Breast cancer (BC) and ovarian cancer (OC) are the first and eighth most common cancers in women in Canada, respectively, with 26,316 and 2,786 cases annually (1). In approximately 12–15 percent of patients with epithelial ovarian cancer (EOC) (Reference Kotsopoulos, Rosen and Fan2–Reference Zhang, Royer and Li6) and 1–5 percent of patients with BC (7;8) the underlying cause of their cancer is a germline mutation in either the BRCA1 or BRCA2 (collectively termed “BRCA”) genes. Identification of a patient's BRCA status can inform treatment strategies; for example, polyadenosine diphosphate-ribose polymerase (PARP) inhibitors have demonstrated efficacy in patients with EOC with a BRCA mutation (Reference Hoskins and Gotlieb9). Establishing the patient's BRCA status is also beneficial to their immediate (i.e., first degree) family who have a 50 percent chance of carrying that BRCA mutation, which results in a lifetime risk of EOC of 35–60 percent if BRCA1 positive or 12–25 percent if BRCA2 positive, and a risk to age 70 years of BC of 65 percent if BRCA1 positive and 45 percent if BRCA2 positive (10;11). Family members may therefore wish to know their BRCA status and subsequent risk of cancer, allowing them to take steps to reduce this risk. This typically involves enhanced cancer surveillance or risk-reducing surgery (RRS), that is, risk-reducing bilateral salpingo-oophorectomy (RRBSO) and/or risk-reducing mastectomy (RRM).
BRCA testing has been available to family members of those affected by BC and OC in Canada for approximately 20 years at no cost to patients. Despite the benefits which testing offers, uptake remains low, with fewer than 20 percent of those diagnosed with EOC on a population basis in Ontario receiving a referral for testing and counseling (12;13). This is likely due to four main reasons: (1) constraints on testing/counseling, especially geographic and manpower related; (2) the physician does not refer their patient; (3) patient lack of knowledge/awareness; and (4) the patient has fears about the consequences of a positive test to themselves and their family (Reference Hoskins and Gotlieb9).
Improving access to and uptake of BRCA testing with subsequent surgical prevention has the potential to both improve outcomes for patients and reduce healthcare system costs. The objective of this study was consequently to evaluate from a Canadian perspective the cost effectiveness of prevention of EOC and BC via RRS in germline BRCA positive family members of index EOC and BC patients with a BRCA mutation, compared to treatment if cancer developed. This study is based on a previous United Kingdom (UK) economic model which considered index EOC cases only (Reference Eccleston, Bentley and Dyer14).
Methods
Model Overview
A patient-level simulation using an annual model cycle was developed in Microsoft Excel® based on a previous model which considered BRCA testing for patients with EOC only from a UK perspective (Reference Eccleston, Bentley and Dyer14). Full details of the model structure have been described previously (Reference Eccleston, Bentley and Dyer14) and an overview of the model structure is provided in Figure 1. Briefly, a simulated population of adult patients with EOC or BC (index population) and their family members transition to one of four health states at the beginning of each cycle: no cancer, EOC, BC, or EOC plus BC. The risk of cancer was dependent on whether the family member underwent RRBSO, RRM, or both. The model used a Canadian healthcare perspective with a 50-year time horizon and 1.5 percent discounting (15), and compared BRCA testing and RRS with no testing and treatment upon development of cancer. The model outputs were costs and quality-adjusted life-years (QALYs), resulting in an incremental cost-effectiveness ratio (ICER) for BRCA testing versus no testing, along with the number of cancer cases and deaths avoided.

Fig. 1. Model schematic.
The index population consisted of 2,786 patients with EOC and 26,316 patients with BC, based on the incidence of these cancers in Canada (1). All index patients were tested for BRCA mutations with only those with the BRCA mutation present entering the model, although the cost of testing patients without a mutation was included. Based on the published data, it was assumed that the BRCA mutation was present in 12.46 percent of patients with EOC [61.58 percent with BRCA1 and 38.42 percent with BRCA2 (Reference Kotsopoulos, Rosen and Fan2)] and 4.70 percent of patients with BC (Reference Malone, Daling and Doody7) [51.06 percent with BRCA1 and 48.94 percent with BRCA2 (16)]. If the index patient was BRCA positive their first-degree relatives were tested (overall risk of positive test: 50 percent) followed by second-degree female relatives in the event of the first-degree relative testing positive (overall risk of positive test: 25 percent) (Reference Petrucelli, Daly and Pal17). The age of simulated relatives was calculated in relation to the age of the index patient.
