Introduction
The arrival of Beaker material culture into Britain and Ireland during the twenty-fifth century bc is a significant moment, coinciding with the appearance of the first metal artefacts from around 2450 cal bc (Allen et al., Reference Allen, Gardiner and Sheridan2012). The first metal implements in Britain were made of copper, later followed by tin-bronzes around 2200–2100 cal bc. Whilst some of these objects were placed in graves, for example with the Amesbury Archer (Fitzpatrick, Reference Fitzpatrick2011) or with the Racton burial in West Sussex (Needham et al., Reference Needham, Kenny, Cole, Montgomery, Jay and Davis2017), the majority of the period's metalwork, in the form of axes, was deposited as single finds or in small hoards (Needham, Reference Needham2017). Early copper alloys within Britain and Ireland were largely derived from arsenical copper ore mined from Ross Island in south-western Ireland (O'Brien, Reference O'Brien2004), which was the source of most of the copper in Britain from c. 2400 cal bc (Bray, Reference Bray, Allen, Gardiner and Sheridan2012). Although copper mines dating to the Early Bronze Age (EBA hereafter) have been discovered in recent decades, such as Copa Hill, Cwmystwyth, in Wales, Ecton Hill and Alderley Edge in the English Peak District (Timberlake, Reference Timberlake2003, Reference Timberlake2014, Reference Timberlake2017; Timberlake & Pragg, Reference Timberlake and Pragg2005), and the largely Middle Bronze Age mine at Great Orme in Wales (Williams & Le Carlier de Veslud, Reference Williams and Le Carlier de Veslud2019), Ross Island is currently the earliest mining site known in Britain and Ireland (Carey et al., Reference Carey, Jones, Allen and Juleff2019), although some of the earliest deposits at Copa Hill could date to a similar period (Timberlake & Craddock, Reference Timberlake and Craddock2013).
Bronze is an alloy of copper and tin; though it has been postulated that tin was obtained from the rich metalliferous deposits of south-western England (Budd & Gale, Reference Budd and Gale1997; Haustein et al., Reference Haustein, Gillis and Pernicka2010), the evidence for its exploitation in the Bronze Age remains largely elusive. The numerous Middle Bronze Age artefacts recovered from tin streamworks in Cornwall provide evidence for prehistoric exploitation (Penhallurick, Reference Penhallurick1986: 173–224), and this has recently been confirmed by a date of 1620–1497 cal bc (3269 ± 27 bp, OxA-36336 at 93.9% confidence) obtained for an antler pick from the Carnon valley near Truro (Timberlake & Hartgroves, Reference Timberlake and Hartgroves2018). Furthermore, two EBA pits recently excavated in Cornwall contained several kilos of cassiterite pebbles, granules, and ore, demonstrating the collection of alluvial cassiterite for processing (see below Truro TEDC; Taylor, Reference Taylor2022).
Evidence for EBA metalworking in the south-western English peninsula, such as furnaces or ‘smithies’, has not been identified and potential data are again restricted to indirect clues including stone moulds and hammerstones (Craddock & Craddock, Reference Craddock, Craddock, Budd and Gale1997; Timberlake & Craddock, Reference Timberlake and Craddock2013; Brügmann et al., Reference Brügmann, Berger, Pernicka and Newman2017). Significantly, fragments of tin slag were recovered from an EBA barrow at Caerloggas in Cornwall (Miles, Reference Miles1975); however, smelting took place off-site, the slag having been deposited in a secondary context. Currently, the earliest in situ evidence for metalworking in southern Britain dates to the Middle Bronze Age, at Tremough (Cornwall), where moulds and a hearth indicate that bronze was cast inside a roundhouse dated to c. 1500–1300 cal bc (Jones et al., Reference Jones, Gossip and Quinnell2015; Webley et al., Reference Webley, Adams and Brück2020: 84).
This sparse evidence for the exploitation of metalliferous resources in south-western Britain and indeed western Europe (Gandois et al., Reference Gandois, Rousseau, Gehres, Le Carlier, Querré, Poissonnier and Gilbert2020) during the EBA presents a paradox, as the Cornish peninsula is rich in copper, gold, and especially tin (Penhallurick, Reference Penhallurick1986; Timberlake, Reference Timberlake2017; Radivojević et al., Reference Radivojević, Roberts, Pernicka, Stos-Gale, Rehren and Bray2018). Recent advances in analytical techniques, including isotopic analysis of artefacts and identification of potential ore sources, have revealed that traces of these metals from south-western England were probably present in Bronze Age artefacts (Rohl & Needham, Reference Rohl and Needham1998; Haustein et al., Reference Haustein, Gillis and Pernicka2010; Bray & Pollard, Reference Bray and Pollard2012). Cornish gold may have been circulated before c. 2000 cal bc to make lunulae, which are found in Ireland and Atlantic Europe (Standish et al., Reference Standish, Dhuime, Hawkesworth and Pike2015), and gold from the river Carnon, along with tin from the Redruth area, may have been used in the Nebra Sky Disc (Esher et al., Reference Esher, Borg and Pernicka2011; Borg & Pernicka, Reference Borg and Pernicka2017). We have good reason, and increasingly good secondary evidence, to believe that sources of tin and gold in south-western Britain were being exploited, but no direct indications of how or where this was happening. Understanding metalworking in this region has implications for our knowledge of this period across north-western Europe: evidence for tin exploitation is a critical missing piece of the puzzle.
Newly Discovered Sites in Cornwall
It is within the context of a lack of evidence of EBA exploitation of metalliferous resources within south-western Britain that three excavated sites in Cornwall are significant: two, Sennen and Lelant, are associated with Beaker pottery, and a third, in Truro, dates to the EBA (Figure 1). These have the capacity, as we will demonstrate, to provide new evidence for tin ore extraction and processing, increasing our understanding of the development of these traditions in Britain and their role further afield. Here we describe the new sites before outlining the analytical tools employed to document the exploitation of tin (for site descriptions contexts given as (XXX) are a deposit or fill, and those given as [XXX] are cut features).
