Introduction
Impacts from climate change and other human activities are causing a rapid global decline of coral reefs (Wilkinson, Reference Wilkinson2008; Burke et al., Reference Burke, Reytar, Spalding and Perry2011; IPCC, Reference Barros, Field, Dokken, Mastrandrea, Mach, Bilir, Chatterjee, Ebi, Estrada, Genova, Girma, Kissel, Levy, MacCracken, Mastrandrea and White2014a, Reference Pörtner, Roberts, Tignor, Poloczanska, Mintenbeck, Alegría, Craig, Langsdorf, Löschke, Möller, Okem and Rama2022). Most of the world's tropical reefs have experienced severe bleaching events in the past 30 years, with widespread bleaching occurring in 1998, 2010 and through 2014–2017 (Heron et al., Reference Heron, Maynard, Van Hooidonk and Eaking2016; Sully et al., Reference Sully, Burkepile, Donovan, Hodgson and Van Woesik2019). Warm-water corals are projected to decline further in the coming decades as they become increasingly exposed to thermal anomalies (Van Hooidonk et al., Reference Van Hooidonk, Maynard, Tamelander, Gove, Ahmadia, Raymundo, Williams, Heron and Planes2016; Hughes et al., Reference Hughes, Kerry, Álvarez-Noriega, Álvarez-Romero, Anderson, Baird, Babcock, Beger, Bellwood, Berkelmans, Bridge, Butler, Byrne, Cantin, Comeau, Connolly, Cumming, Dalton, Diaz-Pulido, Eakin, Figueira, Gilmour, Harrison, Heron, Hoey, Hobbs, Hoogenboom, Kennedy, Kuo, Lough, Lowe, Liu, McCulloch, Malcolm, McWilliam, Pandolfi, Pears, Pratchett, Schoepf, Simpson, Skirving, Sommer, Torda, Wachenfeld, Willis and Wilson2017, Reference Hughes, Anderson, Connolly, Heron, Kerry, Lough, Baird, Baum, Berumen, Bridge and Claar2018). Ocean acidification, storminess and the compounding impact of other anthropogenic pressures further raises the risk to corals into the future (Pandolfi et al., Reference Pandolfi, Connolly, Marshall and Cohen2011; Dutra et al., Reference Dutra, Haywood, Singh, Ferreira, Johnson, Veitayaki, Kininmonth, Morris and Piovano2021; IPCC, Reference Pörtner, Roberts, Tignor, Poloczanska, Mintenbeck, Alegría, Craig, Langsdorf, Löschke, Möller, Okem and Rama2022). Stony corals have a disproportionately important role in the marine environment; changes in species composition can dramatically impair the ecological functioning of the reef with negative, knock-on consequences to associated biological communities (Glynn, Reference Glynn1993; Moberg & Folke, Reference Moberg and Folke1999; Baker et al., Reference Baker, Glynn and Riegl2008; Fisher et al., Reference Fisher, O'Leary, Low-Choy, Mengersen, Knowlton, Brainard and Caley2015). In addition to their ecological importance, coral reefs provide a range of vital services to societies and economies, including food provision, coastal protection and tourism (Moberg & Folke, Reference Moberg and Folke1999; IPCC, Reference Barros, Field, Dokken, Mastrandrea, Mach, Bilir, Chatterjee, Ebi, Estrada, Genova, Girma, Kissel, Levy, MacCracken, Mastrandrea and White2014a), which are particularly important in the case of small oceanic islands.
