INTRODUCTION
The rise of early cities and states in ancient Egypt and Mesopotamia is interwoven with the proximity of the floodplains and associated landforms of major perennial rivers. South Asia's Indus civilisation is also typically regarded as being riverine as the cities and settlements of its urban phase (ca. 2500–1900 BC) were distributed throughout much of the Indus River basin, which is watered by the Indus River and the five major rivers of Punjab. The Nile, Tigris-Euphrates, and Indus River basins are all extensive, but it is arguable that of the three, the Indus River basin is the most complex in terms of hydrology and geomorphology. The settlements of the Indus civilisation were distributed across a region that is distinctive in having considerable climatic and ecological diversity, in part because the Indus River basin straddles an environmental threshold where winter and summer rainfall systems overlap, and each has steep gradients (Fig. 1; Petrie et al., Reference Petrie, Bates, Dixit, French, Hoddell, Jones and Lancelloti2017, Reference Petrie, Parikh, Green, Bates, Frenez, Jamison, Law, Vidale and Meadow2018; Petrie and Weeks, Reference Petrie, Weeks and Chiotis2019). The distribution of the rainfall from these systems combined with the proximity of the Himalayas to the north, the Suleiman Range to the west, and the Thar Desert to the east place a range of constraints on the hydrology and geomorphology of the intermontane valleys and floodplains of the Indus River basin, which in turn affected the ways Indus civilisation populations inhabited this landscape (Petrie, Reference Petrie, Cunningham and Driessen2017; Petrie et al., Reference Petrie, Bates, Dixit, French, Hoddell, Jones and Lancelloti2017, Reference Petrie, Parikh, Green, Bates, Frenez, Jamison, Law, Vidale and Meadow2018).

Figure 1. (colour online) Map showing the distribution of settlements dating to the urban phase of the Indus civilisation, including Harappa and Mohenjo Daro, and the modern courses of the major rivers of the Indus River basin (after Petrie, Reference Petrie, Cunningham and Driessen2017).
Between 2008 and 2014, the collaborative Land, Water and Settlement project investigated long-term human and environment relationships on the plains of northwest India. These extensive plains are composed of the interfluve between the Sutlej and Yamuna Rivers and include the course (or courses) of a major palaeochannel that has been the focus of various research efforts and is often linked to the distribution of Indus civilisation settlements in the region (e.g., Lal, Reference Lal2002; Valdiya, Reference Valdiya2002; Saini et al., Reference Saini, Tandon, Mujtaba, Pant and Khorana2009; Danino, Reference Danino2010; Clift et al., Reference Clift, Carter, Giosan, Durcan, Duller, Macklin and Alizai2012; Giosan et al., Reference Giosan, Clift, Macklin, Fuller, Constantinescu, Durcan and Stevens2012; Singh et al., Reference Singh, Thomsen, Sinha, Buylaert, Carter, Mark and Mason2017). The Land, Water and Settlement project carried out a combination of extensive archaeological survey and integrated geoarchaeological analysis that demonstrated that there is not a simple correlation between visible palaeochannels and settlement location during the Indus period (Petrie et al., Reference Petrie, Bates, Dixit, French, Hoddell, Jones and Lancelloti2017; also Singh et al., Reference Singh, Petrie, French, Goudie, Gupta, Tewari and Singh2008, Reference Singh, Petrie, Singh and Singh2009, Reference Singh, Petrie, French, Neogi, Pandey, Parikh and Pawar2010a, Reference Singh, Petrie, Pawar, Pandey, Neogi, Singh, Singh, Parikh and Lancelotti2010b, Reference Singh, Petrie, Pawar, Pandey and Parikh2011, Reference Singh, Petrie, French, Bates, Pandey, Parikh, Lancelotti and Redhouse2012, Reference Singh, Petrie, Joglekar, Neogi, Lancelotti, Pandey and Pathak2013, Reference Singh, Petrie, Neogi, Pawar, Pandey, Parikh, Parmar, Singh, Tewari and Dimri2015a, Reference Singh, Petrie, Neogi, Pawar, Pandey, Parikh, Parmar, Singh, Tewari and Dimri2015b, Reference Singh, Green, Green, Ranjan, Alam and Petrie2018; Petrie et al., Reference Petrie, Singh and Singh2009, Reference Petrie, Bates, Higham and Singh2016, Reference Petrie, Parikh, Green, Bates, Frenez, Jamison, Law, Vidale and Meadow2018). This article presents a geoarchaeological analysis of the location of four rural Indus civilisation settlements situated in the distinctive alluvial environment of this region. It provides insights into the nature and chronology of landscape morphology and development, soil formation, and the types of decisions influencing populations establishing new settlements in a complex and changing alluvial environment. The analysis presented here suggests that the practices of Indus farmers were well adapted to a dynamic floodplain environment, with limited perennial water availability on the surface. When establishing new settlements, Indus populations made choices that took consideration of elevation, water access, and drainage that assured the agricultural sustainability of those settlements and also aided their resilience to a mid-Holocene climate that was variable (synchronically) within and between years and changing (diachronically) over time.
HYDROLOGY, GEOARCHAEOLOGY, AND THE INDUS CIVILISATION
During the urban phase of South Asia's Indus civilisation (ca. 2600/2500–1900 BC), settlements were distributed across an extensive area of the Indus River basin, which stretches across much of modern Pakistan and parts of western India (e.g., Wheeler, Reference Wheeler1968; Lal, Reference Lal1997; Kenoyer, Reference Kenoyer1998; Chakrabarti, Reference Chakrabarti1999; Possehl, Reference Possehl1999, Reference Possehl2002; Agrawal, Reference Agrawal2007; Wright, Reference Wright2010; Petrie, Reference Petrie and Clark2013). This extensive region is made up of a range of climate zones and geomorphological units, with the northern areas primarily being composed of the fertile alluvial plains adjacent to the Indus and the five rivers of Punjab, which stretch to the Ganges-Yamuna catchment to the east (Fig. 1).
There has been a long history of research into the hydrology and associated geomorphology of the Indus River basin, which has focused on both active rivers (e.g., Oldham, Reference Oldham1893; Pilgrim, Reference Pilgrim1919; Pascoe, Reference Pascoe1920; Fraser, Reference Fraser1958; Mithal, Reference Mithal and Law1968; Schroder, Reference Schroder1993; Meadows and Meadows, Reference Meadows and Meadows1999) and a number of major dried river channels. Palaeochannels were initially recognised on the ground in the nineteenth century (e.g., Oldham, Reference Oldham1874, Reference Oldham1893; Oldham, Reference Oldham1886), subsequently investigated using remote sensing imagery (e.g., Ghose et al., Reference Ghose, Kar and Hussain1979; Yashpal et al., Reference Yashpal, Sood and Agrawal1980; Ramasamy et al., Reference Ramasamy, Bakliwal and Verma1991; Gupta et al., Reference Gupta, Sharma, Sreenivasan and Srivastava2004; Bhadra et al., Reference Bhadra, Gupta and Sharma2009), and, more recently, reconstructed through combinations of remote sensing, coring, provenience analysis, and absolute dating (e.g., Bhadra et al., Reference Bhadra, Gupta and Sharma2009; Saini et al., Reference Saini, Tandon, Mujtaba, Pant and Khorana2009; Gupta et al., Reference Gupta, Sharma and Sreenivasan2011; Clift et al., Reference Clift, Carter, Giosan, Durcan, Duller, Macklin and Alizai2012; Giosan et al., Reference Giosan, Clift, Macklin, Fuller, Constantinescu, Durcan and Stevens2012; van Dijk et al., Reference van Dijk, Densmore, Singh, Gupta, Sinha, Mason and Joshi2016; Orengo and Petrie, Reference Orengo and Petrie2017, Reference Orengo and Petrie2018; Singh et al., Reference Singh, Thomsen, Sinha, Buylaert, Carter, Mark and Mason2017). The presence of these palaeochannels suggests that these floodplains are highly dynamic, which has major ramifications for human settlement.
The investigation of the relationship between hydrologic features and Indus civilisation settlements also has a protracted history with most attention being devoted to the environmental settings and site formation of the major urban sites of Mohenjo-Daro (e.g., Raikes, Reference Raikes1964; Lambrick, Reference Lambrick1967; Flam, Reference Flam1981, Reference Flam and Shroder1993, Reference Flam, Meadows and Meadows1999, Reference Flam, Abraham, Gullapalli, Raczek and Rizvi2013; Cucarzi, Reference Cucarzi, Jansen and Urban1984, Reference Cucarzi, Jansen and Urban1987; Raikes and Dales, Reference Raikes and Dales1986; Balista, Reference Balista, Jansen and Tosi1988; Leonardi, Reference Leonardi, Jansen and Tosi1988; Jorgensen et al., Reference Jorgensen, Harvey, Schumm, Flam and Schroder1993; Harvey and Schumm, Reference Harvey, Schumm, Meadow and Meadows1999; Jansen, Reference Jansen, Meadow and Meadows1999) and Harappa (e.g., Amundson and Pendall, Reference Amundson, Pendall and Meadow1991; Belcher, Reference Belcher, Allchin and Allchin1997, Reference Belcher1998; Belcher and Belcher, Reference Belcher and Belcher2000; Schuldenrein, Reference Schuldenrein2002; Schuldenrein et al., Reference Schuldenrein, Wright, Mughal and Khan2004, Reference Schuldenrein, Wright, Khan and Stone2007; Wright, Reference Wright2010). The analysis at these two city sites has been carried out at relatively high resolution and has detailed the specific relationship between evolving urban settlements and their distinctive and changing local landscapes.