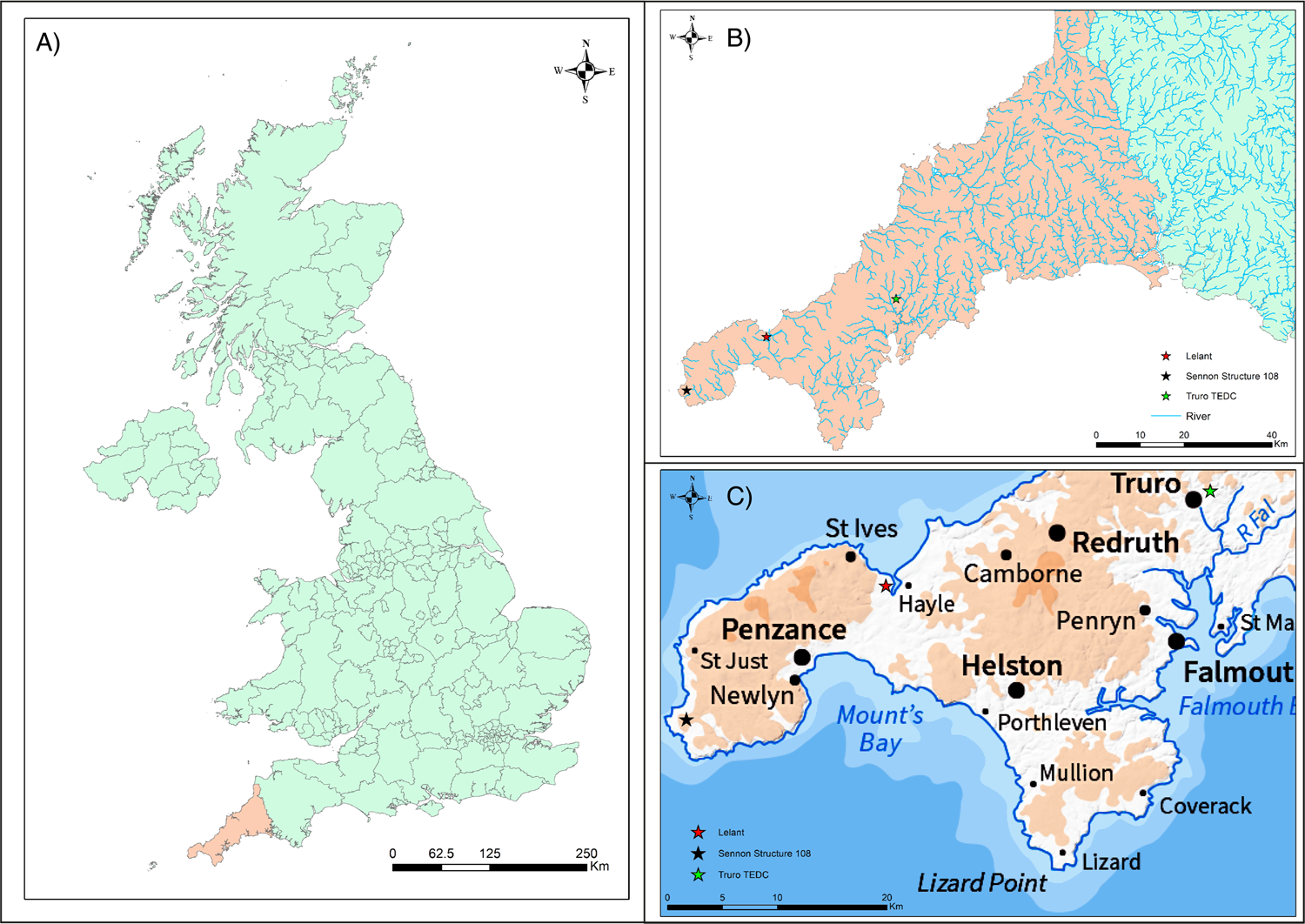
Figure 1. Location of the two Beaker-period and one Early Bronze Age archaeological sites at national (A), regional (B), and sub-regional (C) scales.
Sennen PS07: Structure 108
The excavation of an EBA site at Sennen identified Structure 108, an irregular oval feature measuring 4.2 × 3 m aligned on a north-east to south-west axis (Jones et al., Reference Jones, Taylor and Sturgess2012; Figure 2). The interior was deliberately sunken (by 0.25 m) and around the perimeter were at least eight small postholes, potentially supporting a superstructure. An area of burning indicated a possible internal hearth (103), 1.1 × 0.85 m, located in a slight hollow in the floor in the structure's south-western half. A single, deliberate, backfill (89) sealed the structure and contained many artefacts, including Beaker pottery, twenty-one flints (six waste pieces, one split pebble and fourteen tools, including an engraver that was probably hafted), and thirteen stone tools, including SF2, SF3, and SF5, discussed below. Context (89) dated to 2337–2059 cal bc (SUERC-21077, 3785 ± 30 bp, 95%).
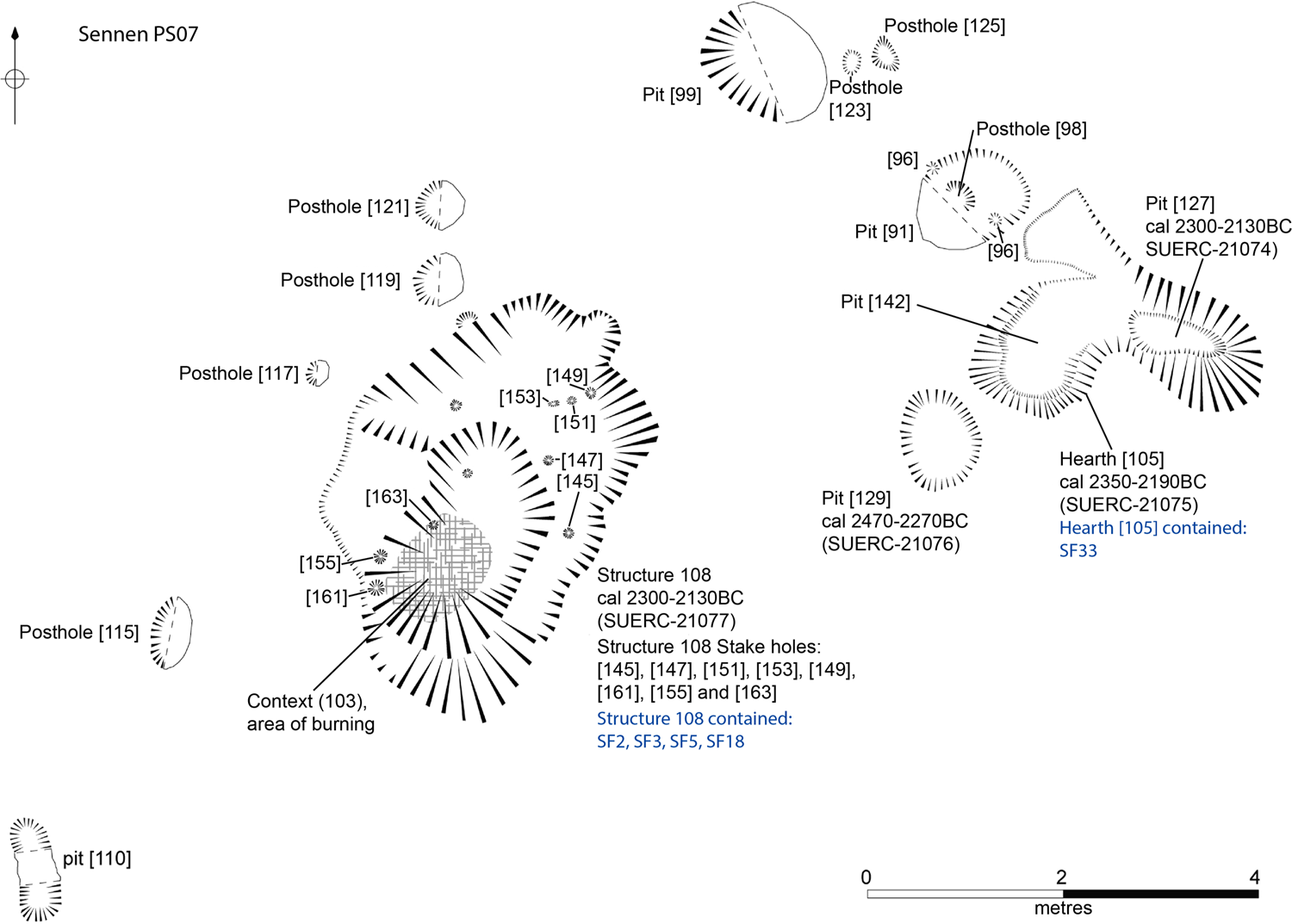
Figure 2. Sennen PS07: plan of Structure 108, with location of key artefacts from this analysis highlighted.
A row of postholes was found outside the north-western side of Structure 108, on the same north-east/south-west alignment, and to the north-east and east of Structure 108 there was an alignment of pits and postholes set at right angles to the posthole row. Several pits were also identified, some of which contained flint, Beaker pottery, and worked stones. To the immediate east was a complex of features which included a hearth [105], the upper fill of which (128) contained a greenstone pestle or pounder (SF33); this fill yielded a date of 2451–2146 cal bc (SUERC-21075, 3825 ± 30 bp, 95%). Radiocarbon determinations from Structure 108 and the surrounding features demonstrate occupation between c. 2300 and 2100 cal bc, and the nature of the architecture indicates that the occupation was short-lived (see Jones et al., Reference Jones, Taylor and Sturgess2012 for a full description). The slight character of Structure 108 is consistent with other contemporary ‘buildings’ associated with Beaker material (Darvill, Reference Darvill, Darvill and Thomas1996; O'Brien, Reference O'Brien2004; Sharples Reference Sharples, Allen, Sharples and O'Connor2009; Gibson, Reference Gibson2019).
