1. Introduction
The southern African Pliensbachian–Toarcian record (Fig. 1) is particularly important in the light of recent advances in the understanding of the global biogeochemical perturbations, especially climate changes, associated with the extensive igneous outgassing in the Karoo–Ferrar Large Igneous Province (LIP; e.g. Jenkyns, Reference Jenkyns1988; Pálfy & Smith, Reference Pálfy and Smith2000; Gröcke et al. Reference Gröcke, Rimmer, Yoksoulian, Cairncross, Tsikos and van Hunen2009; Suan et al. Reference Suan, Mattioli, Pittet, Lécuyer, Suchéras-Marx, Duarte, Philippe, Reggiani and Martineau2010, Reference Suan, Nikitenko, Rogov, Baudin, Spangenberg, Knyazev, Glinskikh, Goryacheva, Adatte, Riding and Föllmi2011; Dera et al. Reference Dera, Brigaud, Monna, Laffont, Pucéat, Deconinck, Pellenard, Joachimski and Durlet2011; Self et al. Reference Self, Schmidt and Mather2014; Guex et al. Reference Guex, Pilet, Müntener, Bartolini, Spangenberg, Schoene, Sell and Schaltegger2016; De Lena et al. Reference De Lena, Taylor, Guex, Bartolini, Adatte, van Acken, Spangenberg, Samankassou, Vennemann and Schaltegger2019; Storm et al. Reference Storm, Hesselbo, Jenkyns, Ruhl, Ullmann, Xu, Leng, Riding and Gorbanenko2020). More specifically, the interbasalt sedimentary rocks in the Pliensbachian–Toarcian Drakensberg Group serve as archives not only for how life was smothered locally by smouldering lava flows and explosive magma–sediment interactions, but also on how LIP magmatism caused a global environmental change in the Early Jurassic (e.g. Svensen et al. Reference Svensen, Jamtveit, Planke and Chevallier2006, Reference Svensen, Torsvik, Callegaro, Augland, Heimdal, Jerram, Planke and Pereira2018; Holzförster Reference Holzförster2007; McClintock et al. Reference McClintock, Marsh and White2008 a, b; Bordy et al. Reference Bordy, Rampersadh, Abrahams, Lockley and Head2020 b, Reference Bordy, Haupt and Head2021). While quantifying the causes of mass extinctions is fundamental for mapping the evolution of life on Earth, it is important to remain cognizant of the value of well-dated baseline studies on the palaeoenvironments, palaeogeography and palaeoecology prior to, during and after these multiphase events (Gröcke et al. Reference Gröcke, Rimmer, Yoksoulian, Cairncross, Tsikos and van Hunen2009; Caruthers et al. Reference Caruthers, Smith and Gröcke2013; Luttinen et al. Reference Luttinen, Kurhila, Puttonen, Whitehouse and Andersen2022).
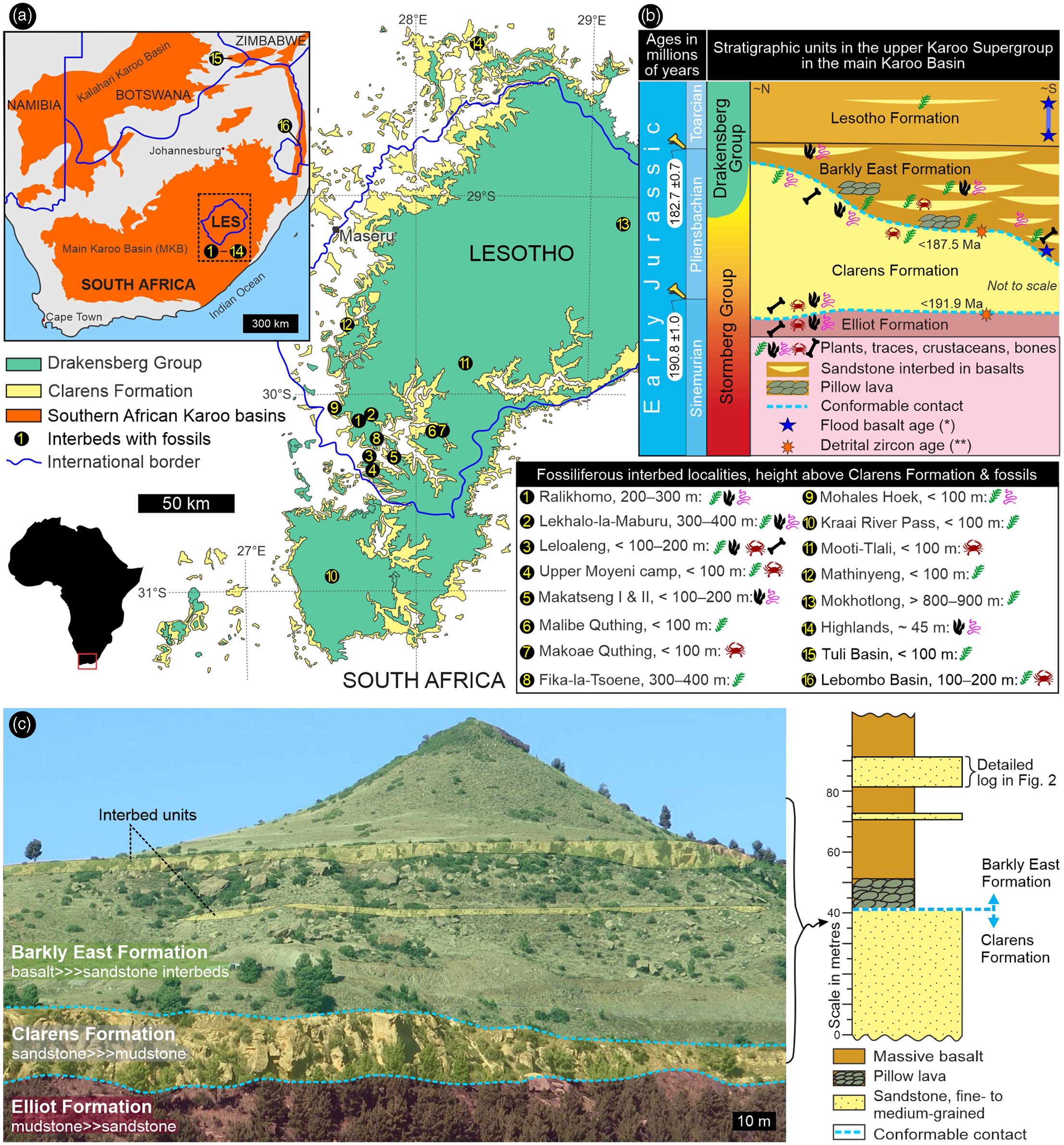
Fig. 1. Location and general stratigraphy of the study sites in southern Africa (Lesotho and South Africa). (a) Position of the fossiliferous interbed sites in the Lower Jurassic Drakensberg Group on a simplified regional geological map. (b) Stratigraphic context of the fossiliferous interbed sites within the upper Karoo Supergroup. (c) Representative outcrop view of two interbed units in the Barkly East Formation at Upper Moyeni (site #4) in Lesotho. Upper Karoo ages: * – Moulin et al. (Reference Moulin, Fluteau, Courtillot, Marsh, Delpech, Quidelleur and Gérard2017); ** – Bordy et al. (Reference Bordy, Abrahams, Sharman, Viglietti, Benson, McPhee, Barrett, Sciscio, Condon, Mundil and Rademan2020 a).
The Pliensbachian–Toarcian rock record in southern and eastern Gondwana encompasses the continental flood basalt outpourings associated with the magmatic events in the Karoo–Ferrar LIP (e.g. Marsh, Reference Marsh1984; Elliot et al. Reference Elliot, White and Fleming2021). Intercalated with the basaltic lava flows and often closely associated with pillow lavas, sedimentary interbeds comprise mostly quartz-rich sandstone with minor mudstone and limestone, and occur throughout the Karoo–Ferrar region from southern (e.g. South Africa, Lesotho, Botswana, Zimbabwe, Namibia) to eastern Gondwana (e.g. Antarctica, Tasmania – e.g. du Toit, Reference du Toit1927, Reference du Toit1929; Bond et al. Reference Bond, Wilson and Raath1970; Ellenberger, Reference Ellenberger and Haughton1970; Smith, Reference Smith1984; Stollhofen et al. Reference Stollhofen, Gerschütz, Stanistreet and Lorenz1998; Rawlings et al. Reference Rawlings, Watkeys and Sweeney1999; Calver, Reference Calver2009; Gierlowski-Kordesch et al. Reference Gierlowski-Kordesch, Weismiller, Stigall, Hembree, Larsen, Egenhoff and Fishman2015). These interbeds are also often fossiliferous, and can contain microbial mats, fragmentary remains of coniferous and herbaceous plant species (e.g. palynomorphs, fronds, tree stumps, ex situ wood), invertebrates (e.g. gastropods, bivalves, various arthropods including conchostracans, ostracods, notostracans, malacostracans, insects), vertebrates (e.g. fish) and trace fossils of both invertebrates and vertebrates (e.g. Rennie, Reference Rennie1937; Ellenberger et al. Reference Ellenberger, Ellenberger, Fabre, Ginsburg and Mendrez1964 a, b; Harrington, Reference Harrington1965; Aldiss et al. Reference Aldiss, Benson and Rundle1984; Bradshaw, Reference Bradshaw1987; Tasch, Reference Tasch1987; Elliot & Hammer, Reference Elliot, Hammer and Mitra1996; Babcock et al. Reference Babcock, Leslie, Elliot, Stigall, Ford and Briggs2006; Garland et al. Reference Garland, Bannister, Lee and White2007; Bomfleur et al. Reference Bomfleur, Schneider, Schöner, Viereck-Götte and Kerp2011, Reference Bomfleur, Schöner, Schneider, Viereck, Kerp and McKellar2014; Stigall et al. Reference Stigall, Hembree, Gierlowski-Kordesch and Weismiller2014; Elliot et al. Reference Elliot, White and Fleming2021). To date, tetrapods have been reported as ichnites and as very rare tiny bone fragments from the interbeds of Lesotho and South Africa (Ellenberger, Reference Ellenberger and Haughton1970; Bordy et al. Reference Bordy, Rampersadh, Abrahams, Lockley and Head2020b, – fig. 1).
In comparison to Karoo fossil groups from pre-Drakensberg successions, this final Karoo fossil assemblage from the Pliensbachian–Toarcian in the main Karoo Basin (Fig. 1) is rather unique. This is because the Drakensberg continental biota is contained in igneous rocks, and thus provides a geochronologically more constrained palaeo-biodiversity, palaeoclimatic and palaeoecological snapshots of life and land that coexisted in the fiery terminal Karoo time during the flood basalt volcanism in the late Early Jurassic.
In this review of the sedimentary processes and fossil biota from terminal Karoo times in southern Africa (Fig. 1), we present the palaeo-biodiversity of this massive volcanic landscape and the – albeit temporary – triumph of life in extraordinarily adverse environmental conditions associated with giant continental mafic magmatic events at a scale unknown in modern volcanic systems. We also demonstrate that southern Gondwana was not a barren volcanic desert at the onset of the voluminous Karoo continental flood basalts and associated igneous activities. Moreover, in a more regional context, this work also suggests a potential final migration route for the famous Karoo biota that has been evolving in the palaeontologically and geologically iconic main Karoo Basin for 110 million years from the late Palaeozoic to the early Middle Mesozoic (e.g. Rubidge et al. Reference Rubidge, Day, Barbolini, Hancox, Choiniere, Bamford, Viglietti, McPhee and Jirah2016).
2. Geological setting
The Pliensbachian–Toarcian Drakensberg Group forms part of the Karoo–Ferrar Large Igneous Province of southern Africa and Antarctica, one of the Earth’s largest continental flood basalt provinces (e.g. Duncan et al. Reference Duncan, Hooper, Rehacek, Marsh and Duncan1997; Marsh et al. Reference Marsh, Hooper, Rehacek, Duncan, Duncan, Mahoney and Coffin1997; Moulin et al. Reference Moulin, Fluteau, Courtillot, Marsh, Delpech, Quidelleur and Gérard2017; Svensen et al. Reference Svensen, Torsvik, Callegaro, Augland, Heimdal, Jerram, Planke and Pereira2018). In the main Karoo Basin (Fig. 1), it is the youngest stratigraphic unit of the Karoo Supergroup and comprises a ∼1400 m thick pile of basaltic and some andesitic lava flows with subordinate clastic interbeds (for recent summaries, see Bordy et al. Reference Bordy, Rampersadh, Abrahams, Lockley and Head2020 b, Reference Bordy, Haupt and Head2021). The contemporaneous intrusive rocks (i.e. a subvolcanic network of dolerite sills and dykes) are geochemically identical and likely fed the voluminous, fissure-like volcanic eruptions across southern Africa. The outpouring lava rapidly covered a vast tract of the southern Gondwanan landscape that now belongs to Zambia, Zimbabwe, Namibia, Botswana, Eswatini, Lesotho and South Africa (Fig. 1a – e.g. Moulin et al. Reference Moulin, Fluteau, Courtillot, Marsh, Delpech, Quidelleur and Gérard2017; Svensen et al. Reference Svensen, Torsvik, Callegaro, Augland, Heimdal, Jerram, Planke and Pereira2018). Although post-Karoo erosion intensely ravaged this once extensive volcanic landscape, the most intact erosional remnant persists as an impressive volume of igneous rocks that is superbly exposed in the highlands of Lesotho and the circum-Lesotho area of eastern South Africa (Fig. 1a).
Interlava sedimentary layers, too, occur throughout southern Africa and some are fossiliferous (Fig. 1a, b – e.g. Rennie, Reference Rennie1937; Aldiss et al. Reference Aldiss, Benson and Rundle1984; Smith, Reference Smith1984), although they are most common and most fossiliferous in the south, in the Barkly East Formation, the older of the two Drakensberg formations (Fig. 1 – e.g. du Toit, Reference du Toit1927; Stockley Reference Stockley1940; Ellenberger et al. Reference Ellenberger, Ellenberger, Fabre, Ginsburg and Mendrez1964 a, b). The clastic interbeds show petrographic characters similar to the conformably underlying quartz-sandstone-dominated Clarens Formation (Fig. 1b), which in its upper part has been described as a wet aeolian system (e.g. Beukes, Reference Beukes and Haughton1970; Bordy et al. Reference Bordy, Haupt and Head2021). The interbed thickness ranges from <1 m to a few metres; the lateral extent is seldom more than a few hundred metres. In the lower Barkly East Formation, the interbeds commonly comprise more than one layer, and locally these interbed units can be as much as 60 m in thickness (Beukes, Reference Beukes and Haughton1970).
To date, the freshwater and terrestrial flora and fauna of the Drakensberg interbeds (Supplementary Material Table 1) have been mentioned only briefly and without any subsequent taxonomic treatments (except for one ichnotaxon in Bordy et al. Reference Bordy, Rampersadh, Abrahams, Lockley and Head2020 b). The informal binominal nomenclature is retained for now, because it is beyond the scope of this contribution to give a revised taxonomic treatment of these Drakensberg fossils. In addition to vertebrate footprints, interbeds contain associated impressions of Early Jurassic raindrops and desiccation cracks (e.g. Ellenberger et al. Reference Ellenberger, Ellenberger, Fabre, Ginsburg and Mendrez1964 a, b; Ellenberger, Reference Ellenberger and Haughton1970, Reference Ellenberger1975), and these are unequivocal evidence for the continental origin of these rocks (see also Marsh, Reference Marsh2020). The regional distribution of the lower Drakensberg pillow lavas further demonstrates that the land surface, over large areas of southern Africa, was partially covered by running and standing water bodies (e.g. Bond et al. Reference Bond, Wilson and Raath1970; McCarthy, Reference McCarthy and Haughton1970; Lock et al. Reference Lock, Paverd and Broderick1974; Aldiss et al. Reference Aldiss, Benson and Rundle1984; Bordy et al. Reference Bordy, Haupt and Head2021).
