Introduction
Schizophrenia (SCZ) is a severe mental disorder affecting 0.25–0.28% of the population (McCutcheon, Marques, & Howes, Reference McCutcheon, Marques and Howes2020), and it contributes to 1.4–1.7% of the economic burden of all diseases (Charlson et al., Reference Charlson, Ferrari, Santomauro, Diminic, Stockings, Scott and Whiteford2018; GBD, 2017 Disease and Injury Incidence and Prevalence Collaborators, 2018). Among the different psychopathologies in SCZ, the symptoms of anhedonia and amotivation have been reported to impair SCZ patients' social functioning, and increase societal burden of the disease (Nordstroem, Talbot, Bernasconi, Berardo, & Lalonde, Reference Nordstroem, Talbot, Bernasconi, Berardo and Lalonde2017; Provencher & Mueser, Reference Provencher and Mueser1997). Anhedonia refers to the diminished ability to experience pleasure (Gard, Kring, Gard, Horan, & Green, Reference Gard, Kring, Gard, Horan and Green2007); amotivation refers to the diminished ability to motivate effortful behavior (Fervaha, Foussias, Agid, & Remington, Reference Fervaha, Foussias, Agid and Remington2013). The putative neurobiological mechanisms for these symptoms have remained elusive, hindering developments of effective interventions targeting at anhedonia and amotivation (Chan, Wang, & Lui, Reference Chan, Wang and Lui2022; van Os & Kapur, Reference van Os and Kapur2009).
One of the putative neurobiological mechanisms for anhedonia and amotivation symptoms in SCZ is impaired reward functioning (Chan et al., Reference Chan, Wang and Lui2022; Gold, Waltz, Prentice, Morris, & Heerey, Reference Gold, Waltz, Prentice, Morris and Heerey2008; Strauss, Waltz, & Gold, Reference Strauss, Waltz and Gold2014; Walter, Kammerer, Frasch, Spitzer, & Abler, 2009). Reward processing involves multiple neuropsychological components (Berridge & Robinson, Reference Berridge and Robinson2003; Berridge, Robinson, & Aldridge, Reference Berridge, Robinson and Aldridge2009), which are discrete yet all supporting an overarching function. Notably, Kring and Barch proposed one of the influential models (Kring & Barch, Reference Kring and Barch2014), which has ‘compartmentalized’ the impaired reward processing in SCZ patients into several neuropsychological components, which include (1) reward anticipation (i.e. the ability to anticipate pleasure supports the formation of ‘incentive salience’ for upcoming reward) (Chan et al., Reference Chan, Wang, Huang, Shi, Wang, Hong and Kring2010; Gard et al., Reference Gard, Kring, Gard, Horan and Green2007), (2) reward consumption (i.e. the ability to experience consummatory pleasure at the receipt of the reward) (Chan et al., Reference Chan, Wang, Huang, Shi, Wang, Hong and Kring2010; Gard et al., Reference Gard, Kring, Gard, Horan and Green2007), (3) reward learning (i.e. the ability to maintain and update the inner representation of rewards) (Barch & Dowd, Reference Barch and Dowd2010; Gold et al., Reference Gold, Waltz, Prentice, Morris and Heerey2008; Kring & Elis, Reference Kring and Elis2013), and (4) effort computation (i.e. the decision-making process of computing the likely reward and required effort which determines an individual's levels of willing for effort expenditure) (Barch, Treadway, & Schoen, Reference Barch, Treadway and Schoen2014; Culbreth, Moran, & Barch, Reference Culbreth, Moran and Barch2018; Treadway, Buckholtz, Schwartzman, Lambert, & Zald, Reference Treadway, Buckholtz, Schwartzman, Lambert and Zald2009; Huang et al., Reference Huang, Yang, Lan, Zhu, Liu, Wang and Chan2016). These four components proposed by Kring and Barch constitute the ‘positive valence systems domain’ in the Research Domain Criteria (RDoC) framework (Barch, Pagliaccio, & Luking, Reference Barch, Pagliaccio, Luking, Simpson and Balsam2016; Cuthbert, Reference Cuthbert2014; Insel et al., Reference Insel, Cuthbert, Garvey, Heinssen, Pine, Quinn and Wang2010). The positive valence systems are believed to interact with other SCZ-associated dysfunctions, such as low pleasure beliefs and memory impairments, and emotion processing problems, resulting in anhedonia and amotivation (Chan et al., Reference Chan, Wang and Lui2022; Strauss & Gold, Reference Strauss and Gold2012).
Neuroimaging research could unveil component-specific neural mechanisms for anhedonia and amotivation. For instance, an fMRI study based on monetary incentive delay (MID) task found decreased activation in the anterior cingulate cortex (ACC), midbrain, thalamus, cerebellum, and striatum during reward anticipation (Nielsen et al., Reference Nielsen, Rostrup, Wulff, Bak, Lublin, Kapur and Glenthøj2012). Another fMRI study found consummatory activation to pleasant stimuli was reduced in the dorsolateral, medial, and ventrolateral prefrontal cortices (Ursu et al., Reference Ursu, Kring, Gard, Minzenberg, Yoon, Ragland and Carter2011). One fMRI study found that SCZ patient exhibited abnormal activation in the caudate, posterior cingulate cortex (PCC), and dorsal medial cortex gyrus during effort computation (Huang et al., Reference Huang, Yang, Lan, Zhu, Liu, Wang and Chan2016). Regarding reward learning, previous research reported reduced activation of the medial temporal lobe, hippocampus gyrus, striatum, and PCC in SCZ (Reinen et al., Reference Reinen, Van Snellenberg, Horga, Abi-Dargham, Daw and Shohamy2016).
