Introduction
The search for evidence of gastrointestinal parasites in past populations allows us to trace the spread and distribution of these parasites throughout human evolution. It shows how changing environments and lifestyles have impacted parasite spread and host switching through time, and offers valuable information about the lifeways of past human groups (Reinhard, Reference Reinhard1992; Dittmar, Reference Dittmar2009; Dutour, Reference Dutour2013; Reinhard et al., Reference Reinhard, Ferreira, Bouchet, Sianto, Dutra, Iniguez, Leles, Le Bailly, Fugassa, Pucu and Araújo2013). In combination with historical and archaeological evidence, such evidence can allow us to explore why certain species flourished in different communities in the past. Parasites are closely linked to environmental conditions due to constraints of their life cycle which rely on specific intermediate hosts, and in some cases the external environment. Endoparasites are necessarily closely linked to human lifestyle, as people are known to alter their environments in ways that can allow certain parasite species to flourish, or may interact with animals in ways that lead to zoonotic infections. Therefore, investigations into the presence of parasites in a range of archaeological settings will inform us about the dynamics of parasitic infection over time (Mitchell, Reference Mitchell2013).
Most of the work investigating ancient parasites in Europe has focused on the Neolithic and Medieval periods, and relatively few studies have focused on the era in between, including the Bronze Age (Aspöck et al., Reference Aspöck, Flamm and Picher1973; Jones, Reference Jones and Bell1990; Šebela et al., Reference Šebela, Vojtkova and Vojtek1990; Le Bailly and Bouchet, Reference Le Bailly and Bouchet2010, Reference Le Bailly and Bouchet2013; Anastasiou et al., Reference Anastasiou, Papathanasiou, Schepartz and Mitchell2018). Furthermore, to our knowledge, only one study has been performed to investigate parasite diversity in Bronze Age Britain, at a farming settlement in southern England (Jones, Reference Jones and Bell1990). To improve our understanding of parasite infections in the population of Bronze Age Britain, we need to study prehistoric people who followed different lifestyles in different environments. This should enable us to determine the degree to which lifestyle, diet and environment may have affected parasite infections in our prehistoric ancestors.
Materials and methods
Must Farm
The Late Bronze Age pile-dwelling settlement at Must Farm was located in eastern England in an area known as the Flag Fen basin, which was part of a large wetland covering 46 000 km2 (Fig. 1). The Flag Fen basin was formed with rising sea levels after the last glacial maximum, and was later drained in the 17th century C.E. This region contains important prehistoric archaeological sites including Flag Fen and Fengate (Pryor, Reference Pryor2001). During the Bronze Age, there were numerous bodies of freshwater and brackish water interspersed by pockets of dry land, with periodic fluctuations in water levels (French, Reference French2003: 108). These environmental conditions supported a species-rich wetland habitat during the occupation of the Must Farm settlement, with widespread pollen evidence for sedge fen/reed swamp and nearby wet woodland dominated by alder (Scaife, Reference Scaife and Pryor2001). The settlement at Must Farm consisted of a series of five stilted timber structures surrounded by a palisade, all built over this freshwater. Analysis of plant and insect remains at the site shows that the water flowed at an extremely slow rate and contained dense aquatic plants including club-rush (Schoenoplectus lacustris), yellow water-lily (Nuphar lutea) and pondweeds (Potamogeton spp.) (Ballantyne, Reference Ballantyne, Knight, Ballantyne, Gibson and Robinson Zeki2018; Langdon, Reference Langdon, Knight, Ballantyne, Gibson and Robinson Zeki2018).

Fig. 1. Map indicating the location of the settlement at Must Farm in the east of England, close to present day Peterborough (marked on the left) and within The Fens (right). Image Credit: Cambridge Archaeological Unit.
Excavations of the site were undertaken in two seasons; a small exploratory excavation in 2006 (Gibson et al., Reference Gibson, Knight and Allen2010; Robinson et al., Reference Robinson, Knight and Murrell2015) and a larger excavation of the rest of the site from 2015 to 2016. These excavations revealed an exceptionally well-preserved Late Bronze Age settlement and associated artefacts including wood, textiles, ceramics and metalwork (Fig. 2). Radiocarbon dates place the period of occupation of the settlement during the 9th century B.C.E., which is the terminal Bronze Age in southern Britain (Knight et al., Reference Knight, Ballantyne, Robinson Zeki and Gibson2019). Dendrochronological evidence suggests that the settlement was constructed and inhabited for a very short period, around one year, before a large fire tore through the settlement causing the structures to collapse into the channel below where they were subsequently covered in fluvial silty clay. This waterlogged and anaerobic environment created ideal conditions for the preservation of organic and inorganic material from the Late Bronze Age settlement.

Fig. 2. Aerial photo of settlement showing timber outlines of structures and collection of sediment samples. Image Credit: Cambridge Archaeological Unit.
Samples
Sediment and coprolites were collected for parasite analysis during the 2015–2016 excavations of the site. We adapted sampling strategies that have been found effective at other prehistoric stilted settlements in Europe (Dommelier et al., Reference Dommelier, Bentrad, Bouchet, Paicheler and Pétrequin1998; Maicher et al., Reference Maicher, Hoffmann, Côté, Palomo Pérez, Saña Segui and Le Bailly2017). Sediment was taken from the occupation deposit (just beneath the ash layer) at 10 different locations across the settlement. Structures 1–4 were sampled, but Structure 5 was not sampled due to its prior excavation for evaluation of the site in 2006. Two locations from each structure were sampled; one from the sediment underneath the centre of the structure, and one from the outer edge of the structure where waste may have been discarded by the inhabitants (Fig. 3). Single samples were also taken from upstream of the entire settlement and downstream of the entire settlement. Sediment in the occupation deposits was a calcitic sandy/silty clay with humified organic material (French, Reference French, Knight, Ballantyne, Gibson and Robinson Zeki2018).

