Allulose in food and exposure levels
D-Allulose, a rare ketohexose, is an epimer of D-fructose. It is produced in nature by epimerases that convert fructose or other ketohexoses into allulose but can also be generated from fructose by chemical conversion mediated by heat and pH. It is thus not surprising that allulose becomes detectable in heat-treated products, particularly when they contain high concentrations of fructose or sucrose. It is also not surprising that heat treatment of food products containing larger quantities of these sugars generates Maillard products, which has been demonstrated for allulose with the model protein ß-lactoglobulin(Reference Zeng, Zhang and Guan1). Based on the analysis of allulose content in conventional food products (with no added allulose), mean daily intake (breakfast, lunch and dinner) of allulose was estimated at 206 mg/d in Japan, including soda drinks and some fruit juices(Reference Oshima, Kimura and Izumori2). Highest amounts of allulose were found in caramel and Worchester sauce, brown sugar, ketchup, coke and selected dried and canned fruits (see Table 1). Although these findings may a priori not be applicable for a proper exposure assessment in other countries, it seems plausible to estimate that normal daily intake rates via Western-style diets (not by enriched products) may not exceed 200 mg/d. It is interesting to note that excretion of allulose via urine from such a background diet was estimated to rank from 25 to 50 mg/d based on 1·5 l of urine(Reference Whistler, Singh and Lake3), which matches with the limited oral availability of allulose.
Table 1. Content of D-allulose naturally occurring in foods
(adopted from(Reference Oshima, Kimura and Izumori2))
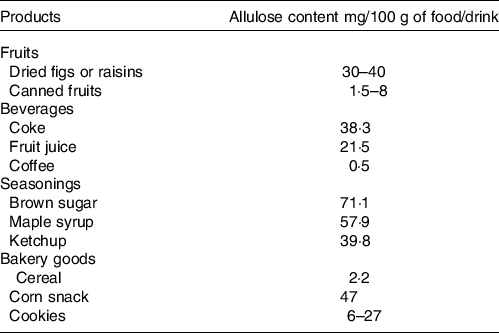
In a recent study in Germany, urine samples from 300 volunteers were analysed for the appearance of about 50 different sugars and sugar derivatives. Food intake data were obtained via 24-h recall. Allulose levels in urine associated positively with the consumption of bread and other bakery goods, which provide a substantial quantity of the daily carbohydrate intake in Germany(Reference Mack, Weinert and Egert4). With the same analytical MS platform, postprandial plasma levels in healthy volunteers and those with insulin resistance or type II diabetes were analysed for appearance of sugars and sugar derivatives when 50 g of maltodextrin was ingested as oral glucose tolerance test. Surprisingly, allulose levels increased steadily over a 90-min period, independent of the health status, while glucose levels displayed characteristic profiles with a peak at about 30–60 min(Reference Mack, Ferrario and Weinert5). Allulose levels in the maltodextrin product were below detection limit. This finding of increasing allulose levels in blood in postprandial state (up to 90 min) may be due to absorption from the ingested test solution but may also reflect an endogenous production from glucose converted in cells to fructose phosphates and fructose. However, which 3-epimerase (likely via a side activity) could mediate such an endogenous production of allulose is unknown.
Assuming that allulose is supplemented in similar products as those found in the USA or Japan and by taking food consumption levels relevant for Europe into account (e.g. as given by the European Food Standard Agency comprehensive food consumption database), a mean intake of D-Allulose of about 400 mg/kg body weight per d from such enriched products can be expected for an adult individual. Such an estimation brings allulose intake close to that of fructose from added sugars in European countries(Reference Azaïs-Braesco, Sluik and Maillot6).
