In the 1980s, British Antarctic scientists (Farman et al. Reference Farman, Gardiner and Shanklin1985) discovered the hole in the ozone layer over Antarctica, and we are now familiar with images of springtime ozone depletion extending beyond the continental margins (Fig. 1a). The largest Antarctic ozone hole occurred in 2006 (Fig. 1b), but in recent years recovery has started to become apparent, with the total column ozone predicted to return to 1980 levels by 2066 (WMO 2022). However, there is still reason to be concerned about the timing and extent of ultraviolet (UV) radiation exposure in Antarctica, as well as how ozone recovery may be jeopardized by climate change-mediated events such as wildfires.
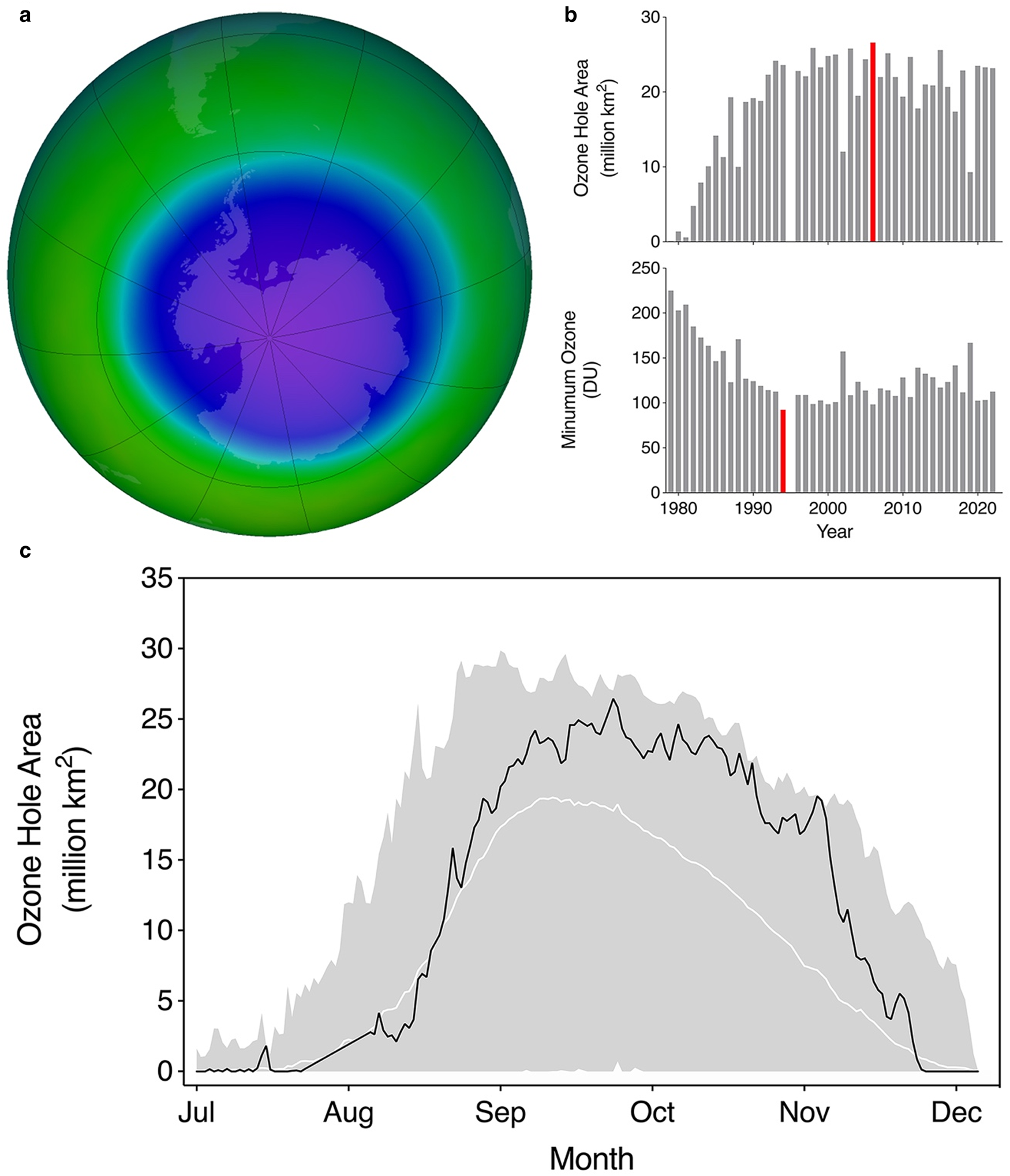
Fig. 1. Antarctic ozone depletion. a. Monthly averaged total ozone over the South Pole for October 2022. b. Graphs showing yearly variation in ozone hole area and minimum ozone depth each year since 1979 (red bars indicating largest area and lowest ozone depth in the top and bottom graphs, respectively). c. The progress of the ozone hole for 2022 (black line) with grey shading indicating the maximum and minimum areas measured since 1979 and the white line indicating the mean values (red numbers indicate the maximum area in 2022). Note: No data were collected in 1995 (images from NASA 2023).
The ozone layer protects the Earth's surface from damaging UV-B radiation. Recent reports demonstrate that over the period of maximum ozone depletion (1990–2020) the maximum spring UV index at Palmer Station (64°S) has increased by 2.5 times compared to the pre-ozone hole era as measured in the early 1970s. Despite the solar angle being much lower in Antarctica, the maximum UV index at Palmer Station in spring can now sometimes exceed that experienced in summer in subtropical regions (San Diego, CA, 32°N; Bernard et al. Reference Bernhard, McKenzie, Lantz and Stierle2022; Environmental Effects Assessment Panel in press).