Fig. 3. Plan of Must Farm settlement indicating sample locations and coprolites. The outer edges of each structure are indicated by hashed lines, sediment sample locations are marked by large black dots, human coprolites are marked by orange circles, canine coprolites are marked by red circles, and uncertain coprolites are marked by blue circles. Image Credit: Vicki Herring.
Waterlogged coprolites (preserved pieces of feces) were also collected from around the structures, a total of fifteen were used for analysis (Fig. 3). Fecal biomarker analyses were conducted on the coprolites to determine their source. The fractions containing the target biomarkers, 5β-stanols and bile acids, were analysed by gas chromatography (GC) and GC-mass spectrometry (GC-MS). Eleven of the 15 coprolites yielded lipid profiles adequate for source identification (see Supplementary Material). Four were of human origin (SF3082, SF3127, SF3631, and SF3642) and seven were of canine origin (SF3175, SF3177, SF3178, SF3492, SF3677, SF3955, and SF4029) while the remaining four had inconclusive profiles due to high levels of compounds from the surrounding environment (SF3080, SF3176, SF3365, and SF4034). However, the size and morphology are consistent with the coprolites of identified origin, indicating that they could also be from humans or canines.
Microscopy
Sample preparation and analysis was undertaken in the Ancient Parasites Lab at the University of Cambridge using our routine methodology (Anastasiou and Mitchell, Reference Anastasiou and Mitchell2013). A 0.2 g subsample of each of the sediment samples and coprolites was disaggregated (made into a liquid suspension) by adding 5 mL of 0.5% trisodium phosphate to the subsample. During the subsampling of the coprolites, samples were taken from the core and obvious large objects such as bone were avoided. Material within the size range of interest was isolated from the disaggregate using stacked microsieves with mesh measuring 300, 160 and 20 µm. The material trapped on the 20 µm sieve was collected for analysis. This would contain any helminth eggs present, as the typical size range of eggs from helminths in Europe is 20–150 µm (Bouchet et al., Reference Bouchet, Guidon, Dittmar, Harter, Ferreira, Chaves, Reinhard and Araújo2003; Garcia, Reference Garcia2016). The suspension was centrifuged at 4000 rpm for 5 min and the supernatant removed. The remaining pellet was mixed with glycerol and the entire subsample was viewed on a digital light microscope at ×400 magnification to visualize any preserved parasite eggs. Egg counts per gram were calculated by multiplying the number of eggs present in the entire 0.2 g subsample by five.
Results
Preserved parasite eggs were identified in samples from all areas of the settlement, as well as in the upstream and downstream samples. Eggs from four taxa of endoparasites were recovered in the sediment: fish tapeworm (Diphyllobothrium sp.), whipworm (Trichuris sp.), Capillaria sp. and giant kidney worm (Dioctophyma renale). The concentration of helminth eggs found in each sediment sample is presented in Fig. 4. Helminth eggs from five taxa were found in the coprolites, and the concentrations of eggs found in the coprolites were higher than those in the sediment samples (Table 1). In addition to the species found in the sediments, the coprolites also contained eggs from Echinostoma sp. All coprolites contained helminth eggs with the exception of SF3080, a coprolite with inconclusive lipid profiles and poor integrity. Out of the sediments studied from the four structures at the site, Structure 3 had the highest concentrations of eggs, and also the highest species diversity. The eggs found in coprolites northeast of this structure (SF4034 and SF4029) also had the highest egg concentrations of all coprolites. The dimensions of all eggs found are summarized in Table 2.

Fig. 4. Parasite eggs and concentrations found in occupation deposits for each structure of the settlement at Must Farm. Sample locations are marked by large black dots and parasite eggs found in the occupation deposit at the sample location are indicated, eggs per gram are in brackets. Samples where no parasite eggs were found are labelled as no eggs. Image Credit: Vicki Herring.
Table 1. Parasite egg taxa and concentrations (eggs/gram) found in each coprolite

Table 2. Dimensions of eggs found in all sediment samples and coprolites

Mean lengths and widths with standard deviations (s.d.) of all eggs, followed by dimensions separated by those eggs with and without opercula or polar plugs.
Eggs of fish tapeworm (Diphyllobothrium sp.) were identified by their ovoid shape, dimensions, operculum, and in a few eggs, an apical knob was noted at the abopercular end (Fig. 5). There are 14 different species in the genus of Diphyllobothrium (Garcia, Reference Garcia2016). The ability to differentiate the species of Diphyllobothrium based on egg morphology has been debated (Scholz et al., Reference Scholz, Garcia, Kuchta and Wicht2009; Kuchta et al., Reference Kuchta, Brabec, Kubáčková and Scholz2013). Currently, there are no reliable structural or morphological characteristics that have been suitable for distinguishing the various species, but the sizes of the eggs do vary based on species and can give an indication (Leštinová et al., Reference Leštinová, Soldánová, Scholz and Kuchta2016). Two species commonly known to cause human infections in Europe are Diphyllobothrium latum and Diphyllobothrium dendriticum. The size of the eggs found at Must Farm is spread across the average size range for these two species (Fig. 6). The length–width ratio (LWR) was also calculated for each egg and they were spread across the overlapping ratios for D. latum and D. dendriticum. Therefore, it is likely that eggs from both species were present at the site.

Fig. 5. Fish tapeworm (Diphyllobothrium sp.) eggs from the settlement at Must Farm. Scale bars indicate 20 µm.

Fig. 6. Length and width of Diphyllobothrium sp. eggs with intact operculum from the settlement at Must Farm. Eggs found in coprolites are marked by dots and eggs found in sediment samples are marked by ‘X's. The typical size range of D. latum eggs is marked by the dashed box and the typical size range of D. dendriticum eggs is marked by the solid box. Size ranges taken from Leštinová et al., Reference Leštinová, Soldánová, Scholz and Kuchta2016.