Mortality within the model was estimated for each individual using Canadian life tables (18) with an annual age-adjusted risk of death applied for patients with cancer (19). In the base-case, rates for RRS were based on a real-world study where 44 percent and 21 percent of patients underwent RRBSO and RRM, respectively (20;21). Index patients with BC were assumed to have received a therapeutic RRM as part of their cancer treatment and received RRBSO if they survived the first year of BC. Index patients with EOC were assumed to have received debulking surgery as part of their cancer treatment (and did not therefore require further RRBSO) but did not receive RRM as EOC is typically diagnosed at a late stage and has poor survival, resulting in a very low risk of future BC (22;23). Index patients with BC therefore have a reduced risk of future EOC as a result of RRS, whereas index patients with EOC have no change in risk of BC.
The age at which RRBSO occurred was assumed to be 54 years (Reference McAlpine, Hanley and Woo20), with RRM occurring at age 44 years (Reference Metcalfe, Ghadirian and Rosen21). If the individual was above this age at model entry, surgery was assumed to occur at this point. Surgery costs and impact on quality of life (QoL) were applied in the year in which surgery took place. In the event of an individual developing cancer, treatment costs commenced and a risk for secondary EOC or BC was applied; in the event of a secondary cancer, a new probability of mortality was applied. Structural uncertainty within the model was addressed via standard modeling approaches and clinical validation of the patient pathway.
Data Sources
The majority of data within the model were Canadian, with data from comparable countries used where Canadian data were not available. A summary of the population data is presented in Table 1. Data from Statistics Canada (24) were used to estimate the mean number of relatives for the index patient, with a 50.41 percent probability of relatives being female (25). It should be noted that the proportion of females in the generated cohort is slightly higher than this because the probability of the index patient's mother still being alive is higher than that for the father due to differences in life expectancy between sexes. Relatives were assigned an age using a normal distribution and age relative to the index patient; based on this age, the relative was determined to be alive or dead using life tables and random numbers.
Table 1. Model cohort summary
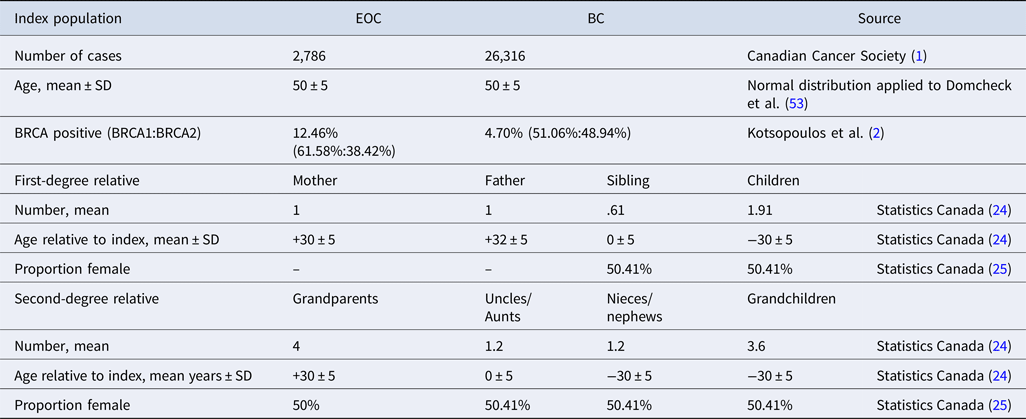
BC, breast cancer; EOC, epithelial ovarian cancer; SD, standard deviation.
Risk of cancer was determined based on age and BRCA mutation type (Supplementary Tables 1 and 2), with the reduction in risk of EOC and BC following RRBSO and RRM determined based on a structured literature review and fixed-effects meta-analysis of eight relevant publications (Supplementary Table 3) (Reference Domchek, Friebel and Neuhausen26–Reference Rebbeck, Lynch and Neuhausen33). Risk reduction following both RRBSO and RRM was considered in only one publication (Reference Rebbeck, Friebel and Lynch32) and no evidence was identified which demonstrated that RRM affected EOC rates. It was therefore assumed that the risk reduction for EOC in patients undergoing both RRBSO and RRM was equivalent to the risk reduction for RRBSO. Cancer-related mortality was estimated using data from the United States National Cancer Institute as Canadian data were not available (Supplementary Table 4) (19).
