Introduction
Lymphatic filariasis is a neglected tropical disease that has debilitating effects on one-third of the approximately 120 million people currently infected worldwide (World Health Organization, 2011). Lymphatic filariasis spreads via parasitic nematodes that utilize a mosquito as a transmission vector and then migrate to and live in human lymphatics. Infective lymphatic filarial parasites (third-stage larvae, L3) are deposited onto human skin when the mosquito carrying them takes a blood meal. After the parasites mature and molt into fourth-stage larvae (L4) and then into sexually mature adults, they produce sheathed microfilariae that circulate in the lymph and blood stream. While infected individuals can be clinically asymptomatic, their lymphatics are dilated and have altered function (Ottesen, Reference Ottesen1994; Norões et al. Reference Norões, Addiss, Santos, Medeiros, Coutinho and Dreyer1996; Dreyer et al. Reference Dreyer, Noroes, Figueredo-Silva and Piessens2000). Those with lymphatic filariasis are at risk of developing lymphoedema or elephantiasis, morbid diseases that significantly reduce one's quality of life (Melrose, Reference Melrose2002).
To minimize disease morbidity, the Global Alliance to Eliminate Lymphatic Filariasis (GAELF) aims to eliminate the epidemic by interrupting disease transmission via mass drug administration (MDA) (World Health Organization, 2011). The standard single- and double-drug MDA have been effective at clearing microfilariae temporarily, while adult parasites appear to continue to live albeit undergo a decrease in microfilariae production (Melrose, Reference Melrose2002; Katiyar and Singh, Reference Katiyar and Singh2011; Ballesteros et al. Reference Ballesteros, Tritten, O'Neill, Burkman, Zaky, Xia, Moorhead, Williams and Geary2016a ). To reduce the need to re-dose, studies explored the sterilization of adult parasites by targeting Wolbachia, bacteria with an endosymbiotic relation with lymphatic filarial parasites (Rao, Reference Rao2005; Johnston et al. Reference Johnston, Wu, Guimarães, Ford, Slatko and Taylor2010; Lustigman et al. Reference Lustigman, Melnikow, Balakrishnan, Contreras, Nandi, Liu, Bell, Unnasch, Rogers and Ghedin2014; Taylor et al. Reference Taylor, Hoerauf, Townson, Slatko and Ward2014). Recently, a clinical trial demonstrated that a single-dose triple-drug regiment led to microfilariae-free individuals two years after dosing (Thomsen et al. Reference Thomsen, Sanuku, Baea, Satofan, Maki, Lombore, Schmidt, Siba, Weil, Kazura, Fleckenstein and King2016). Other macrofilaricides such as auranofin have also been identified through an in vitro screening process (Bulman et al. Reference Bulman, Bidlow, Lustigman, Cho-Ngwa, Williams, Rascon, Tricoche, Samje, Bell, Suzuki, Lim, Supakorndej, Supakorndej, Wolfe, Knudsen, Chen, Wilson, Ang, Arkin, Gut, Franklin, Marcellino, McKerrow, Debnath and Sakanari2015). Adult parasites and microfilariae are thought to be the central cause of pathogenic progression of lymphatic filariasis (Dreyer et al. Reference Dreyer, Noroes, Figueredo-Silva and Piessens2000; Chakraborty et al. Reference Chakraborty, Gurusamy, Zawieja and Muthuchamy2013). However, very little is known regarding the underlying biology that drives L3 migration towards lymphatic vessels. This gap is due in part to the lack of experimental model systems (in vitro and in vivo) that allow researchers to study L3 behaviour in detail. Recent advances in organ-on-a-chip approaches have allowed researchers to study physiologic systems with selected in vivo complexities with the precision and high-resolution imaging capabilities that are afforded by in vitro studies. To this end, we recently reported on a multicellular platform that allowed L3s to be co-cultured in migratory chambers in the presence of lymphatic endothelial cells and dermal fibroblasts (Kassis et al. Reference Kassis, Skelton, Lu, Moorhead and Dixon2014). While the study showed differential motility behaviour in the presence of cells, it was limited to short-term experiments due to the challenges associated with maintaining a high viability of L3s past a week or so in culture. An improved method to study these parasites in an in vitro culture environment will provide the opportunity to gain a better biological understanding of the L3 life-cycle stage and migratory behaviour which could facilitate the development of new therapies to target transmission (by eliminating the establishment of the lymphatic niche) and/or treating lymphatic filariasis-associated morbidities.
In vivo drug assays have the advantage of allowing the investigation of drug effects in their intended physiological contexts, but are low-throughput and costly (Saeung and Choochote, Reference Saeung and Choochote2013). Such an approach is more suited for the testing of a specific compound of interest (Lakshmi et al. Reference Lakshmi, Joseph, Srivastava, Verma, Sahoo, Dube, Mishra and Murthy2010; Wenkert et al. Reference Wenkert, Ramirez, Shen and Kron2010; Ceballos et al. Reference Ceballos, Mackenzie, Geary, Alvarez and Lanusse2014). On the other hand, in vitro drug assays can quickly screen for efficacy in a multitude of compounds. Unfortunately, this approach requires large quantities of parasites, which are a limited resource in the adult stage due to current methods requiring cultivation in an animal model. Several studies have explored how to successfully culture Brugia malayi from L3 to sexually mature adulthood in vitro (Riberu et al. Reference Riberu, Atmosoedjono, Purnomo, Tirtokusumo, Bangs and Baird1990; Smillie et al. Reference Smillie, Vickery, Kwa, Nayar and Rao1994; Falcone et al. Reference Falcone, Zahner, Schlaak and Haas1995). Additionally, many attempts have been made to develop an in vitro cultivation system that provides a suitable platform for the life cycle of filarial parasites, which would allow for the effective screening of new drugs (Taylor, Reference Taylor1960; Mak et al. Reference Mak, Lim, Sim and Liew1983; Saeung and Choochote, Reference Saeung and Choochote2013).