The remote Pitcairn Islands group in the equatorial South Pacific includes the islands of Pitcairn, Oeno, Henderson and Ducie, with an aggregated land area of only 49 km2 (Irving & Dawson, Reference Irving and Dawson2012). These islands are part of an outcrop along the Foundation Seamount chain in the central South Pacific (Figure 1). Pitcairn Island is volcanic and the only inhabited island, with 52 permanent residents as of January 2020 (Pitcairn Miscellany, 2020). The coastline of Pitcairn Island is rocky and steep and exposed to large ocean swells (Avagliano et al., Reference Avagliano, Artzner, Kape and Bocquet2016). Henderson Island is formed from a raised coral reef, and Oeno and Ducie are low coral atolls (Spencer, Reference Spencer1995; Robinson et al., Reference Robinson, New, Popova, Srokosz and Yool2017). The Pitcairn Islands Marine Protected Area (MPA) was designated in 2016 (The Government of the Pitcairn Islands, 2017) and is one of the largest in the world, covering 841,910 km2 (UNEP-WCMC & IUCN, 2021). The Pitcairn Islands MPA encompasses the entire Pitcairn Exclusive Economic Zone (Figure 1) and is a highly protected, no-take area (The Government of the Pitcairn Islands, 2017). Marine Conservation Regulations are in preparation, and in 2017 a Fisheries Management Plan was introduced, to manage the only artisanal fisheries that are allowed within coastal conservation areas, mainly around Pitcairn Island (The Government of the Pitcairn Islands, 2017; Irving et al., Reference Irving, Dawson, Christian and Sheppard2019; Dawson & Irving, Reference Dawson and Irving2020).
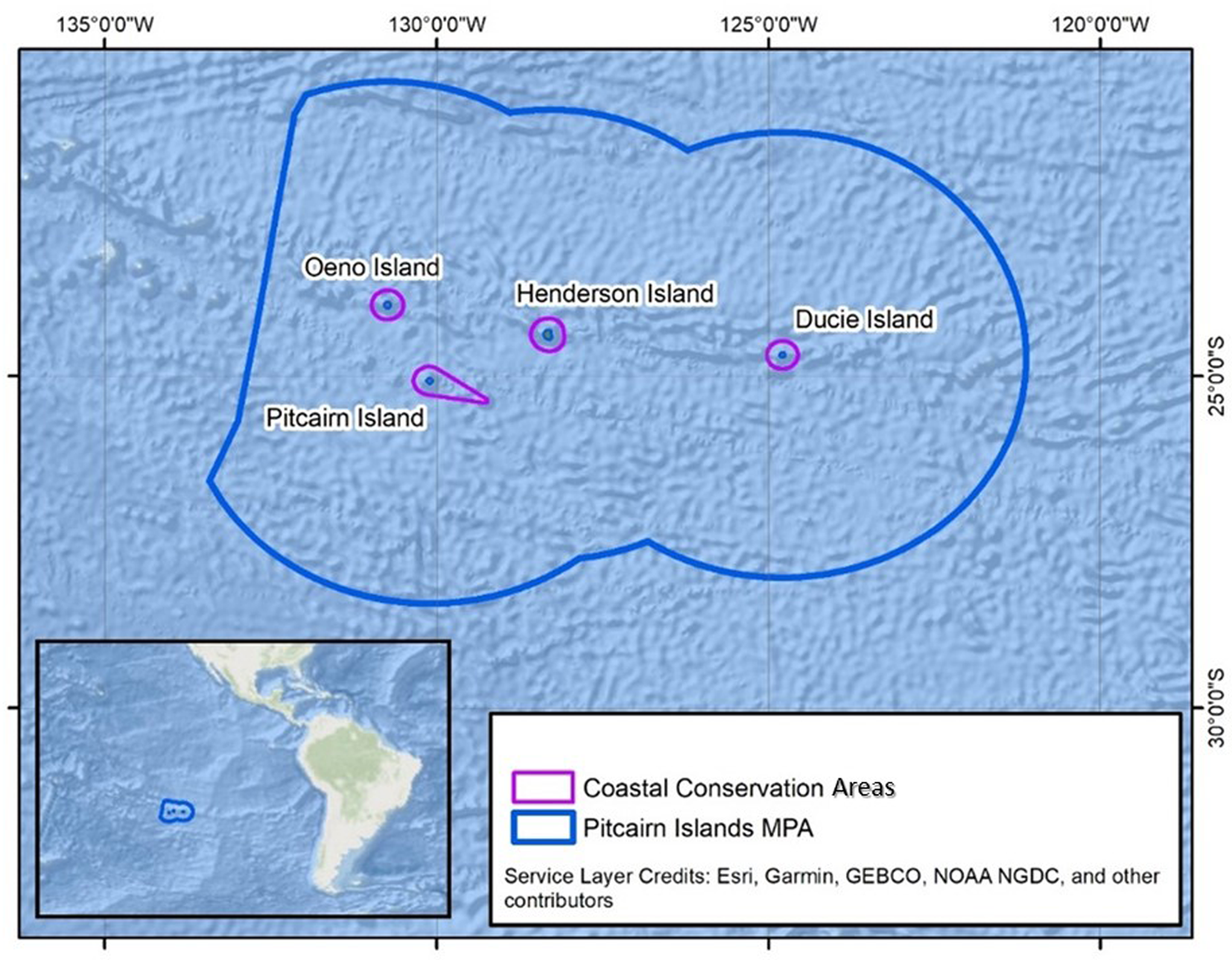
Fig. 1. Map of the Pitcairn Islands designated Marine Protected Area, which occupies the entire Exclusive Economic Zone. The no-take MPA extends over 841,910 km2, with subsistence fishing allowed within coastal conservation areas.
The reefs of the Pitcairn Islands are located on the limits of coral growth at the eastern edge of the Indo-Pacific biogeographic realm, and are distinct from other reef systems due to their isolation. Some endemic species have been identified primarily at Ducie Island, the most remote island in the group, consisting of fish species endemic to the south-eastern tropical Pacific region as well as a few other species only found in these islands (Friedlander et al., Reference Friedlander, Caselle, Ballesteros, Brown, Turchik and Sala2014). Coral reefs are present around all four of the Pitcairn Islands, with Ducie having