Despite these fruitful findings, there are knowledge gaps regarding the putative neural mechanisms contributing to impaired reward processing in SCZ. Although these four components are presumably distinct at the neuropsychological level (Kring & Barch, Reference Kring and Barch2014), they may share overlapping mechanisms at the neural level, such as abnormal dopaminergic transmission (Balleine, Reference Balleine2005; Berridge & Robinson, Reference Berridge and Robinson1998; Samson, Frank, & Fellous, Reference Samson, Frank and Fellous2010). Therefore, it is essential to investigate neural mechanisms that are specific to each reward component as well as those that are shared between them to better understand anhedonia and amotivation. However, many experimental paradigms can only tap into a limited number of components, thus posing difficulty for any direct comparison between neural mechanisms for different components (Kieslich, Valton, & Roiser, Reference Kieslich, Valton and Roiser2022). One way to address this issue is to tease out components from experimental paradigms and then employ meta-analysis to synthesize component-based variables. Following this approach, several previous studies have adopted neuroimaging meta-analysis on reward dysfunction in SCZ (Chase, Loriemi, Wensing, Eickhoff, & Nickl-Jockschat, Reference Chase, Loriemi, Wensing, Eickhoff and Nickl-Jockschat2018; Leroy et al., Reference Leroy, Amad, D'Hondt, Pins, Jaafari, Thomas and Jardri2020; Radua et al., Reference Radua, Schmidt, Borgwardt, Heinz, Schlagenhauf, McGuire and Fusar-Poli2015; Zeng et al., Reference Zeng, Yan, Cao, Su, Song, Luo and Yang2022), but they mainly focused on the components of reward anticipation and consumption, and largely ignored the component of reward learning and effort computation.
Another limitation of previous studies is the restricted selection of regions of interest (ROIs). Reward function is mediated via the limbic cortico-striatal-thalamic circuit that interdigitates with the mesolimbic dopamine pathway (Dichter, Damiano, & Allen, 2012; Haber & Knutson, Reference Haber and Knutson2010; Nestler & Carlezon, Reference Nestler and Carlezon2006). However, most existing studies have focused on only a few regions of the striatal-prefrontal network, such as the striatum and the orbitofrontal cortex (OFC), which might introduce bias when determining alterations of brain activation patterns and neural mechanisms. At the same time, evidence strongly suggested that SCZ is associated with alterations of the whole brain rather than localized regions (Kambeitz et al., Reference Kambeitz, Kambeitz-Ilankovic, Leucht, Wood, Davatzikos, Malchow and Koutsouleris2015; Venkataraman, Whitford, Westin, Golland, & Kubicki, Reference Venkataraman, Whitford, Westin, Golland and Kubicki2012). Therefore, it is necessary to investigate putative neural mechanisms for anhedonia and amotivation in SCZ patients from the ‘whole-brain perspective’.
To address the aforementioned knowledge gaps, this study adopted the ‘whole-brain perspective’ to systematically evaluate brain functional impairment patterns of SCZ-associated with reward processing, and to clarify the neural substrates for each of the component of impaired reward processing. Following the framework of symptom dimension (Keshavan & Ongur, Reference Keshavan and Ongur2014; Pacheco et al., Reference Pacheco, Garvey, Sarampote, Cohen, Murphy and Friedman-Hill2022) and the psychosis continuum model (van Os, Linscott, Myin-Germeys, Delespaul, & Krabbendam, Reference van Os, Linscott, Myin-Germeys, Delespaul and Krabbendam2009), studies recruiting clinical patients with SCZ spectrum disorders or subclinical samples at risk of developing SCZ were included in this meta-analysis, to identify brain functional impairments for the SCZ spectrum. We hypothesized that brain functional alterations related to impaired reward processing would be found mainly in but not limited to the striatal-prefrontal network. Meanwhile, for dysfunctions related to reward components, given their overlapping neural basis, certain brain regions may be responsible for impairments in multiple components, making them potential targets for interventions aimed at addressing anhedonia and amotivation symptoms.
Methods
Study search and selection
Following the PRISMA guidelines (Liberati et al., Reference Liberati, Altman, Tetzlaff, Mulrow, Gøtzsche, Ioannidis and Moher2009), a systematic literature search was conducted to identify relevant studies published as of 1 June 2022 on PubMed and Web of Science. The comprehensive search terms included (‘schizo*’) AND (reward* OR motivation* OR amotivation* OR pleasant* OR pleasur* OR hedoni* OR anhedoni*) AND (‘functional magnetic resonance imaging’ OR ‘fMRI’ OR ‘functional MRI’ OR ‘PET’ OR ‘positron emission tomography’). Additional studies were included in this meta-analysis based on the reference lists of four previous review papers (Barch et al., Reference Barch, Pagliaccio, Luking, Simpson and Balsam2016; Strauss et al., Reference Strauss, Waltz and Gold2014; Treadway & Zald, 2013; Whitton, Treadway, & Pizzagalli, Reference Whitton, Treadway and Pizzagalli2015).
