Advancing age is accompanied by progressive decline in skeletal muscle mass, leading to the development of sarcopenia( Reference Cruz-Jentoft, Baeyens and Bauer 1 ), which results in significant disability, mortality and health care costs( Reference Cruz-Jentoft, Landi and Topinkova 2 , Reference Janssen, Shepard and Katzmarzyk 3 ). Sarcopenia affects 5–13 % of 60–70 year olds and up to 50 % in those aged more than 80 years with higher prevalence in elderly with disease conditions( Reference Morley, Anker and von Haehling 4 ). The world’s ageing population is expected to reach 1·5 billion in 2050( 5 ) and due to the increased use of health and aged care resources, it is important to determine ways to maintain and improve the health of elderly people before muscle deterioration worsens life outcomes.
Preservation of muscle mass and stimulation of muscle protein synthesis (MPS) through nutritional intervention is a potential therapy for sarcopenia( Reference Paddon-Jones and van Loon 6 , Reference Bauer, Biolo and Cederholm 7 ). MPS is stimulated by protein and amino acid (AA) intake( Reference Paddon-Jones and van Loon 6 , Reference Fujita and Volpi 8 – Reference Nowson and O’Connell 10 ). In older adults, inadequate dietary intake of protein( Reference Bauer, Biolo and Cederholm 7 , Reference Nowson and O’Connell 10 ) and the decreased anabolic response to dietary protein intake are believed to contribute to the loss of muscle mass( Reference Fujita and Volpi 8 , Reference Rolland, Dupuy and Abellan van Kan 11 ). Research has further indicated that essential amino acids (EAA) are key regulators of MPS, with leucine a potent muscle stimulator( Reference Volpi, Kobayashi and Sheffield-Moore 12 , Reference Katsanos, Kobayashi and Sheffield-Moore 13 ) and may suppress muscle protein breakdown( Reference Nowson and O’Connell 10 ). EAA supplements containing leucine have produced improvements in physical function in some studies( Reference Aquilani, Opasich and Gualco 14 – Reference Dal Negro, Aquilani and Bertacco 16 ). Dosage and timing of protein intake may also contribute, with studies( Reference Symons, Sheffield-Moore and Wolfe 17 , Reference Bouillanne, Neveux and Nicolis 18 ) demonstrating that protein consumed in ≥30 g bolus intakes are more effective in increasing post-prandial plasma AA concentration, a signal that stimulates MPS( Reference Bouillanne, Neveux and Nicolis 18 ), and may have the additive effect to overcome the anabolic threshold to MPS( Reference Symons, Sheffield-Moore and Wolfe 17 ).
Recent reviews have shown supplements, including high protein oral nutritional supplement (ONS)( Reference Cawood, Elia and Stratton 19 ), EAA( Reference Malafarina, Uriz-Otano and Iniesta 20 ) or higher protein diets( Reference Cermak, Res and De Groot 21 ) improve muscle strength in older adults, but these reviews included healthy and frail elderly participants and included studies using resistance training (RT)( Reference Cawood, Elia and Stratton 19 – Reference Finger, Goltz and Umpierre 22 ). Although RT is effective in improving muscle strength, it may not be viable for all patients. Nutritional supplementation alone or in association with geriatric rehabilitation may be a practical method for improving muscle mass and function, particularly in vulnerable participants such as those that are hospitalised, malnourished or sarcopenic and who are unwilling or unable to undertake RT.
The aim of this meta-analysis, therefore, is to identify evidence for the effectiveness of protein supplements on muscle mass, strength and physical function in malnourished, frail, sarcopenic, dependent or elderly with acute or chronic conditions, alone or in association with rehabilitation, but not undertaking RT and mirrors usual care provided for the elderly.
Methods
This meta-analysis used the Preferred Reporting Items for Systematic Reviews and Meta-Analyses Guidelines( Reference Moher, Liberati and Tetzlaff 23 ) (online Supplmentary Table S1). A literature search was conducted in April 2014 and updated on 17 June 2016, in the databases Medline, BIOSIS, CINAHL, Cochrane Library, EBM Reviews, Embase, Pre-Medline, ProQuest, PubMed and Scopus. Search terms used were synonyms and combinations of: dietary protein, whey, leucine, AA, nutritional status, nutrition supplement, skeletal muscle, muscle weakness, muscle atrophy, muscle strength, muscle function, sarcopenia, cachexia, weight changes, body composition, malnutrition, frailty, elderly and ageing. The search strategy appears in the online Supplementary Table S2. Citations were downloaded into Endnote X7. Interventions retrieved involved participants with mean age ≥65 years and enrolled volunteers aged 60 years or older, and compared increasing protein intake through protein-rich sources of food; food fortification; and orally consumed liquid, powder or tablets containing protein and energy or EAA, as EAA mixtures or single EAA, for example, leucine to a placebo; supplement of lower protein and energy content; usual care; or no dietary intervention control group. Studies of dietary advice alone were not included. We included studies enrolling hospitalised, community-dwelling or institutionalised subjects with conditions such as frailty, malnutrition, sarcopenia, post-orthopaedic surgery, fracture or acute disease. Studies focusing solely on RT, enteral or parenteral nutrition, or with young, well or healthy adults, or older adults with cancer, cirrhosis, renal disease, stroke or gastrointestinal surgery were excluded. Studies with aerobic or physical rehabilitation activities, without use of RT (defined as 60 % of one-repetition maximum)( Reference Rhea, Alvar and Burkett 24 ), were included only if identical rehabilitation components were provided to both intervention and control groups.
Titles and abstracts were checked for relevance, and coded for retrieval or exclusion. Reference lists of included studies and systematic literature reviews were checked for additional articles. Retrieved articles were coded and assessed by two reviewers using the Cochrane Risk of Bias (ROB) Tool( 25 ). Study data from eligible studies were extracted and tabulated for participant characteristics, numbers and withdrawals; type, dose, frequency of intervention; compliance to intervention; and outcome measures for fat-free mass (FFM), muscle strength, and physical function. In order to ascertain the most effective interventions in well-designed studies, studies with low protein density ONS (10 to <15 % energy from protein) or high ROB were excluded from the analysis( Reference Baldi, Aquilani and Pinna 26 – Reference Wouters-Wesseling, Wouters and Kleijer 39 ).
Data extraction
Results for FFM, muscle strength, and physical function were extracted and analysed separately, either as changes from baseline, or baseline and endpoint scores. If the mean and standard deviation were not provided, the median was used and the sd estimated from the interquartile range. Sensitivity analyses indicated that leaving out one study at a time, did not change overall results appreciably. A correlation of 0·75 was used when variance data of the mean difference were not available( Reference Boreinstein, Hedges and Higgins 40 ). Sensitivity analyses was undertaken and showed that the exact correlation value used made little difference to the overall results. Where data was reported without a quantitative value, that is in graphs or figures, study authors were contacted for further information. More than one outcome measure was commonly provided for the outcome of physical function; however, only one measure was included. The outcome measure chosen for inclusion was based on the characteristics of the instrument, that is tools that measured rather than collected self-reported information, and tools testing more advanced functional outcomes, for example walking speed was selected over Katz Activities of Daily Living (ADL). Tools administered more frequently in studies were used in an effort to reduce variability; for example, tests of walking speed were assessed in seven studies( Reference Stange, Bartram and Liao 33 , Reference Kim and Lee 41 – Reference Rosendahl, Lindelof and Littbrand 46 ) and was the measure selected where data was available. If data for multiple time-points were available, the data closest to intervention end were used, as this was the most common time-point analysed.