the highest coral cover, 56%, and Pitcairn the lowest, 5% (Friedlander et al., Reference Friedlander, Caselle, Ballesteros, Brown, Turchik and Sala2014). At Pitcairn Island, macroalgae are abundant (far more so than at the other three islands) and corals are predominantly absent above 10 m depth, possibly due to the combined impact of wave action and sand scouring, but are abundant below 10–30 m and deeper, due to the exceptional water clarity (Irving & Dawson, Reference Irving, Dawson and Sheppard2013; Duffy, Reference Duffy2014; Friedlander et al., Reference Friedlander, Caselle, Ballesteros, Brown, Turchik and Sala2014).
In this study we review the risk of climate change impacts to the coral reefs of the Pitcairn Islands, together with other local human pressures, in order to support the Pitcairn Islands MPA and inform potential management and resilience actions.
Materials and methods
A literature review of climate change and coral reefs of the UK Overseas Territories of the Pitcairn Islands, as well as the British Indian Ocean Territory (BIOT), was undertaken. This review, available as a separate technical report document (see Lincoln et al., Reference Lincoln, Cowburn, Howes, Birchenough, Pinnegar, Dye, Buckley, Engelhard and Townhill2021), covered key drivers of climate change and observed and expected impacts on the coral reefs and other important marine life. A policy summary was also produced, to inform decision making and conservation activities in these territories (see BlueBelt Report Card, 2021). The marine environment around the Pitcairn Islands remains vastly under-studied with few published scientific studies available. The scarcity of relevant literature prevented the use of a systematic review method. Instead, the authors used the information from key published studies of the Pitcairn Islands, and mined further literature from those and from other relevant regional and global studies. Where possible, climate projections were based on either of the main Representative Concentration Pathways (RCP) climate model projections: low (mitigation) emissions scenario (RCP2.6), medium (stabilization) scenarios (RCP4.5/RCP6) and high (baseline) emission scenarios (RCP8.5; Van Vuuren et al., Reference Van Vuuren, Edmonds, Kainuma, Keywan, Thomson, Hibbard, Hurtt, Kram, Krey, Lamarque, Masui, Meinshausen, Nakicenovic, Smith and Rose2011). RCP2.6 and RCP8.5 are extreme scenarios, and it is expected that actual future conditions will fall somewhere in between.