Indus settlements were situated in a range of climatically, ecologically, and environmentally distinct locations, including intermontane valleys, on alluvial fans, at the margins or inside what are today arid zones, in areas that lack perennial rivers but are watered by monsoon rainfall, and even on islands (Wright, Reference Wright2010, pp. 33–38; Petrie and Thomas, Reference Petrie and Thomas2012; Petrie, Reference Petrie and Clark2013, Reference Petrie, Cunningham and Driessen2017; Petrie et al., Reference Petrie, Bates, Dixit, French, Hoddell, Jones and Lancelloti2017). It has been argued that Indus farmers were well adapted to diverse and variable environments, and that this helped them be resilient to climate change (Petrie, Reference Petrie, Cunningham and Driessen2017; Petrie et al., Reference Petrie, Bates, Dixit, French, Hoddell, Jones and Lancelloti2017), but geoarchaeological characterisation of settlements has only been attempted in some of these contexts. In Gujarat, there is evidence for a number of settlements on sand dunes, and the relationship between these settlements and the interdune areas suited for agricultural exploitation has been explored in some detail (e.g., Balbo et al., Reference Balbo, Rondelli, Conesa, Lancelotti, Madella and Ajithprasad2013; Conesa et al., Reference Conesa, Devanthéry, Balbo, Madella and Monserrat2014, Reference Conesa, Madella, Galiatsatos, Balbo, Rajesh and Ajithprasad2015, Reference Conesa, Lobo, Alcaina, Balbo, Madella, Rajesh and Ajithprasad2017).
In the context of the Indus civilisation, understanding the relationship between settlements and dynamic floodplains is particularly significant, and the relationship between former palaeochannels and archaeological settlement distribution has been examined in Sind (e.g., Flam, Reference Flam1981, Reference Flam and Shroder1993, Reference Flam, Abraham, Gullapalli, Raczek and Rizvi2013; Jorgensen et al., Reference Jorgensen, Harvey, Schumm, Flam and Schroder1993), Cholistan (e.g., Stein, Reference Stein1942; Mughal, Reference Mughal1997; also Geyh and Ploethner, Reference Geyh, Ploethner, Adar and Leibundgut1995), and the ancient Beas (Schuldenrein, Reference Schuldenrein2002; Schuldenrein et al., Reference Schuldenrein, Wright, Mughal and Khan2004, Reference Schuldenrein, Wright, Khan and Stone2007; Wright and Hritz, Reference Wright, Hritz, Frenez and Tosi2013) in Pakistan, and northern Rajasthan (e.g., Raikes, Reference Raikes1967; Rajani and Rajawat, Reference Rajani and Rajawat2011) and Haryana in northwest India (e.g., Courty, Reference Courty and Francfort1985, Reference Courty and Douglas1990, Reference Courty and Johnson1995; Courty et al., Reference Courty, Dhir and Raghavan1987, Reference Courty, Goldberg and Macphail1989). However, with the exception of the work along the Beas, much of this analysis been conducted at a large scale and has not involved detailed geoarchaeological analysis at specific archaeological settlements.
THE INDUS CIVILISATION AND THE ALLUVIAL FLOODPLAINS OF NORTHWEST INDIA
There were relatively few Indus settlements large enough to be regarded as cities, and each of these was situated in a different hydrologic zone and ecological context. One of these large settlements, the Indus city at Rakhigarhi (Nath, Reference Nath1998; Shinde et al., Reference Shinde, Green, Parmar and Sable2013, Reference Shinde, Kim, Woo, Jadhav, Waghmare, Yadav and Munshi2018), was situated in the central part of the extensive alluvial interfluve that lies between the Sutlej, which is the easternmost of the Punjab rivers, and the Yamuna, which is the westernmost of the major Gangetic rivers (Fig. 1). Today, this extensive plain lacks perennial rivers, but there is evidence that it has been traversed by a substantial number of ephemeral watercourses, some of which appear to have been relatively large palaeochannels (van Dijk et al., Reference van Dijk, Densmore, Singh, Gupta, Sinha, Mason and Joshi2016; Orengo and Petrie, Reference Orengo and Petrie2017, Reference Orengo and Petrie2018).
Today the Sutlej-Yamuna interfluve is an area where winter and summer rainfall systems overlap, and it is characterised by considerable variability and steep gradients in rainfall distribution, such that areas to the west and south receive little direct rainfall, whereas moving to the east and north, areas receive increasing amounts of summer rain, and in the north in particular, also winter rain (Petrie, Reference Petrie, Cunningham and Driessen2017; Petrie et al., Reference Petrie, Bates, Dixit, French, Hoddell, Jones and Lancelloti2017). A number of archaeological survey and excavation projects have demonstrated that the alluvial plains of the Sutlej-Yamuna interfluve in northwestern India were occupied throughout the late prehistoric and historic periods, including extensive occupation by Indus civilisation populations (e.g., Bhan, Reference Bhan1975; Bhan and Shaffer, Reference Bhan and Shaffer1982; Joshi et al., Reference Joshi, Bala, Ram, Lal and Gupta1984; Possehl, Reference Possehl1999; Chakrabarti and Saini, Reference Chakrabarti and Saini2009; Dangi, Reference Dangi, Osada and Uesugi2009, Reference Dangi2011; Kumar, Reference Kumar, Osada and Uesugi2009; Singh et al., Reference Singh, Petrie, Pawar, Pandey, Neogi, Singh, Singh, Parikh and Lancelotti2010b, Reference Singh, Petrie, Pawar, Pandey and Parikh2011, Reference Singh, Green, Green, Ranjan, Alam and Petrie2018; Parmar et al., Reference Parmar, Sharan, Pawar and Kumar2013; Pawar et al., Reference Pawar, Parmar and Sharan2013; Sharan et al., Reference Sharan, Pawar and Parmar2013; see Green and Petrie, Reference Petrie, Parikh, Green, Bates, Frenez, Jamison, Law, Vidale and Meadow2018). There has been a tendency to associate the distribution of Indus civilisation archaeological sites with the reconstructed courses of palaeochannels (e.g., Lal, Reference Lal2002; Valdiya, Reference Valdiya2002; Danino, Reference Danino2010; Giosan et al., Reference Giosan, Clift, Macklin, Fuller, Constantinescu, Durcan and Stevens2012; Singh et al., Reference Singh, Thomsen, Sinha, Buylaert, Carter, Mark and Mason2017). However, these relationships have not been explored in detail, they have typically been investigated at a very large scale, and the associations between the large urban centre of Rakhigarhi and the settlements in its hinterland, and the local landscape contexts and the palaeochannels that have been identified there, remain unclear.
The Land, Water and Settlement project was a collaboration between scholars from the University of Cambridge and Banaras Hindu University (2008–2014) that investigated the nature of long-term human and environment relationships on the plains of northwest India (Petrie et al., Reference Petrie, Bates, Dixit, French, Hoddell, Jones and Lancelloti2017). The project focused on the period from before the appearance of the cities of the Indus civilisation (Early Harappan: ca. 3000–2500 BC), through the period of their floruit and decline (Mature Harappan: ca. 2500–1900 BC), and the subsequent periods where rural populations made use of progressively changing assemblages of material culture (Late Harappan: ca. 1900–1600/1500 BC; Painted Grey Ware [PGW]: ca. 1500–700 BC). In addition, it also considered the Early Historic period, during which urban centres reappeared (Northern Black Polished Ware: ca. 700–200 BC; Early Historic ca. 500 BC–AD 500) and the Medieval period, when they continued to flourish (ca. AD 500–1500). Much of the research carried out by the Land, Water and Settlement project focused on the investigation of relatively small rural settlements, which appear to have housed the majority of the settled population during all three Indus periods (Madella and Fuller, Reference Madella and Fuller2006, fig. 9; Parikh and Petrie, Reference Parikh and Petrie2019). The field and laboratory work included archaeological surveys and excavations, with the latter leading to integrated archaeobotanical, archaeozoological, stable isotope, and ceramic analysis. Within the overarching research programme, geoarchaeological analysis was conducted at a number of the Indus sites and their surrounding areas in Haryana and western Uttar Pradesh (Fig. 2).