Costs
The model included costs associated with BRCA testing (estimate), genetic counseling (estimate), cancer treatment [Duong et al. (Reference Duong, Wright and Yin34) for EOC and Pataky et al. (Reference Pataky, Armstrong and Chia35) for BC], cost of RRM and RRBSO were retrieved from two different cost-effectiveness studies in British Columbia, Canada (36;37) and palliative care [Hollander et al. (Reference Hollander38) for EOC and Pataky et al. (Reference Pataky, Armstrong and Chia35) for BC] (Supplementary Table 5). All costs are reported in Canadian dollars (CAD) and U.S. dollars (USD) in year 2016. Where applicable, costs were inflated using Bank of Canada inflation rate to inflate costs to 2016 (39) and Canadian costs were converted to USD using Bank of Canada historical exchange rates (40).
As the age at which RRBSO took place was assumed to be 54 years, costs for hormone replacement therapy were not included in the model. Genetic counseling consisted of one post-test session for index patients with a positive test, one pre-test session for all relatives, and one post-test session for all relatives with a positive test. BRCA test, genetic counseling, and RRS costs were applied in the cycle in which they occurred.
Cancer treatment costs were based on previously published cost-effectiveness evaluations from a Canadian perspective for EOC (Reference Duong, Wright and Yin34) and BC (Reference Pataky, Armstrong and Chia35). OC costs were applied annually due to the short life expectancy of patients and the high likelihood of repeat treatments. BC costs were applied for 1 year during the cycle in which they occurred due to higher survival rates in these patients compared with EOC. No further surgery was assumed to be required in patients who had received RRM if they developed BC, whereas additional debulking surgery was assumed to be required in patients who had received RRBSO and developed EOC. Palliative care costs were applied in the year in which the patient died.
Health State Utilities
Utilities for patients diagnosed with EOC or BC were sourced from the UK National Institute for Health and Care Excellence familial BC guideline (41) (Supplementary Table 6) as this provided a more complete data set than was available using Canadian sources. Disease specific utilities were applied to age-specific utilities for women in Canada (42). If a patient developed both EOC and BC, the utilities for these were applied multiplicatively.
Utilities for other health states and treatments were sourced from a time trade-off study in BRCA positive individuals by Grann et al. (Reference Grann, Patel and Jacobson43) which reported a short-term negative impact on QoL for those undergoing RRS, which was applied in the cycle in which RRS occurred within the model. The base-case assumed no disutility for a positive BRCA test.
Sensitivity Analyses
Sensitivity analyses were conducted to assess uncertainty around key model inputs by varying these over a plausible range, based either on the 95 percent confidence interval or clinical opinion. If no estimates were available a variation of ±25 percent was applied (capped at 0 and 100 percent). Probabilistic sensitivity analysis (PSA) was also conducted to assess the impact of varying all parameters simultaneously.
Base-Case Assumptions
The following base-case settings were used in the model:
• All index patients are tested for BRCA status in the “test” arm, and no patients are tested in the “no test” arm.
• The BRCA mutation was assumed to be present in 12.46 percent of patients with EOC (Reference Kotsopoulos, Rosen and Fan2) and 4.70 percent of patients with BC (Reference Malone, Daling and Doody7).
• All BRCA positive female family members of index patients receive RRS.
• The age at which RRBSO occurred was assumed to be 54 years (Reference McAlpine, Hanley and Woo20), with RRM occurring at age 44 years (Reference Metcalfe, Ghadirian and Rosen21).
Other Assumptions
The following assumptions were applied within the model:
• The sensitivity and specificity of BRCA testing was assumed to be 100 percent based on clinical expert opinion.
• Relatives of the index case with a positive test were assumed to have the same BRCA mutation.
• The model population was assumed not to have any previous history of EOC, BC, or RRS.
• Cancer risks were assumed to remain constant over the period in which they applied.
• All RRMs were assumed to be bilateral due to the greater availability of data for this approach in the literature.
• It was assumed that EOC and BC would not develop in the same year to simplify the model; whereas this may occur in clinical practice, it is highly unusual.
• There was no difference in outcomes or costs for patients without a BRCA mutation in either arm, other than the cost of the BRCA test.
• Relatives born after the index case was tested were not considered in the model as it would have been impractically complex to do so.