Subgroup analysis
Pre-determined subgroup analyses were performed based on ROB score, participant characteristics, protein intervention type, and if participants undertook aerobic or physical rehabilitation activities. Participant characteristics were grouped by trial setting (hospital, long-term care (LTC) in institutions, or community) and nutritional status (nourished or undernourished) as defined by study authors, or with low body weight or BMI <25 kg/m2 as used in a previous review( Reference Milne, Potter and Vivanti 47 ). Interventions were characterised as protein-rich foods; EAA (as EAA mixtures or single types of EAA); ONS with protein density of 10 to <15 % (low), 15 to ≤20 % (moderate) or >20 % (high) composition of total energy; and whey-based ONS. Regression based on study duration was also undertaken.
Post hoc analyses was undertaken to determine potential effects of additional nutrients (intrinsically in protein-rich foods, or added as a supplement, e.g. in ONS) and differential effects in studies using a placebo, with meta-regression based on grams of protein or EAA provided by the intervention to determine the effect of dose.
Statistical analysis
Meta-analysis was performed using Comprehensive Meta-Analysis version 2 (Biostat) using the random effects model for heterogeneous samples, as participants were of various health statuses and heterogeneity was likely to be high. Heterogeneity was analysed using the I 2 statistic( Reference Deeks, Higgins and Altman 48 ), with P values used to identify statistically significant heterogeneity. No significant heterogeneity was found for the FFM analysis; however, the random effects model was used across the three outcome variables for consistency. Effect size was reported as the standardised mean difference (SMD) and the 95 % CI. General rules of thumb for effect size used were 0·2 for ‘small’, 0·5 for ‘moderate’ and 0·8 for ‘large’ effect( Reference Durlak 49 ). Overall significance was assumed at P<0·05. Meta-regression on additional EAA and protein dose provided through interventions and intervention length were undertaken and presented as slope, standard error. Meta-analysis data were presented as forest plots, and publication bias was assessed with funnel plots.
Results
A total of 8702 citations were reviewed, with thirty-nine studies fulfilling the selection criteria (Fig. 1).
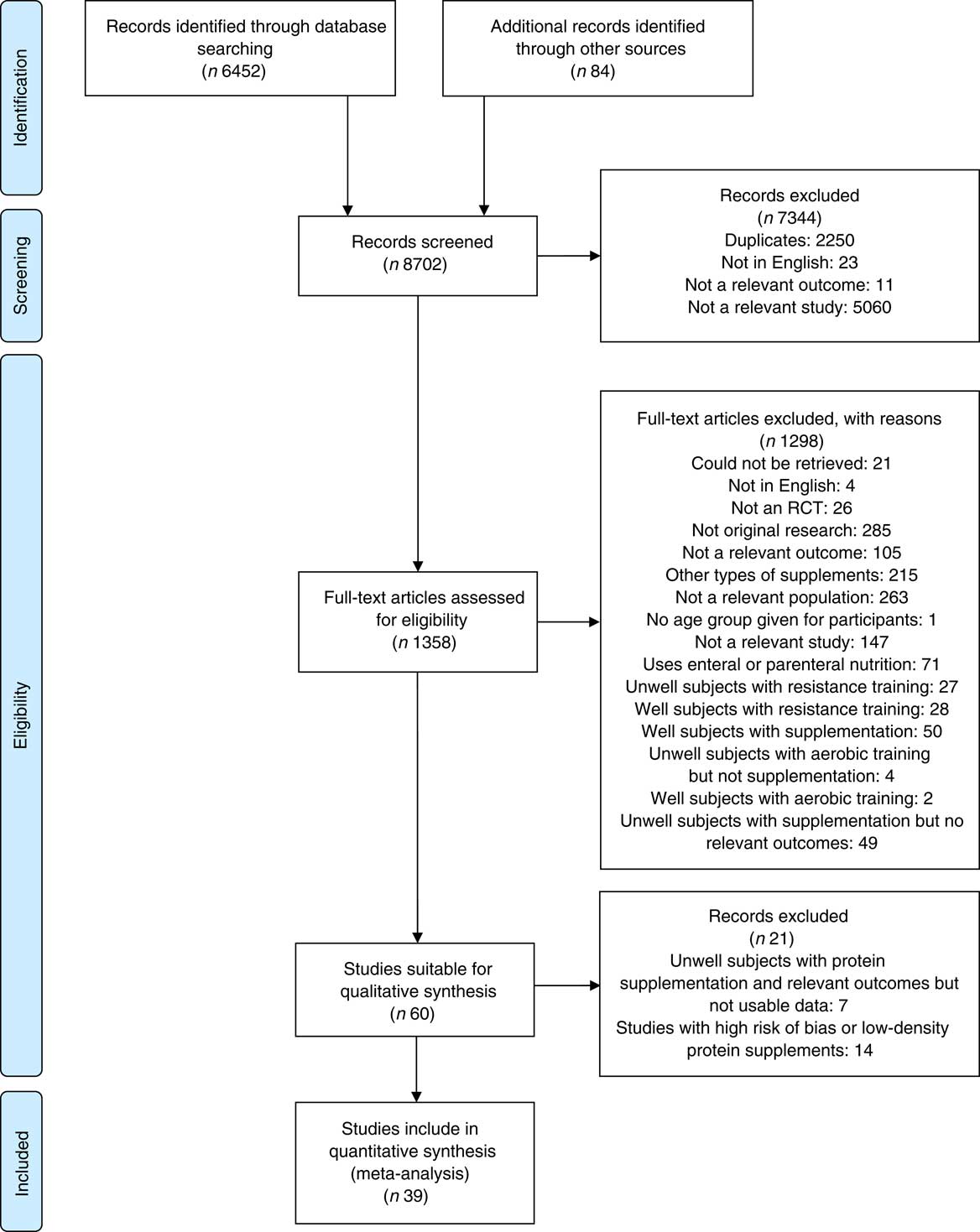
Fig. 1 Preferred Reporting Items for Systematic Reviews and Meta-Analyses flow diagram summarising selection process. RCT, randomised controlled trails.