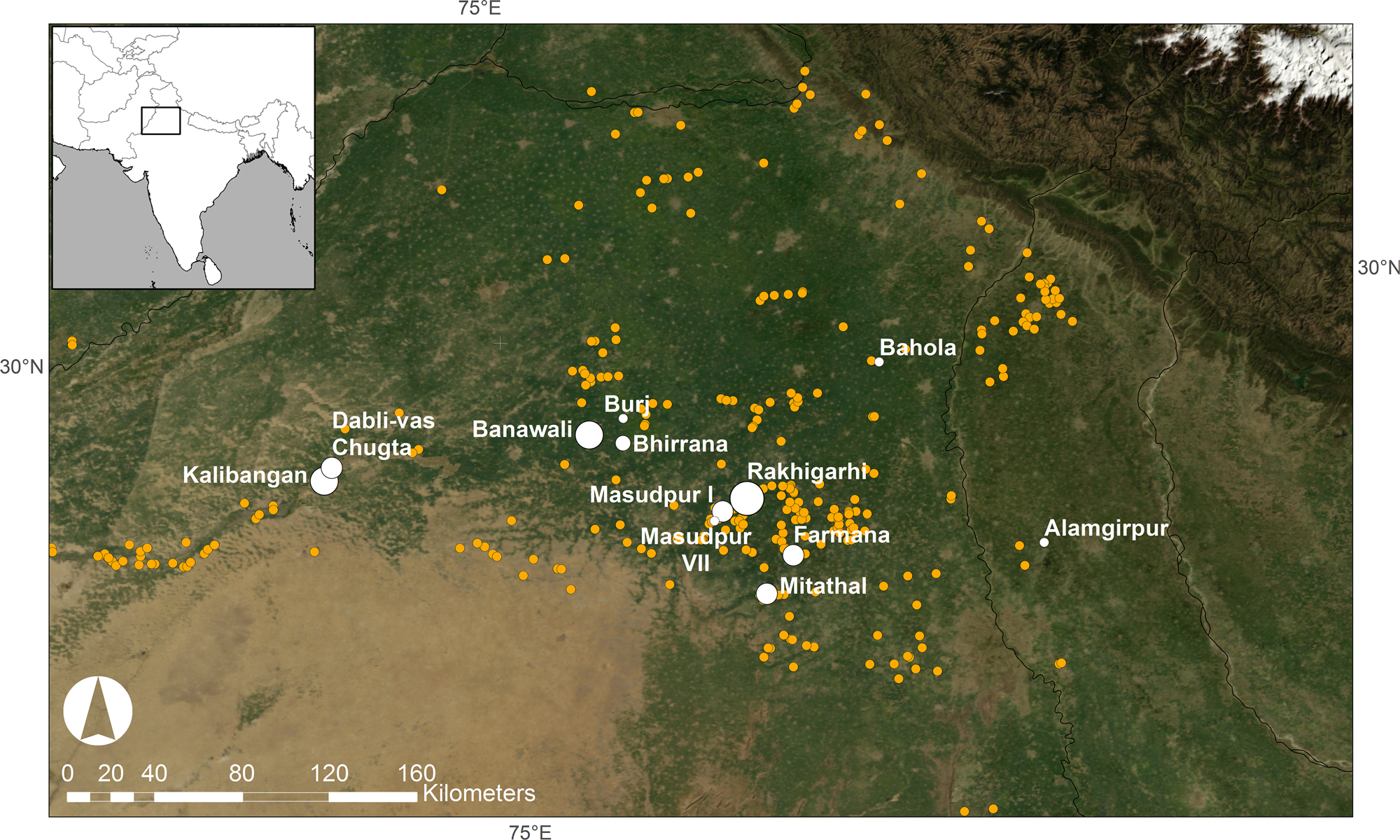
Figure 2. Map of the Sutlej-Yamuna interfluve of northwestern India, with sites studied by the Land, Water and Settlement project and other major sites in the region marked as white dots. The yellow dots show the other urban period Indus sites discovered through various surveys (map: C.A. Petrie). (For interpretation of the references to colour in this figure legend, the reader is referred to the web version of this article.)
In contrast to the diversity of the geoarchaeological research undertaken at Indus civilisation city sites (Mohenjo-Daro and Harappa), there has only been limited research on the geoarchaeology of rural or hinterland sites in Pakistan (e.g., Lahoma Lal Tibba, Chak Purbane Syal; Schuldenrein, Reference Schuldenrein2002; Schuldenrein et al., Reference Schuldenrein, Wright, Mughal and Khan2004, Reference Schuldenrein, Wright, Khan and Stone2007) and northwest India (e.g., Courty, Reference Courty and Francfort1985, Reference Courty and Douglas1990, Reference Courty and Johnson1995; Courty et al., Reference Courty, Dhir and Raghavan1987, Reference Courty, Goldberg and Macphail1989). This article presents the results and interpretations derived from sets of geoarchaeological samples recovered by the Land, Water and Settlement project from soil/sediment sequences in the immediate environs of the Indus sites of Alamgirpur (Mature Harappan, Late Harappan, PGW, and Medieval), Masudpur VII (Early Harappan, Mature Harappan, and Late Harappan), Masudpur I (Mature Harappan and Late Harappan), and Burj (Early Harappan, PGW, and Early Historic). This geoarchaeological investigation set out to gain insight into the location of settlements in the distinctive alluvial environment of northwest India, thereby helping us to understand why the people that established these settlements selected particular locations. In this regard, it was important to establish how the ancient land surfaces developed geomorphologically and pedologically and to ascertain whether there were any common attributes in terms of natural settings. It was particularly important to understand the nature of “local”-scale adaptations that Indus populations made to live in these landscapes in the third and second millennium BC.
MATERIAL AND METHODS
Soil profiles from the hinterland landscapes of each of the sites were recorded and sampled for micromorphological studies (Courty et al., Reference Courty, Goldberg and Macphail1989) and a suite of basic physical analyses (French, Reference French2015: appendix 1) (Supplementary Section 1, Supplementary Table 1). The interpretations of the soil thin sections and associated small bulk samples from individual site areas are presented subsequently, along with the results of optically stimulated luminescence (OSL) dating of samples collected from deposits immediately below the oldest occupation levels at each of these sites. Details of all of the methods used and the descriptions of the soil thin sections and associated small bulk samples are in the Supplementary Materials (see Supplementary Section 1, Supplementary Table 2).
Geoarchaeology
Geoarchaeological approaches are well established and provide very powerful tools for understanding landscape change and associated human adaptation (French, Reference French2015), particularly because of their ability to investigate and interpret environmental and cultural signatures that are typically concealed within the landscape itself (French, Reference French2003). Geoarchaeological field research is aimed primarily at gathering data with which to understand human-landscape relations (Goldberg and Macphail, Reference Goldberg and Macphail2006), and for the study presented here, attention was specifically focused on examining the nature of the land surfaces and the associated soil properties of the plains of northwest India in close proximity to archaeological settlements. The specific aim was to situate Indus archaeological sites in their local environmental contexts. In order to achieve this aim, soil surveys at and around settlement sites were carried out with the use of hand-augering and hand-cut sections to reveal alluvial/occupation/soil/subsoil sequences. At each profile location, the stratigraphy was recorded and sampled for micromorphological and other geoarchaeological analyses (see Supplementary Section 1).
In total, nine “mammoth” soil blocks from a total of 15 key soil profiles that were recorded (Supplementary Table 1) were prepared for thin section analysis at the McBurney Laboratory (Department of Archaeology, University of Cambridge) (after Murphy, Reference Murphy1986; Courty et al., Reference Courty, Goldberg and Macphail1989; Supplementary Table 2). In addition, a suite of basic physical parameters (pH, loss-on-ignition for total organic and carbon contents, magnetic susceptibility, and particle size analysis) was carried out at the Department of Geography (University of Cambridge), on a series of small bulk samples taken in conjunction with the micromorphological block samples (Supplementary Table 3; Avery and Bascomb, Reference Avery and Bascomb1974; Clark, Reference Clark1996, pp. 99–117; French, Reference French2015).
Radiocarbon dating
During the excavations of Alamgirpur, Masudpur I, Masudpur VII and Burj, bulk soil samples were collected for flotation, and the recovered material was used for archaeobotanical analysis and dating. Radiocarbon dates from Alamgirpur (Singh et al., Reference Singh, Petrie, Joglekar, Neogi, Lancelotti, Pandey and Pathak2013) and Masudpur I and Masudpur VII (Petrie et al., Reference Petrie, Bates, Higham and Singh2016) were analysed at the Oxford Radiocarbon Accelerator Unit (ORAU, RLAHA, Oxford), have already been published, and will be referred to where relevant in the subsequent text. The radiocarbon dates from Burj were also analysed at the ORAU and have not previously been published, but the relevant determination is discussed subsequently.
Luminescence dating
Samples for OSL dating were collected by hammering opaque tubes into the sediment stratigraphy, which were opened and prepared under subdued orange light conditions in the Oxford Luminescence Dating Laboratory (School of Geography and the Environment, Oxford; see Supplementary Section 1). A standard sediment preparation procedure was applied to isolate a purified quartz fraction suitable for dating. This involved the removal of carbonate and organic material using hydrochloric acid and hydrogen peroxide, followed by sieving and mineral separation using sodium polytungstate. Hydrofluoric acid was used to remove the alpha-irradiated outer layer of quartz grains, and samples were resieved prior to measurement. The OSL dates from each site are discussed in the subsequent individual sections. Single-grain OSL measurements were made using a Risø TL/OSL luminescence reader fitted with a 10 mW, 532 nm focused laser for stimulation and a 90Sr/90Y beta source (dose rate of ~4 Gy/min) for laboratory irradiation. Ultraviolet luminescence signals were detected through a bialkali photomultiplier tube fitted with 7.5 mm of U340 filters. Equivalent dose (D e) values were calculated from single grains of quartz (grain size range 150–180 µm) using the single aliquot regenerative dose (SAR) protocol (Murray and Wintle, Reference Murray and Wintle2000), with a preheat of 220°C for 10 s and cut-heat of 160°C both for 10 s, selected following combined preheat and dose recovery tests. Dose recovery tests were used to assess the suitability of the SAR protocol for D e determination. Luminescence signals were measured at 125°C for 1 s at 90% laser power and D e values were calculated from the signal measured during the first 0.05 s of stimulation, with the mean background over the last 0.2 s subtracted. Luminescence signals were screened using a standard suite of rejection criteria, and only grains that satisfied the following criteria were accepted for age calculation: (1) recycling ratio within 10% of unity, (2) OSL IR depletion ratio (Duller Reference Duller2003) within 10% of unity, (3) recuperation of less than 5%, and (4) test dose signal at least 3σ greater than background levels (Jacobs et al., Reference Jacobs, Wintle and Duller2003). Dominance of the fast component was assessed by applying the fast ratio (Durcan and Duller, Reference Durcan and Duller2011) to multi-grain quartz OSL signals. For the majority of samples, between 1500 and 3800 individual quartz grains were measured, with between 0.8% and 4.1% of grains providing luminescence signals discernible from machine background levels and that satisfied all rejection criteria. Dose distributions are moderately overdispersed (38%–47%; Table 4) for this suite of samples and are approximately symmetrical around a central value (Supplementary Fig. 1). This pattern suggests that overdispersion in the distributions is not caused by incomplete bleaching, which can be identified from skewed distributions. Instead, post-depositional factors, such as mild microdosimetric variability, as well as intrinsic intra-sample variability of quartz luminescence characteristics, are hypothesized to contribute to these spread in data. On this basis, the central age model (Galbraith et al., Reference Galbraith, Roberts, Laslett, Yoshida and Olley1999) has been used for sample D e calculation, following the approach of Durcan et al. (Reference Durcan, Thomas, Gupta, Pawar, Singh and Petrie2019).