Despite several established in vitro culturing protocols, a consensus of necessary conditions for successful filarial parasite culture is absent. A variety of methods has been proposed in the literature, all with varying results and definitions of success (Table 1). Mammalian hosts are used to prime filarial parasites for a successful molt and continue to be the platform used to provide B. malayi at all life-cycle stages for research purposes (Chen and Howells, Reference Chen and Howells1979; Wisnewski and Weinstein, Reference Wisnewski and Weinstein1993). Meanwhile, reliable, animal model systems are costly and complex (Saeung and Choochote, Reference Saeung and Choochote2013). The extensive and current use of animal models indicates a deficiency in the cultivation of B. malayi in vitro (Michalski et al. Reference Michalski, Griffiths, Williams, Kaplan and Moorhead2011). Unfortunately, it is difficult to draw clear comparisons across studies due to a wide variety of approaches and conditions (Table 1). It is hypothesized that the success of in vitro culture systems is dependent on the serum source and lot. It may be possible that serum supplementation could provide some factors essential to in vitro filarial development and survival, but identification of these factors is complex. For a serum-free method, fatty acids, which can be found in fetal bovine serum (FBS), with the presence of specific species of yeast have been identified to aid in the molting process of this filarial parasite (Smith et al. Reference Smith, Paciorkowski, Babu and Rajan2000). Another group found that ascorbic acid is required for B. malayi to molt from an L3 to an L4; they hypothesize, although it was not actually tested, that ascorbic acid results in the stabilization of the collagen triple helix of the parasite, but B. malayi development and survival post-transition were not reported (Rajan et al. Reference Rajan, Paciorkowski, Kalajzic and Mcguiness2003).
Table 1. Overview of culturing methods of lymphatic filariasis parasites
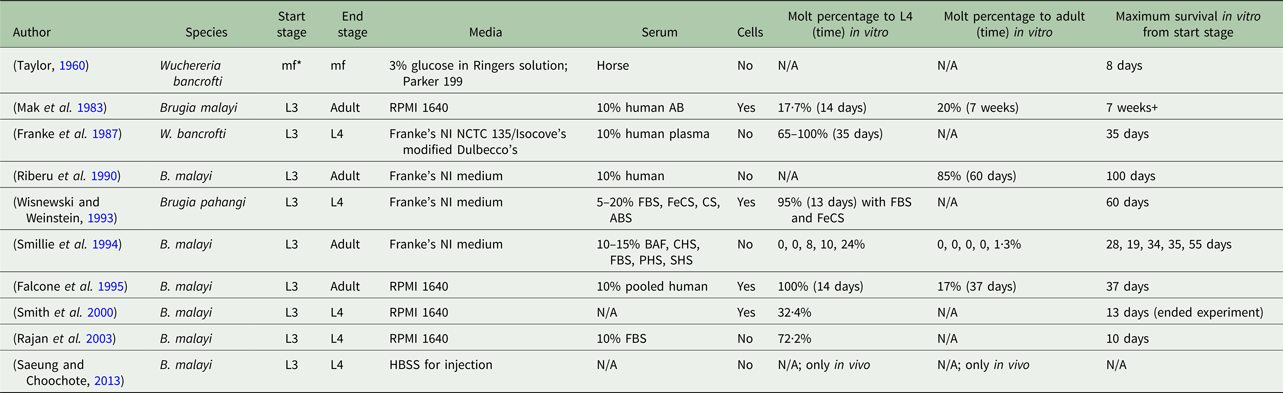
*mf, microfilariae; FBS, fetal bovine serum; FeCS, iron-supplemented calf serum; CS, calf serum; ABS, adult bovine serum; BAF, bovine albumin fraction-V; CHS, commercially obtained human serum; PHS, pooled human serum; SHS, single human serum.
Because a wide variety of non-standardized metrics has been used to quantify parasite viability, the measurements taken are inconsistent. Therefore, evaluating optimal conditions is challenging. Parasite length and width data are likely to be the most consistent metric for determining parasite growth but alone are not enough to characterize filarial health. Survival rate is a useful measurement to gauge parasite viability in culture but is seldom reported, likely due to the low yield of parasites that make it to adulthood. A measure often used for in vitro culture success is molting rate; however, the methods used for determining a molt vary, from counting the number of full cuticles to morphologic analyses of different regions of interest. Variation of parasite length and therefore shed cuticle length could lead to bias and person-to-person variation of the number of molts counted. Motility of the filarial parasite has been assessed, although the methodology is vague and varies with the person performing the assessment. Thus, the goal of this study was to identify key culture components and develop an in vitro system that provides reproducible nematode maturation quantified by easily gathered metrics, as an easy and repeatable system is more likely to lead to consistent in vitro culturing of B. malayi L3.
In this study, various chemical conditions were explored to identify key aspects necessary for successful L3 and adult B. malayi culture, while quantitatively tracking various metrics of parasite viability and maturation over one month of culture. The impact of cell culture media, serum levels, co-cultures with cells derived from the dermal layer and the lymphatics, and use of ascorbic acid were all explored. Metrics of B. malayi health were defined by (1) survival percentage, (2) molting rates from an L3 to an L4, (3) length and (4) a motility coefficient. These proposed measurements captured various aspects of a healthy nematode. This in vitro system allows not only for the successful cultivation of L3 and adult B. malayi but also has the potential to provide the foundation for the discovery of more efficient drugs to combat lymphatic filariasis.
Materials and methods
Brugia malayi receiving, cleaning and preparation for experimentation
Live B. malayi L3 were shipped overnight at ambient temperatures from the National Institutes of Health Filariasis Research Reagent Resource Center (FR3) at the University of Georgia (UGA; Athens, GA, USA) in Roswell Park Memorial Institute (RPMI) 1640 (Michalski et al. Reference Michalski, Griffiths, Williams, Kaplan and Moorhead2011). When received, the parasites were washed three times with RPMI 1640 (Lonza, NY, USA) supplemented with 10% FBS (Lots: L1106, D13057, Atlanta Biologicals, Atlanta, GA, USA) and 1% penicillin–streptomycin–amphotericin (P/S/A) (Gibco, NY, USA). Experiments began on the day B. malayi were received, and the parasites were cultured in a 37 °C incubator with 5% CO2.