Lelant TR18: Pit [6]
Archaeological monitoring at Lelant on the western side of the Hayle Estuary revealed a single pit associated with Beaker pottery, worked stone, and four flint artefacts, which included an end scraper and multi-purpose knife (Jones & Lawson-Jones, Reference Jones and Lawson-Jonesforthcoming) (Figure 3a). Pit [6] had a diameter of 0.7 m, was 0.16 m deep, and was buried beneath aeolian (dune) sands. The pit contained a single deposit, with clustered sherds of Beaker pottery from a single vessel, which, given their poor condition, are likely to derive from a curated vessel. The flint and worked stone artefacts were arranged around the base of the pit. Three of the four flint tools were found together, and two are likely to have come from the same core. The worked stone assemblage comprised three items. The first is a flat beach cobble, which may have been selected for deposition, as distinctive-looking pebbles and stones are often found within ritualized contexts during the Bronze Age (Tilley, Reference Tilley2017). The third object, a greenstone grinder or pounder (S1), is also highly distinctive.
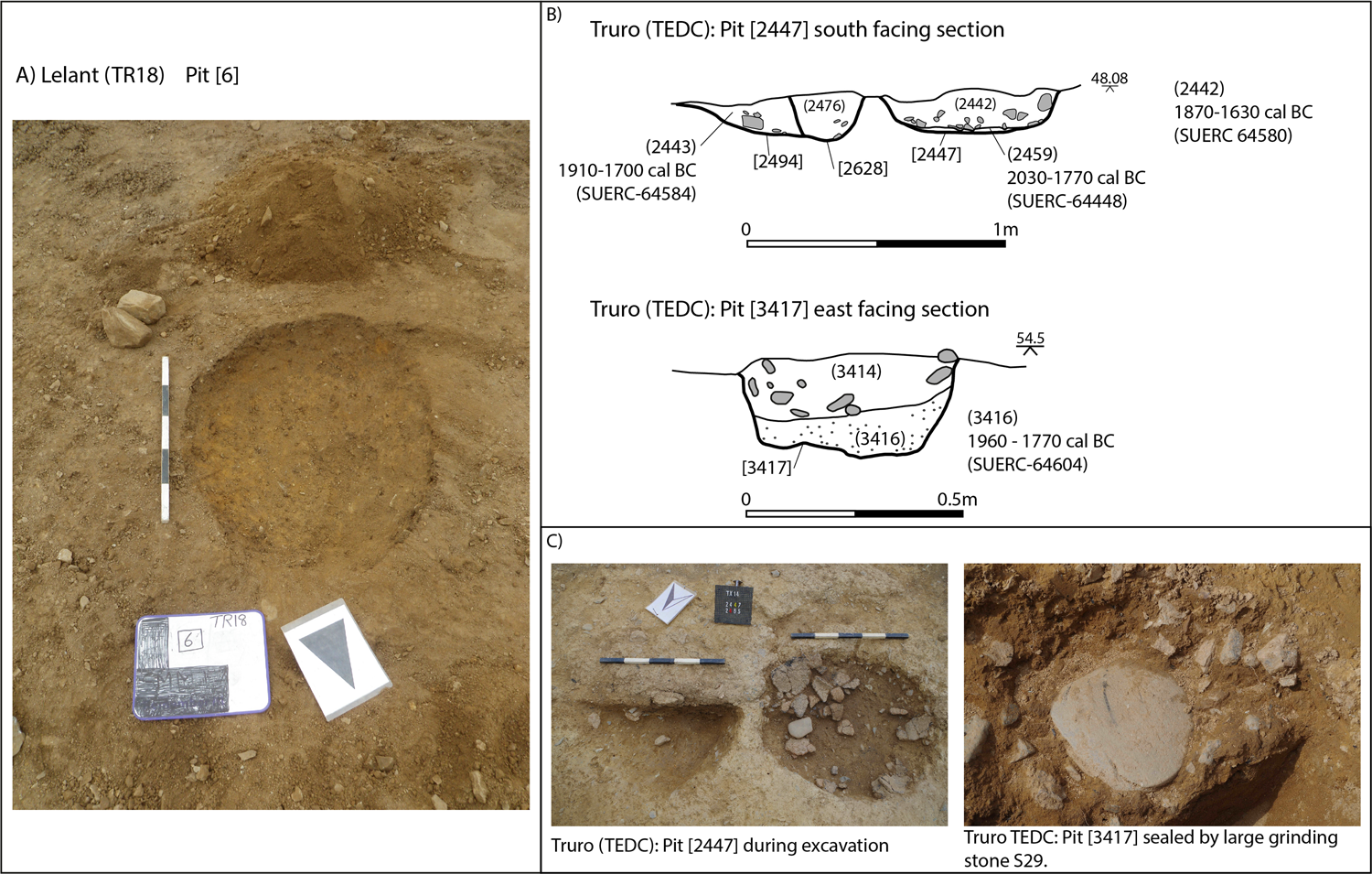
Figure 3. A) Lelant (TR18) pit [6] post excavation working shot; B) Truro: simplified sections of pits [2447] and [3417]; C) pit [2447] during excavation (left) and pit [3417] covered by artefact S29 (right).
The arrangement of the artefacts in pit [6] indicates more than the routine discard of occupation material. The assemblage represents both a deliberate clustering and a separation of different types of artefacts into distinct groups. There was deliberation in the choice of stone-processing tools which were selected and sourced from different geologies and used for separate tasks. The deposit thus contains a set of visually distinctive tools, which are likely to have been associated with particular people, activities, and places. The pit's location close to the Hayle Estuary may be significant as this was an important waterway linking people and places in the Bronze Age (Brück, Reference Brück2019: 220–23; Johnston, Reference Johnston2020: 140–45).
Truro TEDC: Pit [3417]
The Truro Eastern District Centre (TEDC) site on the eastern side of Truro comprised numerous pit groups spanning the Neolithic and EBA, many of which had been deliberately infilled (Taylor, Reference Taylor2022). Two of these pits, securely dated to the EBA, contained cassiterite (Figure 3b and 3c). The bowl-shaped pit [3417], 0.5 m in diameter and 0.24 m deep, was found in isolation and contained two fills. The lower, dated to 2010–1964 cal bc (SUERC-64604, 3543 ± 30 bp, 95%), contained cassiterite-rich cobbles weighing c. 1.25 kg and the environmental sampling residue contained a further 9.1 kg of crushed cassiterite. The upper fill contained 2.6 kg of cassiterite ore and pebbles, and the top of the pit was sealed by a large grinding tool (S29). The deposition of a significant quantity of crushed cassiterite, which was a valued material, coupled with the placing of the grinding tool across the top of the pit strongly suggests that it was a structured deposit. A second pit [2447] was also associated with cassiterite and abraded Collared Urn sherds; five waste flints and small rounded cassiterite granules were found at its base. The upper fill contained a large quantity of Trevisker pottery (a style particular to EBA south-western Britain), flints, and nearly 0.6 kg of cassiterite pebbles. Two radiocarbon determinations were obtained from the lower and upper fills, respectively: 2027–1774 cal bc (SUERC-64448, 3567 ± 7 bp, 95%) and 1870–1622 cal bc (SUERC-64580-3414 ± 28 bp, 95%). The dates for [2447] straddle a long timeframe, allowing for the possibility that infilling was slow, although the pit was shallow and there was no sign of silting between the layers. The abraded Collared Urn sherds in the primary fill also contrast with the Trevisker sherds in the upper fill. This suggests that the pit received two distinct artefact-rich caches in succession.