Publication bias and sensitivity analysis
No obvious evidence of publication bias was found for studies of FFM and physical function, with only minor evidence of publication bias for studies of muscle function, as indicated by the funnel plots (see online Supplementary Fig. S1(a)–(c)). Sensitivity analysis showed small changes in SMD after removal of one study in each outcome analysis, but P values remained significant (all P<0·01), and all thirty-nine studies were included in the analysis. The weights of individual studies ranged from 2·18 to 12·51 for FFM, 2·05 to 5·10 for muscle strength, and 1·02 to 5·48 for physical function.
Characteristics of included studies
A summary of the main characteristics of the thirty-nine studies that met inclusion criteria can be found in Table 1. Two authors who were contacted provided additional information not reported in the original studies( Reference Bouillanne, Curis and Hamon-Vilcot 50 , Reference Payette, Boutier and Coulombe 51 ). The included studies involved 4274 participants, with ages ranging from 60( Reference Zak, Swine and Grodzicki 43 , Reference Dreyer, Strycker and Senesac 52 ) to 103( Reference Smoliner, Norman and Scheufele 53 ). In all, sixteen studies involved community-dwelling participants (n 2350); twelve in hospital (n 1264); and eleven in LTC settings, including residential care and nursing homes (n 660). Most of the studies included undernourished, at risk of malnutrition, sarcopenic or frail participants, with thirteen studies including elderly participants with a mean normal weight:height ratio (BMI) ≥25 kg/m2.
Table 1 Characteristics of included studies

ROB, risk of bias; U, unclear; L, low or lunch; IG, intervention group; CG, control group; NH, nursing home; MCT, medium-chain TAG; LCT, long-chain TAG; CHF, chronic heart failure; TSP, textured soya protein; COPD, chronic obstructive pulmonary disease; SES, socioeconomic status; TKA, total knee arthroplasty; AD, Alzheimer’s disease; F/U, follow up; D, dinner; BF, breakfast; P, protein; OT, occupational therapy; D/C, discharge; EAA, essential amino acids; vit., vitamin; Leu, leucine; ONS, oral nutritional supplement; BCAA, branched chain amino acids; BW, body weight; HP, high protein.
* Intervention (ONS, protein-rich food, etc.) provides additional vitamins and minerals, in addition to protein and kJ.
Thirty-one studies involved supplementation with ONS and protein-rich foods( Reference Kim and Lee 41 , Reference Zak, Swine and Grodzicki 43 , Reference Fiatarone, O’Neill and Ryan 44 , Reference Rosendahl, Lindelof and Littbrand 46 , Reference Bouillanne, Curis and Hamon-Vilcot 50 , Reference Payette, Boutier and Coulombe 51 , Reference Smoliner, Norman and Scheufele 53 – Reference Bonnefoy, Laville and Ecochard 77 ), three of which used whey protein-based ONS( Reference Bauer, Verlaan and Bautmans 56 , Reference Björkman, Finne-Soveri and Tilvis 57 , Reference Sugawara, Takahashi and Kashiwagura 73 ). Eight studies used EAA supplementation, either as EAA mixtures with or without other nutrients( Reference Aquilani, Opasich and Gualco 14 , Reference Dal Negro, Aquilani and Bertacco 16 , Reference Kim, Suzuki and Saito 45 , Reference Dreyer, Strycker and Senesac 52 , Reference Dal Negro, Testa and Aquilani 78 , Reference Abe, Ezaki and Suzuki 79 ) or leucine supplementation only( Reference Leenders, Verdijk and van der Hoeven 80 ). Study duration ranged from 2 weeks( Reference Bonnefoy, Laville and Ecochard 77 ) to 24 months( Reference Dangour, Albala and Allen 62 ), with twenty-six to 1006 participants included. The health characteristics of participants varied, with ten studies focusing on frail participants (n 781); three studies on sarcopenic participants (n 488); and fifteen studies with participants who were malnourished, undernourished or at risk of malnutrition (n 2066). The remaining studies included participants diagnosed with, or recovering from infections or acute illness (n 550); fractures or other surgical operations (n 540); wound healing difficulties (n 38); and catabolic diseases (n 30). Nine studies involved participants with long-term conditions, such as including coronary artery disease, chronic heart failure, type 2 diabetes mellitus, chronic obstructive pulmonary disease, osteoporosis, the metabolic syndrome and dementia (n 517).
Of the thirty-one studies with ONS and protein-rich food interventions, sixteen studies used attention control, no dietary intervention, or standard dietetic care as a comparator; eleven studies used a protein-free comparator; one used a protein-free placebo; and three used alternative ONS with lower energy and protein content. Interventions in these studies could increase energy and protein intake by 355 kJ (85 kcal)( Reference Persson, Hytter-Landahl and Brismar 71 ) to 5040 kJ (1200 kcal)( Reference Miller, Crotty and Whitehead 68 ) , and 4( Reference Persson, Hytter-Landahl and Brismar 71 ) to 50 g( Reference Gariballa and Forster 64 ), respectively. Mean compliance for ONS intake varied from 54 to 100 %.
Of the eight studies using EAA supplementation, five studies used placebos as comparators, and the remaining studies used attention control, no dietary intervention or standard dietetic care. EAA and leucine intake could increase by 3( Reference Abe, Ezaki and Suzuki 79 ) to 40 g/d( Reference Dreyer, Strycker and Senesac 52 ), and 1·2( Reference Abe, Ezaki and Suzuki 79 ) to 7·5 g/d( Reference Leenders, Verdijk and van der Hoeven 80 ), respectively. Only three studies reported compliance to supplements and comparators, of 72 to 100 and 72 %, respectively.
A total of twelve studies involved aerobic or physical rehabilitation activities, which included use of activities as part of standard care( Reference Dreyer, Strycker and Senesac 52 , Reference Cameron, Kurrle and Uy 59 , Reference Myint, Wu and Wong 69 , Reference Volkert, Hubsch and Oster 75 ); participants recruited from rehabilitation units( Reference Bouillanne, Curis and Hamon-Vilcot 50 ); participants discharged from hospital to rehabilitation facilities( Reference McMurdo, Price and Shields 67 , Reference Miller, Crotty and Whitehead 68 , Reference Schurch, Rizzoli and Slosman 72 ); and as novel health interventions( Reference Zak, Swine and Grodzicki 43 , Reference Björkman, Finne-Soveri and Tilvis 57 , Reference Sugawara, Takahashi and Kashiwagura 73 , Reference Yamada, Nishiguchi and Fukutani 76 ).