Radionuclide concentrations were used for dose rate calculations, which were made using the dose rate calculator DRAC (v. 1.2; Durcan et al., Reference Durcan, King and Duller2015). Radioactivity was converted to dose rates using the attenuation factors of Guerin et al. (Reference Guerin, Mercier and Adamiec2011), and infinite-matrix dose rates were adjusted for attenuation by grain size, chemical etching, and moisture content (5 ± 2%). D e values and dose rates are summarised in Supplementary Table 4, along with the calculated OSL ages. Further details of the methods and the results are presented in Supplementary Table 4.
It is recognized that it is not feasible to directly compare the OSL and radiocarbon determinations (Jones, Reference Jones1999). Nonetheless, the samples for the former were collected immediately below the earliest anthropogenic deposits, and those for the latter were collected from the earliest anthropogenic deposits containing datable material from each site. The OSL dates should thus closely correspond to the latest preoccupation deposition, and the radiocarbon dates should correspond to the earliest occupation deposits.
ALAMGIRPUR
Site description
The site of Alamgirpur (Meerut district, Uttar Pradesh; Fig. 2; Supplementary Section 2.1, Supplementary Fig. 2) is the easternmost excavated Indus site, and it was established in the urban phase of the Indus civilisation and occupied during the Mature and Late Harappan, PGW, and Medieval periods (Ghosh, Reference Ghosh and Ghosh1958; Singh et al., Reference Singh, Petrie, Joglekar, Neogi, Lancelotti, Pandey and Pathak2013). The settlement is situated in a landscape composed of Quaternary alluvium to the east of the Yamuna River in the Hindon basin, which is part of the Ganges River basin (Singh, Reference Singh1996). Today, the humid subtropical climate of this region has monsoonal characteristics, and it receives an average annual rainfall of about 800 mm (Weatherbase; http://www.weatherbase.com/weather/weather.php3?s=596039cityname=Meerut- India [accessed March 20, 2017]). The modern village of Alamgirpur lies adjacent to the left bank of the Hindon River floodplain, and the archaeological mound is located ~2 km east of the current perennial river course (Supplementary Fig. 2).
The earliest deposits exposed in the YD2 and SC trenches at Alamgirpur have both been radiocarbon dated to ca. 4.3–4.0 cal ka BP (Singh et al., Reference Singh, Petrie, Joglekar, Neogi, Lancelotti, Pandey and Pathak2013, pp. 50–51, tables 10 and 11). These dates from different locations on the mound are statistically identical and internally consistent, suggesting that the mound was first occupied in the late centuries of the third millennium BC, during the Indus urban period. An OSL date (CAM-9) was obtained from the “natural” sandy silt deposit immediately beneath the archaeological deposit in the SC trench from which the radiocarbon date was obtained. The date of 4.47 ± 0.40 ka predates the radiocarbon dates and suggests that the basal deposits on which the site was established were laid down in the period immediately before it was occupied.
Sampling
During a survey conducted in December 2010, a series of five profiles were observed in and around the mound, and soil block sampling was carried out (see Supplementary Section 2.1, Supplementary Figs. 2 and 3, and Supplementary Tables 1 and 2). The positions of the profiles were chosen to characterise the geomorphological setting and development of the archaeological site. Samples for micromorphological analysis were collected from profiles 1 and 3 (434–454 and 143–153 cm below the modern ground surface, respectively), as these locations were the most likely to reveal information on the environmental conditions prior to the occupation on this mound.
Analytical results
Schematic soil columns that reconstruct the stratigraphic profiles are shown in Figure 3. Sample 1/1 (434–454 cm) is mainly an apedal sandy soil, becoming a moderately well-developed subangular blocky silty clay loam with depth (Fig. 4; Supplementary Table 2). The few fragments of clay included in this soil are likely to be the products of recycling of the much older and preexisting “B” horizon material, which further indicates disruption, local reworking, erosion, and local deposition by biological and geomorphological agents (cf. Brewer 1960; Kuhn et al., Reference Kühn, Aguilar, Miedema, Stoops, Marcelino and Mees2010). Nonetheless, the oriented pure and dusty (silty) clay coatings present in the groundmass and voids as pedofeatures and some blocky ped soil structural evidence suggest that this was a palaeosol with a reasonably well-developed clay-enriched (or Bt) horizon (cf. Fedoroff, Reference Fedoroff1968; Retallack, Reference Retallack1990; Kühn et al., Reference Kühn, Aguilar, Miedema, Stoops, Marcelino and Mees2010), indicative of at least some stabilisation and pedogenesis in the past. In particular, the common illuvial features of microlaminated pure and dusty clay striae and void coating features suggest that this soil was originally formed in a well-vegetated and stable environment (cf. Fedoroff, Reference Fedoroff1968; Retallack, Reference Retallack1990; Kühn et al., Reference Kühn, Aguilar, Miedema, Stoops, Marcelino and Mees2010), but the oxidation/gleying features suggest that this soil was seasonally wet (cf. Lindbo et al., Reference Lindbo, Stolt, Vepraskas, Stoops, Marcelino and Mees2010). The high organic content also suggests that this soil once supported a better surface vegetation (cf. Stolt and Lindbo, Reference Stolt, Lindbo, Stoops, Marcelino and Mees2010), although the organic remains have been largely replaced by amorphous iron (Fig. 5), as a result of oxidization caused by the seasonal rise and fall of the groundwater table (cf. Lindbo et al., Reference Lindbo, Stolt, Vepraskas, Stoops, Marcelino and Mees2010). These soil properties indicate that there was some initial soil development during the earlier Holocene, which then became dominated by much wetter soil conditions. In contrast, sample 3 (143–153 cm) showed a very fine sand interfacing with horizontally bedded white micaceous river sand. It is suggested that the “soil horizon” observed in this profile is a former weathered surface of a levee formed from riverine deposition of the nearby Hindon River. The ancient mound and the modern villages of Alamgirpur and Nandapura are all situated along a levee at the eastern edge of the Hindon floodplain (see Supplementary Fig. 2).

Figure 3. Schematic depiction of soil profiles at Alamgirpur (image: C.A.I. French and C.A. Petrie).

Figure 4. (colour online) Photomicrograph from Alamgirpur/off-site sample 1 showing a serrated intercalation of limpid and impure clay (ic). Notice the dominantly striated b-fabric, the calcite nodule (ca), and the calcite hypocoating (Cross Polarised Light, hereafter XPL) (photo: S. Neogi).

Figure 5. (colour online) Photomicrograph from Alamgirpur/off-site sample 1 showing a typic orthic nodule of iron oxide (n). Notice the diffused boundary (XPL) (photo: S. Neogi).
Interpretation
From the general landscape survey and detailed micromorphological analyses, it can be suggested that the current floodplain/valley edges of the Hindon River are defined by irregular but linear arrangements of sand dunes (ca. 6–8 m high), the origin of which are levees. The spatial configuration of these geomorphological features indicates that levee formations probably began to develop during the Late Quaternary, followed by cycles of soil formation and flooding. The latter process is evidenced in sample 1/1, which shows some disruption through the inclusion of fragments of a preexisting palaeosol in the form of well-developed clay fragments (Supplementary Table 2). Sample 3 represents a fluvisolic palaeosol formed from the deposition of alluvial sediments by the river. Indus period pottery fragments were found within the soil matrix, suggesting that soils were being worked in this period.
These geoarchaeological analyses highlight the formation sequence and postdepositional transformations of the early Holocene palaeosol and the alluvial sequence in the Hindon basin, adjacent to Alamgirpur. The indications of soil formation seen in the micromorphological analysis indicate that this process occurred well before the Indus populations targeted this location for permanent settlement (Fig. 3). Thus, this particular location may well have been chosen for establishing a settlement because it remained above the flooded zone and/or it had soils suitable for agricultural use.
MASUDPUR I AND MASUDPUR VII
Site descriptions
The mound sites of Masudpur I and Masudpur VII (Fig. 2; Supplementary Section 2.2 and Supplementary Fig. 4) are situated in a part of the Sutlej-Yamuna interfluve that today has a semiarid climate and is characterised by scanty and irregular rainfall, hot summers, dry cold winters, prevalent aridity, and desert and saline soils (Kottek et al., Reference Kottek, Grieser, Beck, Rudolf and Rubel2006; Petrie and Bates, Reference Petrie and Bates2017; Petrie et al., Reference Petrie, Bates, Dixit, French, Hoddell, Jones and Lancelloti2017). Masudpur I was a large village- or town-sized settlement (6–8 ha) occupied in the Mature and Late Harappan periods, and Masudpur VII was a small village-sized settlement (1 ha) occupied in the Early, Mature, and Late Harappan periods (Petrie et al., Reference Petrie, Singh and Singh2009, Reference Petrie, Bates, Higham and Singh2016; Parikh and Petrie, Reference Parikh, Petrie, Lefèvre, Didier and Mutin2016, Reference Petrie, Weeks and Chiotis2019).
Today, the area around these settlement sites consists of a flat to undulating plain partly covered with intermittent sand dunes (Petrie et al., Reference Petrie, Singh and Singh2009). Sediments have been characterised as mainly fine alluvium derived from the Himalayas with an admixture of wind-blown sand from the Thar Desert of Rajasthan, to the southwest (Courty, Reference Courty and Francfort1985; Bhatia and Kumar, Reference Bhatia and Kumar1987). It has long been argued that the alluvium was primarily deposited during the Quaternary by large rivers that have since dried up (Ahuja et al., Reference Ahuja, Khanna and Garalapun1980), but rainfall and hydrologic activity during the earlier Holocene have also had a major impact on the distribution of sediments on the floodplain.