Materials and Methods
Sequence of analysis
Initially, the stone tool assemblages from Sennen (PS07, fifteen artefacts), plus selected artefacts from Lelant (TR18, one artefact), and Truro (TEDC, one artefact) were analysed by portable X-Ray Fluorescence (pXRF hereafter) scanning to detect elements associated with metalliferous ores. When elements such as tin (Sn) were detected, the stone tools were subjected to a more detailed pXRF analysis. This used an Innov-X Alpha Series instrument, using a five-minute count time per reading, obtaining indicative readings for Al, Si, P, K, Ti, V, Cr, Mn, Fe, Ni Cu, Zn, As, Rb, Sr, Sn, W, Zr, and Pb. Given the calibration limitations of the pXRF technique, a bespoke set of Sn calibration standards were used to quantify the Sn data values. Principal Component Analysis was used to define geochemical signatures within the data. A selection of artefacts were subsequently subjected to microwear analysis, including those that displayed elevated levels of Sn, as well as some artefacts chosen for their morphological and technological features (e.g. SF2).
Microwear analysis is a technique that identifies microscopic wear traces that develop on the surface of objects during manufacture, use, handling, but also post-depositionally (van Gijn, Reference van Gijn2010, Reference van Gijn2014). Microscopic observations were conducted at low (<100×) and high magnifications (100× and 200×) utilizing a stereomicroscope (Leica M80) with an external, oblique light source and a coaxial illumination unit (Leica M80 LED5000 CXI; magnifications up to 230×), and an incident light (metallographic) microscope (Leica DM1750M) with a Leica MC120 HD digital camera. Previous functional studies of stone tools used in mining activities in Britain have focused on macroscopic inspection (e.g. Gale, Reference Gale1995; Jenkins Reference Jenkins, Timberlake, Davidson, Mal, Marshall and Mighall2021), and other examinations of metalworking tools have used somewhat different techniques (e.g. Cowell & Middleton, Reference Cowell, Middleton and Fitzpatrick2011). This article presents the first application of an integrated approach that combines high-resolution microwear and pXRF analyses of stone metalworking assemblages in Britain and Ireland. For further details of the methods used and the artefacts analysed, see the online Supplementary Material.
Results
Microwear traces and tin concentrations
To establish the use of the selected tools, we combine the results from the two techniques in a cautious approach that looks for agreement between competing lines of evidence. Table 1 summarizes the tools that show evidence of use against a hard mineral material (see also Figure 4). Table 2 combines evidence from the pXRF and microwear analyses to interpret tool use and its relationship with metal production.
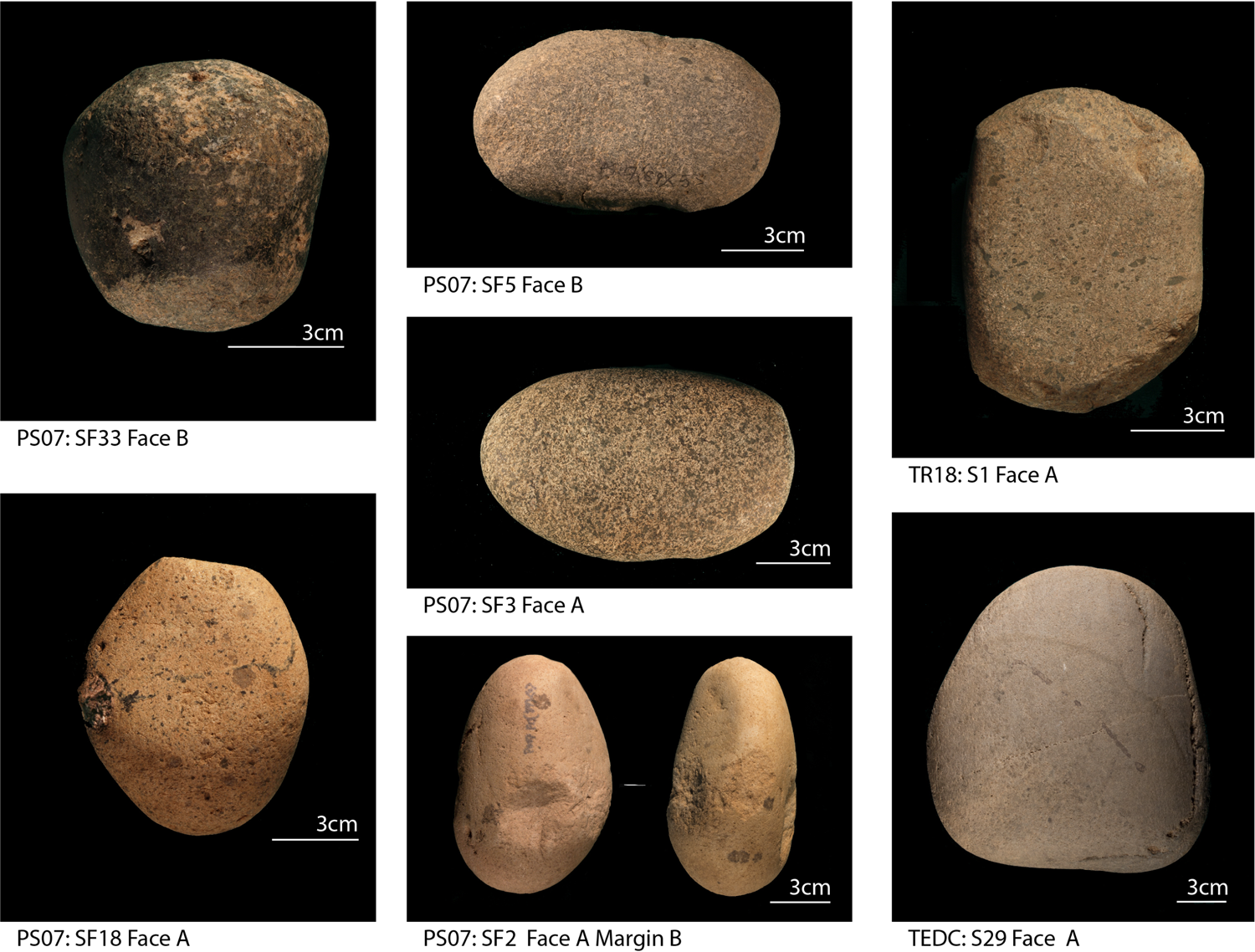
Figure 4. Stone tools presented in this analysis (shown at different scales, all scale bars 3 cm).
Table 1. The tools analysed: lithology, size, and associated pottery (see also Figure 4).
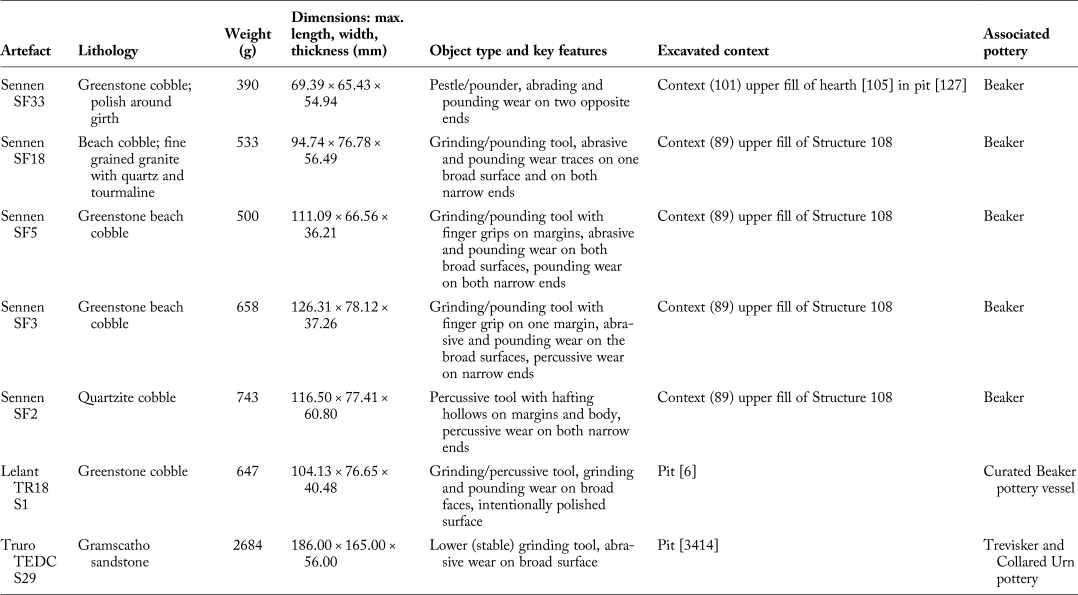
Table 2. Analysed stone tools: key evidence and confidence of the interpretation (see also highlights on Figure 5 and online Supplementary Material).