All the obtained studies were screened by the Zotero 6.0, which identified and removed any duplicate study. Next, we manually identified relevant studies which fulfilled the following criteria for meta-analysis: (1) an original study published in a peer-reviewed journal, (2) adopting brain functional imaging task related to reward processing, (3) having samples of SCZ spectrum [SCZ or schizoaffective disorder patients diagnosed based on the Diagnostic and Statistical Manual of Mental Disorders (DSM) (Frances, First, & Pincus, Reference Frances, First and Pincus1995) or International Statistical Classification of Diseases and Related Health Problems (ICD) diagnostic criteria (World Health Organization, 1992), or high-risk subclinical cases identified by family genetic risk (e.g. first-degree relatives), mental states (e.g. at risk mental state), or specific trait (e.g. schizotypy)], (4) comparing brain activation between SCZ spectrum and HC, (5) employing the whole-brain analysis. Two authors (WX and ZYH) performed the selection of studies based on these criteria independently, and then jointly to arrive at consensus. The reasons for exclusion can be found in PRISMA flow chart (see Fig. 1).

Fig. 1. PRISMA flow-chart depicting the study inclusion and exclusion process. 1Not homogenous thresholding: levels of statistical significance were inconsistent across regions in whole-brain analyses (e.g. small volume correction). 2Not report activation/deactivation coordinates: no hypo- or hyper-activation of SCZ were reported, or no coordinate was reported.
Data extraction and coding
For each study included for meta-analysis, the following data were extracted, including (1) basic information of the article: title, authors, and publication year; (2) information about subjects: sample size, mean age, and sex ratio of SCZ spectrum group and healthy control (HC) group, and clinical stage (e.g. chronic or first-episode patients, and high-risk conditions), diagnostic tool, medication, illness duration (for SCZ samples only); (3) computational settings of fMRI activation analysis, including the experimental condition of interest (e.g. reward anticipation-neural anticipation) in first-level analysis, and statistical comparison setting (e.g. HC-SCZ or SCZ-HC) in the second-level analysis; (4) results of whole-brain analysis: the reference space, the significant threshold, and the coordinates, cluster sizes, statistical indicators (e.g. t-value or z-value) of the significant clusters.
For analyzing reward components, selected studies were classified into four groups based on the components investigated, according to the following criteria: (1) reward anticipation: the paradigm identified the anticipation or prediction process for the forthcoming reward; (2) reward consumption: the experiment focused on the receiving or experiencing process for positive stimuli; (3) reward learning: the experiment separated brain activation related to learning process based on unexpected reward acquisition or omission; and (4) effort computation: the experiment estimated the willingness to spend effort pursuing rewards. Because more than one psychological process could be derived from a single paradigm [e.g. MID task can separate the neuropsychological components of reward anticipation and consumption simultaneously (Knutson, Westdorp, Kaiser, & Hommer, Reference Knutson, Westdorp, Kaiser and Hommer2000)], an individual study can be classified into different groups at the same time.
Data were initially extracted by two authors (ZYH and NYZ), each undertook half of the data extraction work and checked on the other half. The extracted data were then further checked by another independent author (WX). The studies included for this meta-analysis are shown in Table 1 and Supplementary Table S2.
Table 1. Summary of studies included in the meta-analysis

SCZ, schizophrenia; CAARMS, Comprehensive Assessment of At-risk Mental States; CRSAS, Chapmen Revised Social Anhedonia Scale; SPS, Scale of Prodromal Symptoms; SPQ, Schizotypal Personality Questionnaire; ERIraos, Early Recognition Inventory based on IRAOS; SIPS, psychosis-risk syndrome; 1st, first antipsychotic medication; 2nd, second antipsychotic medicate.
Coordinate-based meta-analysis
Meta-analysis was performed using the Seed-based d Mapping with Permutation of Subject Images (SDM-PSI version 6.21). This method estimated the mean maps of random effect though synthesizing the peak coordinates and effect size (Hedges' g) of included studies with subject-based permutation test (Albajes-Eizagirre et al., Reference Albajes-Eizagirre, Solanes, Fullana, Ioannidis, Fusar-Poli, Torrent and Radua2019). Based on the extracted data, basic information of all related studies was entered into the SDM-PSI software for following analyses, including titles, demographic variables of SCZ subjects (i.e. diagnostic group, sample size, mean age, sex ratio, clinical symptoms, and duration), psychological tasks, reward components, and t-value threshold of statistical significance.
The merged and distinct dysfunctional patterns of the four reward components were respectively obtained through the analyses for all included studies and component groups. Both of them were performed following the SDM-PSI guidelines (Albajes-Eizagirre et al., Reference Albajes-Eizagirre, Solanes, Fullana, Ioannidis, Fusar-Poli, Torrent and Radua2019): Preprocessing was performed with default parameters (20 mm full width at half maximum, gray matter mask) to recreate the bounds of the effect-size. We then conducted mean analysis to estimate the mean difference of brain activation between SCZ spectrum and HC. The criteria of significance were set as uncorrected p < 0.0025 (paired one-tailed tests, resulting in two-tailed p < 0.005) and cluster wise k > 10 voxels, since it was found to be optimally balanced between type I and type II errors (Chavanne & Robinson, Reference Chavanne and Robinson2021; Lieberman & Cunningham, Reference Lieberman and Cunningham2009). We also performed family-wise error (FWE) corrections based on 5000 permutations. Finally, Egger's test was implemented for significant clusters to estimate potential publication bias (Egger, Davey Smith, Schneider, & Minder, Reference Egger, Davey Smith, Schneider and Minder1997). At the same time, considering the potential heterogeneity of subclinical groups, the same analyses were performed again only for studies recruiting clinical patients with SCZ spectrum disorders (see Supplementary Material for results).