The earliest deposits exposed in the XA1 and XM2 trenches at Masudpur I have been radiocarbon dated to ca. 4.4–4.1 cal ka BP (Petrie et al., Reference Petrie, Bates, Higham and Singh2016, table S7), suggesting that the mound was first occupied during the Indus urban period. OSL dates obtained from the “natural” sandy silt deposit immediately beneath the archaeological deposits in each of these trenches were obtained, and their ranges are 4.89 ± 0.37 ka for sample CAM-1 (Trench XA1/XM2) and 4.01 ± 0.31 ka for sample CAM-3 (Trench XA1/XM2), with the range of the latter overlapping with the earliest radiocarbon dates. The closeness of these dates suggest that the final phase of basal deposits on which the site was established were laid down shortly before that process took place.
The earliest radiocarbon date from the deposits exposed in the YA2 and YB1 trenches at Masudpur VII is ca. 4.9–4.6 cal ka BP (Petrie et al., Reference Petrie, Bates, Higham and Singh2016, table S6), suggesting that the mound was first occupied before the Indus urban period. OSL dates obtained from the “natural” sandy silt deposit immediately beneath the archaeological deposits in each of these trenches date were obtained, and their ranges are 7.32 ± 0.59 ka for sample CAM-5 (Trench YA2) and 6.47 ± 0.52 ka for sample CAM-3 (Trench YB1). The luminescence and radiocarbon dates taken together suggest that the basal deposits on which this site was established were laid down several millennia before the settlement was founded, assuming that no later sediments had been removed by natural or anthropogenic processes.
Sampling
Geoarchaeological survey of the environs of these sites was undertaken in March 2010, with one profile being recorded near Masudpur VII (profile 15) and a series of four profiles (profiles 10, 11, 12, and 13) being investigated to the north, south, and southeast of the surviving mound of Masudpur I (Supplementary Fig. 4, Supplementary Tables 1 and 3). These locations were chosen with the aim of characterising the geomorphological development of the respective archaeological sites. Masudpur VII profile 15 was observed within the exposed archaeological section of trench YB1 (Supplementary Table 1). The profile shows that the site was established on a sand dune at the terminal end of a north–south oriented chain of dunes, and there are no modern watercourses flowing in the immediate vicinity of the sampling location.
Analytical results
Schematic soil columns that reconstruct the stratigraphic profiles are shown in Figure 6. Profile 10 was exposed 100 m to the north of excavation trench XA1 at Masudpur I (see Petrie et al., Reference Petrie, Singh and Singh2009, Reference Petrie, Bates, Higham and Singh2016). Underneath ~1 m depth of Indus period archaeological deposits at profile 10, there was a 35-cm-thick older land surface comprising organic, dark greyish brown very fine sandy silt over pale brown very fine sandy silt (Supplementary Fig. 5). This stratum developed on a substrate of pale yellowish brown and calcitic very fine sandy silt with frequent calcitic nodules. Profile 11 was exposed 350 m farther to the north of profile 10 and was characterised by 1 m of brown silt over 1.5 m of yellowish/greyish brown fine sandy silt to fine-medium sand with depth. Here, no old surface or soil development was evident. Profile 12 was a well cutting, 50 m to the south of the farmstead adjacent to the settlement mound, and revealed a depth of >1.7 m of homogeneous brown very fine sandy silt with frequent pottery sherds. Profile 13, a dry well 200 m to the southeast of the farmstead recorded 2.15 m of similar homogeneous brown very fine sandy silt above a yellowish brown fine sandy silt. Samples for micromorphological analysis were collected from profiles 10 and 13 (see Supplementary Section 2.2).
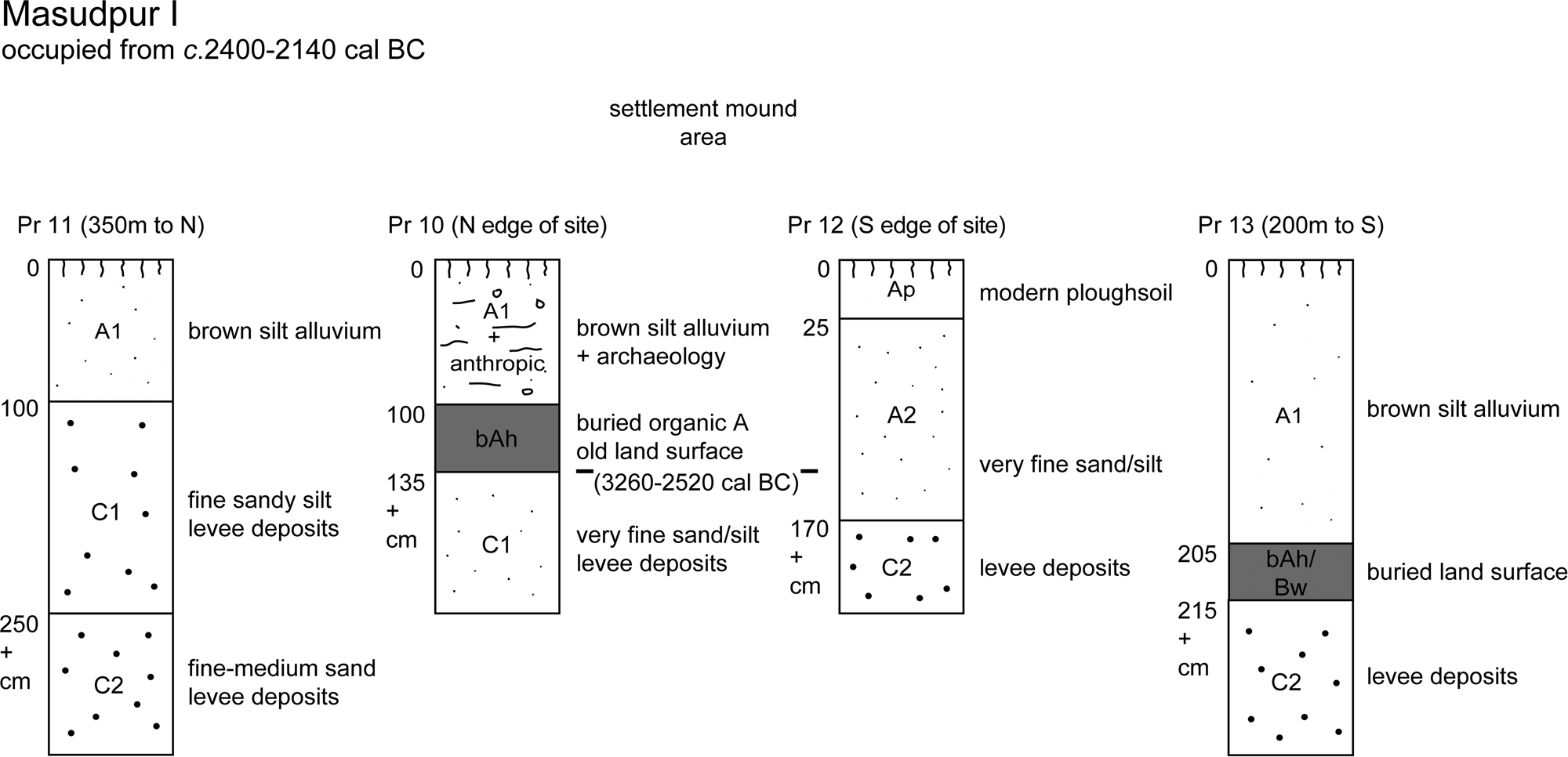
Figure 6. Schematic depiction of profiles at Masudpur I (image: C.A.I. French and C.A. Petrie).
There is relatively little textural difference between profiles 10 and 13, with sand predominating along with a considerable silt content, but relatively low values of clay present (see Supplementary Section 2.2, Supplementary Table 3). The physical characteristics of the Masudpur profiles exhibited strongly alkaline conditions (pH of 8.61 to 9.36), as well as relatively low percentages of organic (0.95%–1.26%) and calcium carbonate (2.6%–7.2%) contents and low magnetic susceptibility values (<20.3 SI/kg x10−8) (Supplementary Table 3). Nonetheless, this soil exhibits illuvial clay and dusty clay that formed coatings and striae in the subsoil horizon (Fig. 7; see Supplementary Section 2.2), much like that observed in profile 1 at Alamgirpur, thus suggesting that this soil developed under stable, well-vegetated, and well-drained conditions for a length of time prior to the combined effects of groundwater rise and fall and burial by Indus occupation deposits.
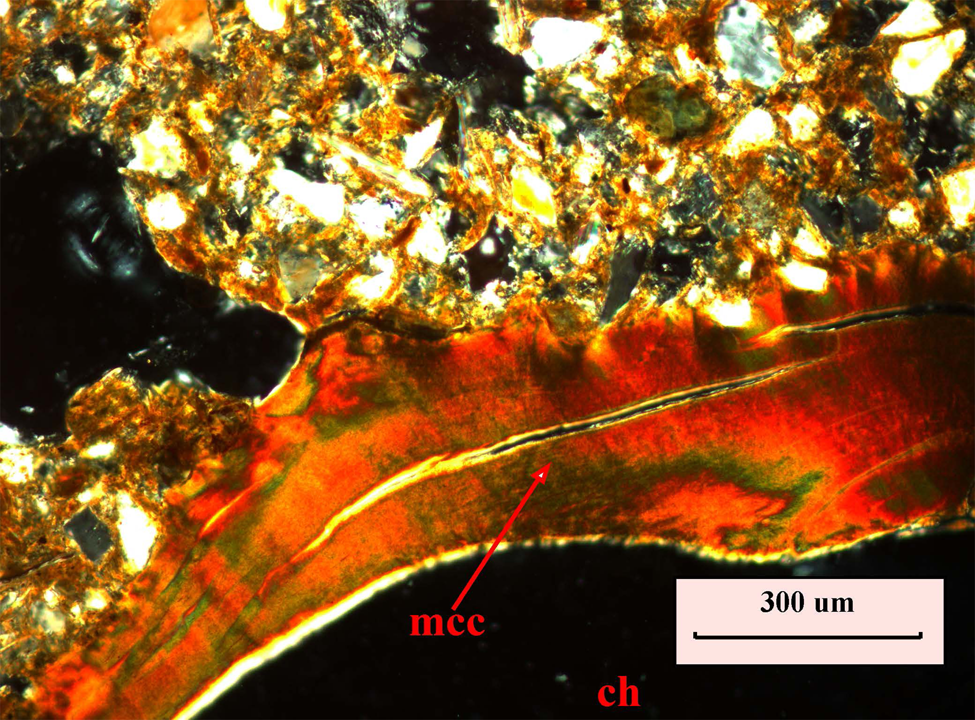
Figure 7. (colour online) Photomicrograph from Masudpur I sample 13 showing continuous coating of well-orientated, microlaminated reddish-brown clay (mcc) around a channel (ch). Note the characteristic extinction band (XPL) (photo: S. Neogi).