Eggs from whipworm (Trichuris sp.) were the second most common helminth found throughout the sediments from the settlement, however, they were found in varying concentrations in the coprolites. Whipworm eggs were identified based on their lemon shape with opposing polar plugs at either end and the uniform thickness of the outer shell (Fig. 7) (Ash and Orihel, Reference Ash and Orihel2015). The mean length of whipworm eggs that had polar plugs was 58.6 µm (s.d. 3.5) and 53.7 µm (s.d. 2.6) without polar plugs, and the mean width of all eggs was 28.3 µm (s.d. 1.7). The majority of eggs were missing polar plugs, only nine eggs had preserved polar plugs. There are many species of whipworm that have varying definitive hosts, among these are Trichuris trichiura in humans and Trichuris suis in pigs. There is an overlap in size between human whipworm and pig whipworm, making it difficult to distinguish the two based on size. The dimensions of the whipworm eggs found at Must Farm compared to the average size ranges of human and pig whipworm eggs without polar plugs can be seen in Fig. 8. Almost all of the whipworm eggs found at the settlement fit within the size range of pig whipworm (T. suis), while only some fall exclusively within the size range of human whipworm (T. trichiura). Even though seven of the coprolites, and possibly more, came from canines, none of the whipworm eggs found in the coprolites were large enough to come from T. vulpis (typical size range 70–90 × 30–40 µm) (Mehlhorn, Reference Mehlhorn2016). Similarly, there is no clustering of the eggs recovered from human coprolites in the size range of T. trichiura. For this reason, it seems most likely that the whipworm eggs at the site originate from pigs, though the concurrent presence of human whipworm cannot be definitively excluded. Pig and wild boar remains were recovered around the settlement area suggesting their presence and use as a food source (Rajkovača, Reference Rajkovača, Knight, Ballantyne, Gibson and Robinson Zeki2018).

Fig. 7. Whipworm (Trichuris sp.) eggs from the settlement at Must Farm: (A) with preserved polar plugs; (B) without polar plugs. Scale bars indicate 20 µm.

Fig. 8. Length and width of Trichuris sp. eggs missing polar plugs from the settlement at Must Farm. Size range of Trichuris trichiura (human whipworm) eggs without polar plugs in solid black line and size range of Trichuris suis (pig whipworm) eggs without polar plugs in dashed line (size ranges from Beer, Reference Beer1976).
Eggs of Capillaria worm (Capillaria sp.) were found in lower concentrations in the sediment samples compared to the coprolites but were present in all coprolites except one human coprolite and SF3080, which did not contain any preserved eggs. Capillaria sp. eggs were found in the upstream sample as well as in coprolites around the other structures. The surface of the eggs of Capillaria appeared to have irregularly dispersed, round punctuations (Fig. 9). The eggs had a darker outer coat, which contained the punctuations, and a thinner inner coat. This ornamentation is consistent with what has been called the punctuated type (Maicher et al., Reference Maicher, Hoffmann, Côté, Palomo Pérez, Saña Segui and Le Bailly2017), or mamillated type (Bouchet, Reference Bouchet1997). The species of Capillaria could not be identified merely based upon the morphology and dimensions of these eggs.
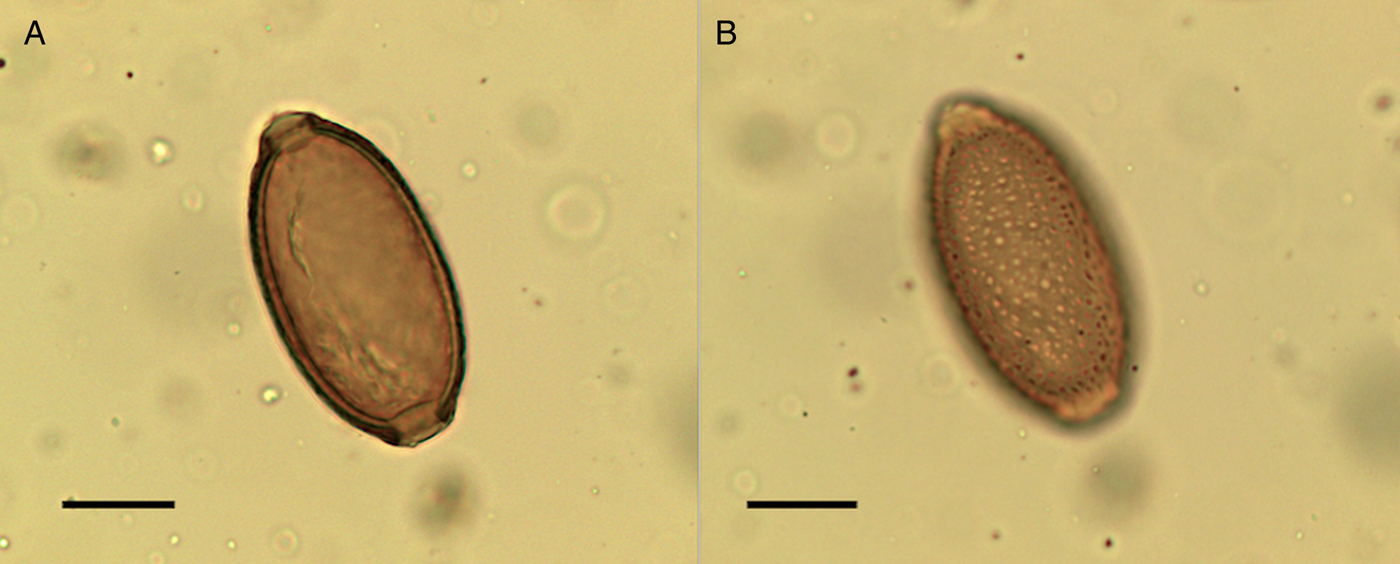
Fig. 9. Capillaria sp. egg from the settlement at Must Farm. Images (A) and (B) are from the same egg; the surface structure of the egg is visible highlighting the punctuated surface (B). Scale bars indicate 20 µm.