Effect of media and serum concentrations on L3 survival
To determine optimal medium and FBS concentrations, B. malayi were cultured in a transwell system (Corning, NY, USA) on 12-well plates in order to simplify media changes. More specifically, the parasites were cultured in the transwell-permeable supports that had a 0·4 µm polyester membrane. Each well for the medium tests had an average of 22 parasites with a range of 6–40 parasites and for the serum tests, each well had 26 parasites with a range of 13–42 in 2 mL of total media, which was achieved by transferring 20 µL of L3s suspended in media at a concentration of 700 L3 mL−1 into the transwell. This volume distribution approached was taken as opposed to manually counting parasites per well to minimize L3 contamination but resulted in varying number of L3s per well. Each experimental group had a n = 12 performed in four independent experiments. Media changes occurred three times per week (every Monday, Wednesday and Friday) by transferring the transwell of parasites onto a new 12-well plate with 2 mL of fresh warmed media per well.
Three types of media were tested: (1) the standard culture media for B. malayi RPMI 1640 supplemented with 10% FBS (Atlanta Biologics) and 1% P/S/A (Gibco); (2) Dulbecco's Modified Eagle Medium (DMEM) (Lonza, NY, USA) supplemented with 10% FBS (Atlanta Biologics) and 1% P/S/A (Gibco); and (3) endothelial basal medium (EBM) (Lonza) supplemented with 10% FBS (Atlanta Biologics) and 1% P/S/A (Gibco). Additionally, FBS levels of 0, 10 and 20% were tested to find optimal B. malayi L3 survival. All experiments were terminated on day 13 to gather baseline survival after the L3 to L4 transition for selecting the best baseline media and serum conditions that would be used for further optimization (see Table 2). To determine survival, the number of live parasites and total parasites per well were counted and recorded. If no motion was detected for 10 s, then the parasite was scored as dead. This procedure was performed at various time points to generate the survival curve. Occasionally due to media changes, a parasite would be lost, but this loss was accounted for in the statistical analysis.
Table 2. Experimental conditions

Based on these results, the new standard medium was the EBM supplemented with 20% FBS (Atlanta Biologicals, Atlanta, GA, USA), 1% penicillin–streptomycin (P/S) (Gibco, NY, USA), 1% Glutamax, 50 µ m DBcAMP (Sigma Aldrich, St. Louis, MO, USA) and 1 µg mL−1 hydrocortisone acetate (Sigma Aldrich, St. Louis, MO, USA). These additional supplements have previously been shown to support lymphatic endothelia cell (LEC) culture, and these were used in experiments with co-culture as described in the next section (Podgrabinska et al. Reference Podgrabinska, Braun, Velasco, Kloos, Pepper and Skobe2002). Experiments were also performed to show that these additional supplements did not affect the survival of B. malayi L3 (figures not included).
Effect of co-culture with cells on L3 survival
Human dermal fibroblasts (HDFs) and LECs were used to see whether a co-culture of cells was critical for B. malayi survival. These cells were expanded in DMEM supplemented with 10% FBS (Atlanta Biologicals, Atlanta, GA, USA) and 1% P/S/A (Gibco, NY, USA). LECs were isolated from human neonatal foreskin as previously described (Podgrabinska et al. Reference Podgrabinska, Braun, Velasco, Kloos, Pepper and Skobe2002). They were expanded in EBM supplemented with 20% FBS (Atlanta Biologicals, Atlanta, GA, USA), 1% P/S/A (Gibco, NY, USA), 1% Glutamax, 50 µ m DBcAMP (Sigma Aldrich, St. Louis, MO, USA) and 1 µg mL−1 hydrocortisone acetate (Sigma Aldrich, St. Louis, MO, USA).
Each cell type was expanded in a T-75 flask coated with type I rat tail collagen solution composed of 50 µg mL−1 (BD Biosciences, San Jose, CA, USA) in 0·1% acetic acid (Sigma, St. Louis, MO, USA). Prior to reaching confluence in the T-75, cells were split and seeded at 100 000 cells per well in a 12-well plate for experiments. These cells were seeded on the bottom surface of the well (Fig. 3A). After a day of culture, the media used for all experimental groups was the fully supplemented EBM with 20% FBS levels, and the parasites were added by inserting them in the transwell (refer to Fig. 3A). We tested L3 co-culture with LECs, HDFs and no cells, and each experimental group had a n = 12 performed in four independent experiments. Each well averaged 24 parasites with a range of 13–44 parasites. The seeding of cells in each well was performed the day before the experiments started and the day before each media change. Media changes were performed every Monday, Wednesday and Friday by transferring the transwell from the old plate to a new one with freshly warmed media and the newly seeded cells at the bottom of the well, in order to ensure that the cells co-cultured with the parasites had not differentiated or died. LECs were used at passage 8–9 and HDFs at passage 13–15.