This article condenses the main findings of the original review report by Lincoln et al. (Reference Lincoln, Cowburn, Howes, Birchenough, Pinnegar, Dye, Buckley, Engelhard and Townhill2021), offers a further insight into climate change risks to the coral reefs of the Pitcairn Islands, and discusses options that could help enhance the resilience of these reefs.
Results
Global Coral Reef Monitoring Network observations at Pitcairn Island between 2009–2014, found coral cover ranging from 10–20% (Moritz et al., Reference Moritz, Vii, Long, Tamelander, Thomassin and Planes2018). An expedition to the islands in 2012 found Ducie to have a higher live coral cover (56%) compared with Oeno (28%) and Henderson (24%) Islands, while Pitcairn Island showed the lowest percentage (5%) of live coral cover (Friedlander et al., Reference Friedlander, Caselle, Ballesteros, Brown, Turchik and Sala2014; Irving et al., Reference Irving, Dawson, Christian and Sheppard2019). Reefs appeared to be mostly composed of Porites spp., known to be thermally resistant, as well as more sensitive Pocillopora and Millepora (Irving & Dawson, Reference Irving, Dawson and Sheppard2013; Friedlander et al., Reference Friedlander, Caselle, Ballesteros, Brown, Turchik and Sala2014; Irving et al., Reference Irving, Dawson, Christian and Sheppard2019).
The following sections examine in detail evidence of climate change impacts and the potential future risks to these coral reefs.
Sea temperature
SST conditions
Sea surface temperature (SST) is defined as the subsurface bulk temperature in the top few metres of the ocean measured by ships, buoys and drifters (IPCC, Reference Stocker, Qin, Plattner, Tignor, Allen, Boschung, Nauels, Xia, Bex and Midgley2013). In the tropical Pacific Ocean, SST has increased by 0.3°C between 1950 and 2009, while in the coral reef province of the western Pacific, SST has risen by over 0.4°C in the warmest months over the same period (IPCC, Reference Barros, Field, Dokken, Mastrandrea, Mach, Bilir, Chatterjee, Ebi, Estrada, Genova, Girma, Kissel, Levy, MacCracken, Mastrandrea and White2014a). Each year, trade winds and currents push warm equatorial water eastwards, creating a ‘warm pool’, also known as the equatorial Pacific warm water volume (Capotondi et al., Reference Capotondi, Deser, Phillips, Okumura and Larson2020). This warm pool extends further east towards the Pitcairn Islands during El Niño years, and its extent has increased since the 1980s (Weller et al., Reference Weller, Min, Cai, Zwiers, Kim and Lee2016; Sutton, Reference Sutton2018). As the equatorial Pacific warm pool expands, a larger area of the tropical Pacific will experience higher than average annual temperatures over the rest of the 21st century (Sutton, Reference Sutton2018; Capotondi et al., Reference Capotondi, Deser, Phillips, Okumura and Larson2020). The warm pool is controlled by the El Niño/Southern Oscillation (ENSO), but the high complexity of the ENSO phenomenon, its cyclic variability and increasing amplitude, hinder the ability of climate models to represent the diversity of El Niño events (Capotondi et al., Reference Capotondi, Deser, Phillips, Okumura and Larson2020). At present, it is not possible to consistently predict changes in ENSO events over the coming century (IPCC, Reference Stocker, Qin, Plattner, Tignor, Allen, Boschung, Nauels, Xia, Bex and Midgley2013; Cai et al., Reference Cai, Santoso, Wang, Wu, Collin, Lengaigne, Power and Timmermann2020; Capotondi et al., Reference Capotondi, Deser, Phillips, Okumura and Larson2020; Fang & Yu, Reference Fang and Yu2020).