Giant kidney worm (Dioctophyma renale) is a roundworm that is acquired from eating raw or undercooked freshwater fish or frogs. It has low host specificity and causes infection in a range of fish eating mammals, including humans. The adult worm develops in the pelvis of the kidneys where it causes the eventual destruction of the kidney tissue as it grows to occupy the entire renal capsule, resulting in kidney failure (Garcia, Reference Garcia2016). One egg from the giant kidney worm was recovered in the sediment from Structure 3, and one egg in a canine coprolite (SF4029) found northeast of Structure 3. This species was identified by its thick shell with characteristic wrinkled sculpturing, elliptical shape, light brown colour, polar plugs and dimensions (Fig. 10). The mean length of the two eggs was 74.9 µm and width 40.2 µm (typical range 60–80 × 39–46 µm) (Pedrassani et al., Reference Pedrassani, Hoppe, Avancini and do Nascmento2009).
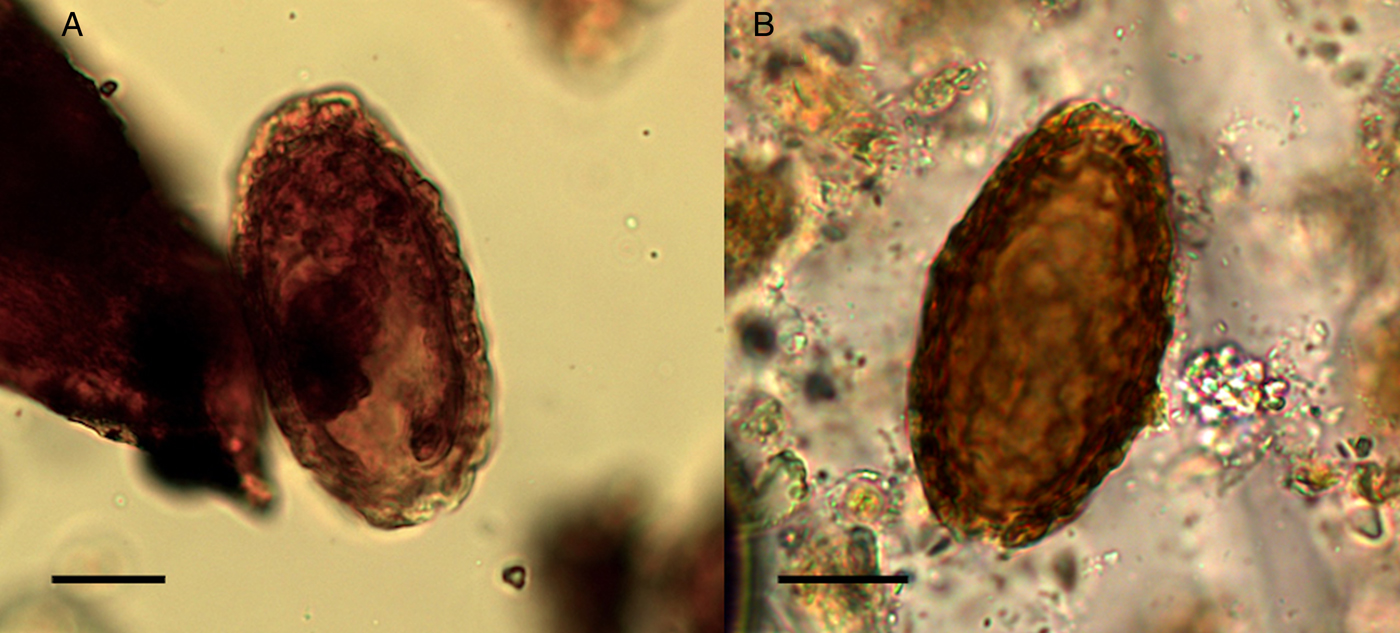
Fig. 10. The giant kidney worm (Dioctophyma renale) eggs: (A) from coprolite SF4029; (B) from sediment. Scale bars indicate 20 µm.
Echinostoma sp. eggs were only recovered from the coprolites. They were present in both canine and human coprolites. Morphologically they appear very similar to Fasciola sp. or Paragonimus sp., but the average size of eggs found in the coprolites (104.4 µm × 69.9 µm) is smaller than the average size of Fasciola sp. and the modern geographical distribution of Paragonimus does not include northern Europe (Mehlhorn, Reference Mehlhorn2016). The eggs had small opercula with barely visible shoulders and a thickened wall at the abopercular end (Fig. 11) consistent with eggs recovered from modern echinostomiasis infections (Toledo and Estaban, Reference Toledo and Esteban2016; Sah et al., Reference Sah, Khadka, Hamal and Poudyal2018). There are many species of Echinostoma that we cannot differentiate based on the morphology of the eggs.