Effect of ascorbic acid on L3 health
While assessing the role of ascorbic acid on L3 B. malayi survival, the transwell system was discontinued due to the inability to image the parasites while in culture. Parasites were cultured in 12-well plates filled with 2 mL of EBM supplemented with 20% FBS (Atlanta Biologicals, Atlanta, GA, USA), 1% P/S (Gibco, NY, USA), 1% Glutamax, 50 µ m DBcAMP (Sigma Aldrich, St. Louis, MO, USA), 1 µg mL−1 hydrocortisone acetate (Sigma Aldrich, St. Louis, MO, USA) and the addition of 75 µ m ascorbic acid. Each well averaged 16 parasites with a range of 8–24 parasites. Each experimental group had a n = 12 performed in two independent experiments. Due to contamination in two wells, the final sample size analysed was n = 10. Media exchanges were performed every Monday, Wednesday and Friday by manually exchanging 75% of the medium in the well. All experiments were stopped on day 30, and all the live B. malayi for each experimental group were shipped overnight at ambient temperatures in the appropriately supplemented media to the UGA for B. malayi molting assessment. For each experimental group, all the live parasites were aggregated into a 1·5 mL centrifuge tube filled with 1 mL of supplemented EBM with 20% FBS. Groups were blinded to UGA prior to molting assessment. Every 4 days, survival of the remaining parasites was manually assessed as described above, and videos were taken for subsequent analysis, as described later in ‘Data acquisition and analysis of L3’. A random sample of three L3s was taken per well for video analysis, as it was difficult to individually image every parasite in the well.
Effect of media types and ascorbic acid on adult female health
Experiments performed on adult female B. malayi mirrored those performed on B. malayi L3. Females were chosen because they release microfilariae and are the primary target of macrofilaricides. To assess whether media type affected the in vitro survival of adult female B. malayi, the three media used were RPMI (n = 16 in two independent experiments), DMEM (n = 16) and EBM (n = 16); each were supplemented with 10% FBS (Atlanta Biologicals, Atlanta, GA, USA) and 1% P/S (Gibco, NY, USA)) to parallel the test described for L3. Later, to determine if ascorbic acid played a role in adult female B. malayi survival, the parasites were cultured in EBM supplemented with 10% FBS and 1% P/S (n = 24 in two independent experiments) and the same supplemented EBM with the addition of 75 µ m ascorbic acid (n = 24). Adult female B. malayi were cultured in a 24-well plate at a density of one parasite per well; this density allowed for a more automated assessment of survival. Each well contained 2 mL of media. Media changes occurred three times per week (every Monday, Wednesday and Friday) by manually exchanging 75% of the media in the well. Videos were taken every hour for 3 weeks and were later analysed.
A summary of the experimental conditions can be found in Table 2.
Data acquisition and analysis on L3
For this study, 10 s video segments for the B. malayi L3 were captured with a Guppy Pro camera (Allied Vision, Exton, PA, USA) at a resolution of 780 × 580 pixels and frame rate of 25 frames/s under 25 × total magnification using a Zeiss inverted microscope. After the videos were collected, they were analysed to gather information about B. malayi motility and length.
A LabVIEW programme was developed to obtain motility measurements automatically from the captured videos. The programme allows the user to specify the regions of interest (i.e. the well containing the parasites) for which they would like to obtain motility data, then the programme runs the analysis on all videos stored in the video acquisition folder; analysing all the experimental videos with only one required region of interest (ROI) setup. The region selection functionality was purposefully not automated to allow a higher level of flexibility for use in custom high-throughput screening (HTS) plates, microfluidic devices and any type of well plate. Finally, parasite motility is quantified using a Lucas–Kanade optical flow algorithm implemented in LabVIEW (National Instruments, Austin, TX, USA). All the magnitudes of the velocity vectors obtained between two subsequent frames were summed for an entire well and then averaged over the length of the video segment giving an aggregated motility metric for a particular parasite (Kassis et al. Reference Kassis, Skelton, Lu, Moorhead and Dixon2014; Schudel et al. Reference Schudel, Kassis, Dixon and Thomas2015). The motility coefficients were then normalized to the average motility coefficient (of all groups) on day 1.
Survival percentages were calculated per well. The length of B. malayi was calculated at various time points (n = 10 in two independent experiments). Using the videos, a single frame was manually isolated where the parasite is mostly ‘straight’ or uncoiled. ImageJ was used to calculate the length of the parasite.
Molting
To determine the per cent of L3s that molted at day 30, 20% of the total parasites were retrieved and the live parasites were separated and sent to the UGA for morphological analysis. Larvae in microfuge tubes were heat-killed by placing them in a heat block at 60 °C for 2 min. Slides for mounting the larvae were prepared by dispensing 200 µL of molten 5% agarose in the centre of a glass microscope slide flanked by spacer slides of slightly greater thickness. A second glass slide was quickly placed over the agar perpendicular to the slide underneath to create a thin agarose pad. Larvae were transferred to the agarose pads in small volumes of culture media (<10 µL) followed by a coverslip.
Larvae were examined at 200 × magnification under an Olympus IX51 inverted microscope and photographs were taken with a Canon Vixia HF M52 attached via a Martin Microscope adapter, model MM99-58 (Martin Microscope, Easley, SC, USA), and a Diagnostic Instruments DBX 1·0X C-mount adapter (SpotImaging, Sterling Heights, MI, USA). To distinguish the larval stage of the parasites, the anatomical features of the anterior and posterior ends were examined, previously detailed by Mak et al. (Reference Mak, Lim, Sim and Liew1983). L3 are characterized by a tapering anterior end, whereas the end of the L4 is more globular in shape. Three terminal papillae can be seen on the posterior end of the L3, while these are absent in the L4. Based on these features, larvae were classified as L3, L4 or ‘unknown’, in the case of damaged parasites or parasites of ambiguous molting status. Representative images of stages L3, L4 and unknown are found in Fig. 1A–C, respectively.

Fig. 1. Representative images of live Brugia malayi on day 13 at stages (A) L3, (B) L4 and (C) unknown. Arrows indicate features used to identify larval stage.