All in all, geological and palaeontological evidence suggests that from the late Pliensbachian into the early Toarcian, the palaeoenvironment in southern Gondwana was dominated by the multiphase Karoo volcanic event and that clastic deposition occurred within a fluvio-lacustrine environment in a seasonally wet palaeoclimate (e.g. Beukes, Reference Beukes and Haughton1970; Bordy et al. Reference Bordy, Rampersadh, Abrahams, Lockley and Head2020 b, Reference Bordy, Haupt and Head2021). Currently, the link between the wet period in the terminal Karoo times (Bordy et al. Reference Bordy, Haupt and Head2021) and the global Pliensbachian–Toarcian atmospheric perturbations due to the gas emissions in the Karoo–Ferrar LIP (e.g. Moulin et al. Reference Moulin, Fluteau, Courtillot, Marsh, Delpech, Quidelleur and Gérard2017) remains unstudied. However, high-resolution chemostratigraphy of the Karoo basaltic lavas and clastic interbeds could further assess this link.
3. Material and methods
3.a. Brief history of the study materials
Paul Ellenberger pioneered early Mesozoic (Late Triassic and Early Jurassic) tetrapod ichnology in Lesotho in the 1950s through the 1970s, producing two monographs (Ellenberger Reference Ellenberger1972, Reference Ellenberger1974) and other papers (Ellenberger, Reference Ellenberger1955, Reference Ellenberger and Haughton1970, Reference Ellenberger1975; Ellenberger & Ellenberger, Reference Ellenberger and Ellenberger1956, Reference Ellenberger and Ellenberger1958, Reference Ellenberger and Ellenberger1959, Reference Ellenberger and Ellenberger1960; Ellenberger et al. Reference Ellenberger, Ellenberger, Fabre, Ginsburg and Mendrez1964 a, b, Reference Ellenberger, Ellenberger, Ginsburg and Amos1969, Reference Ellenberger, Ellenberger and Ginsburg1970) in which he proposed a large number of ichnogenera and ichnospecies for tracks made by both invertebrates and various tetrapods, and keyed them to his biostratigraphic zones (A/1 through C/7). Fossil vertebrate tracks had been known and illustrated by the indigenous peoples prior to the colonization of southern Africa (Ellenberger et al. Reference Ellenberger, Mossman, Mossman and Lockley2005). Although sporadic track documentation both by missionaries (e.g. the track-bearing slab at Morija by Dieterlen, Reference Dieterlen1885 and Christol, Reference Christol1897) and geologists (e.g. Dornan, Reference Dornan1908) did occur, Lesotho’s ichnological (and to a lesser degree palaeontological; see Knoll, Reference Knoll2005) heritage was largely underappreciated before Ellenberger’s interest in fossil footprints started in the 1950s.
Ellenberger worked at a time when travel in Lesotho was difficult, maps were in many cases rudimentary and GPS technology did not exist. He commonly named the fossiliferous sites after the nearest Basotho village or homestead. As part of a careful restudy of Ellenberger’s pioneer work, several of his study localities have been subjected to careful analysis to place them in stratigraphic and sedimentological context and evaluate the validity of the associated ichnotaxa and their regional and global significance. To this end, Ellenberger’s published reports and unpublished documents, notes, site and specimen photographs, specimen inventory with field localities and physical specimens in the Ellenberger Collection, both at the University of Montpellier (UoM) in France and Morija Museum and Archives (MM&A) in Lesotho, have been examined, allowing us to relocate some of his fossil localities for further study. Ellenberger’s figures are modified and republished under a CC BY licence, with permission from the Geological Society of South Africa and Palaeovertebrata.
Revising Ellenberger’s informal binomial nomenclature of the Drakensberg fossils (Supplementary Material Table 1) is beyond the scope of this current study; however, we recognize that some of the taxa, when originally named by Ellenberger, were presented without adequate taxonomic characters, and thus are considered nomina nuda. Some of the remarkably preserved invertebrate traces are part of an ongoing systematic ichnological study.
3.b. Fieldwork and photogrammetry
Because the location of Ellenberger’s highly remote fossiliferous interbeds was not pinpointed on any maps, several field excursions were undertaken by us to find these sites across Lesotho. Preparatory to the excursions, efforts were made, using Google Earth Pro, to identify sandstone interbeds in the Drakensberg Group. Because in most cases, no roads, only footpaths, exist in the Lesotho highlands (aka the ‘Kingdom in the Sky’ with its lowest point being 1400 m above sea level) within ∼ 10–12 km of the ichnosites, sometimes the excursions had to proceed on foot on bridle paths or across the rugged terrain. Unfortunately, in spite of our best and repeated efforts, some sites could not be located. Among others, we attribute the low relocation rate to the dynamics of Basotho villages and homesteads that get deserted or amalgamated with others under different names or turn into densely built, semi-urban areas. Moreover, several specimens mentioned in the UoM specimen inventory had been misplaced or lost, and some specimens in the MM&A collection are without locality labels. Nonetheless, we combined these materials for the sake of presenting a revised overview of the Drakensberg Group fossil biota that is as complete and comprehensive as possible (Supplementary Material Table 1; see supplementary material here: https://doi.org/10.6084/m9.figshare.19636593).
The material studied here consists of our own field discoveries and those from Ellenberger’s sites that we either relocated or assessed from specimens in the Ellenberger Collections at the UoM and MM&A (Supplementary Material Table 1). For example, during fieldwork at Ralikhomo, after locating a track-bearing surface in one of the sandstone interbeds, we recognized a clear trackway of seven consecutive, more or less complete manus–pes sets preserved as natural impressions (concave epireliefs) that has never been mentioned by Ellenberger. In addition to tracing this newly discovered trackway (with an orientation towards azimuth 350°) on transparent plastic, two moulds were made using modelling clay, and these were converted into ‘hard copy’ plaster replicas later the same day. These are preserved as specimens in the Ichnology Collection of the Evolutionary Studies Institute (ESI) at the University of Witwatersrand, South Africa (accession numbers: BP/6/747), with duplicate replicas (UCM 182.14 and 182.15) in the University of Colorado Museum of Natural History (UCM). The field tracing of the trackway is archived as an acetate film (T 1903) at UCM.
Using a Canon PowerShot EOS D1200 and Nikon D5100 digital cameras, both in the field and in the UoM and MM&A Ellenberger Collections, a series of photographs were taken of each specimen for photogrammetric analysis, applying the approach described by Mallison and Wings (Reference Mallison and Wings2014) and Falkingham et al. (Reference Falkingham, Bates, Avanzini, Bennett, Bordy, Breithaupt, Castanera, Citton, Díaz-Martínez, Farlow and Fiorillo2018). Using the Agisoft Metashape Professional (v.1.6.1) software, orthomosaics and photogrammetric 3D models were generated of the in situ and ex situ specimens. Depth-colour image analyses were produced in Cloud Compare (software v.2.6.1) and ParaView (v.5.5.1). All data, including photographs, models and data tables, are available from Figshare (https://doi.org/10.6084/m9.figshare.19636593). Standard morphometric parameters were measured both in situ in the field and ex situ from tracings and photogrammetric 3D models to determine pes and manus lengths (L) and widths (W), pes outward rotation, step, stride and pace angulation where applicable. The graphical illustration of the measured morphometric parameters is shown in Supplementary Material Figure A (https://doi.org/10.6084/m9.figshare.19636593).
4. Sedimentology of the interbeds
Isolated by basaltic lava flows, the sedimentary interbeds of the Barkly East Formation are tabular to lenticular units that are usually <10 m in thickness, and <400 m in length (Figs 1, 2). Prominent channel-shaped units have not been observed in any of the outcrops, and correlation of the interbed units is not possible even over short lateral distances. Comprising fine- to medium-grained, often silty sandstones, the interbeds units vary in thickness from 40 cm to ∼ 10 m, and preserve a variety of primary sedimentary structures (Fig. 2). The sandstones commonly display horizontal lamination (facies Sh) often with parting lineation, low-angle cross-bedding (facies Sl) and planar cross-bedding with foresets of up to ∼1 m in thickness (facies Sp). Massive layers (facies Sm) and soft-sediment deformation features are also common (Fig. 3). Within one succession, weakly defined upward fining of the grain size is common and, at least locally, ripple cross-laminated beds (facies Sr) terminate in surfaces that are covered by symmetrical ripple marks (Fig. 2a, d, e). Less commonly, the sandstones also contain matrix-supported conglomerates (facies Gmm), which are made up of ripped-up, sub-rounded mudstone-chip clasts that are 2–3 cm in diameter (Fig. 2f). Desiccation cracks (facies Dc) are also commonly present and so are raindrop impressions, especially along the clay-draped bedding planes of very thinly bedded, silty sandstones (Figs 2a, f, 3).
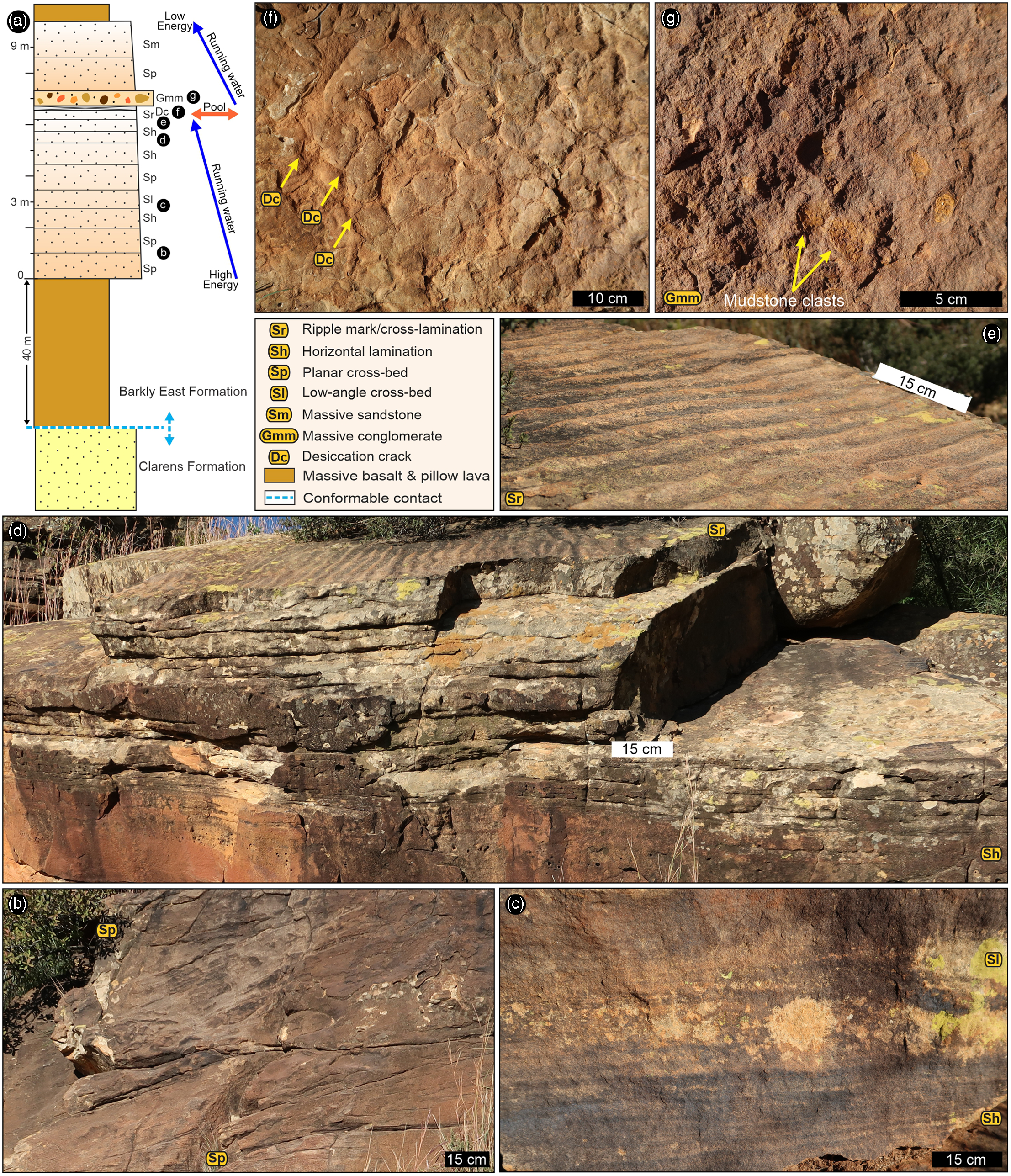
Fig. 2. Sedimentary facies of an interbed unit in the Lower Jurassic Drakensberg Group found <100 m above the top of the Clarens Formation at Upper Moyeni, Lesotho. For location, see Figure 1. (a) Representative centimetre-scale sedimentary facies log, with the stratigraphic position of some key sedimentary facies marked and shown in B–F. (b) Planar cross-bedding (facies Sp) in up to 1 m thick sandstone beds. (c) Horizontal lamination (facies Sh) and low-angle cross-bedding (facies Sl) in fine-grained sandstone. (d) Horizontal lamination (facies Sh) and ripple cross-lamination (facies Sr) below an upper bedding surface covered in symmetrical ripple marks. (e) Close-up of symmetrical ripple marks. (f) Desiccation cracks (facies Dc). (g). Light orange-ochre, sub-rounded, rip-up mudstone-chip clasts in matrix-supported conglomerates (facies Gmm).