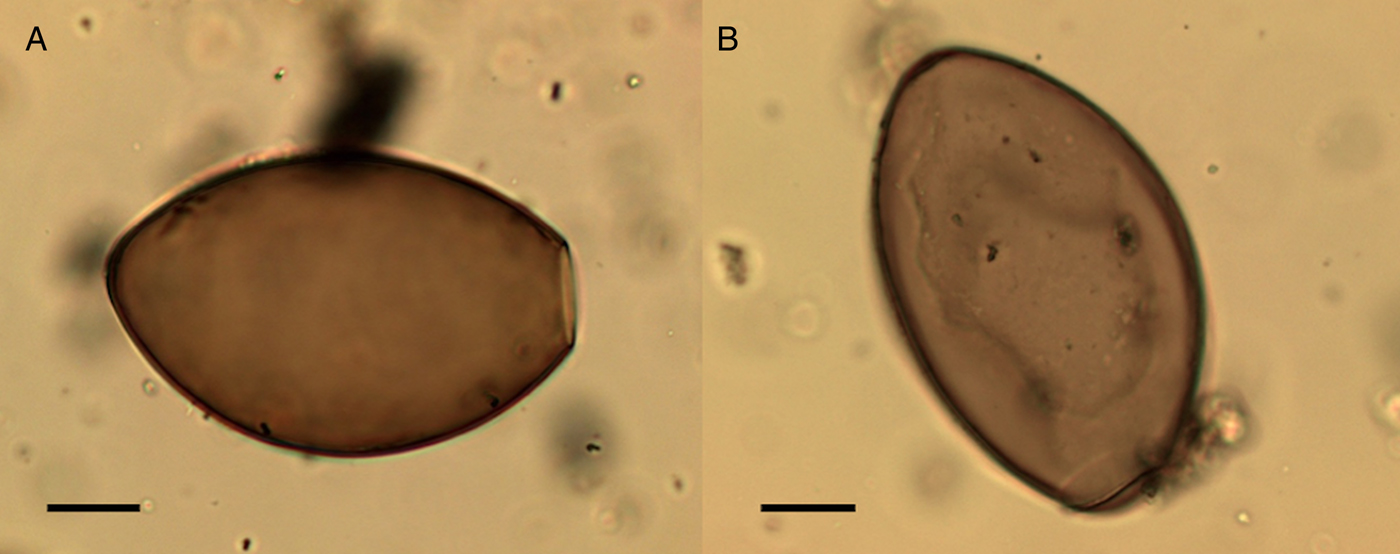
Fig. 11. Echinostoma sp. eggs from the settlement at Must Farm. Egg missing operculum and thickening at abopercular end visible (A), egg with an operculum (B). Scale bars indicate 20 µm.
Discussion
Species identification
Modern geographic distribution of fish tapeworm (Diphyllobothrium) species can be used as an indicator of which species of Diphyllobothrium may have been endemic in Late Bronze Age Britain. Based on reports of parasite larvae found in freshwater fish in Britain and Europe in modern fish samples, the two endemic species in the modern period that cause human infection are D. latum and D. dendriticum (Chappell and Owen, Reference Chappell and Owen1969; Scholz et al., Reference Scholz, Garcia, Kuchta and Wicht2009; Brewster, Reference Brewster2016). Morphometric data including length, width, and LWR from the fish tapeworm eggs (Fig. 6) found at Must Farm fit reasonably into reported ranges for these two species, and the spread of dimensions is too broad to be explained by just one species alone. Therefore, it seems likely that both D. latum and D. dendriticum were endemic in Late Bronze Age Britain. Genetic analysis of these species is currently the best way to generate an accurate species identification (Wicht et al., Reference Wicht, Yanagida, Scholz, Ito, Jiménez and Brabec2010; Kuchta et al., Reference Kuchta, Serrano-Martínez and Scholz2015; Thanchomnang et al., Reference Thanchomnang, Tantrawatpan, Intapan, Sanpool, Lulitanond, Tourtip, Yamasaki and Maleewong2016).
Identification of the species of whipworm (Trichuris sp.) eggs with microscopy can be challenging. Multiple authors have suggested that species of Trichuris can be differentiated based on the dimensions of their eggs (Beer, Reference Beer1973, Reference Beer1976; Confalonieri et al., Reference Confalonieri, Ribeiro-Filho, Ferreira and Araújo1985; Maicher et al., Reference Maicher, Hoffmann, Côté, Palomo Pérez, Saña Segui and Le Bailly2017). However, the size ranges of these two species overlap and there is considerable variation in the sizes of eggs produced by worms of the same species. Virtually all the whipworm eggs found at the site fall within the size range of pig whipworm (T. suis), so based upon egg dimensions, pig whipworm seems the likely diagnosis. While we cannot rule out the possibility that both human and pig whipworm were present at the site, the dimension of eggs found in dog coprolites was the same as those in human coprolites, and since dogs do not get human whipworm infection, there is no reason to propose two different whipworm species were present. The eggs of pig whipworm recovered in the canine and the human coprolites likely represent cases of false parasitism. Zooarchaeological evidence indicates that butchered domesticated pigs and wild boar were present around the site (Rajkovača, Reference Rajkovača, Knight, Ballantyne, Gibson and Robinson Zeki2018) and either of these sources could serve as a definitive host for T. suis. Whipworm has been found in Mesolithic contexts from Britain indicating their prior presence in the region; however, these eggs were also identified as either human or pig (Dark, Reference Dark2004). The eggs at the Must Farm settlement may have ended up in the coprolites around the settlement if porcine intestines were a food source for humans and canines, and ingested eggs from these intestines passed through the gastrointestinal tract of humans and dogs. Further sources of eggs in the sediment could include offal discarded in the water after the butchery of pigs, or if fecal waste from animals feeding near the edge of the channel was washed into the channel. If the freshwater under and around the settlement was used as a source of drinking water for humans and animals, eggs could also be ingested from this contaminated water, and pass through the gastrointestinal tract to end up in the coprolites.