Imaging system and analysis of adult B. malayi
For the adult parasites, an imaging system was designed with readily available components that can be acquired from common suppliers. The resulting setup is modular in nature with each module containing two webcams (Logitech 905c Switzerland, or any compatible USB camera) incorporated within a repurposed storage drawer frame (Walmart, Bentonville, AR, USA). A Huion 17·7 inch LED light board (Amazon.com, Seattle, WA, USA) was used to illuminate the plates from above and provide contrast of the parasites for the camera. Each single module is capable of holding two cell culture plates, or any custom-made plate or device. The cameras are connected directly to a laptop that sits on top of the cell culture incubator via a USB interface (Fig. 2A–C). The modular nature of the hardware allows the setup to be scalable. In addition to the hardware, a user-friendly LabVIEW-based interface was designed to allow the user to specify the duration of the video to be captured as well as the interval between the videos. The software override the webcam's built-in autofocusing mechanism and allows the user to focus each camera individually to obtain the best image possible for each plate. Once set-up is complete, the user starts acquisition and the programme automatically acquires the videos and saves them as time/date-stamped compressed AVI files. The videos are acquired in a sequential manner, where only one camera is recording at a time. Such an implementation provides tremendous flexibility in the number of cameras connected and allows the laptop to only need the processing resources to be able to run one webcam at a time, since a typical 5 s, 960 × 720 pixel video requires around 50 megabytes of storage space.

Fig. 2. Cheap, modular imaging system is built from readily accessible parts and can be placed in an incubator creating a low-cost parasite monitoring system capable of video acquisition and quantification. (A) Overview of imaging system and components: (1) Sterilite Narrow Modular Drawer; (2) Huion LED Light Board; (3) Heavy Duty Twist Ties; (4) Logitech Webcam C905; (5) Rectangular aluminium Bar. Total cost is $97·24. (B) Setup of 24-well plates positioning on aluminium bars directly over cameras. (C) Demonstration of incubator setup showing space for additional imaging unit (dashed box), and real-time imaging setup with laptop equipped with LabVIEW (arrows). (D) Block diagram of acquisition and processing steps of the two LabVIEW-based virtual instruments (VIs). (E) Screenshot of the user interface to analyse the average motility for each parasite for each video segment in a specific region of interest.
To assess motility for adult female B. malayi, the same approach used for the L3s was implemented using a custom LabVIEW virtual instrument (VI) (Fig. 2D and E) with the exception of video post-processing. Due to some lighting and motion artefacts, the videos were post-processed in Adobe After Effects to remove both light banding (due to exposure-LED light mismatch) using a third-party plug-in (Flicker Free) and stabilized using the built-in ‘Warp Stabilizer VFX’. Stabilization was necessary in this case due to vibrations present in the incubator resulting from other concurrent experiments in the incubator. All motility coefficients were normalized to the average motility coefficients of the first day of the control group for each experiment. Parasites with a normalized motility coefficient <0·01 were considered dead as that was determined to be within the pixel noise of the system. To determine survival, the number of live parasites and total parasites per well were counted and recorded. All VIs can be found at https://github.com/TKassis.
Validation of the imaging system with SNAP
In order to demonstrate the use of the system, the effect of the nitric oxide (NO) donor S-N-acetyl D,L penicillamine (SNAP, Sigma, St. Louis, MO, USA) was quantified on parasite motility in a concentration-dependent manner. This experimental configuration has been used in the laboratory to monitor parasite motility and health over time, as well as evaluate parasite responses to anti-parasitic agents (Kassis et al. Reference Kassis, Skelton, Lu, Moorhead and Dixon2014; Schudel et al. Reference Schudel, Kassis, Dixon and Thomas2015). Freshly isolated adult female B. malayi parasites were obtained from the FR3 (Michalski et al. Reference Michalski, Griffiths, Williams, Kaplan and Moorhead2011) and underwent the wash procedure previously described. Individual parasites were subsequently plated in 2·5 mL of complete EBM with 20% FBS in a 24-well culture plate pre-incubated at 37 °C in 5% CO2 for 1 h prior to the addition of parasites. The NO donor, SNAP, was reconstituted in phosphate-buffered saline (PBS, Sigma, St. Louis, MO, USA), adjusted to the correct pH, and added to each treatment well, with PBS alone being added to the control wells. Following the addition of treatments, the parasites were incubated and 5 s video segments at 10 min intervals were recorded. Video acquisition was carried out continuously for 90 h.
Statistical analysis
The Gehan–Breslow–Wilcoxon test was used for the statistical analysis of the per cent of B. malayi survival in various conditions for both L3 and adult stages. To analyse overall differences in motility for B. malayi L3 and adults due to ascorbic acid supplementation, a Mann–Whitney test was used. A two-way analysis of variance followed by Sidak's test to correct for multiple comparisons was performed to compare between the two groups at specific time points among the three media groups for both B. malayi L3 and adults. Main group effects and time effects were analysed. All analyses were run in Prism 6 (GraphPad Software Inc, La Jolla, CA, USA) and significance was defined as P > 0·05 (not significant – ns), P ≤ 0·05 (*), P ≤ 0·01 (**), P ≤ 0·001 (***) and P ≤ 0·0001 (****). All data are presented as a mean ± s.d.
Results
Endothelial basal media with 20% FBS enhances parasite health
Measuring per cent survival after 13 days of culture allowed us to assess the baseline effects of various media and media supplement conditions on B. malayi L3. Specifically, by day 13, B. malayi L3 should have molted or attempted to molt (Mak et al. Reference Mak, Lim, Sim and Liew1983; Smith et al. Reference Smith, Paciorkowski, Babu and Rajan2000; Rajan et al. Reference Rajan, Paciorkowski, Kalajzic and Mcguiness2003); failure to successfully transition in this time frame would result in immature parasites that then die before reaching adulthood. Parasites were cultured in the medium supplemented with 10% FBS; EBM, DMEM and RPMI resulted in 65, 45 and 16% survival, respectively (Fig. 3B) (P < 0·0001). Compared with RPMI, the commonly used media for B. malayi (Smith et al. Reference Smith, Paciorkowski, Babu and Rajan2000; Rao and Well, Reference Rao and Well2002; Rajan et al. Reference Rajan, Paciorkowski, Kalajzic and Mcguiness2003; Winter et al. Reference Winter, McCormack, Myllyharju and Page2013), EBM and DMEM enhanced survival (P < 0·0001 and P = 0·0034, respectively). Next, levels of FBS were assessed in EBM, since this appeared to be the most effective medium of those tested. 20, 10 and 0% FBS in EBM led to 80 ± 12, 65 ± 9 and 11 ± 8% survival by day 13, respectively (Fig. 3C). The highest level of serum (20%) enhanced the survival of B. malayi L3 significantly when compared with 10% and 0% FBS levels (P = 0·0075 and P < 0·0001, respectively). All further experiments with L3s were carried out with 20% FBS in EBM as the baseline media.