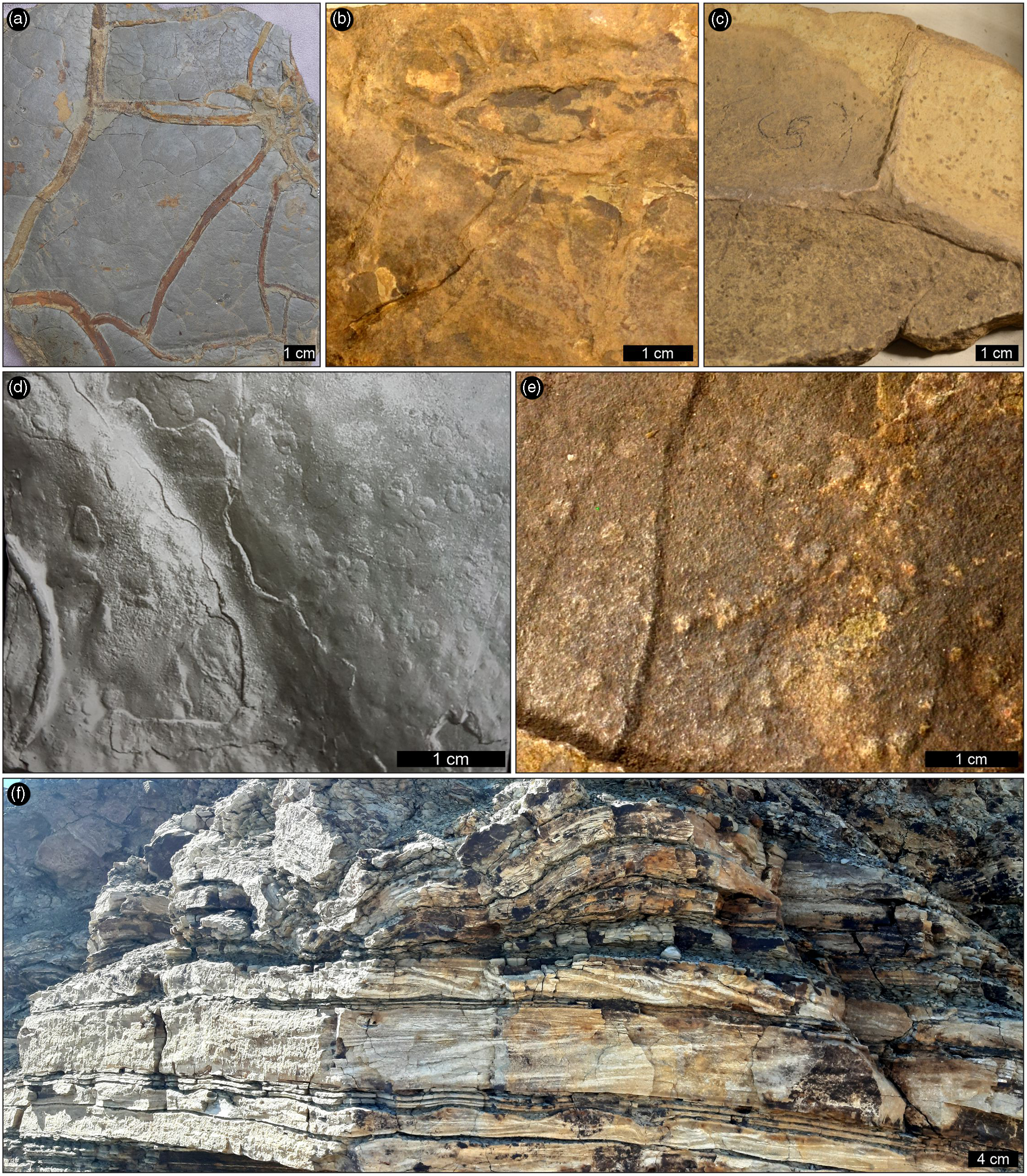
Fig. 3. Shallow-water indicators and water-escape structures in the sandstone interbeds in the Lower Jurassic Drakensberg Group of Lesotho. (a–c) Desiccation cracks found 100–200 m and 500 m above the top of the Clarens Formation at Makatseng, Khorobetloa (∼5 km north of Ralikhomo) and at Lekhalo-la-Maburu, respectively. (d, e) Raindrop impressions, cylindrical invertebrate trail (in d) and (f) water-escape structures (upper part of the image) found 100–200 m above the top of the Clarens Formation at Makatseng. Samples are previously unpublished materials stored in the Ellenberger Collection at the UoM in France, except for (a) and (f), which are discoveries arising from the present study. For locations, see Figure 1.
Overall, the sedimentary interbeds (Figs 1–3) in the lower Barkly East Formation suggest that when the outpouring of the lavas temporarily ceased at a given location, various sedimentary processes took over on the lava-covered land surface. The internal and external sedimentary facies characteristics of the fine- to medium-grained sandstone interbeds (Figs 2, 3) suggest that the sediments were deposited via fluvio-lacustrine processes. In particular, the association of desiccation cracks, raindrop impressions, and symmetrical ripples, together with the laterally restricted, mostly tabular, massive to cross-bedded sandstones, indicate both the shallowness and ephemeral nature of the depositional setting, and may have implications for the adaptations of trackmakers that frequented these areas and for the quality of their tracks.
Cross-stratification in the tabular, non-channelized sandstones (Fig. 2a, b) is evidence for moderate-energy traction currents in wide streams or sheet-like water bodies (i.e. sheetfloods; Gierlowski-Kordesch et al. Reference Gierlowski-Kordesch, Weismiller, Stigall, Hembree, Larsen, Egenhoff and Fishman2015). The cross-beds, with foreset thickness of up to ∼1 m, show down-current migrating subaqueous dunes that were at least 1 m in height, and that the flowing water was, at least locally, up to ∼2 m deep (Miall, Reference Miall2006). Horizontal lamination with parting lineation in fine-grained sandstones (Fig. 2c, d) is indicative of upper flow-regime conditions, whilst the desiccation cracks and raindrop impressions (Figs 2f, 3) are evidence for repeated drying out, and thus for the ephemeral nature of these water bodies. The symmetrical ripple marks atop the sandstones (Fig. 2d, e) point not only to the overall decrease in energy level with time but also to a transition in deposition from running to standing water. This is because in continental settings, symmetrical ripples represent weak, bidirectional, oscillatory currents commonly induced by wave action in a low-energy depositional setting like shallow lakes or pools (e.g. Evans, Reference Evans1941; Allen, Reference Allen1967). Moreover, the vertical grain-size decrease and change in sedimentary facies from the lower to the upper part of the interbed units (i.e. from Sp to Sh to Sr facies; Fig. 2) further show that the general energy level decreased within the deposition of a single interbed succession. The energy level fluctuation within the stream is also recorded by the clay-draped bedding planes as well as the rare matrix-supported conglomerates (facies Gmm; Fig. 2f). The latter facies is indicative of short-lived yet high-energy events that resulted in the scouring of pre-existing, desiccated and consolidated mud layer(s), and subsequent redeposition as mudstone chips. Common soft-sediment deformation features indicate the relatively rapid burial of the wet, unconsolidated sediment.
In summary, based on their sedimentary features and facies associations, the interbeds are evidence for the transition of fast-flowing but short-lived water currents with pulsating energy levels (i.e. from moderate- and upper-flow regime conditions to complete dry-out) into standing water bodies, like pools or ephemeral, shallow lakes. Within the interbeds, field evidence for unequivocal aeolian processes or root structures (but cf. Beukes, Reference Beukes and Haughton1970, p. 323 and Ellenberger, Reference Ellenberger and Haughton1970, p. 352, respectively) were not observed in this study. Moreover, on the balance of the field observations, the explicit association of the interbeds with geologically significant breaks in the lava outpouring is unjustifiable (see Discussion).
5. Palaeontology of the interbeds
5.a. Palaeoflora
Plant remains (Figs 1, 4, 5; Supplementary Material Table 1) in the interbeds range from cm- to dm-scale impressions of leaf-, shoot-, stem- and twig-like fragments of mostly herbaceous (e.g. horsetails) and coniferous species. The fossil plants are found predominantly a few hundred metres (<50–400 m) above the top of the Clarens Formation in the southern outcrop area (e.g. Ralikhomo, Leloaleng, Mohales Hoek), but they have been found up to 900 m from the base of the Karoo flood basalt pile (e.g. Mokhotlong; Ellenberger, Reference Ellenberger1975), and in other parts of southern Africa (Figs 1, 4, 5). Some of the collected fossil plants were briefly described and named by Ellenberger (Reference Ellenberger and Haughton1970; e.g. ‘Pinus vulcaniannensis’ cf. ‘Sphenolepidium leloalengensi’, ‘Equisetum cf. mokhotlongensis’), and others were discovered, although not collected, in recent years (e.g. at Mohales Hoek (Fig. 5)). To date, the plant remains have not been studied in depth (see Anderson & Anderson, Reference Anderson and Anderson1985).
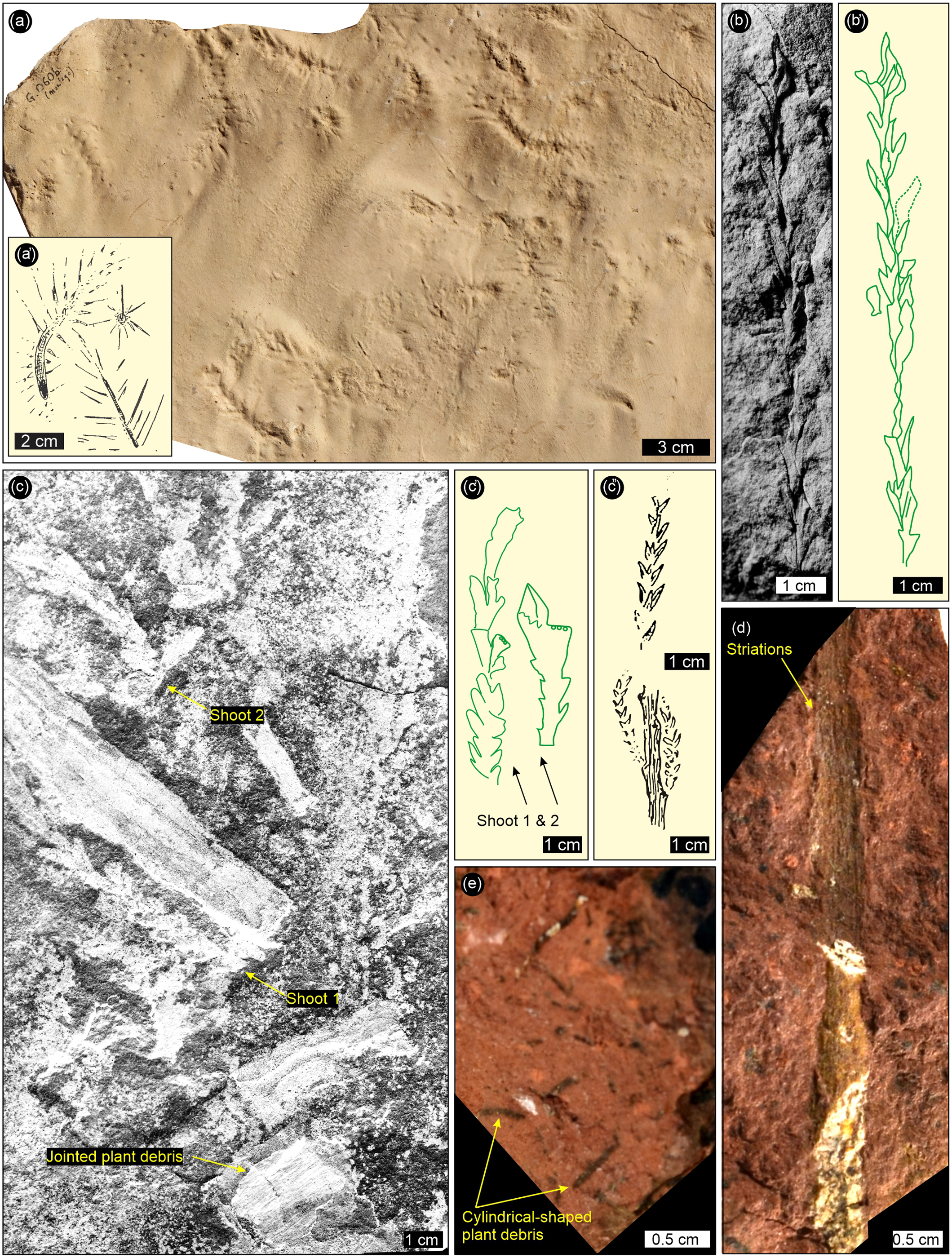
Fig. 4. Plant fragments from the sandstone interbeds in the Lower Jurassic Drakensberg Group of Lesotho. (a) Photo-textured 3D model of slab with curved ‘Pinus vulcaniannensis’ (Ellenberger, Reference Ellenberger and Haughton1970) shoots and fine needle-like leaves found 200–300 m above the top of the Clarens Formation at Ralikhomo. (a′) Interpretative outline drawing of parts of (a) by Ellenberger (Reference Ellenberger and Haughton1970, fig. 142B). (b) Coniferous plant foliage (?Brachyphillium) found <100 m above the top of the Clarens Formation at Leloaleng. (b′) Interpretative outline drawing of (b). (c) Jointed stem segments and shoots of cf. ‘Sphenolepidium leloalengensi’ (Ellenberger, Reference Ellenberger and Haughton1970) found <200 m above the top of the Clarens Formation at Leloaleng. (c′) Interpretative outline drawing of (c). (c″) Outlines (of sample partially shown in (c)) by Ellenberger (Reference Ellenberger and Haughton1970, fig. 133D). (d) Probable sphenophyte stem fragment with longitudinal striations (i.e. ribbed wall) and (e) cylindrical-shaped, randomly distributed plant debris with various diameters (part of the sample shown in (d)). (d) and (e) were collected 800–900 m above the top of the Clarens Formation at Mokhotlong and documented as ‘Equisetum cf. mokhotlongensis’ (Ellenberger, Reference Ellenberger and Haughton1970, Reference Ellenberger1975). Samples are previously unpublished materials stored in the Ellenberger Collection in the MM&A in Lesotho (only (a)) and UoM in France. For locations, see Figure 1.
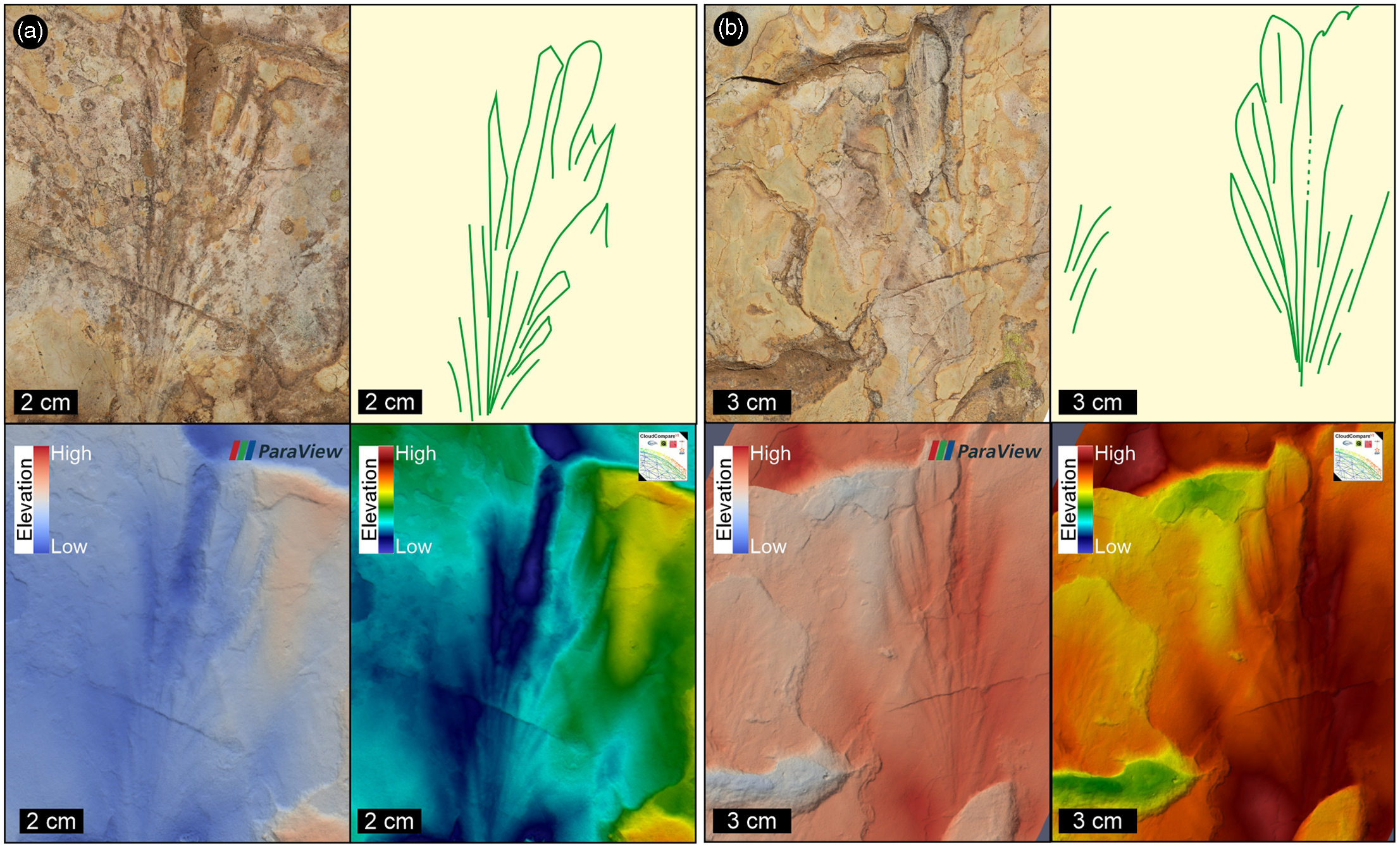
Fig. 5. Impression fossils of leafy shoot-fragments preserved in very fine-grained, silty sandstone interbed found <100 m above the top of the Clarens Formation at Mohales Hoek, Lesotho. For location, see Figure 1. The rounded, tongue-shaped fronds have strong mid-lines that fan out at acute angles. Specimens shown in (a) and (b) are, in the top row, photo-textured Agisoft 3D models, interpretative outline drawings, and in the bottom row, false-colour depth maps from ParaView (left) and CloudCompare (right). Blue to red height: 8 mm.