We performed exploratory analyses to determine the common impaired regions for multiple reward components. The convergences between functional meta-analyses for each two components were analyzed through multi-model meta-analyses. Significance levels were set as corrected p < 0.05 with threshold-free cluster enhancement correction and cluster size >10.
In order to determine the moderating effects of potential factors, meta-regression analyses were performed for included studies between reward-related activations and demographic and clinical variables, including the mean age, the percentage of males, the duration of illness, and negative symptom severity (i.e. raw or conversional scores for PANSS negative symptoms rating) (van Erp et al., Reference van Erp, Preda, Nguyen, Faziola, Turner, Bustillo and Potkin2014). The significance level was set as p < 0.05 and cluster size >10.
Results
Studies included for meta-analyses
Thirty-seven studies met the eligibility criteria for coordinate-based analysis, comprising 810 SCZ spectrum subjects and 862 HCs. We classified studies based on the reward components of interest and found 16 studies related to reward anticipation, 11 studies related to reward consumption, 12 studies related to reward learning, and three studies related to effort computation. Because the number of studies on effort computation was insufficient for the coordinate-based meta-analysis, we only provided a descriptive review rather than quantitative analysis for effort computation.
Merging analysis for reward components
Coordinate-based meta-analysis for all included studies revealed merged dysfunction patterns of multiple reward components. SCZ spectrum subjects showed reduced activation in a wide range of cortical, subcortical, and cerebellar regions, mainly including the striatum, medial and lateral orbital frontal cortex, ACC, thalamus, cerebellar lobule IV/V and Crus I areas, inferior parietal gyri, and calcarine fissure. Findings in the striatum, orbital frontal cortex, and ACC remained significant even after FWE corrections. The Egger's test results did not suggest any publication bias for any peak (more details can be found in Table 2 and Fig. 2).

Fig. 2. Areas with abnormal activation of SCZ spectrum when reward components were merged. Color bars represent SDM-Z scores (negative number for hypo-activations of SCZ spectrum: SCZ spectrum < HC in task > baseline). Results were considered significant for p < 0.0025, and cluster extent >10 voxels.
Table 2. Results of merging analysis for reward components in SCZ spectrum

SDM, Seed-based D Mapping; MNI, Montreal Neurological Institute; FWE, family-wise error.
Meta-analysis on reward anticipation
During reward anticipation, subjects of SCZ spectrum showed significant deactivation in the cingulate cortex [median part: coordinate (4, 4, 36), 443 voxels, z = −4.15, p < 0.001; anterior part: coordinate (−2, 48, 8), 163 voxels, z = −3.29, p < 0.001], as well as right putamen [coordinate (28, 10, 0), 31 voxels, z = −3.23, p < 0.001] and left caudate to ventral striatum areas [coordinate (−16, 24, 0), 23 voxels, z = −3.79, p < 0.001]. After FWE corrections, findings of cingulate cortex still remained significant (median part: pFWE = 0.002; anterior part: pFWE = 0.034). No publication bias was revealed by Egger's test for any peak (see Fig. 3 and Table S3).