Fig. 3. Per cent survival of Brugia malayi in various media, FBS per cent levels and cells presence. EBM with 20% FBS-cultured without cells results in enhanced per cent survival of B. malayi L3. Time points were taken at days 1, 6 and 13. (A) Schematic of the Transwell system. The cells (1) are seeded at the bottom of the well (2), while the parasites (3) are seeded in the transwell (4). (B) Per cent survival of B. malayi L3 was recorded at various time points for three different media. (C) Per cent survival of B. malayi L3 was recorded at various time points for three levels of FBS. (D) Per cent survival of B. malayi L3 was recorded at various time points for two cell lines cultured with a collagen layer, denoted as C.
Co-culture did not enhance L3 health
While it remains unclear why filarial parasites target the lymphatics when establishing a host infection, we decided to test the effects of co-culture with human LECs and HDFs as factors secreted from these cells would come in direct contact with B. malayi. L3s were cultured in the presences of either LECs, HDFs or no cells, which resulted in 86, 83 and 80% survival by day 13, respectively (Fig. 3D). Per cent survival was not significantly enhanced by the presence of human LECs or HDFs by day 13 (P = 0·5680). Because of the lack of obvious benefit of co-culture, at least over this 13-day period, long-term B. malayi L3 survival experiments were conducted without cell co-culture.
Ascorbic acid promoted L3 health
To assess B. malayi health for longer term culture using the optimized media conditions outlined above, multiple metrics (per cent survival, B. malayi length, per cent molting and motility coefficient) were tracked over the course of a month. To further enhance survival and maturation, we investigated continuous supplementation of the media with 75 µ m ascorbic acid as this concentration is similar to what is found in human serum and has been shown to aid in B. malayi L3 molting, presumably through its role in collagen synthesis and cuticle formation (Rajan et al. Reference Rajan, Paciorkowski, Kalajzic and Mcguiness2003; Schleicher et al. Reference Schleicher, Carroll, Ford and Lacher2009). Overall per cent survival was found to be significantly improved between parasites cultured with and without ascorbic acid (P < 0·0001). By day 30, per cent survival was 69 and 46% with and without ascorbic acid, respectively (Fig. 4A). On day 30, 75% of the surviving parasites had molted when ascorbic acid was present in the media, while no molts occurred in parasites cultured without ascorbic acid (Fig. 4B). On day 30, average B. malayi lengths were 1·9 ± 0·1 and 1·7 ± 0·2 mm with (n = 10) and without (n = 10) ascorbic acid, respectively (Fig. 4C). This difference was found to be significant with P = 0·0009, albeit represents a modest amount of growth. In addition to enhancing molting, the supplementation of ascorbic acid resulted in a significant increase in the motility coefficient and length of B. malayi by day 30 (P = 0·0004). Overall, the motility coefficients were significantly different between the control and ascorbic acid supplemented groups (P = 0·0142). It can be noted that this difference can be seen starting on day 14 (P = 0·0012) and persisting through the remaining time of experimentation. By day 30, the average motility coefficients for the control and ascorbic acid supplemented groups were significantly different at 0·72 and 4·49, respectively (Fig. 4D, P = 0·0004). The continuous presence of ascorbic acid led to increased B. malayi per cent survival, per cent molting, length and motility of live parasites. However, it did not produce any adult B. malayi after 30 days in culture.

Fig. 4. Continued presence of 75 µ m ascorbic acid enhances survival, motility and development of Brugia malayi L3 in vitro. When 75 µ m ascorbic acid is added to EBM over the course of 30 days in vitro, B. malayi (A) percentage survival was significantly higher with the presence of ascorbic acid. The parasite's (B) percentage molting was 100% and greater with the presence of ascorbic acid. The parasite (C) length was 1·9 ± 0·1 mm with the presence of ascorbic acid when compared with 1·7 ± 0·2 mm when cultured without ascorbic acid. Brugia malayi (D) motility is greater with the presence of ascorbic acid.
Imaging system provided a platform for adult B. malayi analysis
Having found a condition to successfully culture B. malayi L3, we were interested in whether the same conditions would have beneficial effects on the long-term culture of adult B. malayi. Given the larger size of adult parasites, we utilized a low-cost system that could be placed directly in the incubator for continuously monitoring parasite health over the entire experiment. We performed a concentration-response experiment with a NO donor to establish the sensitivity of the system to detect changes in motility. Parasite motility exhibited a concentration-dependent response to SNAP with a significant decrease in motility at 5·3 mm SNAP, which occurred within 6 h. Control parasites cultured with PBS showed no reduction in motility (Fig. 5A). From the motility data obtained, the effect of the concentration on per cent inhibition (defined as the percentage of the baseline motility each parasite exhibited) was calculated. For the two highest concentrations, 2·7 and 5·3 mm, all parasites died within 6 h of treatment (Fig. 5D). The rate of death was highly correlated with concentrations except for an unexplained middle concentration of 0·3 mm, which did not cause any death within the time course of experimentation (two independent experiments). The IC50 value was calculated at specific time points from each concentration curve (72 h, Fig. 5E) and revealed that longer exposure to the drug reduces the IC50 (Fig. 5F).