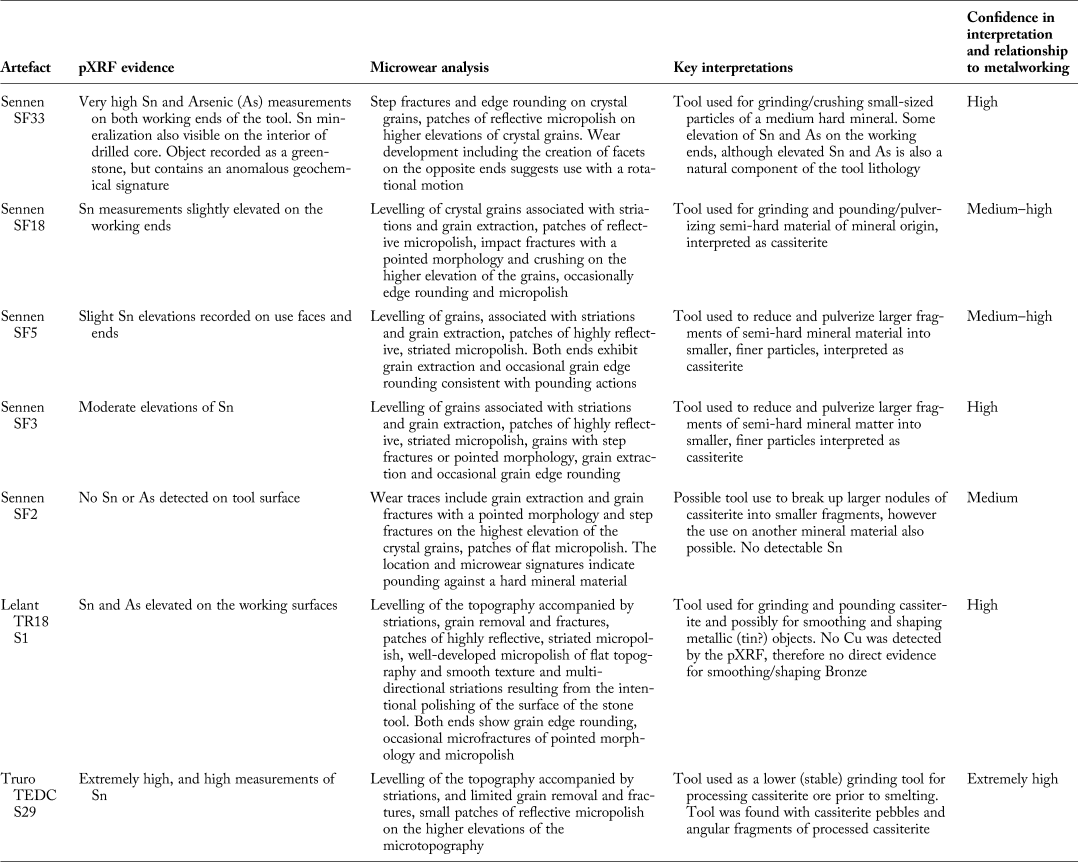
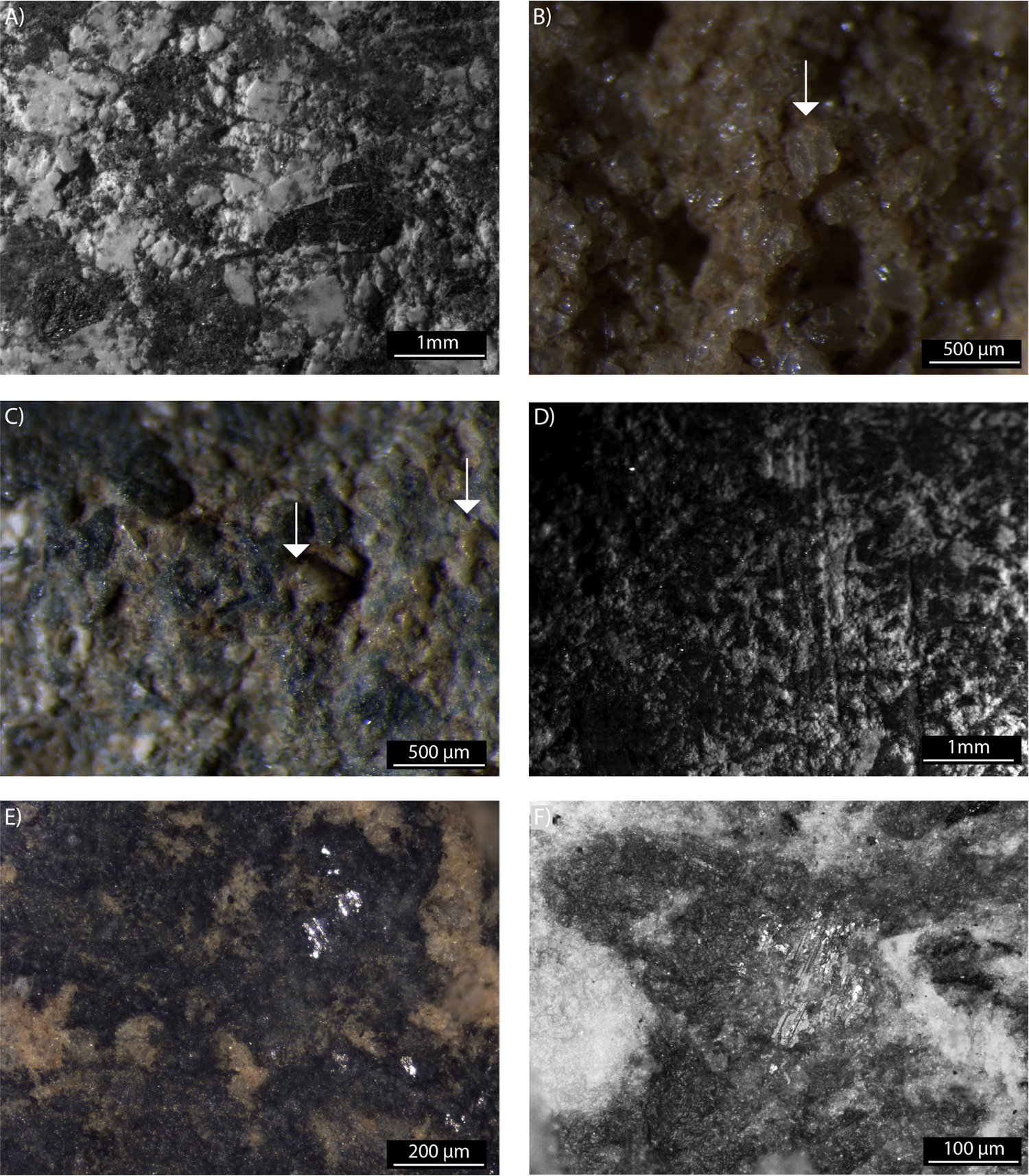
Figure 5. Key examples of macro- and microwear traces observed on the analysed tools: A) levelling of the surface topography (PS07-SF3); B) microfractures of grain crystals (indicated by arrow) (PS07-SF2); C) grain edge rounding (indicated by arrow) (TS18-S1); D) linear traces in the form of parallel, closely distributed striations (PS07-SF5); E) patches of highly reflective micropolish (taken at × 100) (PS07-SF5); F) patch of flat, reflective, striated micropolish (taken at × 200) (TS18-S1) (see Supplementary Material for full analyses).