Risk of bias assessment
Seven studies were at low and thirty-two at unclear ROB. Intention to treat analysis was carried out in eleven studies. Randomisation was inadequately reported in nine studies( Reference Smoliner, Norman and Scheufele 53 , Reference Bakhtiari, Yassin and Hanachi 55 , Reference Björkman, Finne-Soveri and Tilvis 57 , Reference Chapman, Visvanathan and Hammond 60 , Reference Lauque, Arnaud-Battandier and Mansourian 66 , Reference Persson, Hytter-Landahl and Brismar 71 , Reference Volkert, Hubsch and Oster 75 – Reference Bonnefoy, Laville and Ecochard 77 ). Participants were blinded in only nineteen studies( Reference Aquilani, Opasich and Gualco 14 , Reference Dal Negro, Aquilani and Bertacco 16 , Reference Zak, Swine and Grodzicki 43 , Reference Fiatarone, O’Neill and Ryan 44 , Reference Rosendahl, Lindelof and Littbrand 46 , Reference Dreyer, Strycker and Senesac 52 , Reference Bauer, Verlaan and Bautmans 56 , Reference Bonnefoy, Cornu and Normand 58 , Reference Collins, Kershaw and Brockington 61 , Reference Espaulella, Guyer and Diaz-Escriu 63 , Reference Lauque, Arnaud-Battandier and Gillette 65 , Reference McMurdo, Price and Shields 67 , Reference Ng, Feng and Nyunt 70 , Reference Schurch, Rizzoli and Slosman 72 – Reference Tieland, van de Rest and Dirks 74 , Reference Dal Negro, Testa and Aquilani 78 , Reference Leenders, Verdijk and van der Hoeven 80 , Reference Gariballa and Forster 81 ), mainly due to lack of use of a placebo; researchers were blinded in eighteen studies( Reference Aquilani, Opasich and Gualco 14 – Reference Dal Negro, Aquilani and Bertacco 16 , Reference Kim and Lee 41 , Reference Kim, Suzuki and Saito 45 , Reference Rosendahl, Lindelof and Littbrand 46 , Reference Dreyer, Strycker and Senesac 52 , Reference Aleman-Mateo, Macias and Esparza-Romero 54 , Reference Bauer, Verlaan and Bautmans 56 , Reference Collins, Kershaw and Brockington 61 , Reference Espaulella, Guyer and Diaz-Escriu 63 , Reference Myint, Wu and Wong 69 , Reference Schurch, Rizzoli and Slosman 72 – Reference Tieland, van de Rest and Dirks 74 , Reference Dal Negro, Testa and Aquilani 78 , Reference Leenders, Verdijk and van der Hoeven 80 , Reference Gariballa and Forster 81 ) and outcome assessors in fifteen studies( Reference Kim and Lee 41 , Reference Zak, Swine and Grodzicki 43 , Reference Kim, Suzuki and Saito 45 , Reference Rosendahl, Lindelof and Littbrand 46 , Reference Payette, Boutier and Coulombe 51 , Reference Bauer, Verlaan and Bautmans 56 , Reference Cameron, Kurrle and Uy 59 , Reference Collins, Kershaw and Brockington 61 , Reference Dangour, Albala and Allen 62 , Reference McMurdo, Price and Shields 67 – Reference Ng, Feng and Nyunt 70 , Reference Tieland, van de Rest and Dirks 74 ).
Outcomes
Forest plots from the analysis of FFM, muscle strength and physical function outcomes can be found in Figs 2–4, respectively. Statistics, including heterogeneity for all subgroup analyses are reported in the online Supplementary Table S3 with significant effect size results reported below. Studies were alphabetically coded ‘a’ or ‘b’ for studies with multiple intervention arms( Reference Bakhtiari, Yassin and Hanachi 55 , Reference Abe, Ezaki and Suzuki 79 ) or if outcome reporting was separated by intervention subgroups( Reference Volkert, Hubsch and Oster 75 ).
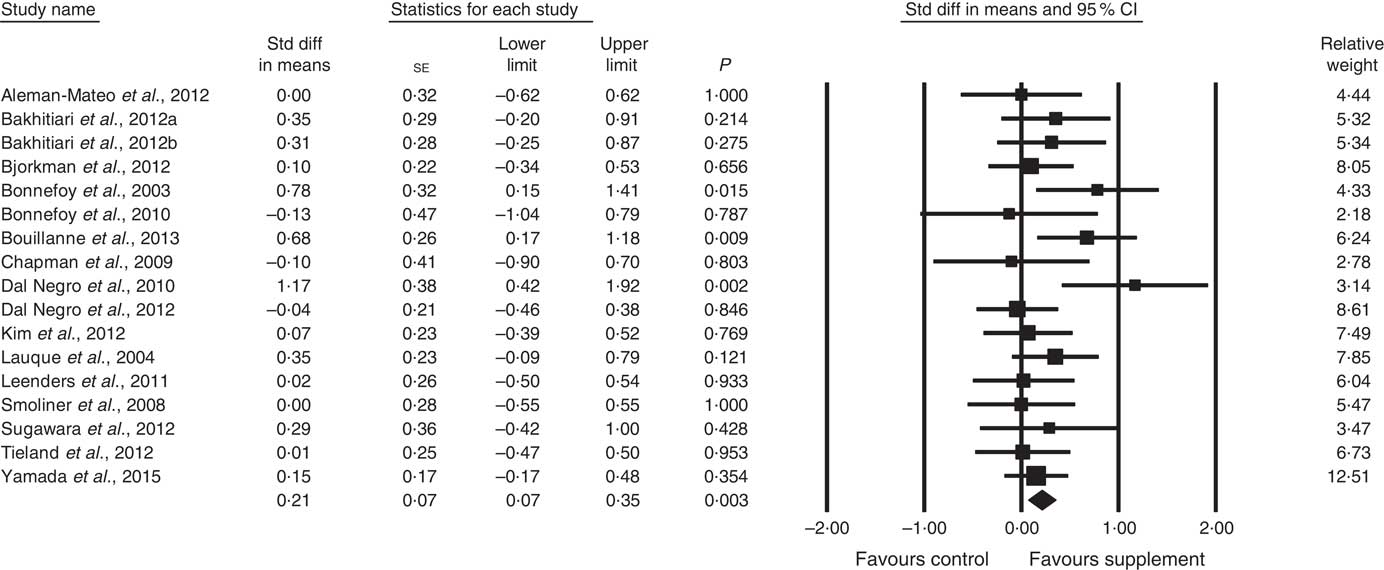
Fig. 2 Forest plot of effect of supplements on fat-free mass. Random effects model. Heterogeneity: I 2 15·1, P=0·28.

Fig. 3 Forest plot of effect of supplements on muscle strength. Random effects model. Heterogeneity: I 2 68·4, P<0·01.

Fig. 4 Forest plot of effect of supplements on physical function. Random effects model. Heterogeneity: I 2 56·8, P<0·01.