• The model is not a standard cancer care model and considered only average costs and utilities for cancer, rather than considering specific treatments and cancer severities. This was deemed appropriate as the model was designed to show the impact of BRCA testing, rather than examine cancer care methodologies.
Results
Base-Case
The total population within the model was 36,290; 29,102 index cases, 6,136 first-degree relatives, and 1,052 second-degree relatives. Of these, 1,668 index cases, 1,593 first-degree relatives, and 275 second-degree relatives had a BRCA mutation. Uptake rates for RRBSO and RRM in current clinical practice in Canada were used in the base-case (Table 2). In this analysis, 44 percent of BRCA positive women underwent RRBSO at age 54 years and 21 percent of BRCA positive women underwent RRM at age 44 years (20;21), resulting in 127 fewer EOC cases, 104 fewer BC cases, and 21 fewer deaths. These outcomes were achieved at an additional cost of CAD 11,777,696 (USD 8,319,986) with a QALY gain of 788, resulting in an ICER of CAD 14,942 (USD 10,555) per QALY gained, which is cost effective at the commonly accepted Canadian willingness to pay threshold of CAD or USD 100,000 per QALY gained.
Table 2. Base-case clinical results and costs

BC, breast cancer; EOC, epithelial ovarian cancer; ICER, incremental cost-effectiveness ratio; QALY, quality-adjusted life-year; RRS, risk-reducing surgery.
Scenario Analysis: RRS Uptake Rates/Age at Uptake
Although BRCA testing and RRS was cost effective in the base-case based on current RRS rates in Canada, scenarios were considered to evaluate the impact of changes in RSS uptake rates and age at RRBSO uptake (Table 3). Increasing RRS uptake rates by 25–100 percent resulted in greater cost effectiveness, and testing dominated no testing (less expensive and more effective) in an “ideal” scenario where RRS rates were assumed to be 100 percent; however, this is not likely to be achievable in the real world. Scenarios were therefore considered to determine realistic goals for changes in current clinical practice to make testing both cost effective and cost saving; these determined that a 50 percent increase in RRS uptake rates (RRBSO 66 percent and RRM 32 percent) combined with a mean age at RRBSO of 50 years would result in lower incremental costs of CAD 3.4M (or USD 2.6M) compared to the base-case, whereas only a 25 percent increase in RRS uptake rates would be required (RRBSO 55 percent and RRM 26 percent) for cost savings if RRBSO were performed at a mean age of 40 resulted in a cost saving of CAD 1.7M.
Table 3. Results of the one-way scenario sensitivity analyses

BC, breast cancer; EOC, epithelial ovarian cancer; ICER, incremental cost-effectiveness ratio; QALY, quality-adjusted life-year; RRM, risk-reducing mastectomy; RRBSO, risk-reducing bilateral salpingo-oophorectomy.
Additional Sensitivity Analysis
In all scenario analyses considered testing remained a cost-effective strategy at the commonly accepted North American threshold of approximately USD or CAD 100,000 per QALY gained (Table 3). The cost effectiveness of testing improved as the age at which RRS occurred was reduced and as costs for cancer treatment increased. Testing remained cost effective versus no testing when index patients with EOC only (no BC) or index patients with BC only (no EOC) were considered in the model. A “real- world” scenario was also considered for BRCA testing in which 30 percent of index patients were assumed to receive a BRCA test and all BRCA positive female relatives selected for RRS. The resulting ICER suggested that testing remained cost effective (CAD 17,848 or USD 13,521). It should be noted that the model generates a unique population distribution when varying population (as per the 30 percent testing scenario); whereas it would be expected that the ICER would remain consistent with the base-case if a 30 percent reduction was applied to a static population, there is consequently variation in the ICER due to this factor.
Probabilistic Sensitivity Analysis
The inputs for PSA are provided in Supplementary Table 7. In the PSA (4,000 simulations), all simulations were in the north-east or south-east quadrant of the cost-effectiveness plane (Supplementary Figure 1), indicating that testing was always more effective than no testing. The probability that testing was cost effective versus no testing was 96.45 percent at CAD 50,000 per QALY gained (or 98.15 percent based on a willingness-to-pay of USD 50,000 per QALY gained) (Supplementary Figure 2).
Budget Impact
Testing followed by RRS inevitably results in a substantial up-front cost, which was CAD 31,571,567 (USD 22,341,644) in year 1 (Supplementary Table 8). However, in subsequent years there is then a cost saving due to fewer cases of EOC and BC which ranged from CAD 137,569 (USD 98,766) in year 2 to CAD 312,113 (USD 210,812) in year 4. Although a longer time period was not modeled, cost savings would continue to occur in all years after this period.