The fate of ingested allulose
In mammals, allulose utilises in essence the same pathways as dietary fructose for uptake into the body, distribution and excretion. Intestinal absorption of allulose is mediated by the transporter GLUT5 in apical membranes of epithelial cells, while the export across the basolateral membrane occurs via GLUT2(Reference Kishida, Martinez and Iida7). As both transporters are uniporters that mediate an equilibrium across the membrane, transport direction is driven solely by the concentration gradient. What appears to be important for intestinal fructose uptake is its metabolism in epithelial cells with the release of glucose and numerous organic acids into portal blood. This has elegantly been shown recently in mice via the use of 13C-labelled fructose(Reference Jang, Hui and Lu8). As in humans, mice show a limited absorption capacity for fructose in the small intestine, which seems to be due to a low density of GLUT5 in apical membranes compared with SGLT1, which is the electrogenic sodium-coupled GLUT for large-scale uphill transport of glucose and galactose. Limited fructose absorption causes intestinal discomfort associated with osmotic diarrhoea and fermentation by microbial communities in distal parts of the intestine leading to gas production and meteorism(Reference Jones, Butler and Brooks9). Gas production (mainly hydrogen) can be easily monitored in exhaled breath following fructose intake and a limited fructose absorption with increased gas production can be detected in many consumers at acute fructose doses exceeding 25 to 50 g. Malabsorption has become a common problem due to increased fructose intake from food and drinks over the past decades. Importantly, fructose malabsorption can be overcome by simultaneous administration of glucose. This effect is dose-dependent and fructose malabsorption is no longer detectable at a 1:1 stoichiometry in the fructose to glucose ratio(Reference Truswell, Seach and Thorburn10). This finding matches with the observation that most patients suffering from fructose malabsorption have no unpleasant intestinal effects when consuming sucrose. The underlying molecular mechanisms by which glucose enhances intestinal fructose absorption are not yet well understood but it has been postulated that glucose allows GLUT2 to integrate into the apical membrane allowing more fructose and glucose to be absorbed(Reference Kellett and Helliwell11,Reference Röder, Geillinger and Zietek12) . What is currently also unknown is whether glucose can enhance allulose absorption by the same mechanism. Allulose, like fructose, shows limited absorption capacity with doses of > 0·4 g/kg body weight/d identified as tolerable without any intestinal discomfort(Reference Han, Choi and Kim13). Overall intestinal absorption rate of allulose has been estimated to be approximately 70 % of oral dose(Reference Tsukamoto, Hossain and Yamaguchi14,Reference Moura15) . Whether more negative side effects of allulose ingestion occur when allulose is administered alone and not in the context of other dietary items (food or drinks) that also contain glucose or sucrose needs to be determined.
Similar to fructose, allulose was also shown to mediate the secretion of glucagon-like peptide 1 (GLP-1) from enteroendocrine L-cells in rats. This epithelial cell type is found throughout the entire intestine but shows highest density in the ileum and colon. GLP-1 reduces the rate of gastric emptying and is considered to maintain a vital ß-cell mass in the pancreas in addition to its acute effect on glucose-dependent insulin production. A study in C57BL/6 mice with intragastric administration of allulose at doses of 1 and 3 g per kg body weight described an increased GLP-1 secretion and a transient reduction in food intake that was no longer observed when animal lacking a GLP-1 receptor were used(Reference Hayakawa, Hira and Nakamura16). In another study in healthy and obese-diabetic animal models, allulose also elicited a GLP-1 release, reduced food intake and showed positive effects on glucose tolerance(Reference Iwasaki, Sendo and Dezaki17). Whether this metabolic activity of allulose is relevant in humans with an expected daily total intake of 10 to 30 g is not known. Moreover, hormone secretion and sensing in the intestine show remarkable differences between rodents and humans. Human studies that specifically assess responses of the gastrointestinal tract to allulose are thus needed.
Since human data on the fate (i.e. organ distribution) of absorbed allulose are not available yet, findings from animal studies and the analogy to fructose help defining the current status of the ‘knowns’ and allow to infer estimates on missing information.
Early animal studies in rats assessed the fate of allulose by using a 14C radiotracer either administered intravenously (i.v.) or orally(Reference Whistler, Singh and Lake3). Radioactivity recovery after i.v. administration revealed that > 95 % was excreted and detected in urine after 6 h only. In contrast, 72 h after oral administration, approx. 40 % of the tracer remained in the carcass, 37 % was found in urine and 15 % as carbon dioxide. The latter strongly suggests that the intestinal microbiota can metabolise allulose that reaches the large intestine, thereby releasing CO2 while 12 % of the tracer was excreted in faeces. A later study employing radiolabelled allulose in mice used autoradiography to identify the organs that retained the tracer(Reference Tsukamoto, Hossain and Yamaguchi14). Only the liver and the urinary bladder revealed significant accumulation 30 min after i.v. injection. Analysis of whole blood demonstrated rapid clearance with a half-time < 15 min. However, when analysed 7 d after the oral administration of 100 mg allulose per kg body weight, both the liver and intestine (including contents) still retained some tracer.