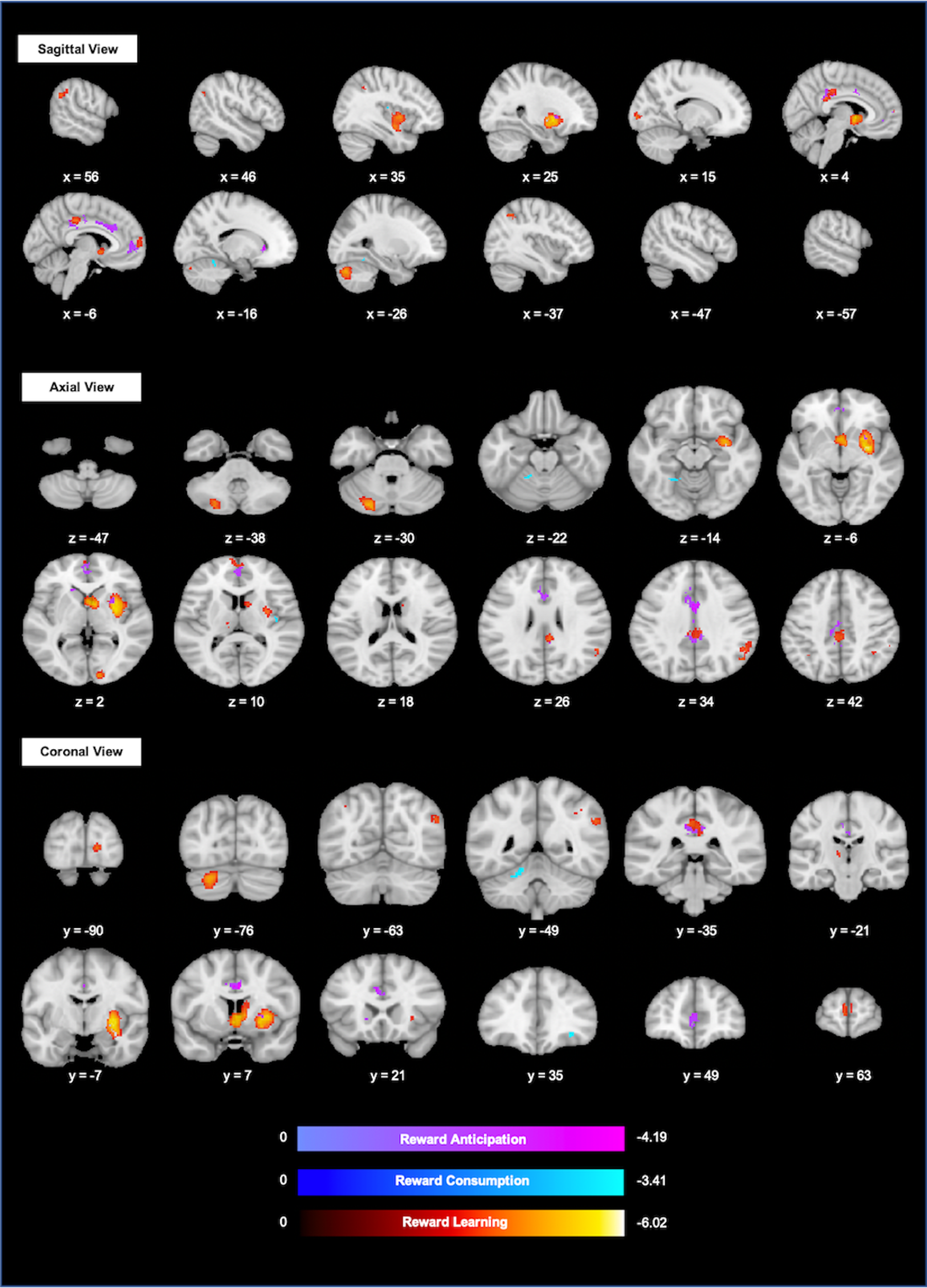
Fig. 3. Areas with abnormal activation related to reward anticipation (purple), reward consumption (blue), and reward learning (Red) in SCZ spectrum. Color bars represent SDM-Z scores (negative number for hypo-activations of SCZ spectrum: SCZ spectrum < HC in task > baseline). Results were considered significant for p < 0.0025, and cluster extent >10 voxels.
Meta-analysis on reward consumption
Meta-analysis on reward consumption revealed the deactivation of left cerebellar lobule IV/V area [coordinate (−18, −48, −24), 57 voxels, z = −3.21, p < 0.001], right insula [coordinate (40, −16, 14), 16 voxels, z = −3.06, p = 0.001], and right inferior frontal gyri [coordinate (32, 36, −10), 12 voxels, z = −3.37, p < 0.001] of SCZ spectrum subjects when compared with HCs. No peak survived FWE correction. Egger's test revealed no publication bias for any peak (see Fig. 3 and Table S3). There was no area with increased activation in SCZ spectrum.
Meta-analysis on reward learning
Regarding brain activity induced by reward learning, SCZ spectrum subjects showed decreased activation in right putamen [coordinate (30, −4, −2), 1149 voxels, z = −5.96, p < 0.001], bilateral thalamus [right: coordinate (6, 4, 0), 442 voxels, z = −5.32, p < 0.001; left: coordinate (−12, −18, 6), 25 voxels, z = −3.14, p < 0.001], left cerebellar Crus I [coordinate (−22, −80, −32), 389 voxels, z = −4.65, p < 0.001], median part of cingulate cortex [coordinate (−4, −28, 40), 341 voxels, z = −3.74, p < 0.001], left superior frontal gyrus [coordinate (0, 60, 6), 108 voxels, z = −3.49, p < 0.001], bilateral inferior parietal gyri [left: coordinate (−36, −56, 48), 52 voxels, z = −3.62, p < 0.001; right: coordinate (40, −50, 40), 12 voxels, z = −3.18, p < 0.001], right angular gyrus and calcarine fissure [coordinate (−14, −92, 2), 83 voxels, z = −4.02, p < 0.001]. After FWE corrections, findings of right putamen (pFWE < 0.001), right thalamus (pFWE = 0.002), and left cerebellar Crus I (pFWE = 0.02) still remained significant. Egger's test revealed no publication bias for any peak (see Fig. 3 and Table S3). There was no area with increased activation in SCZ spectrum.
Qualitative review on effort computation
In all three of the effort-related studies (Avsar et al., Reference Avsar, Weller, Cox, Reid, White and Lahti2013; Huang et al., Reference Huang, Yang, Lan, Zhu, Liu, Wang and Chan2016; Prettyman et al., Reference Prettyman, Kable, Didier, Shankar, Satterthwaite, Davatzikos and Wolf2021), whole-brain analyses found decreased activation of the ventral striatum in SCZ spectrum subjects. In addition, studies of Huang et al. (Reference Huang, Yang, Lan, Zhu, Liu, Wang and Chan2016) and Avsar et al. (Reference Avsar, Weller, Cox, Reid, White and Lahti2013) both reported deactivation of the caudate nucleus, cingulate cortex, prefrontal lobe, and parietal areas. In addition, Avsar et al. (Reference Avsar, Weller, Cox, Reid, White and Lahti2013) reported the functional decline of the thalamus and insula related to this component.