The analysis of the complete pXRF dataset revealed a clear geochemical signature related to cassiterite tin ore (Sn, Pb, As, and W) (Wang et al., Reference Wang, Strekopytov, Roberts and Wilkin2016), defined by Principal Components 4 and 5. The pXRF data detected the presence of some high, but also variable, tin levels on the artefact surfaces, which are interpreted as partly derived from surface residues (based on higher levels on working surfaces) and partly from the artefacts’ natural lithologies. The microwear analysis defined a restricted range of actions, namely crushing and grinding, traces of which were found occasionally on the same surfaces. The microscopic wear traces suggest the processing of a semi-hard material of mineral origin; based on observed wear traces, including micropolish features, and on experimental data (e.g. Hayes et al., Reference Hayes, Pardoe and Fullagar2018), we can exclude the possibility that these traces derived from the processing of materials such as plants or grains, bone, wood, or clay. In places the observed wear traces include a micropolish of highly reflective appearance and in this case mineral micro-residues were incorporated within the micropolish during its formation (Figure 5). This agrees with previous findings that proposed that the presence of copper micro-residues within micropolishes on stone tools was due to metallurgical activities (Hamon et al., Reference Hamon, Réguer, Bellot-Gurlet, Pailler and Brisotto2020: 12–13).
Given that neither technique provides data that unequivocally defines tin ore processing, several lines of reasoning can be used to support our interpretation. The Cornish Gramscatho sandstone of the large grinding tool (TEDC S29) found with the cassiterite ore acts as a comparator for the other stone tools; it has some very high tin levels recorded, but it also has some tin elevations that are directly comparable to some readings on the other tools. Likewise, microwear on TEDC S29 includes traces (e.g. reflective micropolish, grain removal) that are consistently observed on the other stone tools. Moreover, the micropolish and other wear patterns identifiable on the stone tools are consistent with mineral processing. There is a close association between higher tin elevations and tool surfaces with developed micropolishes; this strongly suggests that some of the Sn readings relate to the function of the tools. Finally, it is possible that the tools were used on another hard mineral material that was not cassiterite, e.g. haematite (for use as a dye) or chalcopyrite (copper ore); there are, however, no distinctive staining or pigment particles on the artefacts (see Hayes et al., Reference Hayes, Pardoe and Fullagar2018) to support this, or any corroborating geochemical evidence. For each stone tool, we therefore indicate how confident we are that the stone tools were used within metalworking (Table 2).
Discussion
The stone tools
The microwear patterns and residues observed indicate that the tools were used to process a semi-hard mineral material. The pXRF analysis detected the presence of tin, associated with lower values of arsenic and tungsten, on six implements. Both arsenic and tungsten have been recorded as trace impurities within Cornish cassiterite ore and tailings (Yim, Reference Yim1981; Camm et al., Reference Camm, Glass, Bryce and Butcher2004), in prehistoric and historic Cornish tin slags (Tylecote et al,. Reference Tylecote, Photos and Early2010), and within the probable Bronze Age tin ingots from the Salcombe shipwreck in Devon (Wang et al., Reference Wang, Strekopytov, Roberts and Wilkin2016). The presence of this elemental grouping indicates that cassiterite ore is the signature's source. The stone tools analysed are made from granite, greenstone, quartzite, or sandstone and define a sub-group of artefacts that are geochemically different (due to elevated Sn levels) from the other predominantly local beach cobbles (although TEDC S29 is a sandstone cobble probably collected from a river bed) characterizing the sites’ wider stone assemblages. Significant variations in the relative tin concentrations between artefacts and across the surfaces of individual tools are interpreted in terms of tool function, lithology (especially for the granitic stone tools), and post-recovery artefact cleaning procedures with water, which is likely to have removed or reduced some tools’ residues. Our interpretation has prioritized the information gained from microwear analysis where the pXRF data are weaker. This is because we have a better understanding of the effects of post-depositional processes on microwear traces (see Marreiros et al., Reference Marreiros, Mazzucco, Gibaja, Bicho, Marreiros, Gibaja Bao and Bicho2015; Hayes et al., Reference Hayes, Pardoe and Fullagar2018) than we have on the presence of residues detectable through pXRF analysis.
All tools survive complete, and they retain the cobbles’ original morphology. The tools show consistency in raw material use, with an emphasis on cohesive and dense materials that could effectively withstand impact. This is also suggested by the consistency in the percussive tools’ size and weight (see Table 1). The microwear analysis indicates that they were used for crushing, grinding, and pounding. The cobbles were modified, mainly through use, and they exhibit moderate degrees of wear (see Adams, Reference Adams2014). The creation of hafting hollows or finger grips (‘comfort features’: Adams, Reference Adams2014: 103) on SF2, SF3, and SF5 allowed for easier handling, suggesting that intensive use was intended. This is supported by the employment of multiple surfaces on each tool, possibly indicating an attempt to maximize the potential of each implement. However, with the exception of SF33, none of the recorded implements were used for an extensive period.
The weight of the five Beaker tools (SF2, SF3, SF5, SF18, and TR18 S1) ranges from 500 g to 743 g. Percussive traces on SF2, the heaviest implement (743 g), are consistent with its use for breaking up larger nodules. This tool could be associated with an early phase of a multi-stage ore processing sequence (see O'Brien, Reference O'Brien2015: 224). In this case, the addition of a haft would have increased the tool's downward impact force. Following this stage, grinding or pounding tools weighing less than 700 g (e.g. SF3, SF5, and SF18) were used for crushing and pulverizing the larger fragments into finer particles to produce a fine concentrate for smelting (O'Brien, Reference O'Brien2015: 225). Likewise, Lelant S1 was used for crushing and fine grinding of mineral material, and its intentionally polished surface may have also been used for the smoothing and shaping of metal, thereby providing associations with the final stages of metal production. Tools weighing between 100 g and 500 g (SF33 and SF5) were also potentially used for crushing and pulverizing; in the case of SF33 this may have involved a rotational motion executed in a basin or hollowed surface. Based on their weight and the macro- and microwear traces, the Cornish tools are consistent with processing smaller ore fragments rather than direct mining. Cassiterite pebbles eroded out of tin lodes would have been easily visible and collectable from the gravel bedload in Cornish streams and rivers. The exception is S29 from TEDC18. This tool's lithology differs from that of the other tools and it was used differently as a stable lower grinding surface. At Copa Hill, one larger anvil stone was located amongst generally smaller hammerstones (Timberlake & Craddock, Reference Timberlake and Craddock2013) and thus the size of S29 might relate to its function in processing alluvial tin. However, EBA stone assemblages, as opposed to those associated with Beaker material, tend to be more diverse (Watts, Reference Watts2014: 98-101). The larger size of S29 could thus be related to functional aspects of tool use (as a lower grinding tool), reflect changing styles of artefacts morphologies and/or structured deposition in the EBA.
The tools in their wider context
An important aspect of the stone tool assemblages from Sennen and Lelant are their early date. Needham et al. (Reference Needham, Kenny, Cole, Montgomery, Jay and Davis2017) estimate the earliest tin-bronzes to be in circulation within Britain around 2200–2100 cal bc. Given the relatively quick adoption of bronze at this time (in comparison to continental Europe where copper has a more extensive history), the evidence of processing cassiterite ore in Cornwall between 2300 and 2100 cal bc is highly significant.