The surficial geology beneath Masudpur I appears to be composed of finely bedded sands that are suggestive of former channel fill deposits now surviving as low sinuous ridges (Fig. 6; also Supplementary Section 2.2). The lower-lying areas of the adjacent plain were probably more or less continually affected by the slow, seasonal deposition of alluvium from overbank flooding, presumably associated with monsoonal rains (cf. Gibling et al., Reference Gibling, Tandon, Sinha and Jain2005; French et al., Reference French, Sulas and Petrie2017). Subsequent stability in this system appears to have led to the development of a well-developed soil with organic Ah, eluvial Eb, and illuvial clay-enriched Bt horizons present (see Supplementary Section 2.2). This type of former soil (or Luvisol) would have provided excellent cultivable land for people who appear to have settled on the well-drained higher ground of the former sand bars/ridges.
Interpretation
The surrounding, more low-lying areas are likely to have received alluvial silt deposition seasonally, which would have provided both moisture and nutrients to the soil. This process might have significantly improved the fertility of these levee margin soils, by adding humic, silt, and clay contents to the fine sandy soils, thereby helping to maintain soil structure and the productive capacity of farming in this landscape. However, real improvements in fertility and crop yields would have probably required sustained additions of organic waste, minimal tillage, and multicropping regimes (Weber et al., Reference Weber, Karczewska, Drozd, Licznar, Licznar, Jamroz and Kocowicz2007; Berner et al., Reference Berner, Hildermann, Fliessbach, Pfoiffner, Niggli and Mader2008), and there is palaeobotanical evidence for multicropping practices at these sites (Petrie and Bates, Reference Petrie and Bates2017). Nonetheless, the degree of development of the soil properties shows that the soil system was stable for a relatively long period, and this could have coincided with the Indus occupational phases.
In general, the textures of the soils around the Masudpur I mound are very sandy, and they are therefore very well drained (Fig. 6). However, the micromorphological analysis at Masudpur VII suggests that the soil in that area is comparatively less sandy with a slightly loamier texture. The down-profile illuvial movement of clay and silty clays are evident in almost every thin section, albeit in relatively small amounts, indicating both phases of relative stability and some soil formation, as well as the continuing seasonal influence of alluvium additions at both of these sites.
BURJ
Site description
The site of Burj (see Fig. 2; Supplementary Section 2.3, Supplementary Fig. 6) is situated on the Sutlej-Yamuna interfluve some distance to the north of Masudpur and sits adjacent to the Ghaggar palaeochannel on the opposite side to the well-known Indus site of Kunal. Today, this region has a semiarid climate and appears to be drier than the area around Masudpur (Courty, Reference Courty and Douglas1990; Kottek et al., Reference Kottek, Grieser, Beck, Rudolf and Rubel2006; Petrie and Bates, Reference Petrie and Bates2017; Petrie et al., Reference Petrie, Bates, Dixit, French, Hoddell, Jones and Lancelloti2017).
The earliest deposits exposed at the site were in the ZG9 trench (Singh et al., Reference Singh, Petrie, French, Neogi, Pandey, Parikh and Pawar2010a), and the earliest radiocarbon determinations from there have been dated to ca. 4.8–4.5 cal ka BP (Context 216: OxA-26475, 4031 ± 34 BP), which suggests that the mound was first occupied during the Indus preurban period, though there was also evidence for occupation during the PGW period. One OSL date was obtained from the “natural” sandy silt deposit immediately beneath the archaeological deposits in a neighbouring trench, and its range was 5.48 ± 0.42 ka for sample CAM-11 (Trench ZA2). The OSL and radiocarbon dates taken together suggest that the basal deposits on which the site was established were laid down up to a millennium before the settlement was founded.
Sampling
Five profiles (profiles 1, 2, 3, 72, and 73) were recorded on and around the surviving mound at Burj, which is partially overlain by the modern village (Supplementary Section 2.3, Supplementary Fig. 6, Supplementary Tables 1–3). These profiles were selected in order to characterise the geomorphological context of soils associated with the archaeological site.
Analytical results
Schematic soil columns that reconstruct the stratigraphic profiles are shown in Figure 8. Profile 1 was located ~30 m northeast of the present-day edge of the mound and revealed that 40–65 cm of modern ploughsoil, which had recently been removed by villager quarrying, overlying a 50-cm-thick horizon of yellowish brown, very fine sandy silt, containing occasional bivalve shells, developed on a pale yellowish brown calcitic silt with irregular calcitic nodules (Supplementary Fig. 7). The bivalves have not been assessed in detail, but their presence is interesting given the settlement's proximity to the Ghaggar palaeochannel and suggests that ponding may have resulted from the flooding of parts of the surrounding landscape during periods of seasonal rain. This entire profile was cut by a substantial pit containing PGW period pottery. Profile 2 (Supplementary Fig. 8) was exposed on the northern edge of the settlement mound and had similar properties to that of profile 1, without the intrusive pit. Profile 3 was exposed 100 m to the east of the modern Sikh temple that is situated on the highest point on the mound. The upper 75 cm of this profile was composed of a homogeneous, pale brown silt with occasional pottery sherds, which developed on a 50 cm-thick horizon of horizontally banded archaeological levels of alternating dark reddish brown and pale grey silt. This deposit had in turn accumulated on a pale yellowish brown calcitic silt similar to that already seen in profiles 1 and 2. Profile 72 was cut 120 m east of trench ZA2. Archaeological deposits were found to a depth of 120 cm and overlaid pale yellowish brown sandy silt with concentrations of calcium carbonate, which may indicate the presence of a channel fill deposit. Profile 73 was located 600 m southwest of the archaeological trench of ZA2, amidst a field that was reported as being 80 cm higher some 10 yr earlier, after which time it had been levelled to reach the elevation of the surrounding fields. The profile showed 30 cm of modern topsoil over 30–60 cm of dark greyish sandy silt. Very few sherds of pottery were found from the dark greyish sandy silt, and it was situated on pale yellowish sandy silt with concentrations of CaCO3, again possibly indicative of the presence of channel fill deposits.

Figure 8. Schematic depiction of profiles at Burj (image: C.A.I. French and C.A. Petrie).
A representative set of three block samples from different depths in profile 1 were selected for micromorphological analysis (Fig. 9; Supplementary Section 2.3). The micromorphological analysis clearly exhibits the illuvial movement down-profile and the formation of pure clay and silt coatings, which suggests that pedogenesis was taking place, more or less coincident with the Indus occupation. In particular, the illuviation of pure and dusty clays is only possible when the soil pH is circum-neutral to slightly acidic, and there are stable, moist, and well-vegetated conditions over a considerable length of time, allowing an argillic (or Bt) horizon to develop (Fedoroff, Reference Fedoroff1968, Reference Fedoroff1972; Bullock and Murphy, Reference Bullock and Murphy1979; Kühn et al., Reference Kühn, Aguilar, Miedema, Stoops, Marcelino and Mees2010; IUSS Working Group WRB, 2014). In contrast, the dominant soil forming process in the region today is calcification and high alkaline pH levels, with concomitant seasonally very severe aridity and strong evaporation of soil water in the near surface soil system. These two different processes are unlikely to have developed at the same time (cf. Srivastava and Parkash, Reference Srivastava and Parkash2002). It therefore seems that the soil at Burj was in a unique state of development during the Indus period (Fig. 8), which was not analogous to today, just as has been observed at Alamgirpur and Masudpur.

Figure 9. (colour online) Photomicrograph from Burj sample 1/1 showing a passage feature (XPL) (photo: S. Neogi).
Interpretation
These relatively well-developed soils existing in river edge locations associated with several Indus sites implies a certain level of stability in the floodplain margins and a moister palaeoenvironmental regime. Additionally, the proximity of the river channels and fine overbank flooding from time to time would have continued to benefit agricultural exploitation.
DISCUSSION
This analysis of the buried soils and underlying geomorphological features related to Alamgirpur, Masudpur I, Masudpur VII, and Burj provides a number of important insights into landscape development and geomorphology on an extensive alluvial floodplain in northwest India and, particularly, the importance of this environment for Indus settlements and their hinterlands. These environments are distinct from those occupied by populations in ancient Egypt and Mesopotamia, most particularly because of the influence of both winter and summer rainfall systems and also the combination of an extensive floodplain watered by a combination of perennial and ephemeral river channels. This study highlights the fact that farmers occupying seasonally inundated alluvial plains subject to flooding are constantly faced with risks to which they must adapt. Analysis of the archaeobotanical evidence from these sites has shown that farmers made use of strategies that enabled them to exploit combinations of summer and winter crops that required differing quantities of water, suggesting an awareness of the nuances of living and farming in such landscapes (Petrie and Bates, Reference Petrie and Bates2017).