Discussion
This Canadian analysis demonstrates that current genetic testing of patients with EOC or BC and their family members for BRCA mutations followed by RRS prevents cancer cases and deaths, at an incremental cost which is considered cost effective (an ICER < CAD or USD 100,000 per QALY). However, scenario analysis demonstrates that the cost effectiveness of testing can be improved by increasing RRS uptake rates and performing RRS at an earlier age, with a 50 percent increase in RRS rates and age of RRBSO of 50 years or a 25 percent increase in RRS rates and age of RRBSO of 40 years resulting in cost savings over the long term. The model consequently provides an important argument for improving access to and uptake of genetic testing and RRS.
The number of EOC and BC cases and deaths avoided in BRCA positive family members who undergo RRS may initially appear low given that the risk reduction following surgery is 84–88 percent for EOC and 95 percent for BC. This is primarily due to the age at which RRS takes place, which was 54 years for RRBSO and 44 years for RRM, as there is no risk reduction for family members prior to this point. Reducing the age at which RRBSO and RRM took place to 40 years in sensitivity analysis increased the number of cancers and deaths avoided and improved the cost effectiveness of testing. The number of deaths avoided may also appear low as all-cause mortality was considered, rather than only cancer mortality. Women in both arms consequently have a risk of age-related mortality which may result in the number of lives saved due to avoiding cancers being understated in the results.
The results of the current model are consistent with the UK analysis on which it is based (Reference Eccleston, Bentley and Dyer14), which reported an ICER of £4,339 per QALY for testing versus no testing using the real-world RRS uptake rates considered in scenario analysis in the current model. The current model differs from the UK analysis in that it considers a Canadian perspective and includes patients with both EOC and BC in the index population, compared with only EOC in the UK analysis. Certain model inputs are used in both the current model and the UK model; however, Canadian data were used where available. This results in differences in outcomes between the models, as the cost of cancer treatment (without surgery) is lower in the UK model than in the Canadian model. Differences in cancer treatment costs were considered in sensitivity analysis.
Another key difference in the current model results from the inclusion of both EOC and BC index patients. Although EOC is typically diagnosed at a late stage and has poor survival (resulting in a very low risk of future BC), the greater survival rates for index patients with BC, compared with EOC, made it appropriate to model how RRBSO might affect the development of future EOC in these patients. Index patients with BC with a BRCA mutation were therefore assumed to receive both RRBSO and RRM, with a subsequent reduction in their risk of future cancer. In contrast, the UK model considered only index patients with EOC and assumed RRM was not performed in these patients. This resulted in 63 of the 1,237 index patients with BC avoiding future EOC as a result of RRBSO, with one death avoided. The number of deaths avoided is lower than might be expected given the high mortality in EOC due to the fact that index patients with BC who later developed EOC were likely to do so in their later years, where the risk of all-cause mortality is higher than in younger patients.
Three other previous analyses of the economic implications of BRCA testing and RRS have also been published. Kwon et al. (Reference Kwon, Daniels and Sun44) evaluated the cost effectiveness of testing different categories of patients with EOC and their first-degree relatives, and concluded that testing was cost effective in women with EOC or a history of BC, a family history of EOC/BC, or of Ashkenazi Jewish descent, with an ICER of CAD 32,018 per life-year gained. This study has several notable differences from the current model; only patients with a personal or family history of cancer or Ashkenazi Jewish ancestry were tested, and the traditional genetics referral model was used for index patients. This is a lengthier and more resource-intensive pathway for testing and referral compared with that used in the current model, which assumes that testing and referral for counseling is carried out during regularly scheduled treatment visits. A second study by Manchanda et al. evaluated the cost effectiveness of BRCA testing and RRS in women of Ashkenazi Jewish descent from a UK perspective (Reference Manchanda, Legood and Burnell45).
The population in this study is consequently not directly comparable with that in the current model, due to its higher rate of BRCA mutations. Despite this, Manchanda et al. also concluded that BRCA testing was highly cost effective. The third study, by Tuffaha et al. (Reference Tuffaha, Mitchell and Ward46), evaluated the cost effectiveness of BRCA testing for women with BC with a ≥10 percent pretest BRCA mutation probability, followed by testing of first- and second-degree relatives and RRS. Testing was also cost effective in this analysis, with an ICER of AU$18,900 versus no testing; the primary difference between this publication and the current study was the testing of only index patients with BC (vs. both BC and EOC in the current study).