Convergence of neural mechanisms
The multi-model analyses for reward anticipation and reward learning found common brain functional impairments in the right PCC [coordinate (6, −40, 30), 42 voxels, z = −3.26, p < 0.001] and putamen [coordinate (28, 10, 0), 31 voxels, z = −3.23, p < 0.001] (see Supplementary Fig. S1 and Table S4). There was no convergent finding among other components.
Meta-regression results
Meta-regression revealed that the mean severity of negative symptoms (available in 15 studies) was negatively associated with hypoactivation in the media cingulate cortex [MNI coordinates (0, 10, 40), 366 voxels, z = − 2.15, p = 0.038] in SCZ patients when compared with HC. The mean age of SCZ spectrum groups (available in 37 studies) could positively predict the hypoactivation in the right postcentral gyrus [MNI coordinates (54, −20, 42), 305 voxels, z = 2.80, p = 0.002] and left caudate [MNI coordinates (−14, 20, −2), 67 voxels, z = 2.73, p = 0.003], and negatively predict that of right amygdala [MNI coordinates (26, 0, −10), 312 voxels, z = −2.67, p = 0.004]. There was no brain area significantly associated with the percentage of males (available in 37 studies), and the mean duration of illness (available in 10 studies).
Discussion
This meta-analysis showed abnormal brain activation patterns of the SCZ spectrum, using a ‘component-based approach’ for rewarding processing as well as the ‘whole-brain perspective’ for neural mechanisms. Our findings showed that SCZ spectrum patients exhibited reduced activation in the striatum, orbital frontal cortex, cingulate cortex, and cerebellar areas. Distinct patterns of brain alterations were found for different components. For instance, reward anticipation was associated with decreased activation of the cingulate cortex and striatum; reward consumption was associated with decreased activation of the cerebellum IV/V areas, insula and inferior frontal gyri; reward learning processing was associated with decreased activation of the striatum, thalamus, cerebellar Crus I, cingulate cortex, OFC, and some parietal and occipital areas. Our convergence findings suggested that both reward anticipation and reward learning components were associated with decreased activation in the dorsal striatum and PCC in individuals with SCZ spectrum. These regions could be implicated in impairments of both these two components.
Dysfunctions of the striatal-prefrontal regions
Altered functioning of the striatum, the core region of the reward circuit, appears to be putative neural mechanisms for the reward anticipation and reward learning components in SCZ spectrum groups. Receiving information input from cortical regions, and connecting with midbrain regions (Hunnicutt et al., Reference Hunnicutt, Jongbloets, Birdsong, Gertz, Zhong and Mao2016; Lerner et al., Reference Lerner, Shilyansky, Davidson, Evans, Beier, Zalocusky and Deisseroth2015), the striatum is believed to be an integrative hub between the mesolimbic system and the cortical cognitive and motor areas. Our findings are consistently with the literature suggesting that the striatum plays an important role primarily in reward anticipation and reward learnings processing in non-SCZ populations. For instance, craving and wanting feelings induced by incentives have been reported to be correlated with BOLD signals and dopamine release in the striatum, especially the ventral region (Delgado, Reference Delgado2007). Secondly, activity of striatal (especially the dorsal part) dopaminergic neurons has been shown to signify the discrepancy between expected and actual rewards, i.e. reward learning (Schultz, Dayan, & Montague, Reference Schultz, Dayan and Montague1997). Thus, reduced striatal activation of SCZ spectrum patients could reflect impaired ability of reward anticipation and value updating (Gold et al., Reference Gold, Waltz, Matveeva, Kasanova, Strauss, Herbener and Frank2012; Morris, Quail, Griffiths, Green, & Balleine, 2015).
During the process of reward learning, our findings suggested that SCZ spectrum exhibited decreased activation in the OFC. Neuronal firing of the OFC has been found to be correlated with the value of the stimulus (Padoa-Schioppa & Cai, Reference Padoa-Schioppa and Cai2011; Schoenbaum, Takahashi, Liu, & McDannald, Reference Schoenbaum, Takahashi, Liu and McDannald2011), through associating new stimulus with reinforcers (e.g. water, food, money) (Rolls & Grabenhorst, Reference Rolls and Grabenhorst2008). As such, reduced activation of the OFC related to reward learning in SCZ spectrum groups might reflect stimulus–reinforcer decoupling and impaired value updating, subsequently leading to reduced motivation to pursue rewards.