Fig. 5. Response of adult female Brugia malayi to nitric oxide. (A) The control parasites show no reduction in motility unlike parasites exposed to S-N-acetyl D,L penicillamine (SNAP) that exhibit a concentration-dependent response. (B) The rate of motility change, plotted for the first 24 h, shows that initially the lower concentrations seem to have a stimulatory effect by slightly increasing motility while the higher concentrations immediately begin to show their efficacy by reducing the motility coefficient. (C) Time of death for each parasite is concentration dependent. A SNAP concentration of 0·34 mm did not cause any parasite death. (D) Survival curve where the y-axis is the percentage of parasites that have died. (E) Concentration response at a single time point, 72 h. (F) IC50 values can be obtained from the concentration response curves and plotted as a function of incubation time clearly relating the decreased concentration required for longer incubation times.
EBM and RPMI enhanced adult female B. malayi health
After establishing the utility of the adult parasite imaging analysis system, comparisons of EBM, DMEM and RPMI supplemented with 10% FBS and 1% P/S were carried out to see if adult B. malayi responded similarly to L3s. Overall per cent survival differences among the groups were significant with P = 0·0028. Culturing the parasites in EBM, DMEM and RPMI resulted in 62·5, 18·75 and 62·5% survival, respectively, by day 13 (Fig. 6A). The last surviving parasite in each condition lasted until days 20, 16 and 24. Meanwhile, the median survivals are days 16, 11 and 17 in EBM, DMEM and RPMI, respectively. While the survival of parasites in DMEM was significantly worse than those in EBM and RPMI, there were no significant differences in survival between EBM and RPMI, which is in stark contrast to the results obtained with L3 larva. Overall, the motility coefficients in the EBM condition were significantly greater between EBM vs DMEM (P < 0·0001) and EBM vs RPMI (P < 0·0001). Furthermore, there was a significant difference in motility between DMEM and RPMI (P = 0·0009) (Fig. 6B). However, by day 14, there were no significant differences in mean motility among the groups (EBM vs DMEM, P = 0·5153; EBM vs RPMI, P = 0·9534 and DMEM vs RPMI, P = 0·2404), likely because the parasites were in very poor health for all conditions by this time point.

Fig. 6. Adult female Brugia malayi cultured in EBM, DMEM and RPMI result in differences in survival and motility. (A) Brugia malayi percentage survival by day 13 is 62·5, 18·75 and 62·5% in EBM, DMEM and RPMI, respectively. The last surviving parasite in each group lived until days 20, 16 and 24, respectively. Among the three groups, there was a significant difference. (B) Brugia malayi motility was increased when cultured in EBM, compared with DMEM and RPMI. By day 14, no differences were found in parasite motility among the three groups.
Ascorbic acid does not aid adult female B. malayi health
To determine whether ascorbic acid had an effect on the longevity of adult B. malayi in vitro, parasites were cultured with or without ascorbic acid as described above. No significant differences in survival were observed with ascorbic acid supplementation (Fig. 7A, P = 0·4599). The last surviving parasite lived until the end of the experiment at day 19 for the EBM only condition and day 18 for the EBM with ascorbic acid supplementation condition; the ones who died had a median survival of day 15 for EBM only and with ascorbic acid. The motility coefficients in the EBM only and EBM supplemented with ascorbic acid were not different (P = 0·3906).

Fig. 7. Adult female Brugia malayi cultured with 75 µ m ascorbic acid in EBM results in no difference in survival and motility. (A) Brugia malayi percentage survival began to decrease on day 10. The last surviving parasite lived until the end of the experiment for EBM only and day 18 for the EBM with ascorbic acid supplements. (B) The motility coefficient for parasites cultured with ascorbic acid was not significantly different from that of those cultured in only EBM.
Discussion
The aim of this study was to determine in vitro culture conditions for B. malayi L3 to enhance parasite health. We explored varying types of culture medium, levels of commercially available serum, co-cultures with LECs and HDFs, and the addition of ascorbic acid. In addition to current tools that look at per cent survival and molting rates, we automated the analysis of videos capturing parasite behaviour to output a parasite motility coefficient.
Performing biological studies on B. malayi in vitro has been shown to be a valuable biological tool (Ballesteros et al. Reference Ballesteros, Tritten, O'Neill, Burkman, Zaky, Xia, Moorhead, Williams and Geary2016b ). When comparing the results of our study with previous studies, certain aspects of the results are significantly improved, while previous methods have achieved better success in other aspects. A recent study has indicated that dysregulated genes appeared in the adult B. malayi when cultured in vitro indicating stress (Ballesteros et al. Reference Ballesteros, Tritten, O'Neill, Burkman, Zaky, Xia, Moorhead, Williams and Geary2016b ). Cultured in 10% FBS supplemented RPMI 1640 indicated adult parasite death by 5 days, similar to what we saw in our RMPI conditions. It is worth noting that studies which have achieved culture for periods >30 days and molting to the adult stage suffer from one of two drawbacks: the rate of total parasite viability is very low (<20%) or the success required patient-specific human serum, thus making the platform inaccessible to other researchers. Mak et al. successfully cultured adult B. malayi using RPMI with 10% human AB serum and LCC-MK2 cell co-culture. While 100% of the surviving parasites molted to L4 at 28 days and were longer than the molted parasites from our study, only 51·35% of the original parasites were still alive at this time point indicating a lower survival rate than what was achieved here. Riberu et al. (Reference Riberu, Atmosoedjono, Purnomo, Tirtokusumo, Bangs and Baird1990) was also able to grow adult B. malayi with high viability in cultures that were supplemented with human serum (Franke et al. Reference Franke, Riberu and Wiady1987), but this success was highly dependent on the individual that the serum was collected from and has been difficult to reproduce (Smillie et al. Reference Smillie, Vickery, Kwa, Nayar and Rao1994; Falcone et al. Reference Falcone, Zahner, Schlaak and Haas1995; Smith et al. Reference Smith, Paciorkowski, Babu and Rajan2000). Smillie et al. required fresh human serum to see 24% of parasites molt to L4s, with 1% of these parasites molting to a ‘young adult’. Falcone et al. were able to culture out to L4/young adult stages using RPMI 1640 with 10% human serum (pooled from three individuals) and co-cultured with Jurkat cells and fibroblasts; however, only 15% of the initial larvae were alive at this point. A variety of serum supplements, ranging from fetal bovine to human, has been used, but none has been able to consistently produce B. malayi adults with reasonable yields (Wisnewski and Weinstein, Reference Wisnewski and Weinstein1993; Smillie et al. Reference Smillie, Vickery, Kwa, Nayar and Rao1994).
