known up to the present time except A. microtis and A. rauschorum. Further, A. batsaikhani can be differentiated from A. rauschorum by having a smaller cirrus sac, a smaller cirrus and larger cirrus spines.
Additional considerations of Arostrilepis evolution
To understand the degree of genetic differentiation of A. batsaikhani relative to the other species in the genus, a genetic distance analysis was conducted using cytochrome-b gene sequence data. Table 1 shows the genetic affinities of A. batsaikhani to the rest of the species in the genus for which data on cyt-b were available. Finally, combining both morphology and available molecular cytochrome-b data for all known species enabled us to create a phylogeny to examine the placement of A. batsaikhani relative to the rest of the described species of this genus, see Fig. 3. Data relative to this tree included, for 17 species, 516 base pairs of cytochrome-b DNA, 35 quantitative characters and 18 qualitative characters that were concatenated and run as a single input file to TNT. Our analysis produced a single most parsimonious tree with a consistency index of 56% and retention index of 40%. Our tree (Fig. 3) shows that A. batsaikhani n. sp. is well-embedded within the in-group, with A. microtis as its sister species.
Discussion
The current study describes the 16th species in the genus Arostrilepis which includes a new host record and raises the number of species of known rodent hosts to 28 (see Table 3). The first record of any species of Arostrilepis reported from a subterranean rodent was A. schilleri from Thomomys bulbivorus (Richardson, 1829) from south-east of Corvallis, Oregon by Gardner (Reference Gardner1985), who did not recognize that the species was new at the time (see the correction of this error in Makarikov et al., Reference Makarikov, Gardner and Hoberg2012).
Table 3. Species of Arostrilepis and their hosts with zoogeographic regions of occurrence

Up to this point in time, no other species of Arostrilepis have been reported from subterranean hosts in the Palearctic; however, we expect that additional sampling of subterranean rodents throughout their ranges will reveal greater diversity of parasites in the future. Finally, there are many Arostrilepis samples in museums that remain unidentified and work on these cestodes would serve well to expand the evolutionary scope and future investigations into this interesting group of parasites that would require collecting and analysing the data already existing in museums (Brooks et al., Reference Brooks, Hoberg, Gardner, Boeger, Galbreath, Herczeg, Mejía-Madrid, Racz and Dursahinhan2014).
Supplementary material
The supplementary material for this article can be found at https://doi.org/10.1017/S0031182022000294.
Acknowledgements
We thank all Mongolian Vertebrate Parasite Project (2009–2012) crew members who helped us collect and process samples from Mongolia. We are thankful to Zeiss USA for the continued support of the Manter Laboratory. In addition, permits for work in the countryside of Mongolia were provided by the Ministry of Environment and Tourism of Mongolia, the State Professional Inspections Agency and the Mongolian customs agency. We thank N. Batsaikhan without whom these studies could not have been carried out and were first planned driving through the Gobi in 1999 and implemented through 2009–2012.
Author contributions
S. L. G. conceived the study of parasite biodiversity in Mongolia. S. L. G., D. R. B. and A. T. D. contributed to the field study by collection of the vertebrates and their parasites. S. B. C. collated and manipulated the data. A. T. D. and S. L. G. wrote the article with assistance from D. R. B. and S. B. C.
Financial support
The current research was made possible through the support of the US National Science Foundation via grants DEB-0717214, DBI-0646356, DBI-9631295, DBI-9411976, DBI-1458139 and DBI-1756397 to S. L. G.
Conflict of interest
None.
Ethical standards
Collecting specimens of mammals was done in accordance with the Animal Care and Use protocol (07-06-028D) at the University of Nebraska-Lincoln, USA.