Capillaria sp. helminths infect many mammals. In Europe, the most commonly documented human cases come from Capillaria hepatica. Unlike some other species of Capillaria, eggs are not found in the human stool in C. hepatica infection. However, eggs may be present in the stool in spurious infections (false parasitism), when liver from infected animals is eaten and eggs are passed through the digestive tract and released in the stool (Garcia, Reference Garcia2016). Therefore, the presence of eggs in the samples from the settlement may indicate that humans and canines were eating the liver of infected animals (wild or domesticated) in the area. Other species known to cause human infection include C. aerophila, C. philippinensis and C. plica. The eggs found at the settlement had a punctuated surface, it has been suggested that eggs of the punctuated morphotype come from C. aerophila, a species that lives in the respiratory system, or species that parasitize fish (Moravec, Reference Moravec1980; Bouchet, Reference Bouchet1997; Fugassa et al., Reference Fugassa, Taglioretti, Gonçalves, Araújo, Sardella and Denegri2008; Traversa et al., Reference Traversa, Di Cesare, Lia, Castagna, Meloni, Heine, Strube, Milillo, Otranto, Meckes and Schaper2011). Due to the aquatic nature of the site, and the presence of other helminth species that flourish in aquatic environments, the presence of Capillaria spp. that parasitize fish would be compatible with the context of the site.
Prehistoric lifeways revealed through parasite remains
These findings tell us a considerable amount about the lifestyle of inhabitants of the Must Farm settlement, including their waste and sanitation practices, risks for parasitic infection and the use of habitation areas.
Helminth eggs were found throughout the settlement area, including upstream and downstream of the settlement. This indicates that there was a general contamination of the settlement area with the fecal material of host organisms. The absence of toilet infrastructure in Late Bronze Age Britain seems to have resulted in inhabitants of the settlement disposing of fecal material into the water around the settlement. The channel that the structures were built over was very slow moving, in part due to aquatic vegetation and the wetland environment of the Fens. This means that waste would have accumulated in the channel without being immediately washed away with the flow of the water.
Disposal of human or animal waste into the surrounding water allows for completion of the life cycle of Echinostoma sp., fish tapeworm and the giant kidney worm. This would especially be the case if raw or poorly cooked aquatic organisms were eaten. Faunal remains from the site indicate that fish was eaten with some regularity by the inhabitants of the site (Knight et al., Reference Knight, Ballantyne, Robinson Zeki and Gibson2019). Some of these fish were likely to contain larvae from Diphyllobothrium dendriticum, Diphyllobothrium latum, Dioctophyma renale or Echinostoma sp. since we know that these parasites were present in the aquatic environment of the settlement. Most cases of echinostomiasis are caused by ingestion of molluscs. However, reports of human infections have cited freshwater fish, snails, frogs and tadpoles as potential intermediate hosts that if ingested can lead to the development of the adult worm in the intestines (Toledo and Esteban, Reference Toledo and Esteban2016; Chunge and Chunge, Reference Chunge and Chunge2017; Sah et al., Reference Sah, Khadka, Hamal and Poudyal2018).
Eggs from the giant kidney worm are released in the urine of infected hosts. Therefore, it is not surprising that an egg was recovered in the sediment as it appears that fecal waste was deposited in the water, and urine likely would have been as well. However, the recovery of an egg in one of the coprolites indicates that either urine was mixed in with feces when deposited, or that this is a case of spurious infection and the egg was ingested in contaminated water from around the settlement. After finding eggs of Dioctophyma renale in coprolites from a Neolithic site in Switzerland, Le Bailly et al. (Reference Le Bailly, Leuzinger and Bouchet2003) suggested that this nematode may have caused more human infections in prehistory than it does in modern populations, where human infections are rare. This may have been the case for the inhabitants of this settlement, where the parasite egg distributions and diversity suggest that fecal material from humans and animals was discarded in the water surrounding the settlement and fish were then eaten from this same water resulting in ingestion of eggs from giant kidney worm and Echinostoma sp. that rarely cause human infections in the region today. Most coprolites contain low egg concentrations of all taxa, making it difficult to distinguish spurious infections from true infections, as an unknown number of eggs may have been lost since the feces were deposited in the Late Bronze Age. There are two coprolites that have very high egg concentrations of Capillaria sp., fish tapeworm and Echinostoma sp. which are more indicative of true infections. Fecal lipid biomarkers identified one of these as a canine coprolite, indicating an infection of dogs with these parasites. However, the lipid biomarkers from the other coprolite were inconclusive in determining whether the coprolite was from a human or dog. Regardless, we have convincing evidence that eggs from these species were ingested by humans, and a number of infections likely occurred in humans living at the settlement.
In the past, it has been common to assume that any fish tapeworm eggs found in human contexts belonged to D. latum as this is the species that is thought to cause the most human infections globally today (Scholz et al., Reference Scholz, Garcia, Kuchta and Wicht2009). Recent work in parasitology aided by genetics has shown that in fact some cases of D. dendriticum may have been misdiagnosed as D. latum (Kuchta et al., Reference Kuchta, Brabec, Kubáčková and Scholz2013). In a time when we are seeing decreasing rates of helminth infections in developed countries due to adequate sanitation, hygiene and medical treatment, there has been a recent increase in rates of fish tapeworm infection and spread of these two species to parts of the world where they were previously uncommon (Dupouy-Camet and Peduzzi, Reference Dupouy-Camet and Peduzzi2004; Sampaio et al., Reference Sampaio, de Andrade, Lucas, Fung, Gagliardi, Santos, Mendes, Eduardo and Dick2005; Kuchta et al., Reference Kuchta, Brabec, Kubáčková and Scholz2013; Gustinelli et al., Reference Gustinelli, Menconi, Prearo, Caffara, Righetti, Scanzio, Raglio and Fioravanti2016; Scholz and Kuchta, Reference Scholz and Kuchta2016). These recent increases in fish tapeworm infection have been linked to long distance trade of fish products and increased consumption of raw fish in cultural dishes such as sushi, smoked salmon, carpaccio and ceviche. Evidence from the past has shown how cultural and social factors in conjunction with climate influence the spread and prevalence of parasites (Le Bailly et al., Reference Le Bailly, Leuzinger, Schlichtherle and Bouchet2007; Arriaza et al., Reference Arriaza, Reinhard, Araújo, Orellana and Standen2010; Yeh et al., Reference Yeh, Pluskowski, Kalējs and Mitchell2014). For example, fish tapeworm may have been spread in Roman Period Europe with the consumption of the uncooked fish sauce garum (Mitchell, Reference Mitchell2017). Similarly, widespread evidence for fish tapeworm in northern Europe during the Medieval Period may be associated with the spread of Christianity, which brought with it religious guidelines for fish consumption on certain days of the week (Mitchell, Reference Mitchell2015). Sites such as this settlement at Must Farm emphasize the role of dietary sources, sanitation and waste removal in parasite prevalence. These periodic fluctuations in fish tapeworm prevalence throughout human history exemplify how humans have a strong influence on the ecology of human parasites through our constantly changing sociocultural practices.