As with all economic models, the current model has limitations in both design and data inputs. First, the current model does not attempt to consider cancer severity, differences in costs and benefits for cancer treatment, or overall and progression-free survival. These would typically be included in cost-utility analyses for cancer treatment; however, their exclusion from the current model was a necessary simplification to allow a comparison of only the impact of BRCA testing and RRS versus no testing using average cancer survival rates and treatment. The exclusion of these items also resulted in two necessary assumptions: enhanced surveillance of BRCA positive family members as an alternative to surgery could not be considered, and there was assumed to be no benefit to index EOC patients from BRCA testing. The assumption of no benefit to BRCA testing is a conservative assumption, as knowledge of an index patient's BRCA status can benefit them in several ways which are not considered in the model. BRCA status can inform treatment choices in patients with recurrent disease (47;48) and BRCA positive index patients would be able to access PARP inhibitors in clinical practice, which have been shown to have a demonstrated benefit in EOC (Reference Ledermann, Harter and Gourley49–Reference Ledermann, Harter and Gourley51) and BC (50;52;53).
Second, RRS was assumed to have no morbidity and mortality. Although this may bias the analysis in favor of testing, rates of morbidity and mortality are generally low for patients undergoing RRS (Reference Kauff, Satagopan and Robson29).
Third, the mortality rates for EOC were based on a structured literature review and fixed-effects meta-analysis of eight relevant publications (Reference Domchek, Friebel and Neuhausen26–Reference Rebbeck, Lynch and Neuhausen33). This provided a 5-year survival rate of 76.5 percent at age <45 years, 59.1 percent at 45–54 years, 49.5 percent at 55–64 years, 38.7 percent at 65–74 years, and 20.9 percent at ≥75 years. Although the rates for patients aged <45 years are credible, in clinical practice BRCA positivity is seen mainly in high grade serous patients who have worse outcomes; lower survival rates would therefore be expected for all other ages. However, this is a conservative assumption and any reduction in survival rates would make testing more cost effective.
Fourth, the utilities associated with EOC and BC by time from diagnosis within the model were not available for Canada and were instead taken from a UK source (41). It is possible that these utilities would be different in a Canadian population than in the UK; however, given the similarities in healthcare systems and culture between these nations it is unlikely that there would be substantial differences. The increase in utility over time in the EOC population was an assumption based on expert guidance from a panel of clinicians and patients; however, it should be considered that such an increase may be unlikely in clinical practice as EOC progresses and the chance of treatment benefit falls. Decreasing these utility values would make testing more cost effective relative to no testing.
Finally, the current model is not able to vary BRCA testing rates within the testing and no testing arms for index patients or family members, for example, to compare a scenario where 80 percent of family members receive testing versus one where 30 percent of patients/family members receive testing. It therefore cannot compare an “ideal” testing scenario versus testing rates based on current guidelines/clinical practice. An attempt was made to consider this in scenario analysis by examining an index population of 30 percent of the base-case (ICER of CAD 17,848 or USD 13,521). Future adaptations of this model may wish to consider this to allow comparisons between real-world BRCA testing rates and scenarios where these rates are increased. In addition, the conclusion of greater cost effectiveness with higher RRS uptake rates suggests that it would be interesting to examine the cost effectiveness of health promotion activities which could increase the uptake of both testing and RRS. Although the current model has limitations, these generally result in a bias against testing. The model results consequently provide both a humanistic and economic rationale for expanding access to and uptake of BRCA testing and RRS.
Conclusion
In the base-case analysis using real-world RRS uptake rates, testing and RRS was cost effective versus no testing and treatment on cancer development, with 127 and 104 fewer cases of EOC and BC, respectively, and twenty-one fewer all-cause deaths. Testing remained cost effective versus no testing at the commonly accepted North American threshold of approximately CAD or USD 100,000 per QALY gained in all scenario analyses; however, these analyses demonstrate that current RRS uptake rates may be suboptimal, as cost effectiveness improved as uptake rates increased.
Supplementary material
The supplementary material for this article can be found at https://doi.org/10.1017/S0266462319003519.
Acknowledgments
This study was funded by AstraZeneca Canada.
Conflict of interest
The authors have nothing to disclose.