Evidence for forested areas with mature trees only exists in the lowermost part of the Karoo lava pile, close to or at the contact with the Clarens Formation (e.g. Stockley, Reference Stockley1940, p. 453; Bordy et al. Reference Bordy, Haupt and Head2021). Moreover, cm- to dm-scale layers and pockets of coaly, carbonaceous and charcoalified plant material associated with the lowermost interbeds was also reported at sites #10 and #12 (Fig. 1; also see e.g. du Toit, Reference du Toit1905, pp. 113, 120) as well as ∼40 km ESE of site #14 (M Bamford, pers comm., 2022). Considering the taphonomic conditions of interbeds (e.g. Jefferson et al. Reference Jefferson, Siders and Haban1983; Babcock et al. Reference Babcock, Leslie, Elliot, Stigall, Ford and Briggs2006; Garland et al. Reference Garland, Bannister, Lee and White2007) and noting that the conifers are typically thought to be trees, the extremely limited occurrence of fossil wood in the interbeds (Supplementary Material Table 1) may reflect a more scrubby, bushy habit in these plants. This and the overall morphological variation in coniferous–herbaceous fossil plants indicate a likely patchy vegetation cover of soft, herbaceous to hardy, bushy foliage during the deposition of the interbeds. Apparent charred plant material might be an indication of wildfires, as anticipated in active volcanic landscapes.
5.b. Palaeofauna
Invertebrate fossils in the interbeds are represented by microbially induced sedimentary structures, locally abundant bioturbation structures (burrows, tracks, trails) and body fossil impressions of branchiopods (clam shrimp or ‘conchostracans’) and other freshwater crustaceans (Figs 6, 7; Supplementary Material Table 1). The bioturbation structures can be attributed to isopods and other invertebrates with tubular or cylindrical body shapes. Stratigraphic distribution of the fossil invertebrates in the Karoo lava pile is comparable to that of the fossil flora (Fig. 1; Supplementary Material Table 1), and shows that invertebrate activity during the deposition of the interbeds was mainly limited to the early phase of lava pile emplacement, and mostly, but not only, in the southern study area (e.g. Ralikhomo, Mohales Hoek; Figs 1, 6, 7).
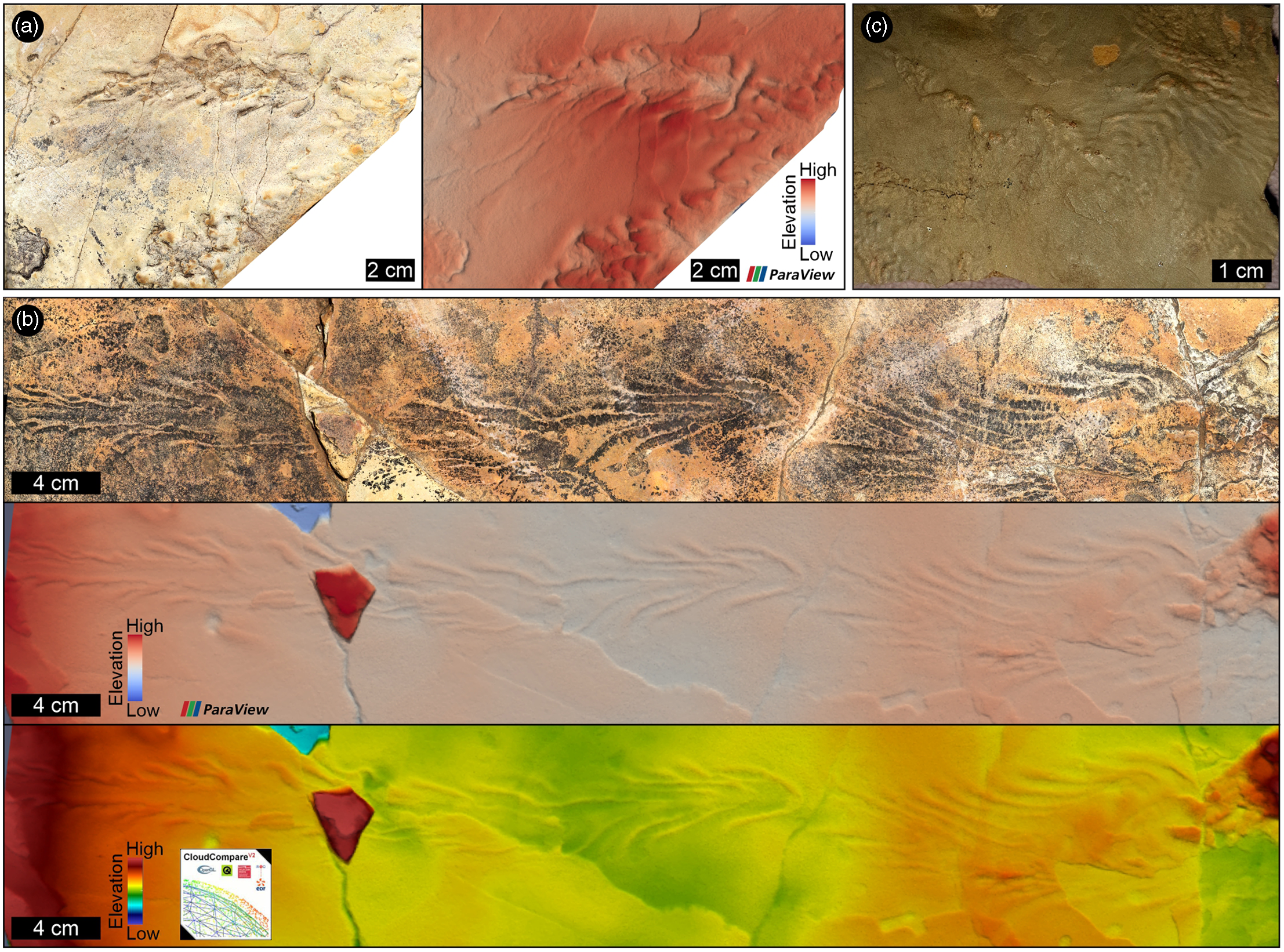
Fig. 6. Microbially induced sedimentary structures (MISS) in the sandstone interbeds in the Lower Jurassic Drakensberg Group in Lesotho. In (a) and (b), the chevron pattern in the microbial wrinkles likely formed when a current-dragged, twig-like object deformed the biofilm-covered substrate. (a) and (b) are the photo-textured Agisoft 3D models, and the false-colour depth maps from ParaView and CloudCompare (only (b)). Blue to red height: 7 mm. (a, b) and (c) are from the sandstone interbeds found <100 m above the top of the Clarens Formation (zone C1) at Mohales Hoek and Makatseng, respectively. For locations, see Figure 1.
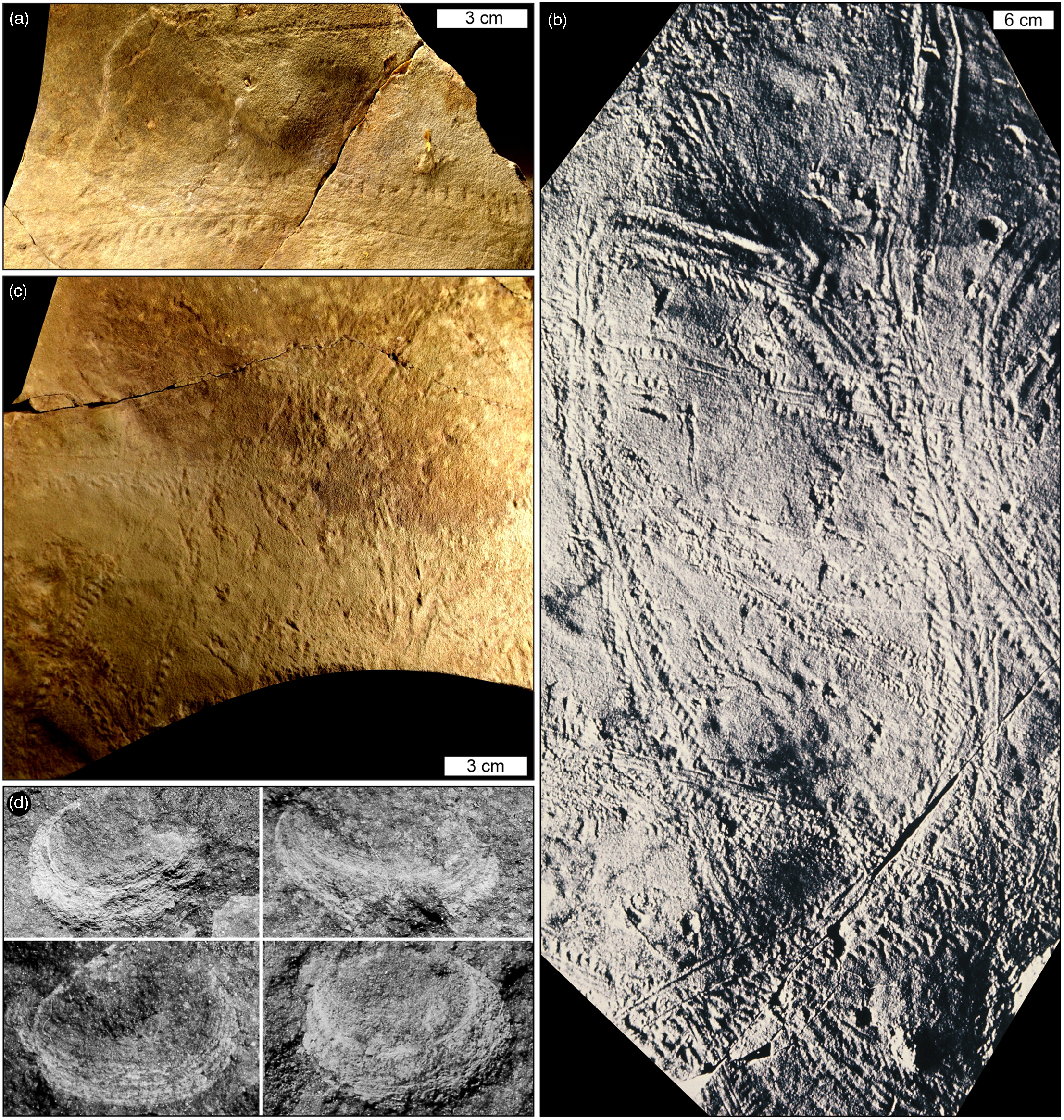
Fig. 7. Invertebrates and their traces from the sandstone interbeds in the Lower Jurassic Drakensberg Group in Lesotho. (a–c) Trackways were possibly made by freshwater crustaceans (likely isopods; cf. Uchman et al. Reference Uchman, Gazdzicki and Blazejowski2018) from the sandstone interbeds found 100–200 m above the top of the Clarens Formation (zone C2) at Makatseng. (a, b) ‘Apodidichnus mirabilis’ (Ellenberger, Reference Ellenberger and Haughton1970). (c) ‘A. mirabilis’ and ‘A. tenuis’ (Ellenberger, Reference Ellenberger and Haughton1970). (d) Four ‘conchostracan’ or branchiopod carapaces (clam shrimp: ?Estheria cf. drakensbergi; Ellenberger, Reference Ellenberger and Haughton1970) with an estimated valve length of ∼5 mm (original photos lack scale) found <200 m above the top of the Clarens Formation (zone C2) at Leloaleng. Samples are previously unpublished materials stored in the Ellenberger Collection at the UoM in France. For location, see Figure 1.
Although the surface textures shown in Figure 6 may be regarded as abiotic primary sedimentary structures (e.g. Noffke et al. Reference Noffke, Gerdes, Klenke and Krumbein2001; Davies et al. Reference Davies, Liu, Gibling and Miller2016), we consider them as microbially induced sedimentary structures (MISS) that formed when algal biofilms actively modified the fine-grained silty sandy substrate, i.e. they are ‘traces … left by microorganisms’ (sensu Noffke Reference Noffke2010, p. 4). These putative interbed MISS or microbial wrinkles are listed here because they co-occur with a variety of other Drakensberg fossils (e.g. plant impressions, tracks) as well as sedimentary structures indicative of shallow water conditions, and as such are considered trace fossils (see Stimson et al. Reference Stimson, Miller, MacRae and Hinds2017). Microbial wrinkles are common in low-energy, aquatic settings in various continental environments as well (e.g. seasonal streams, ponds, lakes (Noffke, Reference Noffke2010; Davies et al. Reference Davies, Liu, Gibling and Miller2016)). Studies on mechanisms of formation and the role of MISS in ancient ecosystems show that microbial binding is not only essential in sediment stabilization but can also enhance the fossilization of other biota, particularly tracks (e.g. Carvalho et al. Reference Carvalho, Borghi and Leonardi2013). The locally profuse occurrence of isopod and trace fossils (locomotion and feeding traces (e.g. Fig. 7a–c; Supplementary Material Table 1)) suggests highly nutritious sediment substrates, whereas the body fossils of freshwater crustaceans (Fig. 7d; Supplementary Material Table 1) are again fair proxies for shallow water bodies. While most of the interbed invertebrate fossils, on their own, are ambiguous palaeoenvironmental indicators, their association with diagnostic sedimentological (e.g. raindrops) and palaeobotanical (e.g. herbaceous remains) evidence collectively reiterates that the host sediments formed in short-lived and likely shallow freshwater lakes and abandoned streams.