Reconstructing parasite biodiversity in Bronze Age Europe
As well as a better understanding of the lifeways of the Late Bronze Age population of the Must Farm settlement, the evidence gained from this study informs our understanding of parasite biodiversity in the past. Currently there are only four other archaeological sites that have revealed evidence for parasite species in Bronze Age Europe. These sites are located in Austria, Britain, France and the Czech Republic (Fig. 12). The eggs of roundworm (Ascaris sp.), whipworm (Trichuris trichiura), hookworm (Ancylostoma duodenale), fish tapeworm (Diphyllobothrium sp.), Lancet liver fluke (Dicrocoelium sp.) and the cysts of Entamoeba histolytica have been identified from these sites (Aspöck et al., Reference Aspöck, Flamm and Picher1973; Jones, Reference Jones and Bell1990; Šebela et al., Reference Šebela, Vojtkova and Vojtek1990; Gonçalves et al., Reference Goncalves, da Silva, de Andrade, Reinhard, da Rocha, Le Bailly, Bouchet, Ferreira and Araujo2004; Le Bailly and Bouchet, Reference Le Bailly and Bouchet2010, Reference Le Bailly and Bouchet2013; Anastasiou, Reference Anastasiou and Mitchell2015). Additionally, Trichuris sp. eggs have been reported, but not published, from a Bronze Age refuse pit at the site of Apalle in Sweden (Bergman, Reference Bergman2018). Previously the only other Bronze Age archaeological site in Britain that has been studied for evidence of ancient parasites was Brean Down, a coastal farming site in the Bronze Age (Jones, Reference Jones and Bell1990). Unlike the Must Farm settlement, this site was not located near any bodies of freshwater. Roundworm and whipworm eggs were identified in Middle Bronze Age coprolites from the site, which are dated between 1500 and 1100 B.C.E. Roundworm and whipworm, both soil-transmitted helminths, appear to be widespread during the Bronze Age as these two parasites were found together at three of the four previous sites studied. It is interesting that the range of species found at the Must Farm settlement is completely different to that found at Brean Down, and the absence of roundworm is particularly notable as roundworm has been found at many archaeological sites from the Neolithic Period through the Medieval Period in Europe (Anastasiou, Reference Anastasiou and Mitchell2015).

Fig. 12. Bronze Age sites in Europe where intestinal parasites have been found in archaeological samples. These include Brean Down, Britain (Jones, Reference Jones and Bell1990); Grésine, France (Gonçalves et al., Reference Goncalves, da Silva, de Andrade, Reinhard, da Rocha, Le Bailly, Bouchet, Ferreira and Araujo2004; Le Bailly and Bouchet, Reference Le Bailly and Bouchet2010; Le Bailly and Bouchet, Reference Le Bailly and Bouchet2013); Hallstatt salt mines, Austria (Aspöck et al., Reference Aspöck, Flamm and Picher1973); and Hulín, Czech Republic (Šebela et al., Reference Šebela, Vojtkova and Vojtek1990). Parasite taxa found are listed in light grey boxes beside site names.
Perhaps the most ecologically similar site that has been previously studied in Bronze Age Europe would be Grésine, a lakeside settlement in France (Kerouanton, Reference Kerouanton2002). At this site, evidence for Lancet liver fluke, fish tapeworm and Entamoeba dysentery was found (Gonçalves et al., Reference Goncalves, da Silva, de Andrade, Reinhard, da Rocha, Le Bailly, Bouchet, Ferreira and Araujo2004; Le Bailly and Bouchet, Reference Le Bailly and Bouchet2010, Reference Le Bailly and Bouchet2013). In fact, this is the only other evidence for fish tapeworm in the entire Bronze Age, suggesting that living in close proximity to freshwater was a major risk factor for fish tapeworm infection. It seems likely that the wetland environment of Must Farm played a key role in establishing different types of parasites in the settlement compared to dryland settlements. Living over slowly moving water may have protected the inhabitants from infection by soil-transmitted parasites like roundworm, but put them at risk for echinostomiasis, fish tapeworm or giant kidney worm when they ate freshwater fish, frogs or molluscs. Similar patterns have been noted for lakeside settlements in Neolithic Period Europe, where the distinct lack of eggs from the genus Ascaris is noted, but with the presence of fish tapeworm eggs (Bouchet et al., Reference Bouchet, Petrequin, Paicheler and Dommelier1995; Dommelier et al., Reference Dommelier, Bentrad, Bouchet, Paicheler and Pétrequin1998; Le Bailly, Reference Le Bailly2005; Le Bailly et al., Reference Le Bailly, Leuzinger, Schlichtherle and Bouchet2005; Maicher et al., Reference Maicher, Hoffmann, Côté, Palomo Pérez, Saña Segui and Le Bailly2017, Reference Maicher, Bleicher and Le Bailly2019).