Body composition
In all, sixteen studies reported data on FFM (analysed n 970) with methods including dual-energy X-ray absorptiometry and bioelectrical impedance analysis. The effect of ONS and EAA supplementation on FFM was significant with a small effect size (SMD 0·21; 95 % CI 0·07, 0·35, P<0·01, no evidence of heterogeneity, I 2=15·1, P=0·28) (Fig. 2). Only one study had low ROB( Reference Tieland, van de Rest and Dirks 74 ), with no significant effect on FFM seen. However, a small effect was seen in studies with unclear ROB (SMD 0·23; 95 % CI 0·08, 0·37, P<0·01, no evidence of heterogeneity, I 2=17·6, P=0·25). Other subgroup analysis showed improvements in FFM in studies using protein-rich foods (SMD 0·26; 95 % CI 0·02, 0·50, P=0·03, no evidence of heterogeneity, I 2=0·0, P=0·43); studies using supplements with additional vitamins or minerals (SMD 0·24; 95 % CI 0·09, 0·38, P<0·01, no evidence of heterogeneity, I 2=0·0, P=0·56) and with patients not participating in rehabilitation programmes (SMD 0·23; 95 % CI 0·06, 0·41, P=0·01, no evidence of heterogeneity, I 2=29·3, P=0·14) (online Supplementary Table S3). Meta-regression of the protein (slope 0·007, se 0·012, P=0·57) or EAA (slope 0·08, se 0·15, P=0·60) dose provided in interventions showed no evidence of dose–response, although the number of EAA studies was small (n 4). Duration of intervention also showed no significant effect (slope −0·003, se 0·007, P=0·62). Studies (n 4) using a true placebo showed no significant effect.
Muscle strength
A total of twenty-eight studies reported measurements of muscle strength (analysed n 1940), with handgrip strength the most common assessment, followed by leg quadriceps strength. The remaining test measures included were hip and knee, bicep, knee and cycling strength. One study( Reference Bonnefoy, Cornu and Normand 58 ) was excluded from analysis, as its SMD results were more than 2 sd from the mean. The effect of supplementation on muscle strength in the twenty-eight studies (Fig. 3) was found to be statistically significant with a small effect size (SMD 0·27; 95 % CI 0·10, 0·44, P<0·01, significant heterogeneity, I 2=68·4, P<0·01). Five studies had low ROB( Reference Zak, Swine and Grodzicki 43 , Reference Rosendahl, Lindelof and Littbrand 46 , Reference Bauer, Verlaan and Bautmans 56 , Reference Ng, Feng and Nyunt 70 , Reference Tieland, van de Rest and Dirks 74 ) and no significant effect on muscle strength was found; however, a small effect was seen in studies with unclear ROB (SMD 0·31; 95 % CI 0·10, 0·52, P<0·01, significant heterogeneity, I 2=72·0, P<0·01). Other subgroup analysis showed improvements in studies using EAA (SMD 0·82; 95 % CI 0·35, 1·28, P<0·01, significant heterogeneity, I 2=76·9, P<0·01) (Fig. 5), but not other supplements used. Studies using supplements with or without additional vitamins or minerals were both effective, with greater effect size seen with supplements with no added vitamins and minerals (SMD 0·77; 95 % CI 0·22, 1·33, P<0·01, significant heterogeneity, I 2=82·3, P<0·01) than those with added vitamins and minerals (SMD 0·12; 95 % CI 0·00, 0·25, P=0·04, no evidence of heterogeneity, I 2=27·7, P=0·11). Supplements were effective in studies with undernourished participants in community (SMD 0·55; 95 % CI 0·09, 1·02, P=0·02, significant heterogeneity, I 2=84·6, P<0·01) or LTC settings (SMD 0·42; 95 % CI 0·05, 0·79, P=0·03, significant heterogeneity, I 2=55·7, P=0·04); in patients not participating in rehabilitation programs (SMD 0·37; 95 % CI 0·13, 0·61, P<0·01, significant heterogeneity, I 2=75·8, P<0·01); and in studies measuring upper body muscle strength (online Supplementary Table S3). Meta-regression of the protein (slope −0·001, se 0·005, P=0·78) or EAA (slope 0·006, se 0·071, P=0·94) dose provided in interventions showed no evidence of dose–response. Duration of intervention also showed no significant effect (slope 0·010, se 0·006, P=0·11). Studies (n 5) using a true placebo showed a marginally significant effect on muscle strength (SMD 0·67; 95 % CI −0·08, 1·41, P=0·08, significant heterogeneity, I 2=88·4, P<0·01).

Fig. 5 Forest plot of effect of essential amino acids on muscle strength. Random effects model: Heterogeneity: I 2 76·9, P<0·01.
Physical function
In all, thirty-four studies assessed physical function (analysed n 3396) using a variety of methods. Composite physical performance scores included the physical component sections of the Short Form-12 and −36 Health Surveys, the short physical performance battery, Barthel Index or other measures of ADL. Other tests included walking tests, measured for time, distance or speed; and sitting and standing tests. The effect of supplementation on physical function (Fig. 4) was statistically significant with a small effect size (SMD 0·24; 95 % CI 0·13, 0·36, P<0·01, significant heterogeneity, I 2=56·8, P<0·01). Analysis of seven studies with low ROB( Reference Zak, Swine and Grodzicki 43 , Reference Rosendahl, Lindelof and Littbrand 46 , Reference Bauer, Verlaan and Bautmans 56 , Reference Espaulella, Guyer and Diaz-Escriu 63 , Reference Gariballa and Forster 64 , Reference Ng, Feng and Nyunt 70 , Reference Tieland, van de Rest and Dirks 74 ) indicated no significant effect; however, a small effect was seen in studies with unclear ROB (SMD 0·30; 95 % CI 0·15, 0·45, P<0·01, significant heterogeneity, I 2=60·7, P<0·01). Other subgroup analysis showed improvements in studies using EAA (SMD 0·82; 95 % CI 0·52, 1·12, P<0·01, no evidence of heterogeneity, I 2=33·6, P=0·16) (Fig. 6) and moderate protein density ONS (SMD 0·15; 95 % CI 0·00, 0·30, P<0·05, no evidence of heterogeneity, I 2=14·6, P=0·31), with marginally significant improvement in higher v. lower protein density ONS (SMD 0·35; 95 % CI −0·01, 0·72, P=0·06, no evidence of heterogeneity, I 2=11·9, P=0·32), but not other supplement types. Studies using supplements with or without additional vitamins or minerals were both effective, with greater effect seen with supplements with no additional vitamins and minerals (SMD 0·83; 95 % CI 0·49, 1·17, P<0·01, no evidence of heterogeneity, I 2=44·3, P=0·11) compared with supplements with additional vitamins and minerals (SMD 0·13; 95 % CI 0·04, 0·22, P<0·01, no evidence of heterogeneity, I 2=24·0, P=0·12). Supplements were effective in studies undertaken with undernourished participants in community settings (SMD 0·50; 95 % CI 0·17, 0·82, P<0·01, significant heterogeneity, I 2=76·5, P<0·01); in patients not participating in rehabilitation programs (SMD 0·31; 95 % CI 0·15, 0·46, P<0·01, significant heterogeneity, I 2=64·9, P<0·01); and in studies measuring physical function through usual physical activity tests, the short physical performance battery, and walking tests (online Supplementary Table S3). Meta-regression of the protein (slope 0·006; se 0·003, P>0·05) or EAA (slope −0·039, se 0·038, P=0·30) dose provided in interventions showed no evidence of dose–response. Longer intervention length showed marginal effect, with longer study length corresponding to smaller SMD (slope −0·002; se 0·001, P=0·02). Studies (n 5) using a true placebo showed a significant effect on physical function (SMD 0·67; 95 % CI 0·05, 1·30, P=0·04, significant heterogeneity, I 2=81·6, P<0·01).