Reinvestigation of the tetrapod ichnofauna in the Drakensberg Group (Supplementary Material Table 1; Fig. 8) as reported by Ellenberger (Reference Ellenberger and Haughton1970, Reference Ellenberger1975) has proved challenging, for several reasons: (1) despite Ellenberger’s important pioneer work in remote locations, most of his results were published prior to the tetrapod ichnology renaissance that began in the 1980s; (2) as a result, Ellenberger was a ‘splitter’ strongly inclined to provincial ichnotaxonomy, and he is today regarded as having named too many difficult-to-distinguish traces, which, based on present standards and quality of preservation scales (e.g. Belvedere & Farlow, Reference Belvedere, Farlow, Falkingham, Marty and Richter2016), would not be considered suitable for formal ichnotaxonomic assignments; and (3) more importantly, prior to the recent work of the present team and colleagues, many of the tracksites he described have not been reinvestigated in detail, nor have the specimens (originals and replicas) he collected been restudied.
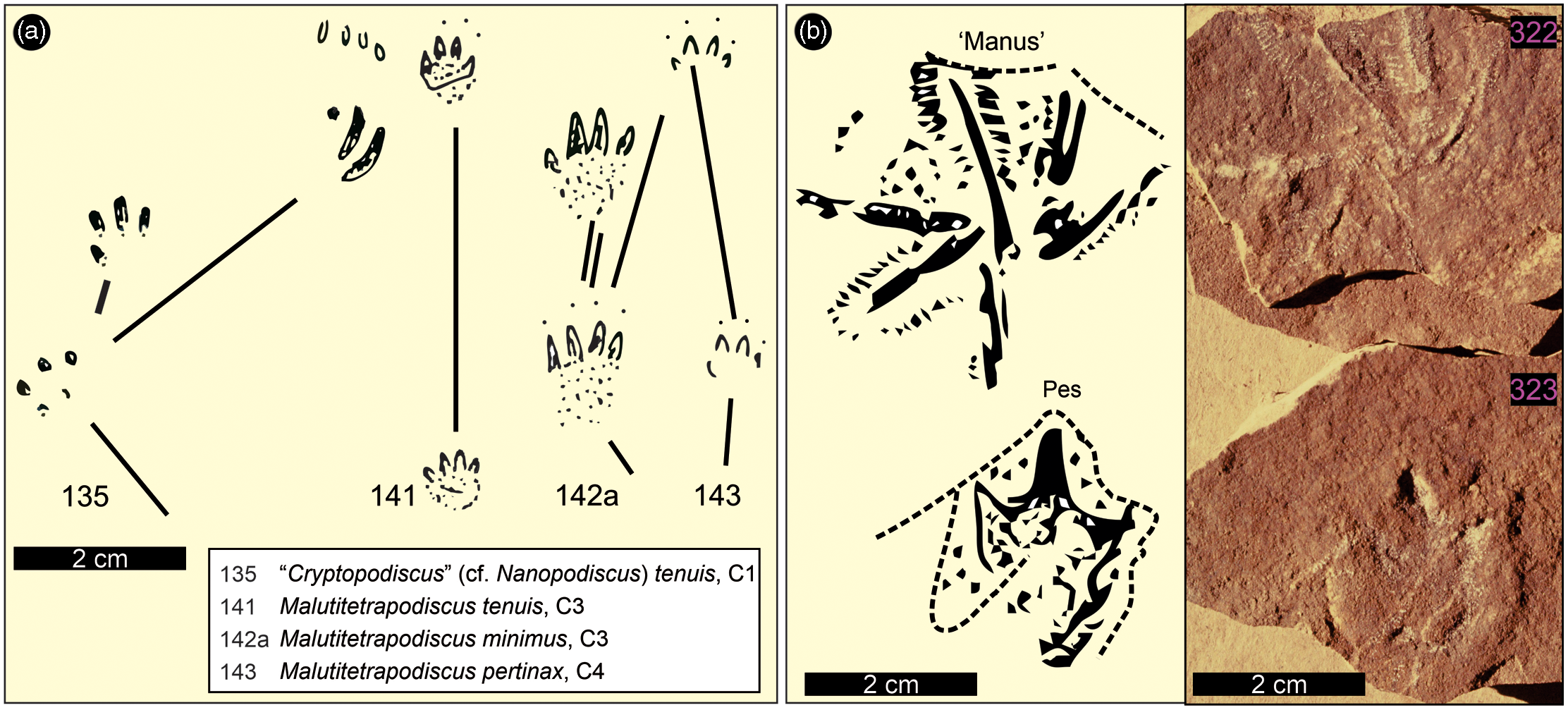
Fig. 8. Vertebrate track outlines from the Lower Jurassic Drakensberg Group of Lesotho as documented by Ellenberger (Reference Ellenberger and Haughton1970, Reference Ellenberger1974). (a). ‘Cryptopodiscus’ (cf. Nanopodiscus) tenuis, Malutitetrapodiscus tenuis, M. minimus, M. pertinax (Ellenberger, Reference Ellenberger and Haughton1970, figs 135, 141, 142a, 143). Specimens are from Leloaleng (135), Ralikhomo (141, 142a) and Lekhalo-la-Maburu (143). (b) Ralikhomopus aviator (Ellenberger Reference Ellenberger1974, plate O: fig. 140A) and a previously unpublished photograph of specimens LES 322 and 323. For locations, see Figure 1; for specimen details, see Supplementary Material Table 1; for photogrammetric models, see Supplementary Material Figure B. Note that M. saltator (Ellenberger, Reference Ellenberger and Haughton1970, fig. 140) is shown in Figure 9 herein.
As we reiterated in our previous study (Bordy et al. 2020, p. 5), the Karoo continental flood basalt pile yielded ‘mostly small, tetradactyl tracks … attributed to mammalians … assigned to the ichnogenus Malutitetrapodiscus (e.g. M. saltator, M. tenuis, M. minimus, M. perlinax…) … [and] … one tridactyl track, Ralikhomopus aviator … attributed to … small, bird-like dinosaurs by Ellenberger … which he called [the] “tiny proto-avian of Drakensberg”’. For sake of completeness, these Ellenbergian tracks are reproduced herein in Figures 8, 9 and Supplementary Material Figure B, and a relevant excerpt from Ellenberger (Reference Ellenberger and Haughton1970, p. 352) is given as follows: ‘Malutitetrapodiscus saltator (32 × 32 mm) progressed by jumping with both feet (in leaps of 29 cm). The “pes” has 4 splayed digits ending in fine claws arching towards the ground. Another trackway indicates a gallop with four limbs touching the ground at the same point (Fig, 140). M. tenuis (8 – 10 × 10 mm) (Fig. 141), M. minimus (7 × 8 mm). The step is only 50 mm, the claws on the ground (Fig. 142 A).’
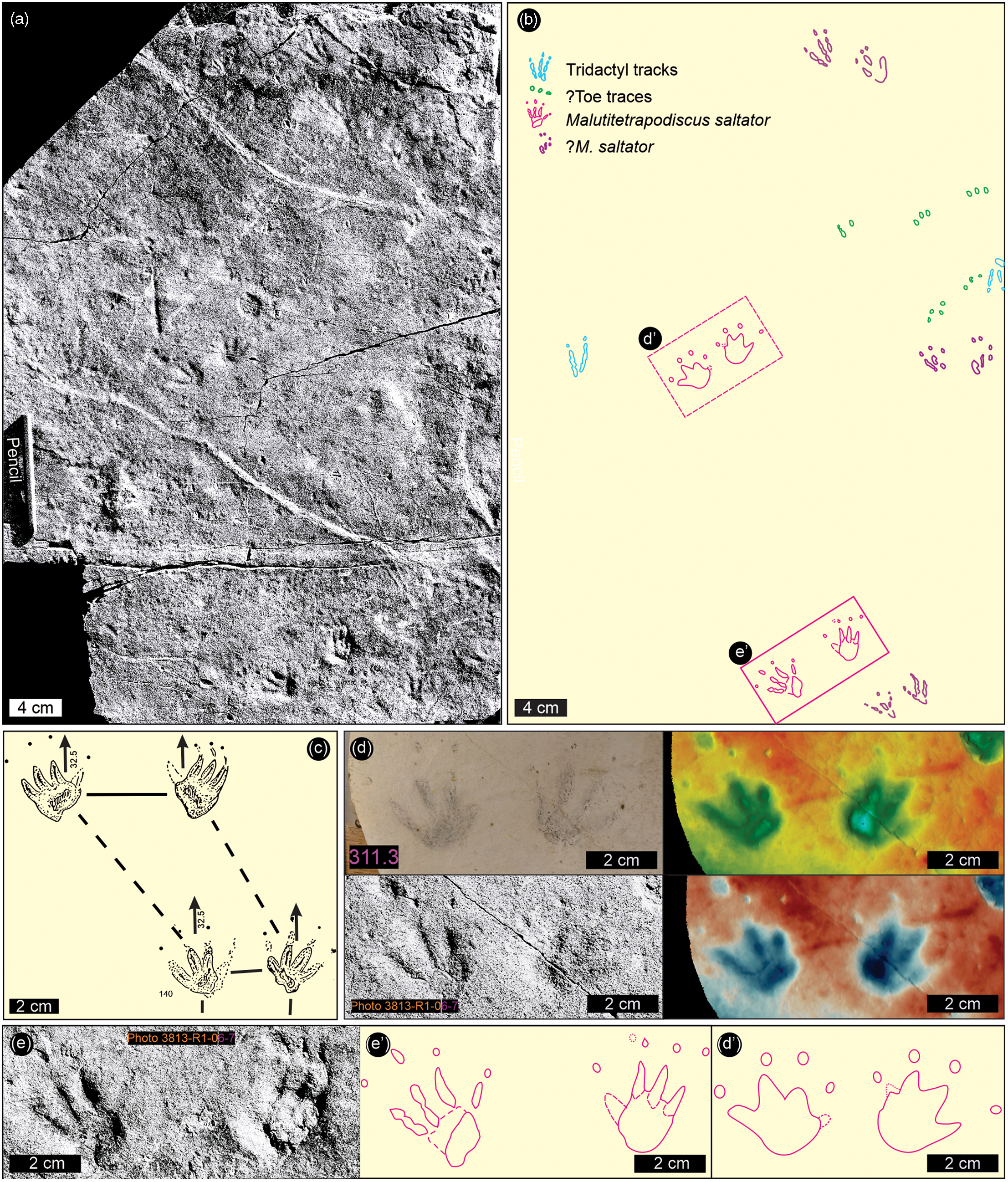
Fig. 9. Malutitetrapodiscus saltator – tetradactyl synapsid and other tracks from Ralikhomo (200–300 m; zone C3), Lesotho. (a) Previously unpublished field photograph by Ellenberger of the tracking surface with holotype M. saltator trackway comprising two conspicuous pairs of side-by-side tetradactyl tracks in ‘hop set’ configuration. (b) Two ‘hop set’ pairs and other vertebrate track outlines at same scale as in (a). (c) Original illustration of the trackway by Ellenberger (Reference Ellenberger and Haughton1970, fig. 140) shows distortion inconsistent with his measurements given for hop distance. (d & d′) Detail of distal ‘hop set’ pair based on UoM plaster cast (LES 311.3), Ellenberger’s black-and-white field photo, and false-colour depth maps (on the far right: CloudCompare and ParaView) and outline traced from overlay (bottom right). (e, e′) Detail of proximal ‘hop set’ pair based on Ellenberger’s black-and-white photo and outline traced from overlay (bottom middle). See Figure 11 for comparison with well-authenticated trackways of small hopping tetrapods. For location, see Figure 1. Blue to red height: 9 mm.
Prior to the present study, no photograph of M. saltator was available to confirm the jumping (saltation) gait, suggested by the illustration presented by Ellenberger (Reference Ellenberger and Haughton1970, fig. 140), which shows a distorted view, with the hop (leap) distance too short and veering to the left. This distortion appears to have been done to fit the outlines of two consecutive ‘hop sets’ onto a page crowded with other illustrations. However, the discovery of the photo shown in Figure 9a clarifies the true trackway pattern by confirming the proportions described by Ellenberger in describing the tetradactyl tracks (∼32 mm long and wide) as comprising two conspicuous ‘hop set’ pairs with a hop (leap) distance of ∼29 cm. One ‘hop set’ is preserved as a replica that serves as the holotype (Fig. 9d herein).
5.c. The Ralikhomo quadruped trackway
The most conspicuous feature of the Ralikhomo tracksite is a single trackway consisting of seven consecutive Batrachopus manus–pes sets representing a trackmaker with quadrupedal progression and pes larger than manus (i.e. clear heteropody). Some of the tracks contained infill from an overlying layer. The tracks are moderately well-preserved, showing up to four diagnostically configured pes digit impressions and up to three manus digit traces.
The pes is longer (L) than wide (W) with mean L, W and L/W of 5.94 cm, 4.80 cm and 1.24, respectively. Corresponding values for the manus are 2.72 cm, 3.28 cm and 0.83, indicating a manus wider than long. The pes shows strong outward rotation (mean 27°). The trackway is fairly narrow (mean outer trackway width for pes 9.52 cm) with a mean step and stride of 22.7 cm and 42.4 cm respectively and a variable pace angulation between 180° and 130° (mean 155.2°). As discussed below, these features are fairly typical of Batrachopus (Figs 8, 10; Table 1).
Table 1. Morphometric parameters for the Batrachopus trackway at Ralikhomo. For location, see Fig. 1
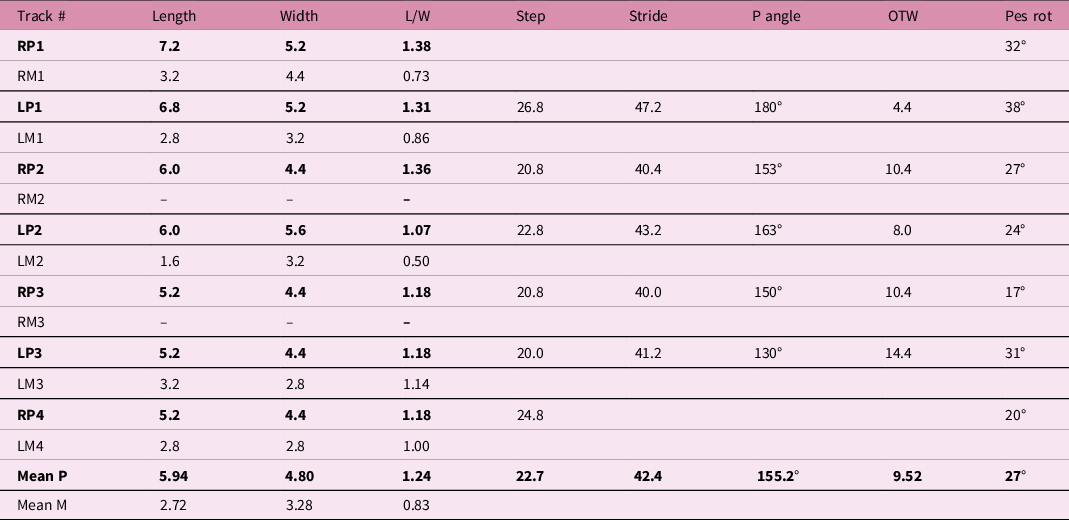
Abbreviations: R = right; L = left; P = pes; M = manus; P angle = pace angulation; OTW = outer trackway width for pes; pes rot = pes rotation.