With the exception of two sites located close together (Masudpur I and VII), the ancient settlements considered in this article were situated considerable distances apart. In principle, the landscape information from each site should be treated as being relatively discrete, but importantly, they share a number of similarities. For instance, all four of these Indus settlement sites were situated on former sandy levees or riverbank deposits of Quaternary river systems, which also appear to have been active during the early Holocene. These features are evident in the geomorphic sampling locations (Fig. 10) and also in processed digital elevation models that highlight microrelief (Fig. 11). These locations were both slightly higher in elevation and also better drained than the surrounding lower-lying alluvial plain landscapes and were more likely to have remained above the floodwater levels and associated disruption during the wet season. These levees appear to have been widely targeted for both settlement and agricultural exploitation, and it is notable that this has also been observed at Harappa, which is situated in a different part of the Indus River basin (Belcher and Belcher, Reference Belcher and Belcher2000; Schuldenrein et al., Reference Schuldenrein, Wright, Mughal and Khan2004, Reference Schuldenrein, Wright, Khan and Stone2007). Importantly, these slightly elevated areas had relatively well-developed soils, unlike those in the area today, which would have been a significant beneficial factor for these settlements. In contrast, the adjacent lower-lying parts of the landscape were characterised by much finer textured soils derived from silts and clays, indicating the continuing seasonal input of fine overbank alluvial material into an aggrading floodplain system. These patterns are distinct from those seen in the lower parts of the Indus River basin, where the major river channels of Punjab have consolidated into larger channels, which produced sizable meanders and show evidence of pronounced migration over the last 4000 yr (Flam, Reference Flam and Shroder1993, Reference Flam, Meadows and Meadows1999; Schuldenrein et al., Reference Schuldenrein, Wright, Khan and Stone2007).
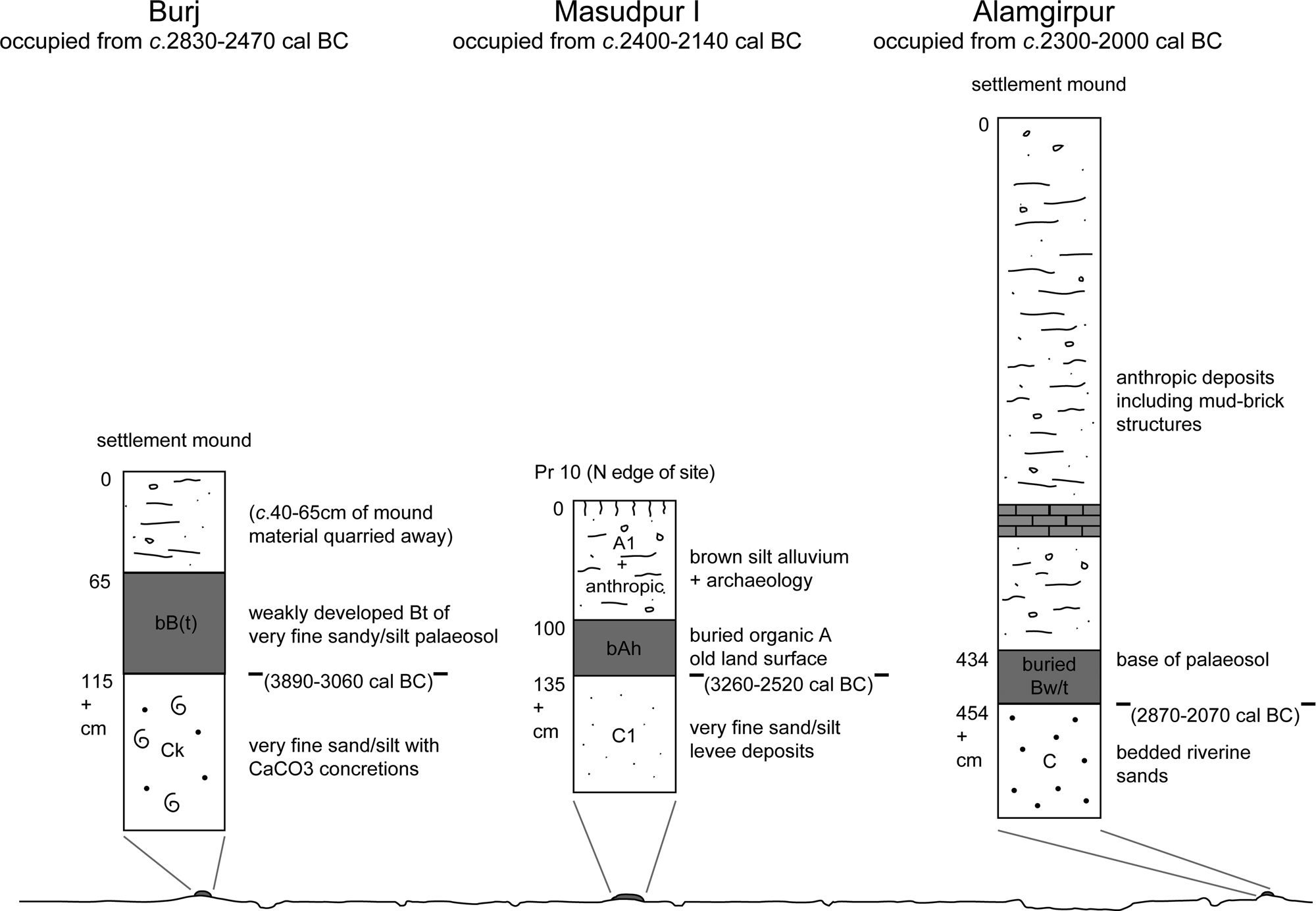
Figure 10. Schematic illustration comparing the relationship between the settlements at Burj, Masudpur I, and Alamgirpur and their underlying soil and levee deposits, above a diagrammatic representation of a section across the Sutlej-Yamuna interfluve, showing multiple channels, and settlements situated on elevated areas.
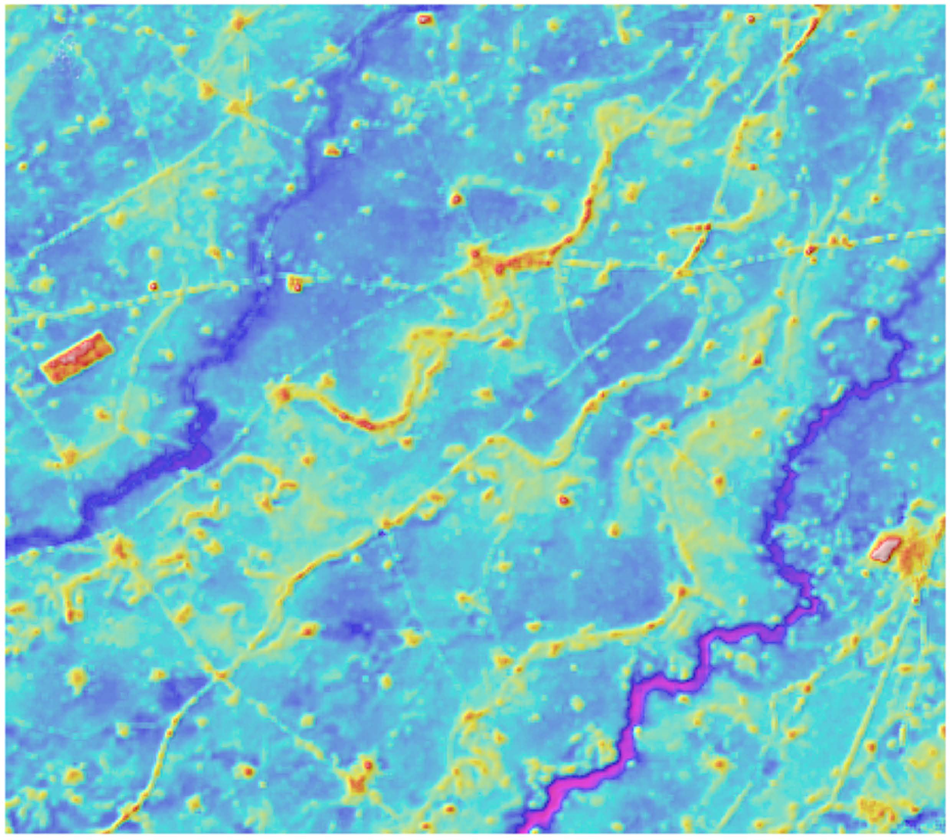
Figure 11. Detailed digital elevation model (DEM) of an area of the Sutlej-Yamuna interfluve that provides a particularly clear illustration of the plains geomorphology, highlighting the form and relative elevation of the levees (visible as yellow meanders), areas of lower terrain (in light blue), and the courses of a number of palaeochannels (in dark blue). This DEM image was produced by H. Orengo using 12 m TanDEM-X imagery (after Orengo and Petrie, Reference Orengo and Petrie2018, fig. 2). (For interpretation of the references to colour in this figure legend, the reader is referred to the web version of this article.)
The micromorphological analysis presented here demonstrates that the former land surfaces on levees and their margins in different parts of northwest India exhibited reasonably well-drained and structured soils that had undergone some pedogenesis. Importantly, these soils all contain a relatively minor but significant fine illuvial silt and clay content in the voids and groundmass, unlike the sandy subsoils beneath. The consequent silty clay “argillans” that occur in semidesert soils in this region have been interpreted as being a result of in situ weathering (Kooistra, Reference Kooistra1982) or being derived from the breakdown of fine particles related to surface crusting and seasonal floods (Courty and Fedoroff, Reference Courty and Fedoroff1985). As vegetation cover is often poor/degraded in semiarid areas, surface crusts may modify the easy downward translocation of fines into deeper horizons. Sandy soils with a single grain structure also act as a very favourable porous medium for water infiltration and percolation, especially under semiarid conditions. Suspended clay and silt are deposited in water films and preferentially deposited around sand grains by capillary action. Also, the strong adherence of clay particles to the sand grains explains the persistence of clay when these soils have been partly reworked by the wind (Wieder and Yaalon, Reference Wieder, Yaalon and Delgado1978).