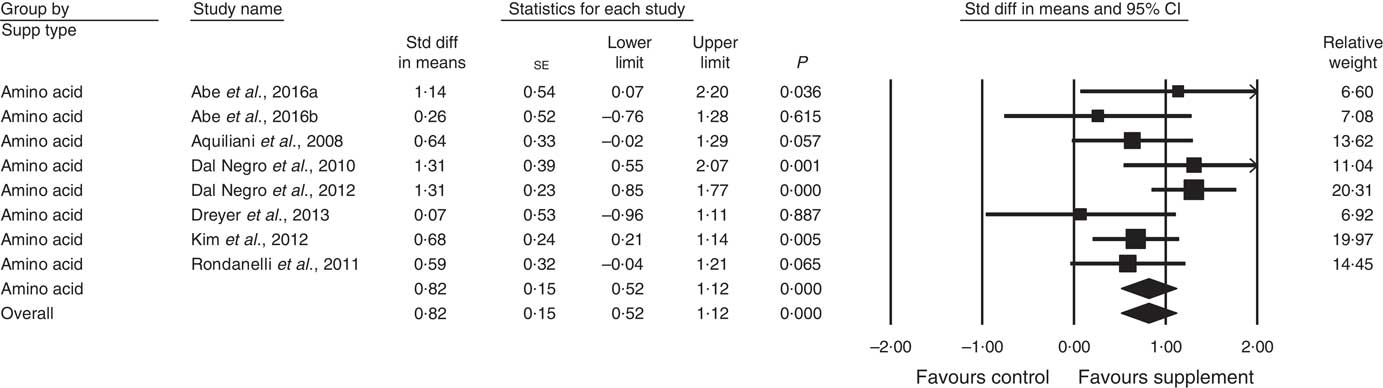
Fig. 6 Forest plot of effect of essential amino acids on physical function. Random effects model: Heterogeneity: I 2 33·6, P=0·16.
Discussion
Overview
This systematic review and meta-analysis found consistent positive effects, however, significant heterogeneity was evident for the outcomes of muscle strength and physical function, and analysis of only low ROB studies showed no effect on any outcome. The study suggests that supplementation with EAA, and to a lesser extent protein through ONS or protein-rich foods, improves parameters of FFM, muscle strength and physical function for malnourished, frail, sarcopenic, dependent elderly, or those with acute or chronic conditions. This effect was seen even without the addition of RT exercise.
Risk of bias and heterogeneity
Studies with high ROB were excluded from the analysis( 82 , Reference Higgins 83 ), however, only seven of the thirty-nine studies had low ROB. Inadequate or poor reporting of allocation concealment, outcome data and blinding were common issues found. In many studies, the lack of a placebo with the control group receiving no intervention, or usual care, increases the likelihood of bias towards the intervention group.
For trials with low ROB, there was no significant effect for any outcome measure of FFM (one study, n 65), muscle strength (five studies, n 537) or physical function (seven studies, n 706). Subgroup analysis of trials with unclear ROB showed supplements were effective, with a small effect size similar to the overall result (SMD 0·23–0·31 v. SMD 0·21–0·27 for all analysed). This difference may be due to the smaller number of low ROB studies reducing statistical power, compared with the unclear ROB subgroup (fifteen to twenty-seven studies; n 905–2690). In addition, no low ROB studies used EAA, which gave the largest effect in this analysis. The following discussion is based on studies of unclear and low ROB combined.
Most effective supplement
In predetermined subgroup analysis, we found protein-rich foods improved FFM (SMD 0·26), moderate protein density ONS benefitted physical function (SMD 0·15), but EAA produced the largest change in muscle strength and physical function (SMD 0·82, both).
EAA are required for stimulation of MPS( Reference Volpi, Kobayashi and Sheffield-Moore 12 ), with approximately 2·0–2·5 g leucine proposed to be required( Reference Bauer, Biolo and Cederholm 7 , Reference Breen and Phillips 84 ). Five studies using EAA-only formulations, found significant improvements after 6–8 g EAA and 2·5 g leucine/d in predominantly undernourished patients( Reference Aquilani, Opasich and Gualco 14 – Reference Dal Negro, Aquilani and Bertacco 16 , Reference Kim, Suzuki and Saito 45 , Reference Dal Negro, Testa and Aquilani 78 ). Leenders et al.( Reference Leenders, Verdijk and van der Hoeven 80 ) supplemented leucine only to normally nourished elderly with type 2 diabetes and 1·0 g protein/kg body weight per d, but found no effect of an additional 7·5 g leucine daily. This may be due to patients normal nutritional status, adequate baseline diets, and the threshold of MPS being reached( Reference Paddon-Jones, Campbell and Jacques 85 ). The result is consistent with recent reviews showing the short term impact of leucine on MPS but not longer term muscle mass or strength effects( Reference Xu, Tan and Zhang 86 , Reference Ham, Caldow and Lynch 87 ).
The large effect of EAA, and smaller effect of ONS and protein-rich foods highlight that the effects of protein consumed as whole food and supplements differ. There are many factors influencing MPS, including the quality and source of the protein ingested. These determine the AA composition, leucine concentration, protein digestibility and subsequent availability of absorbed AA for protein synthesis( Reference Volpi, Kobayashi and Sheffield-Moore 12 , Reference Deutz, Bauer and Barazzoni 88 – Reference Tang and Phillips 93 ). Jonker et al. recently found that a high-leucine EAA mixture was superior to a similar mixture with the AA composition of complete proteins, in improving whole-body net protein gain( Reference Jonker, Deutz and Erbland 94 ), and suggests possible benefits and effectiveness of EAA over complete protein( Reference Paddon-Jones, Sheffield-Moore and Katsanos 92 ).
Protein source influences digestion and absorption rates, and the concept of ‘fast’ and ‘slow’ proteins( Reference Bauer, Biolo and Cederholm 7 , Reference Boirie, Dangin and Gachon 95 ) has emerged. This differentiates proteins based on digestion and absorption kinetics with rapidly digested and absorbed proteins (e.g. whey protein), producing higher post-prandial serum AA concentrations, compared with more slowly digested and absorbed proteins (e.g. casein)( Reference Nowson and O’Connell 10 , Reference Walrand, Gryson and Salles 89 ). Research shows higher doses of soya and casein are required to stimulate MPS compared with whey, in part due to faster absorption rates of whey protein( Reference Boirie, Dangin and Gachon 95 , Reference Witard, Wardle and Macnaughton 96 ).