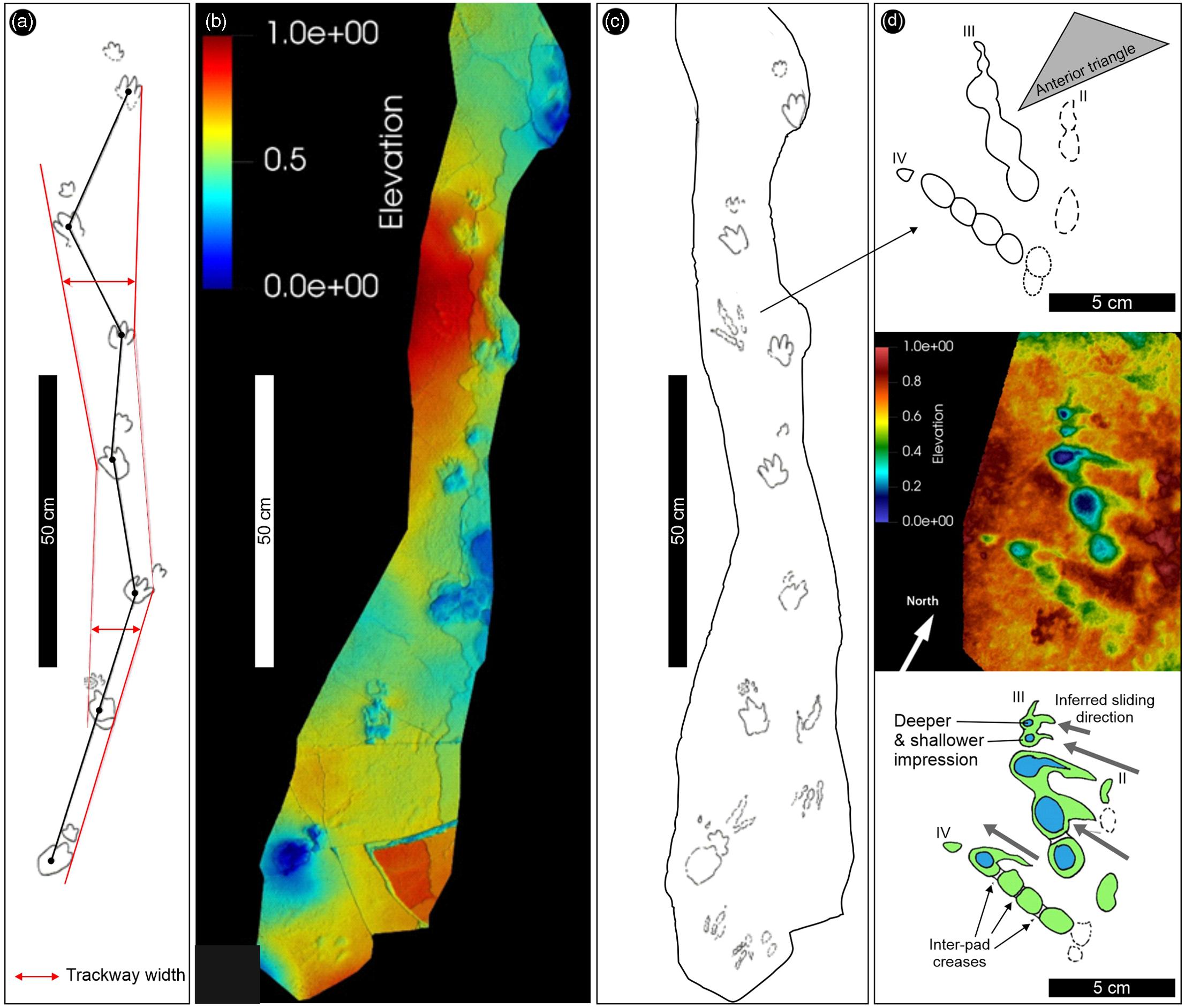
Fig. 10. Details of the Batrachopus-bearing trackway surface found 200–300 m above the top of the Clarens Formation at Ralikhomo. (a) Interpretative trackway outline showing variable trackway width and a slight turn to left. (b) False-colour depth maps from CloudCompare of the trackway surface showing faint traces of small tridactyl tracks. (c) Field tracing of the trackway surface showing outlines of faint tridactyl tracks as well. (d) Enlarged detail of small, shallow but well-preserved tridactyl track with the false-colour depth map (CloudCompare) in the middle, and interpretative outline drawings above and below. Note faintness of digit II impression and possible slide marks with the inferred sliding direction. Inter-pad creases are shown on digits III and IV. Blue to red height: 10 mm. See text for details. For location, see Figure 1.
5.d. Biped tracks at the Ralikhomo tracksite
The well-preserved Batrachopus trackway is associated with complete tridactyl dinosaur footprints, partial tracks as well as other small toe traces. Some of these are similar to some of the tracks shown in Ellenberger’s field photographs (Fig. 9a). The best-preserved tridactyl track (Fig. 10c, d) has a length (L) and width (W) of 10.0 cm and 7.5 cm respectively (L/W = 1.33). The track has weak mesaxony (anterior triangle L/W is 0.4 determined from the distal end of digital pads, not claw traces), which is more typical of Anomoepus than Grallator, although this feature alone is of limited use in a sample of one (see below). Digit divarication is ∼ 24° between digits II and III, and 31° between digits III and IV (II–IV = 55°).
Digit III registered the deepest trace, with three digital pad impressions, and the claw trace is well-defined (cf. Avanzini, Reference Avanzini1998). Likewise, the four equidimensional digital pad traces and distal claw are well-preserved, with the most distal pad most deeply impressed. These phalangeal pad traces appear to be separated by rather wide, double, inter-pad creases, as seen in many Anomoepus specimens (e.g. Olsen & Rainforth, Reference Olsen, Rainforth, LeTourneau and Olsen2003, figs 19.4–19.6, 19.9). Such ‘double’ creases are not typical of Grallator. There appears to be an additional metapodial pad trace postero-lateral to the four equidimensional digit IV pad traces. Arguably, this can be seen in some of the Newark Group Anomoepus including specimens figured by Olsen and Rainforth (Reference Olsen, Rainforth, LeTourneau and Olsen2003, fig. 19.23) as well as in an illustration by Hitchcock (Reference Hitchcock1844, pl. III: fig. 4), thus suggesting a 2-3-5 phalangeal pad formula for digits II, III and IV rather than the typical 2-3-4 formula of theropods. However, it is possible that these shallow traces represent some other, interfering trace as it is not obviously diagnostic of either small theropod or ornithischian tracks such as Grallator and Anomoepus, respectively. Although shallow, and not recognized in the field when the sun was overhead, the quality of preservation is very good and could be ranked as 3 on the four-point (0-1-2-3) scale proposed by Belvedere and Farlow (Reference Belvedere, Farlow, Falkingham, Marty and Richter2016).
The distal-most pad of digit IV and the two most distal pads of digit III show what appear to be slide marks oriented obliquely, at c. 45° across the alignment of the track (i.e. digit III) axis from postero-medial to antero-lateral (Fig. 10d). This orientation is consistent in the case of all three pads, as well as the distal digit III claw traces. The slide marks appear to widen in the antero-lateral direction towards the final pad registration position. As the track-bearing surface consists of a very thin clay drape over a very thinly bedded and laminated sandstone (∼1 cm in thickness) with a flat upper bedding plane, it is possible that these inferred slide marks represent the antero-lateral motion of the foot as it came into contact with the substrate. This inference appears consistent with the greater track registration depths of the anterior portions of the traces of digits III and IV and the very weak registration trace of digit II, i.e. weight/force was preferentially directed outward towards digit IV, which is more divergent from digit III than digit II is. In addition to this ‘best-preserved’ tridactyl track, there are at least four other shallow faint tridactyl tracks preserved at the southern end of the track-bearing surface (Fig. 10d). It is not easy to determine whether these represent Grallator-like or Anomoepus-like morphotypes (see Discussion).
6. Discussion
6.a. Palaeoenvironment and palaeoclimate
Sedimentological and palaeontological evidence from the Karoo interbeds comprises an association of ancient aqueous dunes with raindrop impressions, symmetrical ripples, desiccation cracks, as well as tracks of invertebrates and vertebrates, plant and animal fossils. These collectively demonstrate not only the ephemeral nature but also the shallowness of the water in which the thin (<10 m), laterally restricted (<400 m), tabular to lenticular interbeds were generated. Although most of the running and standing water bodies were shallow and narrow, field evidence also shows (e.g. Bordy et al. Reference Bordy, Haupt and Head2021) that some lakes were up to 10 m deep and facilitated the development of lava-fed deltas. Based on the body and trace fossils as well as sedimentological evidence (see Results), the shallow lakes may be assumed to have been short-lived (e.g. Stigall et al. Reference Stigall, Hembree, Gierlowski-Kordesch and Weismiller2014 but cf. Hethke, Reference Hethke2014; Olsen, Reference Olsen2016; Hegna & Rogers, Reference Hegna and Rogers2020). While estimating the longevity of the deeper lakes is impracticable, facies studies of Namibian interbeds suggest that at least some of the lakes were relatively permanent (Gierlowski-Kordesch et al. Reference Gierlowski-Kordesch, Weismiller, Stigall, Hembree, Larsen, Egenhoff and Fishman2015).
In addition to the above-mentioned sedimentary proxies indicative of running and standing water, evidence for diverse biotic activities across southern Africa also reveals sufficiently humid conditions that were favourable for the: (1) development of microbial mats; (2) sustained growth of soft, herbaceous plants and hardy, bushy shrubs, as well as (3) supply of potable water for small to medium-sized tetrapods. Therefore, this study confirms that the warm temperate, and at least seasonally wet, climatic conditions shown for southern Gondwana in the Late Pliensbachian (see Bordy et al. Reference Bordy, Haupt and Head2021) were still in existence at the onset of the Karoo volcanism (also see e.g. Gierlowski-Kordesch et al. Reference Gierlowski-Kordesch, Weismiller, Stigall, Hembree, Larsen, Egenhoff and Fishman2015; Bordy et al. Reference Bordy, Rampersadh, Abrahams, Lockley and Head2020 b). These seasonally wet, warm temperate conditions agree with the moderately warm, humid (i.e. non-arid but rather temperate or warmer) and strongly seasonal climate interpreted for the neighbouring Ferrar region (e.g. Bradshaw, Reference Bradshaw1987; Elliot & Hammer, Reference Elliot, Hammer and Mitra1996; Garland et al. Reference Garland, Bannister, Lee and White2007; Elliot et al. Reference Elliot, White and Fleming2021).
While the presence of truly permanent lakes in this region of the Karoo–Ferrar LIP is not supported by the data reviewed herein, the temporary lakes and watercourses at the turn of the Pliensbachian–Toarcian likely wielded a substantial impact on the dynamics of the phreatomagmatic/pyroclastic and flood basalt processes that resulted from hydrothermal vent complexes to lava deltas (e.g. Marsh, Reference Marsh1984; McClintock et al. Reference McClintock, Marsh and White2008 a, b; Moulin et al. Reference Moulin, Fluteau, Courtillot, Marsh, Delpech, Quidelleur, Gérard and Jay2011, Reference Moulin, Fluteau, Courtillot, Marsh, Delpech, Quidelleur and Gérard2017; Bordy et al. Reference Bordy, Haupt and Head2021). Moreover, in our view, these temporary aquatic to semi-aquatic continental settings, associated with fast-flowing streams and up to 10 m deep lakes and ponds, are inadequate for assessing the depth of the regional palaeo-groundwater level in flood basalt terrains (cf. Gierlowski-Kordesch et al. Reference Gierlowski-Kordesch, Weismiller, Stigall, Hembree, Larsen, Egenhoff and Fishman2015). This is because in these volcanic landscapes, built by rapid extrusion of profuse amounts of lava that accumulated in complex aggradational patterns (i.e. volcanic topography built by both jacking-up/inflating and simple piling-up (Jay et al. Reference Jay, Marsh, Fluteau and Courtillot2018)), the relative base level may be decoupled from the regional palaeo-groundwater level, which during voluminous eruptions may rapidly end up several tens of metres below the land surface of the thickening lava pile. In these dynamic volcano-sedimentary settings with complex constructional patterns of the landscape, the position of the relative base level (i.e. palaeo-groundwater table) had a lesser effect on the preservation of the sedimentary interbeds (and their fossil biota) than volcanic entombing and subsequent, hydrothermally driven diagenetic/taphonomic processes (i.e. ‘preservational paradox’: Jefferson et al. Reference Jefferson, Siders and Haban1983; Babcock et al. Reference Babcock, Leslie, Elliot, Stigall, Ford and Briggs2006; Garland et al. Reference Garland, Bannister, Lee and White2007).
6.b. Hopping, skipping or jumping: trackway evidence of behaviour
According to Ellenberger (Reference Ellenberger and Haughton1970, Reference Ellenberger1975), the trackway of Molapopentapodiscus saltator represents a hopping or jumping mammalian trackmaker, as the name implies, found in his zone B/4 (which is the transition of the upper Elliot and Clarens Formation). Thus, this report represents a stratigraphically older (Sinemurian) occurrence than the Drakensberg trackway, detailed above, which he named ‘Malutitetrapodiscus saltator’ and which is purported to represent a hopping mammal trackmaker from zone C/3 (note same trivial ichnospecies name for different ichnogenera and the frequent use of ‘salt’ root to indicate jumping). Such trackway evidence of hopping (saltating) has proved intriguing for two reasons: first, such trackways are rare in the fossil record, and second, they suggest an early origin (at least Early Jurassic) for this type of gait among small early, rodent-sized mammals. (It cannot be assumed that such trackmakers were rodents, only that they appear to represent rodent-sized animals. Indeed, as noted below it cannot even be assumed the trackways represent mammals.)
Van Dijk (Reference Van Dijk1978, p. 113) stated ‘that bipedal hopping sequences are in evidence’ in the lower Clarens Formation and illustrated two examples (his figs 3, 4), while following Ellenberger (Reference Ellenberger and Haughton1970, Reference Ellenberger1975) in naming them Molapopentapodiscus saltator. Only the uncatalogued specimen shown in his figure 4 shows a paired configuration that could be construed as evidence of a hopping gait (see Fig. 11 herein); however, even in this case, the pairing across the trackway mid-line is not symmetrical. Van Dijk’s interest in such trackways is highlighted in a short paper catchily entitled ‘Jurassic bipeds that could hop? Perch? Pounce? Fly?’ (Van Dijk, Reference Van Dijk2001) in which he cited tracks attributed to the two aforementioned ichnogenera (Molapopentapodiscus and Malutitetrapodiscus) as the prime examples of evidence for hopping gaits; he included an illustration of a ‘synthesized’ trackway of Molapopentapodiscus (M. supersaltator). Note that Ellenberger (Reference Ellenberger and Haughton1970, plate X1; 1975, plate III) distinguished two ichnospecies (M. saltator and M. supersaltator) evidently based on track size and length of hop (12 cm and 20 cm, respectively, according to notations on his plates). Van Dijk (Reference Van Dijk2001, fig. 2) included a stereo pair of tracks labelled M. saltator, which were re-illustrated by Van Dijk and Eriksson (Reference Van Dijk and Eriksson2021, fig. 2) as the holotype of the new ichnospecies Saltirecarpipes tinleyi (specimen NMQR 3949, National Museum Bloemfontein). As discussed below, this ichnotaxon bears a striking resemblance to the ichnogenus Gwyneddichnium from the Upper Triassic of Utah (Lockley, Reference Lockley2006), and as such may not represent a hopping mammal at all.