Dysfunction of the cingulate cortex
Our findings also supported the involvement of the ACC for reward anticipation in SCZ spectrum groups, and its potential role in effort computation. The ACC enables integrating cognition and emotion processing (Etkin, Egner, & Kalisch, Reference Etkin, Egner and Kalisch2011; Kolling et al., Reference Kolling, Wittmann, Behrens, Boorman, Mars and Rushworth2016) due to its connections with cortical and subcortical areas (Du et al., Reference Du, Rolls, Cheng, Li, Gong, Qiu and Feng2020; Neafsey, Terreberry, Hurley, Ruit, & Frysztak, Reference Neafsey, Terreberry, Hurley, Ruit, Frysztak, Vogt and Gabriel1993). During reward processing, it encodes the decision-making process based on reward prediction and outcome simulation, which could decide the allocation of cognitive and effort resources, i.e. effort computation (Chudasama et al., Reference Chudasama, Daniels, Gorrin, Rhodes, Rudebeck and Murray2013; Hadland, Rushworth, Gaffan, & Passingham, Reference Hadland, Rushworth, Gaffan and Passingham2003; Hayden, Pearson, & Platt, Reference Hayden, Pearson and Platt2009). There are two possible explanations for decreased activation in ACC in SCZ spectrum groups. On the one hand, dysfunction in the ACC may be an inherently damaged region in SCZ spectrum (Stark, Uylings, Sanz-Arigita, & Pakkenberg, Reference Stark, Uylings, Sanz-Arigita and Pakkenberg2004), resulting in reduced ability of decision-making for reward approaching; On the other hand, a decreased functional recruitment of the ACC in SCZ spectrum might reflect lower cognitive load during decision-making. Appreciating that high-effort high-reward conditions suggest that the magnitude of effort would commensurate with the magnitude of reward, it is likely to be a cognitive loading process. SCZ patients may experience limited cognitive demand because they tend to underestimate the value of reward (Dolan et al., Reference Dolan, Fletcher, Frith, Friston, Frackowiak and Grasby1995; Park et al., Reference Park, Chun, Park, Koo, Park, Kim and Kim2015).
We found the decreased activation of PCC of SCZ spectrum related to reward anticipation and reward learning. Although there has been no consensus on the function of the PCC (Leech & Sharp, Reference Leech and Sharp2014), its dysfunction can be explained from two point of views. First, the PCC is the central node of the brain's default mode network (Raichle, Reference Raichle2015), as a coordinator to balance between internally and externally directed cognition, then to modulate brain reaction flexibly and properly in changing environment (Leech, Braga, & Sharp, Reference Leech, Braga and Sharp2012; Smallwood et al., Reference Smallwood, Bernhardt, Leech, Bzdok, Jefferies and Margulies2021). Given the disrupted connection and metabolic changes of the PCC have been widely demonstrated in SCZ spectrum (Joo et al., Reference Joo, Chon, Rathi, Shenton, Kubicki and Lee2018; Shimizu et al., Reference Shimizu, Hashimoto, Ochi, Fukami, Fujisaki, Koike and Iyo2007; Wang et al., Reference Wang, Zeng, Chen, Yin, Tan and Hu2015), decreased activation in the PCC during reward processing might reflect SCZ spectrum patients' impairments in these basic cognitive functions (e.g. attention, switching). Second, the PCC has functional involvement in processing ‘self’-related information, through representing self-location (Brewer, Garrison, & Whitfield-Gabrieli, Reference Brewer, Garrison and Whitfield-Gabrieli2013), immersing in experience (Guterstam, Björnsdotter, Gentile, & Ehrsson, Reference Guterstam, Björnsdotter, Gentile and Ehrsson2015), and retrieval of autobiographical memories (Leech & Sharp, Reference Leech and Sharp2014; Maddock, Garrett, & Buonocore, Reference Maddock, Garrett and Buonocore2001; Svoboda, McKinnon, & Levine, Reference Svoboda, McKinnon and Levine2006). Self-processing impairments may disrupt the unity, wholeness, and coherence of one's understanding and response to the external world (Borda & Sass, Reference Borda and Sass2015; Sass & Parnas, Reference Sass and Parnas2003). Taking this perspective, reduced activation of the PCC in reward anticipation and reward learning processing might suggest the inconsistency of individuals' reward representation.
Dysfunction of the cerebellum
Interestingly, we found reduced activation of the cerebellum in patients with SCZ spectrum disorders during reward processing, especially related to reward learning and consumption. Recent evidence supported the extended role of the cerebellum, from motion to a wide range of cognitive processes (such as learning, attention, emotion) (Brady et al., Reference Brady, Gonsalvez, Lee, Öngür, Seidman, Schmahmann and Halko2019; Schmahmann & Caplan, Reference Schmahmann and Caplan2006). According to the ‘internal model’ (Ito, Reference Ito2008; Wolpert, Miall, & Kawato, Reference Wolpert, Miall and Kawato1998), the basic function of cerebellar cortex is to monitor internal and external signals, construct internal models to predict potential consequences, and to update such models based on predictive errors (Raymond, Reference Raymond2020; Schmahmann, Reference Schmahmann2019). The cerebellum may be involved in reward processing and learning in a similar way (Carta, Chen, Schott, Dorizan, & Khodakhah, Reference Carta, Chen, Schott, Dorizan and Khodakhah2019; Raymond, Reference Raymond2020) via its connection with the basal ganglia and the prefrontal lobe (Bostan, Dum, & Strick, Reference Bostan, Dum and Strick2013; Bostan & Strick, Reference Bostan and Strick2018). Our findings as such appear to support this notion. Furthermore, cerebellar alterations have been widely reported in SCZ patients (Lungu et al., Reference Lungu, Barakat, Laventure, Debas, Proulx, Luck and Stip2013; O'Neill, Mechelli, & Bhattacharyya, 2019; Picard, Amado, Mouchet-Mages, Olie, & Krebs, Reference Picard, Amado, Mouchet-Mages, Olie and Krebs2008). Specifically, the cortico-cerebellum-thalamus-cortical (CCTC) model posits its important role in the neurobiological mechanisms of SCZ (Andreasen, Paradiso, & O'Leary, Reference Andreasen, Paradiso and O'Leary1998; Andreasen & Pierson, Reference Andreasen and Pierson2008). The disruption of the CCTC circuit could lead to ‘cognitive dysmetria’, resulting in various symptoms of SCZ (Andreasen, Reference Andreasen1999; Andreasen et al., Reference Andreasen, Paradiso and O'Leary1998). Empirical studies supported that cerebellar abnormalities in SCZ are correlated with neurological soft signs (Cai et al., Reference Cai, Wang, Wang, Zhou, Huang, Wang and Chan2021), hallucinations (Pinheiro et al., Reference Pinheiro, Johnson, Amorim, Roberto, Schwartze, Kotz and Shenton2021), negative symptoms (Cao et al., Reference Cao, Wei, Zhang, Xiao, Zeng, Sweeney and Lui2021), and impaired cognitive and social functioning (Bernard & Mittal, 2015; Lungu et al., Reference Lungu, Barakat, Laventure, Debas, Proulx, Luck and Stip2013). Our findings concur with previous findings, and suggest that cerebellar damages might result in amotivation and anhedonia.