At the Neolithic pile-dwelling of Alvastra in Sweden, eggs of Trichuris sp. and Fasciola hepatica (liver fluke) were found in a sediment sample (Bergman, Reference Bergman2018). Again there is a lack of Ascaris consistent with other settlements, but the parasite diversity is lower than other Neolithic lakeside settlements. The absence of Fasciola sp. at the Must Farm settlement is notable as it is found in many archaeological samples (Ledger and Mitchell, Reference Ledger and Mitchell2019). Though lamb remains were recovered from the site (Rajkovača, Reference Rajkovača, Knight, Ballantyne, Gibson and Robinson Zeki2018), accompanying sheep/goat dung pullets are dominated by seeds of terrestrial plants (Clapham, Reference Clapham2019). Therefore, sheep and cattle may have been herded away from the settlement area and terrestrial plants favoured over aquatic plants which can transfer the infection to humans.
The only other prehistoric site where eggs of Dioctophyma renale have been identified is one of these Neolithic lakeside settlements, the site of Arbon-Bleiche in Switzerland (Le Bailly et al., Reference Le Bailly, Leuzinger and Bouchet2003). Today echinostomiasis is mostly limited to Asia and the Far East. Palaeoparasitological studies have identified eggs of Echinostoma sp. in coprolites from Brazil (1390 C.E.) and Mexico (600 C.E.) (Jiménez, Reference Jiménez, Gardner, Araújo, Fugassa, Brooks, Racz and Reinhard2012; Leles et al., Reference Leles, Cascardo, Freire, Maldonado, Sianto and Araújo2014). There are two other reports of possible eggs from Echinostoma sp. in the Old World. In Mesolithic sediment samples from a lake island in Ireland (Perri et al., Reference Perri, Power, Stuijts, Heinrich, Talamo, Hamilton-Dyer and Roberts2018), and sediment samples from the Neolithic lakeside settlement of Zürich-Parkhaus-Opéra, Switzerland (Maicher et al., Reference Maicher, Bleicher and Le Bailly2019). The evidence presented from Must Farm is the earliest evidence for Echinostoma sp. in Britain.
Though the parasites found at the Must Farm settlement are broadly similar to Neolithic lakeside settlements in the Alpine region of Europe, there is a lower species diversity at Must Farm compared to the Neolithic sites. Based on current data, when we compare the parasite taxa found at archaeological sites in the European Bronze Age sites to the preceding Neolithic Period we see a contraction of the parasite ecosystem through time. This fits within the broad changes in patterns of a parasite infection that have been noted from the Neolithic Period to the Industrial era (Reinhard and Pucu de Araújo, Reference Reinhard, Pucu de Araújo and Zuckerman2014). With changes in subsistence strategies, population size and population density we see a move from zoonotic infections gained from wild reservoirs in the Neolithic Period to zoonotic infections from domestic reservoirs, and the eventual ubiquity of fecal–oral parasites (such as roundworm and whipworm). Overall, fewer parasite species have been found at Bronze Age sites in Europe compared to Neolithic sites; nine different taxa in the Bronze Age including our results from Must Farm compared to fourteen in the Neolithic (Anastasiou, Reference Anastasiou and Mitchell2015; Maicher et al., Reference Maicher, Hoffmann, Côté, Palomo Pérez, Saña Segui and Le Bailly2017). Some Neolithic sites have evidence for as many as nine different taxa in one settlement (Dommelier-Espejo, Reference Dommelier-Espejo2001; Le Bailly et al., Reference Le Bailly, Leuzinger and Bouchet2003; Gonçalves et al., Reference Goncalves, da Silva, de Andrade, Reinhard, da Rocha, Le Bailly, Bouchet, Ferreira and Araujo2004; Maicher et al., Reference Maicher, Hoffmann, Côté, Palomo Pérez, Saña Segui and Le Bailly2017). The five taxa found at Must Farm is higher than the number of taxa found at other Bronze Age sites thus far, and the taxa represented are similar to those seen in Neolithic lakeside settlements. This indicates the important role of ecology alongside sociocultural practices such as diet, waste disposal, and animal-human relationships for parasite transmission in the past.
Conclusions
We have identified prehistoric evidence for fish tapeworm, Echinostoma worm, giant kidney worm, probable pig whipworm and Capillaria worm in Britain. This shows that these parasites were endemic in Late Bronze Age Britain and part of the ecosystem of the wetlands in which the Must Farm settlement was built. Therefore, we know that the inhabitants of this settlement were at high risk of infection with these species and both human and canine coprolites recovered from the site contained eggs from multiple taxa.
The parasite species found at the Must Farm settlement were different from those found at the dry, coastal Bronze Age site of Brean Down in southern Britain and to other sites studied in Bronze Age Europe. This highlights the important role of site ecology combined with past human activities in determining the species of parasites that can flourish in various localities. Both the dumping of human and animal excrement into the freshwater channel that the settlement was built in, and consumption of aquatic organisms from the surrounding area, created an ideal nexus for infection with various species of the intestinal helminth. The similarity of the taxonomic diversity found at the Must Farm settlement to Neolithic lakeside settlements suggests that living at the wetland site of Must Farm protected the inhabitants from some species of parasite spread by human feces, such as roundworm, but put them at risk of Echinostoma sp., fish tapeworm and giant kidney worm due to their aquatic environment.
Supplementary material
The supplementary material for this article can be found at https://doi.org/10.1017/S0031182019001021
Acknowledgements
We would like to thank the archaeological team who excavated the site of Must Farm.
Financial support
The Must Farm project is funded jointly by Historic England and Forterra Building Projects Ltd. The work of Marissa Ledger was funded by a Social Sciences and Humanities Research Council of Canada Doctoral Award [752-2016-2085], The Cambridge Commonwealth, European and International Trust, and Trinity Hall College, University of Cambridge.
Conflicts of interest
None.
Ethical standards
Not applicable.