There is currently only one human study that reports postprandial plasma concentrations of D-allulose after oral administration when provided in a single dose of 0·5 g/kg body weight(Reference Kuzawa, Ikeda and Kagaya18). In comparison with the administration of glucose and fructose, maximal plasma levels of allulose reached almost 3 mM and increased thus similar to that of glucose but fructose levels increased only by about 300 µM. Whereas glucose levels peaked at 30 min, allulose levels reached the maximum at 1 h but remained above fasting levels for over 6 h. These findings are in line with substantial metabolism of fructose in the gut preventing major increases in peripheral blood while with lack of metabolism allulose levels mimic those of glucose – although due to insulin, glucose is more rapidly removed from blood than allulose.
The rapid clearance of molecules from the blood occurs via glomerular filtration in the kidneys and excretion via urine. However, the apical membrane of renal proximal tubular cells contains as well GLUT5, which means that there will always be a certain level of allulose that is retained in the renal tissue. Assuming that 50 to 70 % of a dose of allulose absorbed in the intestine finally appears in urine, the urogenital tract is exposed to rather high concentrations of allulose. When calculating the mean urinary concentrations of allulose at an ingested dose of 10 to 40 g per d from enriched food and drinks, allulose levels in urine may easily exceed 10 mM. The potential impact on urogenital tract infections by providing an extra substrate for bacteria has been articulated as a safety concern by experts and authorities. However, the treatment of diabetic patients with SGLT2 inhibitors for enhancing glucose excretion into urine by blocking tubular reabsorption of the filtered glucose via SGLT2 also brings glucose levels above 10 mM(Reference List and Whaley19). This had also been defined as a potential risk to promote infections in the urogenital system early in the development of SGLT2 inhibitors. However, a recent meta-analysis based on long-term observations in thousands of patients failed to find any evidence for such an increase in the incidence of urogenital system infections(Reference Dave, Schneeweiss and Kim20).
Allulose and commensal gut microbes
Due to the limited capacity of GLUT5 to transport allulose, ingested amounts not fully absorbed in the upper small intestine reach the ileum and the colon and may become metabolised by the commensal bacteria residing there. A certain level of utilisation was already inferred by the finding of CO2 production from labelled allulose in the early rodent studies aforementioned(Reference Whistler, Singh and Lake3). More systematic studies on the utilisation/fermentation of allulose in rats studied the dose dependence with 10, 20 or 30 % (w/w) of allulose in the diet followed by analysis of SCFA in cecal contents. A dose-dependent increase in the concentrations of acetate, propionate and butyrate(Reference Matsuo, Tanaka and Hashiguchi21) was observed and this profile was similar to that of other fermentable carbohydrates including fructose or fructans. Although SCFA are considered to be beneficial, studies with 13C-labelled fructose in mice(Reference Zhao, Jang and Liu22) have shown that the acetate as the dominant SCFA species of colonic fermentation can be utilised by rodent liver fatty acid synthase as a starter substrate which could be one mechanism by which high doses of fructose in rodents and humans cause non-alcoholic-fatty liver disease. But that would imply that allulose could in essence cause as well liver fat synthesis via the acetate produced by commensal bacteria. In addition, these studies also address the energetic value of allulose. Although the sugar is not metabolised in mammalian cells, the production of SCFA would provide energy to the host – similar to other fermentable substrates. However, for allulose a lower ‘caloric yield’ with about 0·4 kcal/g has been proposed(Reference Moura15) because only a fraction of the ingested dose reaches the colon and moreover, the fermentation rate of allulose in humans could be much smaller than in rodents. This is suggested by a study that used hydrogen gas analysis in breath (H2) as an indicator for fermentation in analogy to a standard fructose malabsorption test. When provided in three doses up to 0·33 g/kg body weight and compared with the same doses of fructo-oligosaccharides, there was no increase in breath H2 in case of allulose but a dose-dependent elevation in breath H2 in case of the oligofructoses(Reference Iida, Hayashi and Yamada23). The authors therefore concluded that allulose in a reasonable dose (of 23 g for a 70-kg person) does not show significant fermentation and thus does not yield energy via SCFA. Moreover, in vitro studies employing 35 bacterial strains usually present in the commensal microbiome failed to demonstrate significant fermentation of allulose when incubated for 96 h with either 0·5 % solutions of D-glucose or D-allulose followed by pH recordings as a measure of production of organic acids from the substrates(Reference Iida, Hayashi and Yamada23). No significant increase in SCFA levels in faeces was reported in mice fed a high fat (40 energy% fat) diet for 16 weeks supplemented or not with 5 % per weight of D-allulose(Reference Han, Park and Choi24). Microbiome analysis of faecal samples of the mice based on 16S rRNA gene sequence analysis revealed some alterations in microbiota composition, but these changes correlated all with the changes in body weight in the animals leaving it unanswered of whether allulose has a direct or an indirect weight-mediated effect on the microbiome. The same authors using the same diets and same design (likely from the same study) reported identical microbiome changes, but the effects on the SCFA levels were different(Reference Han, Yoon and Choi25). That partially identical data were presented in three papers and has already been identified (PubPeer: https://pubpeer.com/publications/1C5ADDF6BF9F4E555B598F39AE9B5A).