Implications
Taken together, consistent with our hypothesis, the brain functional alteration of SCZ spectrum groups during reward processing could be found not only in striatal and prefrontal areas, but also in cingulate cortex, cerebellum, and other regions. Different reward components were associated with specific patterns of brain impairment, but there was also regional overlap. These findings give us a hint that there might be a common substrate for multiple brain regions' functional impairments, which results in their abnormal manifestations for relevant reward components. This common substrate might be dopamine dysfunction, which is an enduring view of the pathogenesis of SCZ (Davis, Kahn, Ko, & Davidson, Reference Davis, Kahn, Ko and Davidson1991; Howes & Kapur, Reference Howes and Kapur2009). Neurotransmitters studies found that patients with SCZ were associated with excessive dopamine synthesis in mesolimbic areas (Fusar-Poli & Meyer-Lindenberg, Reference Fusar-Poli and Meyer-Lindenberg2013; Kegeles et al., Reference Kegeles, Abi-Dargham, Frankle, Gil, Cooper, Slifstein and Laruelle2010). The excessive dopamine release could mask adaptive phasic responses dopaminergic neurons, leading to aberrant salience on reward stimulus, and contributing to relative behavioral symptoms (McCutcheon, Abi-Dargham, & Howes, Reference McCutcheon, Abi-Dargham and Howes2019). Dopamine dysfunction arises not only from genetic susceptibility, but also from environmental risk factors, such as social isolation and subordination (Howes & Kapur, Reference Howes and Kapur2009; Howes, McCutcheon, Owen, & Murray, Reference Howes, McCutcheon, Owen and Murray2017). Therefore, early identification of risk factors (e.g. social anhedonia) and precise modulation of dopamine phase responses should be critical for addressing symptoms of motivational deficits and pleasure deficits. Moreover, other psychiatric conditions, such as major depression disorders and substance use disorders (Baskin-Sommers & Foti, Reference Baskin-Sommers and Foti2015), are believed to have altered reward processing. Future research can utilize transdiagnostic samples to identify shared and distant neural mechanisms for impaired reward processing.
Limitations
Several limitations of this study should be borne in mind. First, we did not find enough number of studies on the neural substrate for effort computation; therefore, we only presented qualitative rather than meta-analytic findings. Second, although a component-based approach was used, the studies included in each group remained heterogeneous regarding several factors, i.e. the types and the delivery way of reward stimuli, the fMRI data processing and reporting. Third, in order to minimize the influence of subjective factors, we did not score the quality of included studies. Lastly, only a small number of studies conducted correlation analysis between activation and symptoms, and these studies differed in ROIs selection and computation methods, we did not conduct the meta-analysis on the effect of correlation. The ‘brain–symptom’ relationship was roughly estimated by meta-regression analysis, which can only estimate the effects of group differences of behavioral indicators on group-level brain dysfunctions (Thompson & Higgins, Reference Thompson and Higgins2002).
Conclusions
This study comprehensively examined putative brain dysfunction patterns for different components of impaired reward processing in people with SCZ spectrum disorders using the whole-brain perspective. Our findings mainly suggested neural alterations in the striatum, orbital frontal cortex, cingulate cortex, and cerebellar areas. We also explored component-specific and shared neural alterations. Our findings partially support the ‘compartmentalization’ of reward processing in the Kring and Barch's model, and provided evidence to support putative neural mechanisms for amotivation and anhedonia symptoms in the SCZ spectrum.
Supplementary material
The supplementary material for this article can be found at https://doi.org/10.1017/S0033291723000703.
Acknowledgements
This study was supported by the Jiangsu Provincial Key Research and Development Programme (BE2020661), by the Scientific Foundation of Institute of Psychology, Chinese Academy of Sciences (E2CX3415CX), and the Phillip K.H. Wong Foundation.
Conflict of interest
Note.