These soils also exhibit more ubiquitous gleyic properties, which are normally a consequence of seasonal wetting and drying and proximity to the groundwater table (cf. Lindbo et al., Reference Lindbo, Stolt, Vepraskas, Stoops, Marcelino and Mees2010). It is difficult to be sure whether this relates to modern and/or past monsoonal flooding and alluviation, but given today's extensive network of pump irrigation and control of groundwater levels, it is unlikely that it can be ascribed solely to past causes.
Importantly, the buried palaeosols with more well-developed silt- and clay-enriched B horizons that have been observed do not show the accumulation of calcium carbonate (CaCO3), which is ubiquitous in most modern soils of this region. This signals that transpiration and evaporation did not outweigh precipitation (Durand et al., Reference Durand, Monger, Canti, Stoops, Marcelino and Mees2010). The absence of these properties in the palaeosols associated with the well-developed Bt horizons at Alamgirpur, Masudpur, and Burj suggests that the climatic and vegetational conditions at these sites were somewhat different in the past than today. These soils must therefore have been better vegetated and more moist and, consequently, under a different precipitation and groundwater regime, and thus potentially more fertile. This observation is notable as significant shifts to drier conditions in this region have been identified at different points during the Holocene (Dixit et al., Reference Dixit, Hodell and Petrie2014a, Reference Dixit, Hodell, Sinha and Petrie2014b, Reference Dixit, Hodell, Giesche, Tandon, Gázquez, Saini and Skinner2018). Climate models for the region around Harappa (Wright et al., Reference Wright, Bryson and Schuldenrein2008) and palaeoclimatic proxy records from various locations in northwest India (Dixit et al., Reference Dixit, Hodell and Petrie2014a, Reference Dixit, Hodell, Giesche, Tandon, Gázquez, Saini and Skinner2018) suggest that the mid-Holocene was characterized by relatively stronger winter and summer rainfall, and a reassessment of a sediment core off the Pakistani coast has indicated that both rainfall systems were weaker from 4.2 ka (Giesche et al., Reference Giesche, Staubwasser, Petrie and Hodell2019; also Dixit et al., Reference Dixit, Hodell and Petrie2014a). The weakening of these precipitation regimes occurred during the mid–late phases of the Indus urban period, during which Masudpur I, Masudpur VII and Alamgirpur were all occupied.
The slow-moving floodwaters associated with seasonal inundation and overbank flooding through monsoonal run-off appear to have contained clay, silt, and fine organic matter, which would have replenished the soils annually. In turn, these fine alluvial additions would have gradually altered soil textures to be finer and more moisture retentive, counteracting the detrimental effects of the free-draining sandy parent material (cf. Moody, Reference Moody and Lal2006), and thus affecting fertility positively. Furthermore, these soils could have been easily worked (cf. Greenman et al., Reference Greenman, Swarzenski and Bennett1967), even without the need for ploughing, which would facilitate simple forms of agriculture (cf. Hillel, Reference Hillel2004). Seasonal flooding would have also mitigated against water stress, especially for moisture-hungry cereal crops. Consequently, these soils that were associated with Indus urban period settlements had a degree of stability and resilience supported by annual replenishment. Thus, both the levees and their floodplain margins could have been relied on to support annually successful arable crops. It is also possible that the association of these alluviated “good” soils with large artefact scatters around these settlements may imply a form of fertilisation with domestic refuse to additionally enhance the properties of these levee soils (cf. Wilkinson, Reference Wilkinson2003, pp. 117–118).
The associated river systems and their lower-lying floodplains adjacent to the settlement sites were regularly affected by the seasonal aggradation of overbank flood deposits. These were mainly composed of fine sand and silt as soil run-off associated with monsoonal rains and riverine flooding and gradually encroached onto the soils on the margins of the higher areas of the levees. This led to some new soil processes occurring such as gleying and the secondary formation of calcium carbonate and amorphous oxides, as well as soil thickening and textural alterations with sand/silt alluvial sediments and/or the reworking and secondary deposition of channel bed derived sands. These potentially extensive “skirtland” alluviated areas around every site would have initially also provided a naturally and seasonally replenishing soil and groundwater system available for agricultural use with both nutrient and fine soil additions and a seasonally high groundwater table. Over time, these areas would have become seasonally very dry, calcitic Fluvisols and would therefore have been “too risky” for maintaining a viable cropping regime until more recent times without drainage and/or nutrient additions.
Other studies of the pre-Indus civilisation soil complexes are relatively few and far between, with the investigation of the Upper Beas palaeochannel and associated floodplain margin soils associated with the sites of Harappa, Lahoma Lal Tibba, and Chak Purbane Syal offering the most comparable analytical detail (Schuldenrein et al., Reference Schuldenrein, Wright, Mughal and Khan2004, Reference Schuldenrein, Wright, Khan and Stone2007). Essentially three phases of pre-Indus civilisation soil formation have been recognized that exhibit remarkable similarities to the picture that has emerged from our study of sites much farther to the east in the Ghaggar-Hakra valley zone of northern India. These Upper Beas soils are typically preserved on the margins of slightly higher areas of ground on channel/floodplain margins. The earlier Holocene phase (ca. 10–7 ka) saw the development of weakly developed A-B horizon soils, which show some signs of clay illuviation and overlay either Bk and/or Ck horizons with carbonate nodules, often with thick, late Quaternary alluvial or wind-blown deposits beneath. In the second period between ca. 7 and 4 ka, these soils developed further into A-B-Bwt profiles with the argillic Bt horizon exhibiting particular development. Then, just before the settlement mounds were established by about 2400–2200 cal BC, there was a more mixed and increasingly unstable picture with the first signs of overbank alluviation and channel avulsion.
Thus, there again appears to be clear evidence of the stabilization of floodplain margin landscapes with optimal climatic conditions with a stable rainfall regime and moderate evapotranspiration, which enables good soil development during the early to mid-Holocene. This was followed by some destabilisation of the environment and the development of thinner soils with channel migration just before the urban phase of the Indus civilisation.
CONCLUSIONS
This geoarchaeological study has shown that at least some and perhaps many Indus populations living on the Sutlej-Yamuna interfluve had a preference for targeting low terraces and sandbar/ridges on the margins of the ancient floodplains for the establishment of their settlements. Although the immediate environs of only four sites have been investigated, their floodplain edge locations would have reduced the risk of settlements being inundated, which would certainly have affected the adjacent alluvial plains throughout the Holocene, and are documented up to the present. Significantly, the combination of relatively good soils on these areas of higher ground and the associated naturally and seasonally replenishing alluvial soil system adjacent combined to provide a most important resource that assured agricultural sustainability. Moreover, this earlier to mid-Holocene soil development can only have been associated with a better moisture regime with less risk of drought, and relative landscape stability, which is mirrored by geoarchaeological and soil analytical studies in the Upper Beas (Schuldenrein et al., Reference Schuldenrein, Wright, Mughal and Khan2004). This combination of factors is probably the essence of the establishment and sustainability of the agricultural system in this region during the Indus periods. This type of relationship between humans, their locale, and good soil development is an important aspect of Indus cultural adaptations to diverse, variable, and changing environments through time, and it is likely that many variations on such adaptations were widespread across the Indus River basin (Petrie, Reference Petrie, Cunningham and Driessen2017; Petrie et al., Reference Petrie, Bates, Dixit, French, Hoddell, Jones and Lancelloti2017, Reference Green and Petrie2018).
Our knowledge of these landscapes will only increase with further research, and it is notable that additional samples for geoarchaeological analysis and OSL dating have been collected from buried landscapes and palaeochannels in various locations across this region (Durcan et al., Reference Durcan, Thomas, Gupta, Pawar, Singh and Petrie2019). Significantly, it appears that the more favourable and stable landscape regimes that had existed throughout much of the earlier to mid-Holocene had begun to change by ca. 4.2 ka, when Indus civilisation settlement sites were distributed across an extensive area. In the past, the landscape was more undulating, with variable soil development on the floodplain margins and levees versus the wet alluvial zones alongside former channels. The wide floodplain areas have gradually aggraded with alluvial material, and this process continued during the post-Indus and historic periods. In combination with modern levelling activities, this process has created the ostensibly flat to gently undulating surface topography that is evident today.
ACKNOWLEDGMENTS
This paper presents research carried out as part of the Land, Water and Settlement project (https://www.arch.cam.ac.uk/research/projects/land-water-settlement/), which was a collaboration between researchers at the University of Cambridge and Banaras Hindu University (BHU) in Varanasi. The field research was conducted with the permission of the Archaeological Survey of India. We would like to thank UK–India Educational Research Initiative for funding the PhD research of the first author and also supporting a number of other aspects of the Land, Water and Settlement project. Additional funding for the Land, Water and Settlement project was provided by the British Academy's Stein-Arnold Fund and the McDonald Institute for Archaeological Research. The radiocarbon dates that are reported were analysed at the Oxford Radiocarbon Accelerator Unit (ORAU, RLAHA, Oxford), with the support of several awards from NERC. The writing of this paper was made possible thanks to funding from the European Research Council under the European Union's Horizon 2020 research and innovation programme (grant agreement no. 648609) for the TwoRains project (https://www.arch.cam.ac.uk/research/projects/two-rains) and support from the DST-UKIERI programme, both of which continue the collaborative relationship between Cambridge and BHU. We would particularly like to thank A.K. Pandey from BHU and Dr. Vikas Pawar from Maharshi Dayanand University, Rohtak, for their support during the fieldwork. The laboratory analytical work was conducted in the McBurney Laboratory with particular assistance from Dr. Tonko Rajkovaca. Finally, we would like to thank Dr. Sean Taylor for reading early drafts of this paper and offering a range of helpful suggestions.
SUPPLEMENTARY MATERIAL
The supplementary material for this article can be found at https://doi.org/10.1017/qua.2019.70