Food form also contributes to digestibility, with liquid meal replacements( Reference Conley, Apolzan and Leidy 97 ) providing greater AA and leucine levels than the same formulation ingested as solid food. Minced meat is also superior to whole steak as it is more rapidly digested, absorbed and provides greater improvement in whole-body protein balance in older men( Reference Pennings, Groen and van Dijk 98 ).
The interventions using protein-rich foods, which found improvement in FFM, utilised a variety of supplements but many used milk or milk powders, which are high quality proteins containing EAA and leucine with a Digestible Indispensable Amino Acid Score of greater than 1·0( Reference Phillips 99 ). In addition, extra protein was used to fortify usual food, likely at meal times and the dose of protein provided may have reached the threshold for MPS.
The small effect seen with ONS compared with EAA interventions may be due to the quality of the protein and the dose administered by ONS. Most ONS products, although easily absorbed liquids, contain mixtures of whole proteins, for example soya and casein, without additional leucine and may have similar digestibility to ‘slow’ rather than ‘fast’ proteins. Further, the co-ingestion of macronutrients such as fat may slow gastrointestinal transit.
Three studies investigated whey protein supplements, containing 20–40 g whey protein with no overall effect seen. Bauer et al.( Reference Bauer, Verlaan and Bautmans 56 ) reported significant increase in appendicular FFM and improved grip strength in the whey-supplemented group, and Björkman et al.( Reference Björkman, Finne-Soveri and Tilvis 57 ) found significant reduction in ADL assistance needed in the whey protein group, although not the overall ADL score. Only Sugawara et al.( Reference Sugawara, Takahashi and Kashiwagura 73 ) found between-group improvements in strength and 6-min walk distance after supplementation with whey containing ONS. Previous studies show approximately 2 g of leucine, in 20 g of whey protein, may be required for MPS( Reference Breen and Phillips 84 ); however, only Bauer et al.( Reference Bauer, Verlaan and Bautmans 56 ) provided adequate information on amounts of leucine provided. Further studies are required to ascertain the effect of whey protein supplements, and authors should provide better information of supplement ingredients.
The largest effect of any supplement was seen with EAA for muscle strength and physical function, but not for FFM. Only three studies using EAA assessed FFM, whereas eight studies assessed muscle strength and physical function, and lack of power may have affected results. However, changes in strength and function, without change in FFM have been found previously. It is known that the correlation between muscle mass and strength reduces with age( Reference Correa-de-Araujo, Harris-Love and Miljkovic 100 ), and studies have shown short-term RT increases strength but not muscle size( Reference Beyer, Fukuda and Boone 101 ). The quality of muscle, including muscle composition, morphology, contractile quality and neural innervation also impact strength( Reference Beyer, Fukuda and Boone 101 – Reference Clark and Manini 103 ), but muscle mass assessments only determine mass and do not account for these other factors( Reference Correa-de-Araujo, Harris-Love and Miljkovic 100 ). This may contribute to the lack of observed effect of EAA on FFM.
Timing of supplements
With the exception of one study( Reference Bouillanne, Curis and Hamon-Vilcot 50 ), no studies reported quantity of protein consumed per meal or the effect of protein bolus size, and those reporting timing of intake only reported that consumption was during or between meal times. Bouillanne et al.( Reference Bouillanne, Curis and Hamon-Vilcot 50 ) investigated ‘pulse’ (72 % of daily protein at midday meal) compared with ‘spread’ protein (evenly distributed protein intake, with 30 % of daily protein at midday meal), and found improvements in FFM, but not muscle strength or physical function in undernourished hospitalised patients in the pulse group.
Amount of protein required
Dose–response analysis was conducted and showed no evidence of any effect of daily grams of protein or EAA for any outcome. For EAA, dose varied from 3 to 8 g in the seven studies assessing the outcome of muscle strength, and 3 to 20 g for the seven studies assessing physical function. The number of studies was small for FFM (n 3). Patient disease-related factors and nutrient requirements, as well as baseline functional and nutritional status, are likely to be important in determining any effect; however, we were unable to analyse specific doses further, due to the diversity of interventions, patient health conditions and the lack of adequate information provided by studies.
Subgroup analysis of three studies investigated higher v. lower protein density intake with different ONS( Reference Cameron, Kurrle and Uy 59 , Reference Collins, Kershaw and Brockington 61 , Reference McMurdo, Price and Shields 67 ), and trended towards benefits in physical function from higher energy and protein intake. In these studies, there were differences between the intervention and control group intake, of 8·5–27·6 g protein and 945–1680 kJ (220–400 kcal) energy from supplementation. Marginally beneficial effects on physical function (SMD 0·35, P=0·06), but not muscle strength were still found, suggesting higher protein and energy, with additional micronutrient intake, remains beneficial for physical function. The inclusion of these three studies in the full analysis may have reduced the overall result found for muscle strength and physical function, as the additional protein and nutrients in the lower protein group may have reduced the differences seen between groups.
Contribution of other nutrients
Specific vitamin supplementation was uncommon and most studies provided supplements with small additional amounts of a variety of nutrients, supplied by complete ONS or protein-rich foods containing other nutrients, for example addition of milk protein or ricotta cheese also adds energy, Ca and vitamin B12. Complete ONS contain a complete range of micronutrients, along with energy. Only protein-rich foods, with intrinsic nutrient content, provided a small improvement in FFM; however, no effect was found when only true placebo studies were analysed. For muscle strength and physical function, supplements with and without additional nutrients were both effective. In fact, a greater effect was seen in studies using supplements with no added nutrients, likely due to inclusion of EAA studies in this subgroup, as EAAs independently had the largest effect on muscle strength and physical function. Analysis of studies using a true placebo, found a marginal beneficial effect for muscle strength and a large positive effect for physical function (SMD 0·67, P=0·08 and SMD 0·67, P=0·04), respectively. Four of the five true placebo studies for these analyses used EAA and support the conclusion that EAA rather than other nutrients were the effective component.
There is evidence that vitamin D impacts skeletal muscle. A recent meta-analysis( Reference Beaudart, Buckinx and Rabenda 104 ) found a small positive effect of vitamin D supplementation on global muscle strength (SMD 0·17) with greater benefit in elders with 25-hydroxyvitamin D level <30 nmol/l. In our meta-analysis, four studies supplemented with vitamin D additional to that intrinsically provided by the supplement, but were not designed to determine effect of vitamin D( Reference Bauer, Verlaan and Bautmans 56 , Reference Ng, Feng and Nyunt 70 , Reference Persson, Hytter-Landahl and Brismar 71 , Reference Abe, Ezaki and Suzuki 79 ) and we were unable to quantify any effect. Only one study, supplementing with EAA and vitamin D( Reference Abe, Ezaki and Suzuki 79 ) found an improvement in hand grip strength and walk time compared with the no treatment control group.