Taken together, the current knowledge base for the fate of non-absorbed allulose in rodents and humans is insufficient to allow any conclusion on whether a regular consumption of larger quantities of allulose from enriched products would cause major effects on microbiota composition or the colonic metabolite spectrum.
Allulose and pathogenic bacteria
Safety concerns were raised about allulose consumption based on reports suggesting that allulose may represent a substrate for potentially harmful bacteria(Reference Blin, Passet and Touchon26). Allulose consumption may thereby confer a growth advantage for these bacteria and enhance the incidence and/or severity of an infection(Reference Martin, Cao and Wu27).
A similar scenario had earlier been suggested for another carbohydrate, trehalose. Medically relevant strains of Clostridioides difficile, an opportunistic pathogen causing colitis mainly in elderly hospitalised patients following antibiotic therapy, were shown to metabolise trehalose(Reference Collins, Robinson and Danhof28). Based on these findings, trehalose administration in humans was proposed to confer enhanced susceptibility to C. difficile infection and promote colonisation and symptomatic infection. Subsequent studies, however, failed to confirm trehalose-induced C. difficile toxin production or a more severe course of the infection in a murine infection model and the presence of the trehalose gene cluster in C. difficile isolates was not associated with an adverse outcome in a large human cohort(Reference Eyre, Didelot and Buckley29,Reference Saund, Rao and Young30) . Thus, the ability of bacteria to metabolise a nutritional substrate does not necessarily confer an enhanced risk of infection in substrate-exposed individuals.
Likewise, the ability for allulose metabolism was shown for environmental and clinical isolates belonging to the Klebsiella pneumoniae species complex and associated with a utilisation gene cluster(Reference Blin, Passet and Touchon26,Reference Wyres, Lam and Holt31) . Notably, K. pneumoniae can use a broad spectrum of more than 100 different carbon sources and the detailed function and specificity for allulose of the eight genes encoded by an operon that would enable utilisation have not been determined. Also, allulose utilisation has not been associated with enhanced expression of virulence factors. It is rather thought to confer a competitive metabolic advantage and thus facilitate faster growth in its ecological niche or at the site of infection. K. pneumoniae is a common member of the enteric microbiota in humans and colonisation is per se not associated with an adverse outcome. Although K. pneumoniae can cause infections in healthy individuals such as, for example, urinary tract infections, the majority of K. pneumoniae infections occur in individuals with underlying diseases and in intensive care units(Reference Martin and Bachman32). Under these conditions, however, properties of K. pneumoniae such as rapid growth, the ability to become resistant to almost all antibiotics and its resistance against environmental factors play a critical role(Reference Chen, Mathema and Chavda33). Nevertheless, it cannot be excluded that renal excretion and elevated urinary concentrations of allulose promotes local growth and infection of the urinary tract(Reference Tsukamoto, Hossain and Yamaguchi14). More recently, community-acquired liver abscesses by K. pneumoniae in the absence of biliary disease in apparently immunocompetent individuals have been described particularly in the Asian/Pacific region(Reference Russo and Marr34). The underlying mechanisms have not been identified. Consumption of allulose, on the market in some countries in this region for several years, however, has so far not been linked to these infections.