Although the formula for many commercial cell culture media is proprietary, EBM is comprised of more amino acids and inorganic salts than the other media tested that may provide the necessary conditions for improved B. malayi culture. Serum also provides some vital factor(s) in L3 development. Typical cell culture of LECs uses a maximum of 20% serum supplementation. Our attempts to culture L3 in 100% serum resulted in 100% death by day 7; thus, we did not explore other serum levels. Studies suggest that cells were a requirement for healthy parasite cultivation in vitro (Mak et al. Reference Mak, Lim, Sim and Liew1983; Falcone et al. Reference Falcone, Zahner, Schlaak and Haas1995; Smith, Reference Smith2000). Our study did not show an improvement with LEC and HDF co-cultures, and this discrepancy could be explained by the supplements present in the culture conditions, which may provide the necessary factor(s) typically provided by cell types not studied in this paper. It is possible that the LECs could provide a beneficial survival factor at later stages in the life cycle; however, we did not investigate the benefits of cell co-culture beyond 13 days and thus further study is warranted in order to draw conclusions in this regard. It was advantageous, however, that the in vitro system could be simplified by removing the requirement of cells in the parasite culture by providing the appropriate medium and supplements.
Ascorbic acid plays an important role in collagen formation in that it stabilizes the collagen triple helix by regulating enzymes that hydroxylate amino acids, lysine and proline, at body temperature (37 °C) (Gerster, Reference Gerster1987). Since the cuticle of B. malayi is composed primarily of collagens, it is critical for the L3-to-L4 transition and may even be necessary for the L4-to-adult transition since B. malayi undergoes another molt to reach adult stage. Because adult parasites no longer molt, we hypothesize that the addition of ascorbic acid would not aid in survival and motility due to their lack of need for extensive collagen formation, albeit they need to produce collagen for microfilariae production. While we did see released microfilariae from the adult parasites, it is unclear whether microfilariae development occurs prior to receiving the parasites from the FR3 and we did not attempt to quantify the difference in MF production between ascorbic acid supplemented parasites and controls. We were interested in how a constant source of ascorbic acid could affect parasite health on a longer time scale at 75 µ m ascorbic acid, which is the optimal concentration and is the concentration found in human serum (Rajan et al. Reference Rajan, Paciorkowski, Kalajzic and Mcguiness2003; Schleicher et al. Reference Schleicher, Carroll, Ford and Lacher2009). The combination of ascorbic acid and serum-supplemented EBM leads to reproducible results, and this knowledge is an important step towards determining the necessary in vitro conditions for B. malayi L4 growth to adulthood. Additionally, ascorbic acid has been found to be unnecessary for Caenorhabditis elegans growth due to its cultivation at 15 °C, which is a temperature where collagen stability does not require the hydroxylation of prolines. Thus, it would be interesting to explore the role of ascorbic acid as a supplement in the cultivation of other mammalian filarial parasites in vitro (e.g. Brugia timori, Wuchereria bancrofti, Onchocerca volvulus, Litomosoides sigmodontis and Loa loa) and assess their ability to successfully molt and progress in vitro due to a more stable collagen helix (Rajan et al. Reference Rajan, Paciorkowski, Kalajzic and Mcguiness2003).
The in vitro culture conditions described in this paper are limited in that L4 B. malayi have not successfully molted and matured into adults. With our approach and experimental setup, we are not able to track individual parasite molting from L3 to L4 and motility data that would allow us to correlate molting and motility. The motility metric used to define a healthy parasite assumes live parasites are motile and therefore maintain a greater motility coefficient. Additionally, the platform to monitor adult parasites requires constant light to illuminate the plate for data collection. It has been seen that microfilariae of W. bancrofti have granules that act as photoreceptors responsible for negative phototaxis (Aoki et al. Reference Aoki, Fujimaki and Tada2011). Thus, for future works where motion behaviour is studied, the platform setup may need to be altered to ensure that natural motility behaviour is exhibited. Additionally, studies that reported cultivation of B. malayi adults all utilized human serum. Thus, in conjunction with an altered setup, future experiments would integrate our findings of a richer medium, higher serum levels and continued presence of ascorbic acid with the use of human serum to continue efforts to culture B. malayi to adulthood.
In conclusion, we have demonstrated that there is a reproducible method of culturing (1) B. malayi L3 to L4 and (2) adult female B. malayi in an in vitro environment for well over a week. Using per cent survival, per cent molting, length and motility, the health of B. malayi could be quantified. With this knowledge, we systematically determined the combination of medium, serum level and supplements that provide a high rate of molting for surviving L3s and more motile adult female parasites. This platform to culture L3s reproducibly into L4s is the first step to effectively culture B. malayi in vitro in a manner that provides a sufficient supply of adult parasites for research needs ranging from drug testing to more fundamental understanding of B. malayi behaviour.
Acknowledgements
We would like to thank the NIAID/NIH Filariasis Research Reagent Resource Center (FR3) for supplying L3 and adult female Brugia malayi. We thank Andrés García (Georgia Institute of Technology) for providing human dermal fibroblasts.
Financial support
This work was made possible by grants from the Bill and Melinda Gates Foundation's Grand Challenges Explorations Round 10 grant (OPP1087138), the American Heart Association and the National Science Foundation Graduate Research Fellowship Program under Grant No. DGE-1148903. Any opinion, findings, and conclusions or recommendations expressed in this material are those of the authors and do not necessarily reflect the views of the National Science Foundation.