Energy intake is also important to consider. The provision of additional energy as well as protein was common, particularly if ONS, milk-based drinks or food fortification was used. Protein and EAA are required for cellular functions as well as formation of muscle. However, in times of energy deficit, protein is utilised as an energy source and muscle breakdown occurs( Reference Murphy, Churchward-Venne and Mitchell 105 ). Thus, an energy deficit accelerates muscle protein breakdown and inhibits MPS, which may partly explain why undernourished subjects, most likely in energy and protein deficit, were the most to benefit from supplementation. Additional energy from EAA supplements is unlikely to impact energy intake as the energy provided is only 184–355 kJ (44–85 kcal)/d. In contrast, ONS provided between 623–5040 kJ (149–1200 kcal)/d, but was less effective than EAA in producing an effect on muscle strength and function.
Duration of intervention
Duration of intervention was investigated and a marginal negative effect of longer duration was found for physical function, but no effect was seen for other outcome measures. This suggests intervention time had little or no impact on short-term outcomes, with no blunting of effect due to duration of supplementation. The use of ONS for 6 weeks and 12 months is acceptable to patients and effective in improving both nutritional status and reducing rates of hospital readmissions( Reference Cawood, Elia and Stratton 19 , Reference Stratton, Hebuterne and Elia 106 ). The time period for improvements in muscle function may be weeks, but continued improvement is unlikely once the individual’s threshold of strength and function has been reached. However, additional nutrients may be beneficial to support continued muscle strength and function in the longer term, unless other effective strategies, such as RT are incorporated.
Participants experiencing the greatest benefits
It is important to identify patient groups where interventions are most efficacious. Subgroup analysis of studies in undernourished, but not nourished community-dwelling elderly indicated significant benefits on muscle strength (SMD 0·55) and physical function (SMD 0·50). Muscle strength was also improved in undernourished elderly in LTC (SMD 0·42), indicating that undernourished groups may experience the greatest benefits from supplementation.
Undernourished individuals generally have lower dietary intakes and higher requirements, often due to disease, for example wound/fracture healing, catabolic effects of inflammatory disease or for whole body protein repletion( Reference Bauer, Biolo and Cederholm 7 , Reference Nowson and O’Connell 10 ). In situations of energy deficit, muscle protein breakdown increases( Reference Murphy, Churchward-Venne and Mitchell 105 ) and more protein is needed to compensate for the use of protein as an energy source. Our result is consistent with a Cochrane review finding that undernourished patients benefitted most from supplementation( Reference Milne, Potter and Vivanti 47 ). Nutritional status was defined either by the individual trial authors or by BMI measures, and this is not ideal. Future studies should use current consensus definitions or standard tools to better classify nutritional status, frailty and/or sarcopenia. Few studies reported background dietary intake and this is an important modulator and should be provided in future studies.
Rehabilitation combined with nutrition supplementation
Supplementation with EAA and protein without rehabilitation exercise produced a small effect (SMD 0·23–0·37). We found effect sizes for the outcomes of muscle strength and physical function, but not FFM, were significant in studies without rehabilitation, but not in studies including rehabilitation. Supplements have been shown to augment rehabilitation effects on muscle strength and physical function( Reference Sugawara, Takahashi and Kashiwagura 73 , Reference Creutzberg, Wouters and Mostert 107 ), and the lack of effect may be due to the smaller number of studies within the rehabilitation subgroup (n 3–12; studies without rehabilitation n 13–22).
Concerns related to higher protein intake
The thirty-nine studies included elderly from age 60 to 103 years, and other than mild gastrointestinal upset, no complications were reported. The most common concern related to higher protein intake is kidney function deterioration and this topic has been addressed in recent reviews( Reference Bauer, Biolo and Cederholm 7 , Reference Gaffney-Stomberg, Insogna and Rodriguez 108 ). These suggest 1·0–1·2 g protein/kg body weight per d is suitable for elderly people without a negative impact on kidney function being seen. The International Society of Renal Nutrition and Metabolism( Reference Ikizler, Cano and Franch 109 ) recently published guidelines for chronic kidney disease patients, and recommend 1·0 g protein/kg body weight per d for patients with coexisting disease or injury. Given this, additional protein is unlikely to have negative consequences, particularly in undernourished patients. The use of EAA supplements, effective in smaller doses than whole protein-containing foods or ONS, may be a more suitable yet effective alternative to higher protein diets.
Study weights
Individual study weights may have impacted the overall effect size; however, sensitivity analysis that removed individual studies for each outcome showed only a small difference in SMD and marginal change in significance levels, with all SMDs remaining significant. One study, Dal Negro et al.( Reference Dal Negro, Testa and Aquilani 78 ), with a mid-range weighting of 3·69 for muscle strength, produced the highest individual SMD; when this study was removed during sensitivity analysis, the overall SMD was reduced from 0·27 (P<0·01) to 0·19 (P<0·01). For physical function, removal of both Dal Negro et al.( Reference Dal Negro, Aquilani and Bertacco 16 ) (weighting of 1·7) and Dal Negro et al.( Reference Dal Negro, Testa and Aquilani 78 ) (weighting of 3·11), reduced overall SMD from 0·24 (P<0·01) to 0·17 (P<0·01). Both studies supplemented EAA to sarcopenic patients with chronic obstructive pulmonary disease.
Limitations
As previously discussed, this meta-analysis is limited by the varied study quality with only seven low ROB studies, which limits the strength of the conclusions, and may lead to overestimation of intervention effects. This should be considered in the interpretation of results. Only trials published in English were included and the search strategy used a limit of 65 years and older, which may have eliminated some studies. However, all included papers and reviews found were hand-searched for additional studies. Heterogeneity was significant for analyses of muscle strength and physical function due to wide variation in outcome measurements, participant characteristics and intervention supplements. Thus, a random effects model was used for all statistical analyses, and pre-determined subgroup analyses according to setting, nutritional status, intervention type and duration of intervention were conducted. Additional post-hoc analysis to determine the effect of true placebo studies, additional nutrients, and dose–response analysis were undertaken.
Conclusions
Given the ROB of the studies, no definitive conclusions can be made regarding the effects of specific supplements. This meta-analysis does however, provide preliminary data suggesting that EAA may be the most effective supplements and undernourished subjects are the most likely to benefit. More studies with low ROB, using a placebo control group, and adequate reporting of intervention characteristics and dietary intakes are needed. Appropriate interventions in this highly vulnerable group of participants will assist in the maintenance or improvement of strength and functional status, and is important from both a clinical and public health perspective.
Acknowledgements
The authors would like to thank the University of Sydney Medical Librarians for their assistance with database searching.
There were no funding or grants for the completion of this study.
F. O. L. and B. D. designed the study; H. C., J. K., C. U., P. P. and F. O. L. performed the data extraction; P. P., F. O. L., V. H. and H. C. analysed the data; H. C., F. O. L. and V. H. drafted the manuscript and all authors read and approved the final manuscript.
The authors declare that there are no conflicts of interest.
Supplementary material
For supplementary material/s referred to in this article, please visit https://doi.org/10.1017/S0007114517003816