Comparing K. pneumoniae isolates from infected and colonised hospitalised patients, Martin RM et al. (Reference Martin and Bachman32) found an overrepresentation of allulose utilisation cluster-positive and presumably allulose utilisation-competent strains among isolates obtained from infected individuals as compared with colonised individuals. They also noted bacterial growth in the presence of allulose and this growth was dependent on a functional permease for allulose import. Finally, using simultaneous infection of a permease-proficient and a permease-deficient K. pneumoniae strain in a competitive lung infection model, they observed a slightly enhanced organ growth of permease proficient bacteria(Reference Martin, Cao and Wu27). Based on these findings and similar to the above-mentioned trehalose/C. difficile example, allulose consumption was proposed to promote colonisation and confer enhanced susceptibility to K. pneumoniae infection. Important questions, however, remain. First, the specificity of the genes within the operon for allulose utilisation has not formally been demonstrated and the allulose concentration at different body sites is in essence unknown. Also, the K. pneumoniae abundance within the enteric microbiota following oral allulose consumption but in the notable absence of other selective factors such as antibiotic therapy requires further investigations as a potential reservoir for subsequent infection. In respect to infection, urinary tract infections may be of particular interest given the renal excretion of allulose(Reference Tsukamoto, Hossain and Yamaguchi14,Reference Iida, Hayashi and Yamada23) . However, enhanced carbohydrate concentrations in urine may not confer an increased risk of infection. Enhanced renal glucose excretion following treatment with SGLT-2 inhibitors did not lead to an enhanced incidence of urinary tract infection(Reference Dave, Schneeweiss and Kim20). Finally, the proposed benefit of allulose metabolisation for K. pneumoniae in the presence of glucose and other carbohydrates during infection at systemic body sites needs to be demonstrated. Notably, K. pneumoniae exhibits a broad core metabolism and its growth in the presence of glucose occurred much faster as with a similar concentration of allulose(Reference Martin, Cao and Wu27).
Allulose and overall metabolic health effects
The majority of published studies on allulose have focused on its putative health benefits as a sugar replacer with a negligible energetic value. Both rodent studies and also human experiments assessed the effects of allulose on short-term metabolic response such as insulin secretion and glycemic control or in medium-term studies on weight management and biomarkers of diseases. We shall be focusing on human studies since in almost all rodent studies allulose was used in doses that cannot be administered in humans (based on allometric dosing) because of intestinal discomfort and side effects that may occur at doses of > 30 g of allulose. To assess allulose effects on glycemic parameters in response to an oral glucose tolerance test with 75 g of D-glucose or defined test meals, several studies have been performed in both healthy individuals and individuals with pre-diabetes or overt type 2 diabetes. In a recent study from Canada, either 0, 5 or 10 g of allulose were added to 75-g glucose with fructose as a comparator and given to healthy individuals. There was no effect of the highest allulose dose on the AUC of the glucose profiles(Reference Noronha, Braunstein and Glenn35). The same investigators conducted a similar study in individuals with type 2 diabetes but here they found a modest effect of allulose on glycemic control while insulin levels remained unaffected(Reference Braunstein, Jarvis and Noronha36). However, in a study where 75 g of maltodextrin rather than glucose was provided to healthy individuals in the absence or the presence of 2·5, 5 or 7·5 g of allulose, a significant reduction in the AUC of blood glucose and insulin levels was observed at the two higher doses of allulose(Reference Iida, Kishimoto and Yoshikawa37). This effect was explained by a possible inhibition of the mucosal α-glucosidase by allulose but that has not been verified experimentally. A very recent study in healthy European volunteers assessed allulose effects at different doses on background of a 50-g sucrose test solution and observed a significant reduction of plasma glucose peaks at 30 min at the highest allulose doses of 7·5 and 10 g co-ingested with sucrose(Reference Franchi, Yaranov and Rollini38). However, a meta-analysis summarising the data from three human studies in Asia on allulose effects and glycemic control concluded that allulose failed to show any beneficial actions(Reference Noronha, Braunstein and Blanco Mejia39).