Antarctic ozone depletion generally peaks between September and October, when most Antarctic terrestrial vegetation and soil biota will be frozen, dormant and hopefully protected under snow cover. Similarly, much marine life will be protected by sea-ice cover, although some seals and birds might be breeding on the ice at this time. Usually by the time summer arrives the ozone layer has recovered (see white lines in Fig. 1c).
However, for the past 3 years ozone depletion has been extensive (Fig. 1b) and long-lasting, extending into early summer (e.g. 2022; see black lines in Fig. 1c). Since 2019, November–December total ozone column depth for latitudes 60–90°S have been the lowest since records began in the 1980s (NASA 2023). From a biologist's perspective, ozone depletion in early December is far more concerning, given that this is closer to the solstice, meaning that all solar radiation is higher, including the UV index. A peak in UV index coincident with snowmelt and the emergence of vegetation as well as during the peak breeding season at the start of the summer is of particular concern, as more biota are likely to be exposed to this higher incident UV-B radiation. For some organisms, such exposure may also occur at a more vulnerable time in their life cycles. The effects of climate change through earlier snowmelt and heatwaves (Robinson et al. Reference Robinson, Klekociuk, King, Pizarro Rojas, Zúñiga and Bergstrom2020, Environmental Effects Assessment Panel in press) is likely to be enhancing the spring and summer UV exposure of Antarctic organisms, as noted in the latest United Nations Environment Program Environmental Effects Assessment Panel report (Barnes et al. Reference Barnes, Robson, Zepp, Bornman, Jansen and Ossola2023; Environmental Effects Assessment Panel in press).
The Montreal Protocol is an extremely successful environmental treaty. As a result of this treaty, the release of ozone-depleting compounds (e.g. chlorofluorocarbons; CFCs) has been controlled, and the ozone layer is predicted to recover by 2066 (WMO 2022). Actions taken due to the Montreal Protocol and its Kigali 2016 amendment have also contributed to reducing greenhouse gas emissions and slowing global temperature increases through the substitution of those CFCs that contributed to greenhouse gas emissions with compounds that do not contribute to climate forcing. It is estimated that this has prevented at least 0.5–1.0°C of warming in the mid-latitudes and prevented more than 1.0°C of warming in the Arctic (WMO 2022; Environmental Effects Assessment Panel in press).
But we cannot afford to be complacent. The extended ozone depletion observed in recent years may have been exacerbated in part by climate change impacts. Two of the recent large ozone holes were influenced by distinct events: the Australian Black Summer bushfires of 2019–2020 and the eruption of the La Soufriere volcano in 2021 (Yook et al. Reference Yook, Thompson and Solomon2022). Research shows that the extensive Australian wildfires injected aerosols into the stratosphere that are capable of depleting ozone (Damany et al. Reference Damany-Pearce, Johnson, Wells, Osbourne, Allan and Belcher2022; Yook et al. Reference Yook, Thompson and Solomon2022) and probably contributed to the larger ozone hole area in 2020 (Fig. 1b; Solomon et al. Reference Solomon, Stone, Yu, Murphy, Kinnison, Ravishankara and Wang2023). Unlike the original causes of ozone depletion, which are largely restricted to the coldest polar regions, wildfire smoke could cause the breakdown of ozone across the globe. Solomon et al. (Reference Solomon, Stone, Yu, Murphy, Kinnison, Ravishankara and Wang2023) suggest that the Australian wildfire aerosols could have been responsible for 3–5% of the total ozone depletion at Southern Hemisphere mid-latitudes in 2020; by comparison, recovery of ozone in this region (due to the Montreal Protocol) is on the order of ~1% per decade. Therefore, these findings increase concerns that wildfires, as well as volcanic eruptions, could delay ozone recovery (Solomon et al. Reference Solomon, Stone, Yu, Murphy, Kinnison, Ravishankara and Wang2023).
We cannot control volcanoes, and it is possible that the massive Hunga Tonga-Hunga Ha'apai volcanic eruption in 2022 will emerge as a driver of the large 2022 ozone hole (https://atmosphere.copernicus.eu/three-peculiar-antarctic-ozone-hole-seasons-row-what-we-know#:~:text=The%20ozone%20depletion%20process%20causing,(PSCs)%20and%20solar%20radiation). But we do know that as Earth's climate warms, increasing droughts and extreme climate events are predicted to result in more frequent and extreme bushfires. At the same time, global warming and especially heatwaves could result in earlier snowmelt and faster sea-ice loss, potentially adding increased UV radiation to the list of stressors that Antarctic organisms are already being exposed to (Robinson et al. Reference Robinson, Klekociuk, King, Pizarro Rojas, Zúñiga and Bergstrom2020, Constable et al. Reference Constable, Harper, Dawson, Holsman, Mustonen, Piepenburg and Rost2022). It is ironic that just as confidence in the recovery of the Antarctic ozone layer was increasing, we face concerns that this recovery could be delayed. We are all aware that what happens in Antarctica affects the globe (Chown et al. Reference Chown, Leihy, Naish, Brooks, Convey and Henley2022). This is just another example of why action on climate change is essential to protect both Antarctica and ourselves.
Acknowledgements
This work was supported by the Australian Research Council SRIEAS Grant SR200100005 Securing Antarctica's Environmental Future and ARC Grant DP200100223. Figure 1 has been redrawn by Hao Yin from data downloaded from the NASA Ozone Watch webpage.