Research on copper sources, both in terms of their excavation (e.g. Dutton & Fasham, Reference Dutton and Fasham1994; O'Brien, Reference O'Brien1994, Reference O'Brien2004, Reference O'Brien, Fokkens and Harding2013, Reference O'Brien2015; Timberlake, Reference Timberlake2003, Reference Timberlake2014, Reference Timberlake2017; Timberlake et al., Reference Timberlake, Gwilt and Davis2004; Timberlake & Pragg, Reference Timberlake and Pragg2005) and the understanding of their chemical signatures (Ixer & Budd, Reference Ixer and Budd1998; Rohl & Needham, Reference Rohl and Needham1998; Bray & Pollard, Reference Bray and Pollard2012), has been very successful over the last three decades. Yet the discovery of tin mining and processing remains elusive. Copper-containing deposits, such as malachite, azurite, fahlore, bornite, and chalcopyrite, were exploited in Bronze Age Europe generally by open-cast techniques alongside surface pits, surficial deposit exploitation, and underground workings (O'Brien, Reference O'Brien, Fokkens and Harding2013: 447; Reference O'Brien2015). These sources were mined with relatively simple lithic technologies, often in combination with wooden and bone tools (though at Great Orme, between 1600 and 1400 cal bc, bronze tools were potentially used in the mining process: Williams & Le Carlier de Veslud, Reference Williams and Le Carlier de Veslud2019: 1181). Firesetting was commonly used to break up rock surfaces, allowing lithic tools, likely hafted, to be swung underarm to break up the surface and extract the ore (Timberlake & Craddock, Reference Timberlake and Craddock2013). These technologies and techniques reflect the nature of the copper deposits exploited at this time in Europe, where solid mineral deposits were being extracted from bedrock lithologies. Tin (and gold) are quite different, in that they often occur as eroded fragments in secondary deposits, such as alluvial gravels and other surficial deposits; therefore, they do not require the same extractive techniques. Timberlake (Reference Timberlake2017: 719, 722) has argued that prospection for early gold from alluvial sources may have been linked to the discovery of tin in those same environments, thus strengthening our perception of the connection between Ireland and south-western England and suggesting a movement back and forth of gold, tin, and copper. He also noted the lack of evidence for the exploitation of the rich copper deposits of south-western England, suggesting that the exploitation of the two metals may have been mutually exclusive (Timberlake, Reference Timberlake2017: 723), at least during the EBA (2450-1600 cal bc).
Many mining sites have been identified as belonging to the Bronze Age on the basis of the hammerstones recovered there. As Timberlake and Craddock (Reference Timberlake and Craddock2013: 39) discuss, a wide range of terms are employed for these tools, including stone mining mauls, stone hammers, hammerstones, pounders, crushers, stone picks, and stone anvils; they prefer to use the term ‘cobble stone mining tool’ because this captures their variability and interchangeable uses. The Ross Island lithic assemblage (containing over 7000 hammerstones) includes percussive tools, derived from well-rounded alluvial waterworn cobbles selected for their rounded shape (often elliptical, oval, or pear shaped) and lithology. The weight of the percussive tools used for processing ore was a significant variable, with a preference for cobbles weighing between 500 g and 1500 g for hammerstones, and lighter cobbles (100–500 g) used as specialized pecking tools; these cobbles were brought to Ross Island specifically for mining and processing ores and 86 per cent have some kind of modification to allow hafting (O'Brien, Reference O'Brien2004: 341–47). The assemblage is comparable to the slightly later material from Mount Gabriel (O'Brien, Reference O'Brien2004: 339), though only 47 per cent of the Mount Gabriel hammerstones show signs of hafting (O'Brien, Reference O'Brien2004: 347); they are thought to have had a shorter use life.
The analysis of the cobble stone mining tools from Copa Hill revealed that at least 79 per cent were smoothed and rounded, suggesting they came from beaches or rivers; the majority weighed around 2–2.25 kg and were around 15–25 cm long and 8–13 cm wide (Timberlake & Craddock, Reference Timberlake and Craddock2013: 42). Timberlake and Craddock (Reference Timberlake and Craddock2013: 42) argue that the cobbles were selected not only for their texture and size but also for their shape, with a clear preference for cylindrical or flat-sided exemplars. They note that there is a slight preference for finer-grained and harder rocks, which may indicate that form and size were generally more important than geology. The probable source of the cobbles used at Copa Hill was at the storm beach shingle bars near the mouth of the river Ystwyth some 25 km away (Timberlake & Craddock, Reference Timberlake and Craddock2013: 42).
There are some key overlaps and differences between the stone tools from these mining sites and the stone tools associated with tin processing presented here. The stone tools we analysed are well-worn and rounded cobbles that appear to have been selected for their shape and their cohesive dense lithology suitable for heavy work. The assemblage discussed here is lighter in weight and smaller in size than the tools from Ross Island, Mount Gabriel, and Copa Hill but this is not unexpected; the latter were used to extract mineral ore associated with solid bedrock, whilst the tools from our sites were primarily used at a later stage in the chaîne opératoire, processing alluvial cassiterite that was already broken down into smaller particles through natural fluvial weathering. There is also an overlap with some of the grinding slabs and anvil stones identified at the settlement site associated with Ross Island (O'Brien, Reference O'Brien2004: 356–59) and S29 from TEDC, which was also used as a grinding slab. These Ross Island tools are associated with the processing (rather than mining) of copper ore, being used as a surface for grinding and crushing, and the same processes are suggested for S29, albeit for alluvial tin (Figure 6).
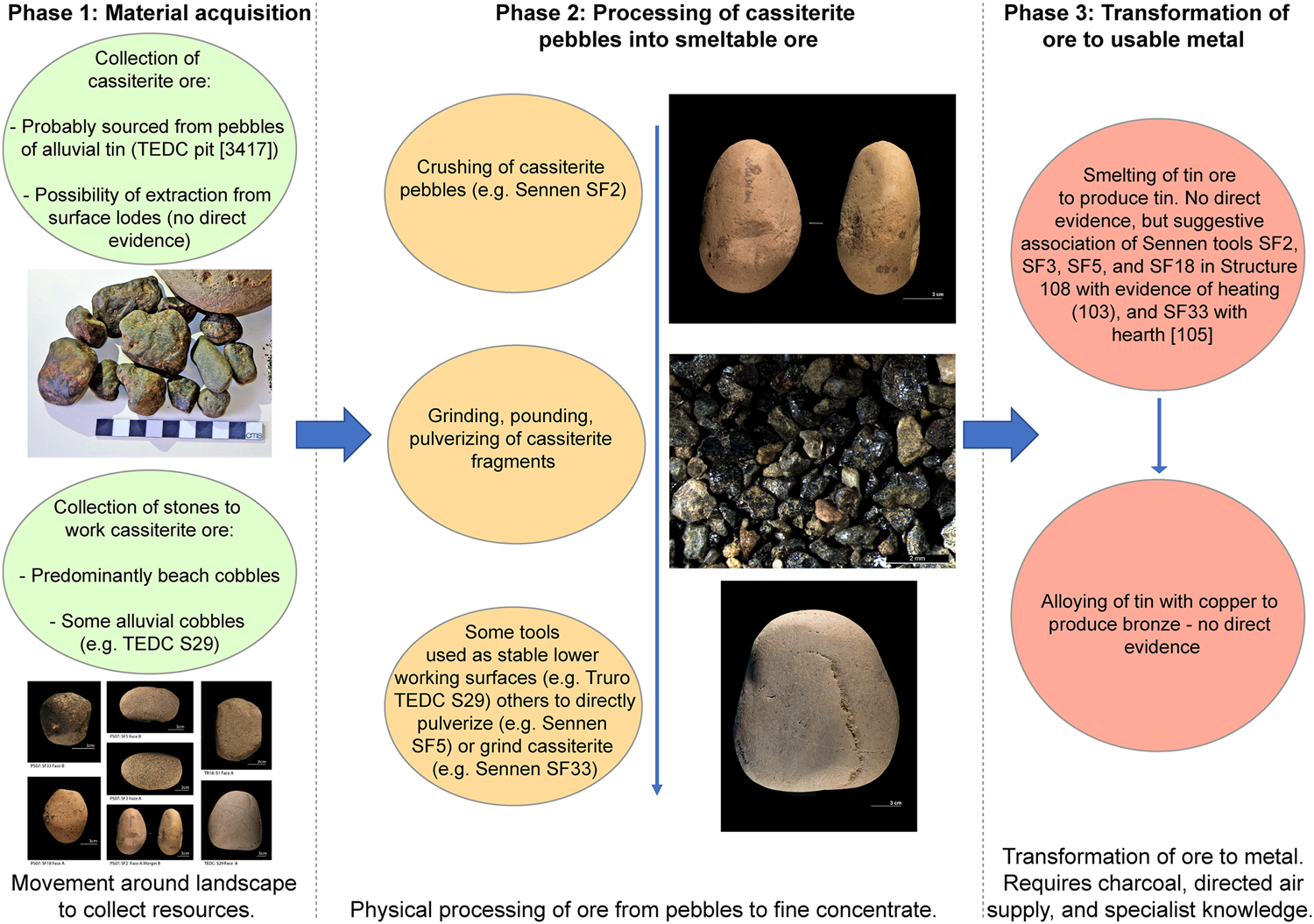
Figure 6: Simplified chaine opératoire of tin processing. The evidence from our work pertains to Phases 1 and 2. Phase 3 remains necessarily speculative given the evidence presented in this paper, although the association of tools with evidence of heating at Sennen (PS07) is suggestive.