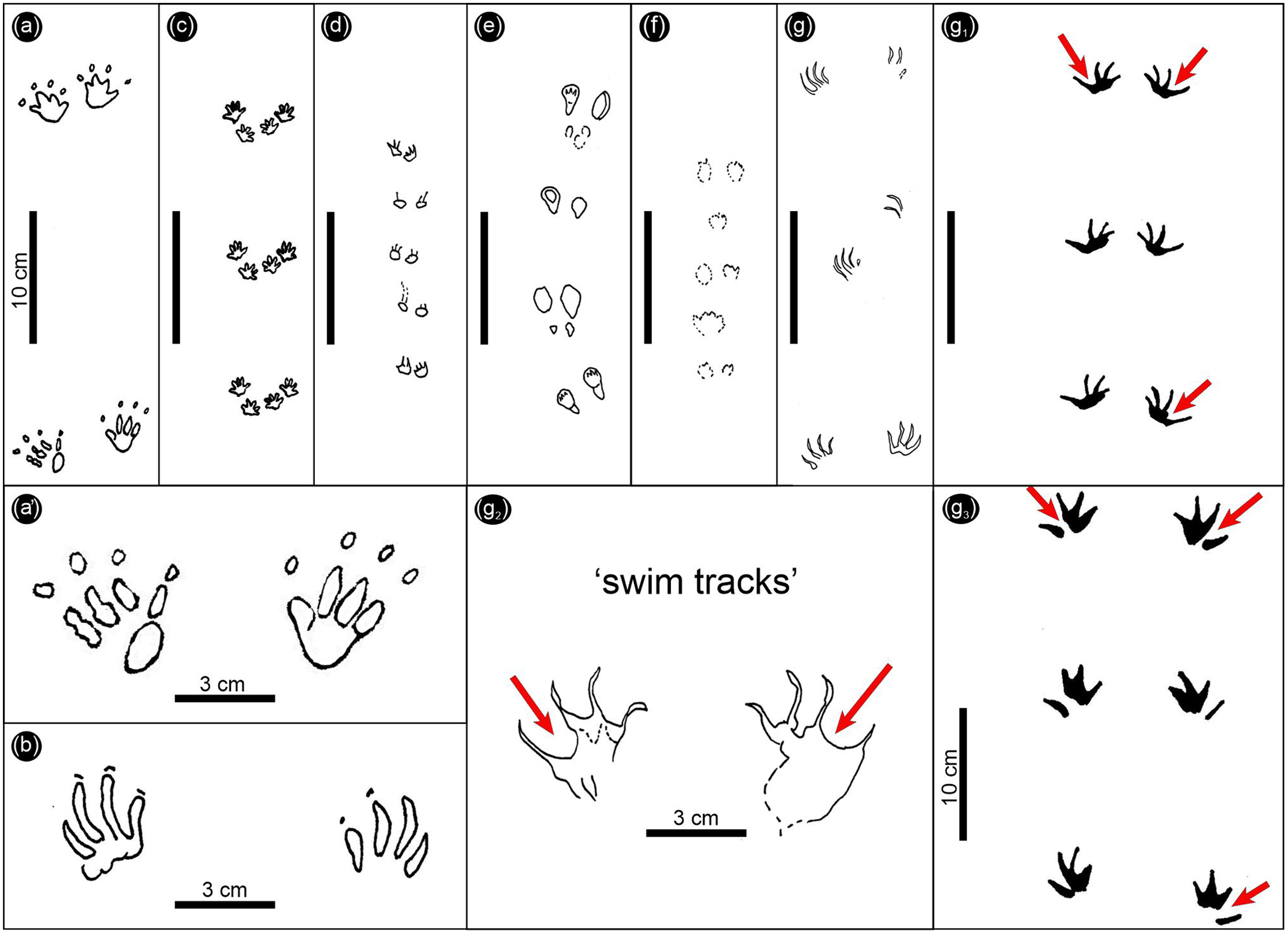
Fig. 11. Fossil mammal trackways inferred to represent hopping or bounding gaits. (a, a1) Malutitetrapodiscus saltator (Early Jurassic, Pliensbachian–Toarcian). (b) Molapopentapodiscus supersaltator (Early Jurassic, Sinemurian). (c) Ameghinichnus patagonicus (Middle Jurassic). (d) Koreasaltipes jinjuensis (Early Cretaceous). (e) Musaltipes occidens (Miocene). (f) Musaltipes ichnosp. indet. (Pleistocene). (g, g1) Molapopentapodiscus supersaltator. (g2) Holotype of Saltirecarpipes tinleyi. (g3) Gwyneddichnium isp. Trackways in the g–g3 series are interpreted as swim tracks. Red arrows in g1–g3 indicate pronounced digit III–IV hypicies.
Before discussing Saltirecarpipes tinleyi further, it is important to place these trackways of purported hopping mammals, or other tetrapods, in perspective in the track record as a whole. There have been very few reported examples of well-preserved fossil trackways attributed to hopping mammals. These can be briefly summarized, using only unequivocal examples, as shown in Figure 11. For ease of comparison, all are illustrated at the same scale, and include Malutitetrapodiscus saltator, here illustrated for the first time using one of Ellenberger’s original photographs (Figs 9a, 11a, a1); Molapopentapodiscus supersaltator (sensu Ellenberger, Reference Ellenberger and Haughton1970, plate X1; 1975, plate III; Fig. 11b herein); Ameghinichnus patagonicus (Casamiquela, Reference Casamiquela1961; De Valais, Reference De Valais2009; Fig. 11c); Koreasaltipes jinjuensis (Kim et al. Reference Kim, Lim, Lockley, Xing and Choi2017; Fig. 11d); Musaltipes occidens Lockley and Milner (Reference Lockley and Milner2014; Fig. 11e); and Musaltipes ichnosp. indet. (Lockley et al. Reference Lockley, Helm, Cawthra, De Vynck, Dixon and Venter2022; Fig. 11f).
As shown in Figure 11g–g3, there are four trackways which are of doubtful mammalian affinity. Trackways labelled as Molapopentapodiscus supersaltator (Fig. 11g, g1) after Van Dijk (Reference Van Dijk1978 and Reference Van Dijk2001, respectively) show a remarkable resemblance to Gwyneddichnium swim tracks (Lockley, Reference Lockley2006; Fig. 11g3) known from the Upper Triassic of Utah. Among the notable points of comparison between this tetradactyl track type is the apparent web between digits I and II and between II and III, but not between III and IV where there are deep hypicies (red arrows in Fig. 11g1–g3). The statement that this ‘group of tracks is characterized by a very long outer digit and three inner digits grouped closer together’ (Van Dijk & Eriksson, Reference Van Dijk and Eriksson2021, p. 5) is strikingly reminiscent of the statement regarding diagnostic features of Gwyneddichnium that ‘digits I–III are separated from digit IV by a pronounced gap’ (Lockley, Reference Lockley2006, p. 172). The evidence of web traces alone has significant implications, i.e. suggesting an aquatic trackmaker rather than a terrestrial hopping mammal (sensu Ellenberger, Reference Ellenberger and Haughton1970, Reference Ellenberger1975) or dinosaur (sensu Van Dijk & Eriksson, Reference Van Dijk and Eriksson2021).
It is confusing that the same tracks named and illustrated as Saltirecarpipes tinleyi by Van Dijk and Eriksson (Reference Van Dijk and Eriksson2021, fig. 2; Fig. 11g herein) were originally illustrated and named Molapopentapodiscus supersaltator (Van Dijk, Reference Van Dijk2001, figs 1, 2; Fig. 11g–g1 herein). The former paper does not list M. supersaltator as referred material as part of the ichntaxonomic revision, and incorrectly stated that this material had been referred to as a ‘New Genus’ (Van Dijk & Eriksson, Reference Van Dijk and Eriksson2021, p. 5). It may have been the intent of Van Dijk (Reference Van Dijk2001) to anticipate describing the new ichnotaxon (Saltirecarpipes tinleyi) since it is subsequently stated that ‘it is the fourth, outermost digit seen in the Saltirecarpipes tracks that is very different from any of the other inferred hopping taxa’ (Van Dijk & Eriksson, Reference Van Dijk and Eriksson2021, p. 7).
Trackways shown in Figure 11b, g, g1, both apparently originally labelled as M. supersaltator, differ only in the openness of the II–IV hypicies, with the former trackway (g) being less open than the latter (g1). In this regard, as stated, we consider the tracks, especially as illustrated in Fig. 11g1, as quite different from the mammaliform morphotypes (Fig. 11a, c–f), i.e. they are Gwyneddichnium-like, and we do not agree that ‘Saltirecarpipes appears to have been a bipedal hopping dinosaur’ (Van Dijk & Eriksson, Reference Van Dijk and Eriksson2021, p. 7). Gwyneddichnium has traditionally been attributed to an aquatic Tanytrachelos-like trackmaker (Lockley, Reference Lockley2006 and references therein). The slenderness and curvature of the digit traces both suggest very flexible digits, and the evidence of a semi-web between digits (I and III) both in the South African and North American ichnites strengthens the comparisons. Lockley (Reference Lockley2006, p. 172, fig. 7) noted that ‘the paired organization of tracks suggests a saltating type of progression … accomplished by use of only the hind feet …[which] can be produced by aquatic organisms, such as marine turtles, swimming with synchronous strokes of their flippers.’ It was also noted that ‘swim tracks show much wider digit divarication angles than the walking trackways, as if splayed while swimming.’
The only illustrated Molapopentapodiscus supersaltator trackway (now Saltirecarpipes tinleyi; Fig. 11g, after Van Dijk, Reference Van Dijk1978, fig. 4) was twice re-illustrated in different idealized or synthesized form (compare Van Dijk, Reference Van Dijk2001, fig. 2, and Fig. 11g herein, with Thulborn, Reference Thulborn1990, fig. 9.4 h). In both cases, a mammalian trackmaker was not inferred (contra the inferences of Ellenberger, Reference Ellenberger and Haughton1970, Reference Ellenberger1975). The type of Molapopentapodiscus supersaltator (Van Dijk, Reference Van Dijk1978, fig. 4) barely meets the guidelines of Peabody (Reference Peabody1955) and Sarjeant (Reference Sarjeant1989) for a diagnostic trackway configuration. By contrast, photographs and replicas of Malutitetrapodiscus saltator (Fig. 9) allow for an adequate description, different in digit trace curvature and hypicies configurations from Molapopentapodiscus supersaltator. One of the two type (topotype) Molapopentapodiscus supersaltator slabs displays ‘quite deep skid marks’ (Van Dijk, Reference Van Dijk1978, p. 114, fig. 3). Such features raise quality of preservation questions (Belvedere & Farlow, Reference Belvedere, Farlow, Falkingham, Marty and Richter2016). The tracks occur in ‘“playa lake” sediments where “the action of thin films of water by runzel marks… [and] …etch marks and drainage rills” occur, and where “impressions were transmitted to a deeper layer” than the surface on which the footprints were made.’ These may ‘be clearer than the actual footprints, since wind may wrinkle the footprinted surface, producing runzel marks’ (Van Dijk, Reference Van Dijk1978, p. 113).
The interpretation presented herein of Gwyneddichnium, as well as Saltirecarpipes tinleyi and/or Molapopentapodiscus supersaltator, as likely representing ‘swim tracks’ is at least consistent with the inferred ‘“wet palaeoenvironment of small ponds” … a “wet desert” facies … [with] … fine, largely laminated sediments’ (Van Dijk & Eriksson, Reference Van Dijk and Eriksson2021, pp. 1–5; see Section 4 for comparison herein and in Bordy et al. Reference Bordy, Rampersadh, Abrahams, Lockley and Head2020 b). The presence of Batrachopus, widely attributable to a crocodylomorph trackmaker, is also important in assessing palaeoecology. Ellenberger reported and illustrated Batrachopus-like, crocodylomorph tracks in the Lower Jurassic of Lesotho (Ellenberger, Reference Ellenberger and Haughton1970, Reference Ellenberger1974). The name Malutitetrapodiscus saltator (Ellenberger, Reference Ellenberger, Ellenberger and Ginsburg1970; Reference Ellenberger1974, fig. 140; Fig. 9 herein) implies a hopping gait. There is no such evidence for the Batrachopus trackmaker reported here (Fig. 10) or elsewhere (Olsen & Padian, Reference Olsen and Padian1986; Lockley et al. Reference Lockley, Cart, Foster and Lucas2018 and references therein). Van Dijk (Reference Van Dijk2001) in fact dismissed the suggestion of Olsen and Galton (Reference Olsen and Galton1984) that Molapopentapodiscus supersaltator (and other hopper tracks from zone B/4) could be placed in synonymy with Batrachopus, especially after Thulborn (Reference Thulborn1990) had accepted M. supersaltator as a hopping trackmaker.
6.b. Palaeodiversity and the last Karoo vertebrates
The sedimentary rock record, from incipient soils to fossiliferous clastic interbeds, in the Karoo continental flood basalt succession provides a better understanding of how the early phases of the Karoo–Ferrar igneous events impacted the local ecosystems across the Pliensbachian–Toarcian boundary. Biodiversity represented by various fossil plants, microbes, invertebrates as well as herbivorous and carnivorous tetrapods (Figs 8–12) shows that the Karoo region of southern Gondwana had a complex food web. These aqueous, semi-aqueous and terrestrial organisms coexisted in an environment characterized by dynamic switching between sedimentary and volcanic processes. The variable morphology of the interbed footprints attests to a moderate tetrapod diversity in southern Gondwana during the Pliensbachian–Toarcian, as the Drakensberg tracks show affinity to dinosaurs, crocodylomorphs and synapsids, of which at least one is attributed to hopping mammals (Figs 8–12).