Allulose has been found to reduce body weight and body fat stores in a variety of animal models of obesity induced by diets or by genetic means in animals prone to obesity and diabetes. In contrast to these rodent studies, only a very few studies are available that assessed effects in humans. Significant weight loss with appropriate reduction in fat mass measured via CT scans was observed in healthy volunteers with moderate to severe overweight when receiving over 12 weeks either two times per d 4 g or 7 g of allulose provided by test drinks(Reference Han, Kwon and Yu40). As placebo served a sucralose sweetened drink. However, the effect of D-allulose on body weight was modest, with a decrease in BMI by 0·38 kg/m2 in the high-dose v. the placebo group. Blood lipid markers (lipoproteins) and markers of glycemic control (HbA1c, fasting glucose and insulin, HOMA-IR) and other biomarkers did not reveal any significant alterations. This establishes one more time that allulose effects in humans are less likely as impressive as shown in rodents in which usually unrealistic high doses of allulose are administered.
In a small clinical study in people with impaired glucose metabolism, an increase in total and LDL-cholesterol was observed after 12-week intake of 15 g D-allulose per d(Reference Tanaka, Kanasak and Hayashi41). In a subsequent randomised controlled trial in 90 individuals with moderately elevated LDL-cholesterol, the effect of long-term consumption of D-allulose for 48 weeks was studied. Participants were divided into a high-dose D-allulose (15 g/d), a low-dose D-allulose (5 g/d) and placebo subgroup. No significant alterations in total and LDL-cholesterol were observed, and long-term D-allulose consumption did not change other established cardiovascular risk factors. A modest improvement in hepatic enzyme activities and of a fatty liver score was reported. Although anthropometric data were not shown, the authors mention that changes were not clinically significant(Reference Tanaka, Kanasak and Hayashi41).
Due to the structure and absorption of D-allulose, there was interest from the beginning of human studies on the tolerability of D-allulose. In a recent systematic analysis, Han et al. studied the dose dependency of the gastrointestinal tolerability of D-allulose(Reference Han, Kwon and Yu40). When the dose of D-allulose was stepwise increased, severe diarrhoea and other GI symptoms were noted at a dose of 0·5 g/kg body weight. Therefore, the authors suggested a maximum single dose and maximum total daily dose of D-allulose of 0·4 g/kg body weight and 0·9 g/kg body weight, respectively. This aspect was also considered in the long-term trial of Tanaka et al., where the authors did not report significant differences in the incidence of adverse events between the placebo group and the D-allulose groups(Reference Tanaka, Kanasak and Hayashi41).
Summary
The knowns
Allulose can be considered as safe for human use and that is also represented by the generally recognized as safe status and safe use in a number of countries. As an epimer of D-fructose, it follows in absorption, distribution and excretion by routes and mechanisms fructose – except for lack of metabolism in mammalian cells. The tolerable dose in humans was estimated to account for 400 mg/kg body weight and single meal. The oral availability is 50 to 70 % of an oral dose with the non-absorbed fraction delivered to the microbiota. The available data and in particularly those in human studies show a very low rate of fermentation (if at all) with little SCFA production and thus a very low ‘caloric yield’. Its demonstrated small effects on body weight and body fat by otherwise minimal impact on other metabolic markers as shown in humans make it a proper sugar replacer, potentially useful in weight management.
The unknowns
There is clearly a lack of human studies that analyse allulose effects in realistic dosing regimens and with clearly defined endpoints or intermediate markers. Interesting observations from rodent studies need proof in human intervention studies such as the potential for eliciting the secretion of gastrointestinal hormones such as GLP-1. It also seems necessary to study possible protein modifications because allulose when consumed on a regular basis and in higher doses could as a reducing sugar undergo Maillard reactions known to modify proteins and other biological entities. Moreover, alterations in the gut microbiota as described in mice require careful analysis in human studies including the assessment of a possible growth advantage for pathogenic bacteria as part of a commensal microbiome or with respect to systemic effects and clinical relevance. It seems advised that such studies use other established sugar replacers like polyols or other monosaccharides as a reference.
Acknowledgements
This research received no specific grant from any funding agency, commercial or not-for-profit sectors.
All authors have contributed to writing and/or correcting sections of the manuscript with H Daniel as the main author.
There is no conflict of interest by any of the coauthors.