The tools analysed here are not those used for extraction and mining but are those used in processing. In terms of the structures they are associated with, there are also some similarities and differences between Ross Island (particularly Structure C) and Sennen's Structure 108. They are of similar size and shape, although the Ross Island structures were defined by gullies and several of the structures are much larger, whilst Sennen Structure 108 was sunken. There is also more evidence for intensive activity, centred around a mine, on the Ross Island site and its chronological span is longer (c. 500 years, but see Carlin, Reference Carlin2018 who questions their association with the site's active period of mining). The site at Sennen is interpreted as shorter lived and more likely to have been associated with the collection of readily available cassiterite from streams, which may have contributed to its temporary nature.
Finding Bronze Age tin
The absence of evidence for tin mining and processing has long been a puzzle in Bronze Age Britain and Europe. At Sennen, the stone tools interpreted as having been associated with cassiterite processing were not randomly scattered across the site; all were found close to areas of burning or in features with other burnt material. Four tools (SF2, SF3, SF5, and SF18) were found above the burnt area within the infill of Structure 108, whilst SF33 was found in the hearth complex outside the structure. The association with fire is suggestive and it is possible that these hearths were used to smelt tin. Such smelting would not require a specialized furnace lining; tin can be smelted in a small ceramic vessel in a hearth with a directed air supply (Ottaway & Roberts, Reference Ottaway, Roberts and Jones2009). The excavation at Sennen yielded no moulds or crucibles, which is in line with much of the evidence for metalworking in Britain and Ireland in this period (see Webley et al., Reference Webley, Adams and Brück2020). Instead we have an assemblage of stone artefacts more closely associated with processing, involving the primary pulverizing and crushing of cassiterite pebbles and subsequent grinding to produce a fine concentrate for smelting. Structure C at Sennen, whilst it has a definable relationship to cassiterite processing, is not definably a metal workshop; rather it is a slight, seasonal or temporary structure associated with a range of activities (e.g. SF14 was associated with plant processing identified from microwear analysis), similar to the situation described by Hamon et al. (Reference Hamon, Réguer, Bellot-Gurlet, Pailler and Brisotto2020) in Brittany. All three of our sites had evidence of the deliberate deposition of materials and objects. In certain respects, this is comparable with practices of the later Bronze Age (Webley et al., Reference Webley, Adams and Brück2020: 184–86), when moulds and crucibles, as well as objects, were increasingly deposited in formal deposits.
Our analysis suggests that the search for evidence for tin exploitation is unlikely to be resolved by following traditional assumptions of what metalworking sites look like. We have, in effect, been looking for the wrong things. Instead, the primary evidence may lie in the close examination, through geochemical but especially microwear analysis, of stone tools. Stone tools in the Bronze Age are often paid less attention than lithics from earlier periods. Our evidence for the extraction of tin differs from that for the extraction of copper. In the case of copper exploitation, which targeted bedrock concentrations, it has long been stone tools that have led us to mining sites. In the case of tin, alluvial and surficial deposits of cassiterite do not require mining, but they do require similar processing, potentially undertaken with a similar toolkit of multifunctional cobbles. It is in these objects, often only briefly summarized or ignored, that the detailed evidence of how early metals were worked may lie. In the case of the tools analysed here, their deposition suggests their critical importance for people at the time, but it is in attending to the microhistories of their wear and the tiny traces lodged in their chemical composition that their role in shaping past histories can more clearly emerge.
Conclusion
The small-scale collecting and smelting of tin ores, primarily from cassiterite pebbles, is unlikely to have left direct traces in the archaeological record, and hence we must apply other suites of analyses to detect such activity. The study of artefacts, using geochemistry alongside microwear analysis, can substantively contribute to understanding the processing of metal ores and the finishing of metal artefacts (see Hamon et al., Reference Hamon, Réguer, Bellot-Gurlet, Pailler and Brisotto2020). Our study highlights the value of applying combined microwear and pXRF analyses to stone tools from EBA sites.
In an article summarizing the state of research concerning Bronze Age mining and metal production, Simon Timberlake (Reference Timberlake2017: 716) wrote: ‘There is good circumstantial evidence and a strong narrative tradition which asserts Cornwall (and Devon) to be the European home(s) of prehistoric tin.’ Our results strongly suggest that Cornish tin sources were being processed from as early as c. 2300–2200 cal bc, and that ores from these sources were integrated into the circulation of metals, first across Britain and Ireland and subsequently in the wider Atlantic region and beyond (Berger et al., Reference Berger, Wang, Brügmann, Lockhoff, Roberts and Pernicka2022). This ties in with the goldwork evidence, in terms of the Atlantic distribution of the broadly contemporary lunulae found in Ireland, Cornwall, and Brittany (Taylor, Reference Taylor1980; Needham, Reference Needham2000), and with respect to geochemical analyses which have identified Cornish gold in other artefact types (Esher et al., Reference Esher, Borg and Pernicka2011; Standish et al., Reference Standish, Dhuime, Hawkesworth and Pike2015; Krause et al., Reference Krause, Breuer, Korolnik, Pernicka, Schorer and Spatzier2021). We can therefore potentially see tin and gold from Cornwall, and copper from Ireland and Wales, as forming part of a wider Atlantic exchange network. The discoveries presented here constitute not only the earliest secure evidence for tin exploitation in Britain, but also they showcase a new methodological approach for identifying tin processing, opening up a critical new perspective on the emergence of bronze.
Supplementary Material
To view supplementary material for this article, please visit https://doi.org/10.1017/eaa.2022.30.
Acknowledgements
We would like to thank Henrietta Quinnell and Simon Timberlake for reading this article and Sean Taylor for information on the Truro TEDC site. The microwear analysis on the stone tools discussed here was carried out as part of the Leverhulme-funded ‘Beyond the Three Age System’ project (RPG-2017-375). We would also like to thank David Dawson for introducing the research teams.