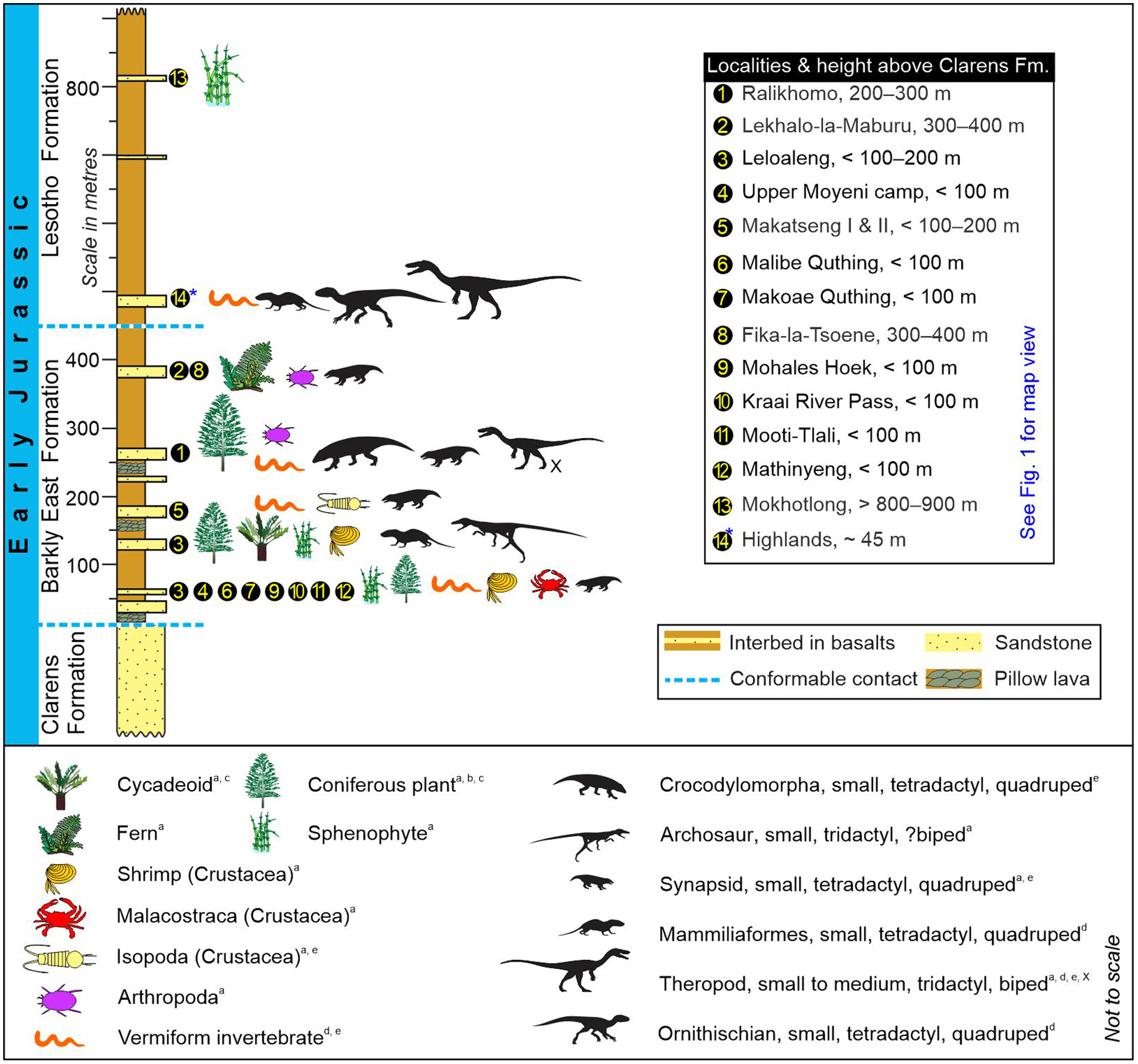
Fig. 12. Stratigraphy of the palaeo-biota in the Lower Jurassic Drakensberg Group (Karoo Supergroup) as interpreted from the body and trace fossil assemblages of the fossiliferous interbeds in South Africa and Lesotho. Because the Highlands interbeds (#14) in the north are within the Lesotho Formation, they are considered younger than Lekhalo-la-Maburu interbeds (#2) in the south, even though these sites are found ∼45 to ∼400 m above the top of the Clarens Formation, respectively. Taxonomic identifications: (a) Ellenberger (Reference Ellenberger and Haughton1970); (b) Plumstead (Reference Plumstead1969); (c) Anderson & Anderson (Reference Anderson and Anderson1985); (d) Bordy et al. (Reference Bordy, Rampersadh, Abrahams, Lockley and Head2020 b); (e) this study. X – the tridactyl trackmaker at Ralikhomo may have been an ornithischian. Locations are shown in Figure 1 and Supplementary Material Table 1. The tetrapod silhouettes are adapted from various sources listed in Bordy et al. (Reference Bordy, Abrahams, Sharman, Viglietti, Benson, McPhee, Barrett, Sciscio, Condon, Mundil and Rademan2020 a).
Fossiliferous interbeds are particularly well recognized in the lower part of the Karoo lava pile, in the Barkly East Formation that is limited to the southern outcrop areas (Figs 1, 12 – e.g. Stockley, Reference Stockley1940, p. 458; Marsh, Reference Marsh1984; Bordy et al. Reference Bordy, Rampersadh, Abrahams, Lockley and Head2020 b). Unlike in the rest of the Karoo–Ferrar LIP (e.g. Babcock et al. Reference Babcock, Leslie, Elliot, Stigall, Ford and Briggs2006; Gierlowski-Kordesch et al. Reference Gierlowski-Kordesch, Weismiller, Stigall, Hembree, Larsen, Egenhoff and Fishman2015), where evidence for tetrapods is lacking, the different vertebrate tracks in the main Karoo Basin, including those of medium-sized carnivorous dinosaurs (Figs 8–12), show that tetrapods were diverse in this part of southern Gondwana.
Considering the general energetic constraints of carnivory (Carbone et al. Reference Carbone, Teacher and Rowcliffe2007), this could suggest that the Karoo was more habitable for diverse life forms, especially when considering its lower palaeo-latitudinal position at ∼40–45° S (e.g. Elliot & Hammer, Reference Elliot, Hammer and Mitra1996; Duncan et al. Reference Duncan, Hooper, Rehacek, Marsh and Duncan1997; Svensen et al. Reference Svensen, Torsvik, Callegaro, Augland, Heimdal, Jerram, Planke and Pereira2018) at the Pliensbachian–Toarcian boundary. Additional to the ∼20° palaeo-latitudinal difference between the Karoo and Ferrar, the shorter lava emplacement interval in the latter (e.g. Burgess et al. Reference Burgess, Bowring, Fleming and Elliot2015) could have also limited the sustainability of an intricate consumer-resource system in the Ferrar region.
The Highlands interbeds in the north (#14 in Figs 1, 12), found <50 m above the top of the Clarens Formation and enclosed in the younger Lesotho Formation (e.g. Marsh, Reference Marsh1984; Bordy et al. Reference Bordy, Rampersadh, Abrahams, Lockley and Head2020 b), likely represent the time by which the lava pile had built up by several hundreds of metres in the southern part of the basin. If so, this northern fossil biota in the Drakensberg Group is younger than those in the south (e.g. Ralikhomo, Lekhalo-la-Maburu), and thus the Highlands footprints are tracks made by the last Karoo vertebrates, including the ultimate Karoo dinosaurs known as yet from this part of southern Gondwana. Based on Lower to Middle Jurassic biostratigraphic considerations, the faunal elements from the Highlands interbeds are comparable to those in Zimbabwe and Argentina (Bordy et al. Reference Bordy, Rampersadh, Abrahams, Lockley and Head2020 b). This can potentially suggest the north- and westward dispersal of the biota, away from the stressful volcanic Karoo landscape, where sprawling basaltic lava flows were increasingly profuse. However, to meaningfully test this idea and investigate the regional to global correlations of the terminal Karoo, the interbed geochronology (and chemostratigraphy) needs to be addressed across southern Africa (i.e. from South Africa to Zimbabwe; Fig. 1).
6.c. Karoo lava-emplacement tempo
Generally, interbeds are taken as indicators of the more intermittent emplacement history of the lowermost Karoo lavas (e.g. du Toit, Reference du Toit1927, Reference du Toit1929; Rawlings et al. Reference Rawlings, Watkeys and Sweeney1999), even though some sedimentary intercalations in flood basalts are considered to represent minimal or even negligible repose periods in extrusive magmatism (e.g. Olsen et al. Reference Olsen, Kent and Et-Touhami2012). Many of the Karoo interbeds contain bedding plane sedimentary features (raindrop impression, desiccation cracks, etc.), as well as animal trails, tracks, etc., as shown in this study (Figs 2–10; Supplementary Material Table 1), and are thus compelling evidence for clastic deposition on the surface of lava flows (versus sediment infiltration into lava tubes and other cavities within basalt flows). Furthermore, assuming roughly similar lava emplacement rates throughout the Karoo volcanism, the lowermost Drakensberg Group in the south, where the Karoo lava pile contains more numerous, often fossiliferous interbeds (see above and Figs 1, 12), may represent a longer overall accumulation time, punctuated by time gaps between consecutive lava flows that were long enough for biotic colonization to occur. Likely, this assumption led to the earlier suggestion (Ellenberger et al. Reference Ellenberger, Ellenberger, Fabre, Ginsburg and Mendrez1964 a, p. 327) that a ‘very premature outpouring’ of the Karoo lavas occurred at least in the southern outcrop region.
The spatiotemporally different lava emplacement model in the south versus north is in line with more recent work showing that the older Barkly East Formation is confined to the southern outcrop areas (Fig. 1; e.g. Marsh, Reference Marsh1984). Here, the first pulse(s) of Karoo volcanism predated, and was likely also more protracted than, the main phase (i.e. Lesotho Formation) that occurred in only <0.5 Ma (e.g. Moulin et al. Reference Moulin, Fluteau, Courtillot, Marsh, Delpech, Quidelleur and Gérard2017). If the spatiotemporal abundance of the fossiliferous interbeds in the Barkly East Formation (Figs 1, 12) is not a sampling artefact (i.e. as, undeniably, the Lesotho Formation is physically more remote), the biostratigraphic pattern in the Drakensberg Group can be considered as an additional proxy for the faster tempo of lava outpourings in the Lesotho Formation.
However, the Karoo interbeds, and, in general, interbeds in any other large igneous province, should be used with caution for gauging the magnitude or frequency of the volcanic dormancy periods, in the light of the spatial heterogeneity of the volcanic processes (e.g. McClintock et al. Reference McClintock, Marsh and White2008a; Bryan et al. Reference Bryan, Peate, Peate, Self, Jerram, Mawby, Marsh and Miller2010; Jay et al. Reference Jay, Marsh, Fluteau and Courtillot2018 a, b; Famelli et al. Reference Famelli, Lima and Carmo2021) and the vastness of regions covered by continental lava flows. Replacing the simplistic, layer-cake view of their formation with a more comprehensive and sophisticated genetic model is long overdue. A new model ought to reflect the protracted and often synchronous processes of continental sedimentation and volcanism that were spatiotemporally highly dynamic across vast lava-flow fields.
For southern Gondwana, we also postulate that this heterogeneous, mosaic habitat dynamically shifted towards central Africa and South America from the late Early to early Middle Jurassic (see above and Bordy et al. Reference Bordy, Rampersadh, Abrahams, Lockley and Head2020 b). The habitat shifts possibly facilitated the migration of the ultimate Karoo biota north- and westwards, away from the main Karoo land of fire. The scarcity of the interbeds and their very limited fossil content in the Lesotho Formation (Fig. 1) may be used as evidence for the incessant emplacement of the upper Karoo lava flows (and by extension, to support the proposed habitat shift). However, a progressively diminishing clastic sediment input, due to the growing carapace of continental flood basalts over the landscape, should also be considered when accounting for the lack of interbeds in the upper Karoo lava pile. Be that as it may, because sediments are the most straightforward archives of ancient life, the habitability and palaeoclimate during this truly terminal Karoo time remain unquantified as yet. This, however, can be improved upon with high-precision geochronology combined with chemo- and volcano-stratigraphic studies that target lava-flow surfaces, especially for macroscopically unfossiliferous and indistinct weathering products (e.g. incipient palaeosols (Lock et al. Reference Lock, Paverd and Broderick1974; Moulin et al. Reference Moulin, Fluteau, Courtillot, Marsh, Delpech, Quidelleur, Gérard and Jay2011; Jay et al. Reference Jay, Marsh, Fluteau and Courtillot2018), which may act as potential climatic proxies (e.g. Sayyed, Reference Sayyed2014; Duraiswami et al. Reference Duraiswami, Sheth, Gadpallu, Youbi and Chellai2020) in the Pliensbachian–Toarcian.
7. Conclusions
Assessing the ultimate Karoo fossil biota and improving its geological (i.e. sedimentological, volcano-stratigraphical) framework allowed for the reconstruction of a multifaceted freshwater palaeoecological system that was intermittently engulfed by voluminous lava outpourings, shortly before the initial dismantling of Gondwana. Pieced together from evidence provided by the sedimentary archive and coeval volcanics, this palaeoecological summary reveals that:
-
The ultimate Karoo habitat was undeniably both aquatic and terrestrial, and fully continental (e.g. vertebrate tracks, plants, freshwater invertebrates, raindrop impressions, desiccation cracks and lack of unequivocal dry/aeolian indicators), and that the climate, on a wet–dry spectrum, was warm temperate and seasonally wet rather than arid at the onset of Karoo–Ferrar magmatism.
-
The Karoo region of southern Gondwana had a moderate palaeo-biodiversity that formed a complex food web of aqueous, semi-aqueous and terrestrial organisms, and included tetrapods too (e.g. carnivorous and herbivorous dinosaurs, mammals, crocodylomorphs), unlike in the Ferrar region of the Karoo–Ferrar LIP.
-
For a robust assessment of the association between the multiphase magmatic events in the Karoo ecosystem and the Pliensbachian–Toarcian global environmental perturbations, the geochronology and chemostratigraphy of the interbeds need to be investigated, which in turn would further refine the age of the ultimate Karoo fossil biota and improve regional and global correlations.
Supplementary material
To view supplementary material for this article, please visit https://doi.org/10.1017/S0016756822001169
Acknowledgements
The work was supported from research grants obtained by EMB (as PI) from GENUS (DST-NRF Centre of Excellence in Palaeosciences; grant numbers GENUS CoE Palaeo 2019, 2020, 2021). Postgraduate bursaries were received by AR, RM and HH from the National Research Foundation (NRF) of South Africa and GENUS (DSI-NRF CoE Palaeo: 2019–2021). MGL’s fieldwork in southern Africa was partially supported by the University of Cape Town (Visiting Scholars’ Fund).
We acknowledge curators Ms Suzanne Jiquel at the Université de Montpellier (France), as well as Ntate Stephen Gill and Mme Keletso Selialia Lesego at the Morija Museum and Archives (Lesotho), for granting us repeated access to the Ellenberger Collections in their care. We are thankful to Dr Fabien Knoll and Dr Raquel López-Antoñanzas not only for many insightful discussions on the Early Jurassic palaeontology of southern Africa but also for accommodating us and being wonderful hosts during our Montpellier study visit. We also gratefully acknowledge Ms T’Nielle Haupt for field assistance with the sedimentology of the Moyeni interbeds; Prof Alfred Uchman for isopod trail identification; Dr Rose Prevec for advice on plant fossils; Mme Matsosane Molibeli for her support of our work and granting our research permits; and Mme Maposholi Mokhethi for repeatedly liaising with Basotho rural authorities. Insightful comments by Tony J Martin (Emory University), Anonymous and Bas Van de Schootbrugge helped improve the overall quality of this study. Opinions expressed and conclusions reached are those of the authors and are not necessarily to be attributed to GENUS (DSI-NRF CoE Palaeo) or NRF or anybody else.
Financial support
The authors receive no financial benefit from this research.
Conflict of interest
None.
Data archiving statement
The data that support the findings of this study are openly available under DOI 10.6084/m9.figshare.19636593 in Figshare. This archived, online-only Supplementary Material includes:
-
a) Supplementary Material Figure A, an illustration of the measured ichnological morphometric parameters of the Batrachopus trackway at Ralikhomo (cf. Fig. 10).
-
b) Supplementary Material Figure B (I–VII), several illustrations of the vertebrate tracks in the sandstone interbeds of the Lower Jurassic Drakensberg Group in Lesotho identified by Ellenberger (Reference Ellenberger and Haughton1970) and in his unpublished data.
-
c) Supplementary Material Text 1, a translation of excerpts from Ellenberger (Reference Ellenberger1975).
-
d) Supplementary Material Table 1 , inventory of the Drakensberg fossil biota.
-
e) Photogrammetric data: photographs used in the photogrammetric models of the Drakensberg fossils in this study (including Supplementary Material), and the cleaned and aligned 3D models as recommended by Falkingham et al. (Reference Falkingham, Bates, Avanzini, Bennett, Bordy, Breithaupt, Castanera, Citton, Díaz-Martínez, Farlow and Fiorillo2018).