The Pitcairn Islands are situated along the south-eastern edge of the tropical Pacific, where heat is absorbed near the equator and then cools towards the poles (Sutton, Reference Sutton2018). Three intense marine heatwaves were detected in 1995, 2006 and 2017 respectively, with temperature peaks that were more noticeable at Oeno, Pitcairn and Henderson Islands (Figure 2). However, the natural variability of SST of the Pacific Ocean, which is driven by the Pacific Decadal Oscillation and ENSO system and also influenced by the Pacific warm pool, makes it difficult to attribute SST increases to climate change (IPCC, Reference Barros, Field, Dokken, Mastrandrea, Mach, Bilir, Chatterjee, Ebi, Estrada, Genova, Girma, Kissel, Levy, MacCracken, Mastrandrea and White2014a). Nevertheless, recent reports indicate that ocean conditions in the wider South Pacific region are changing faster and more acutely than initially predicted due to climate change (IPCC, Reference Pörtner, Masson-Delmotte, Zhai, Tignor, Poloczanska, Mintenbeck, Nicolai, Okem, Petzold, Rama and Weyer2019a; Grose et al., Reference Grose, Narsey, Delage, Dowdy, Bador, Boschat, Chung, Kajtar, Rauniyar, Freund, Lyu, Rashid, Zhang, Wales, Trenham, Holbrook, Cowan, Alexander, Arblaster and Power2020 – specifically for the region of Australia; IPCC, Reference Masson-Delmotte, Zhai, Pirani, Connors, Péan, Berger, Caud, Chen, Goldfarb, Gomis, Huang, Leitzell, Lonnoy, Matthews, Maycock, Waterfield, Yelekçi and Zhou2021). Bearing in mind these uncertainties, under medium emissions scenarios RCP4.5 or RCP6 (Van Vuuren et al., Reference Van Vuuren, Edmonds, Kainuma, Keywan, Thomson, Hibbard, Hurtt, Kram, Krey, Lamarque, Masui, Meinshausen, Nakicenovic, Smith and Rose2011), SST around the Pitcairn Islands is projected to rise by ~0.5°C by the middle of this century and by 0.9°C by the end of this century (NOAA Climate Change Portal, 2020). Under a high emissions scenario RCP8.5 (Van Vuuren et al., Reference Van Vuuren, Edmonds, Kainuma, Keywan, Thomson, Hibbard, Hurtt, Kram, Krey, Lamarque, Masui, Meinshausen, Nakicenovic, Smith and Rose2011) and by the end of this century, average SST is expected to warm by 2–2.5°C (Sellar et al., Reference Sellar, Jones, Mulcahy, Tang, Yool, Wiltshire, O'Connor, Stringer, Hill, Palmieri, Woodward, de Mora, Kuhlbrodt, Rumbold, Kelley, Ellis, Johnson, Walton, Abraham, Andrews, Andrews, Archibald, Berthou, Burke, Blockley, Carslaw, Dalvi, Edwards, Folberth, Gedney, Griffiths, Harper, Hendry, Hewiit, Johnson, Jones, Jones, Keeble, Liddicoat, Morgenstern, Parker, Predoi, Robertson, Siahaan, Smith, Swaminathan, Woodhouse, Zeng and Zerroukat2019).

Fig. 2 Plots of thermal stress in the Pitcairn Islands showing: Time series of annual total Degree Heating Weeks (DHWs) from the four main islands (top right); time series of mean annual sea surface temperature (SST) (top left); and maps of DHWs during strong thermal stress years 1995, 2006, and 2017, showing the position of the islands. The dark blue areas denote no data. Data obtained from NOAA Coral Reef Watch (2018).
Degree Heating Weeks (DHW) or Degree Heating Months (DHM) represent the number of weeks or months the corals are exposed to warming above a given threshold multiplied by temperature above the threshold. Based on SST satellite data, peaks of thermal stress expressed as DHWs occurred in 1995, 2006 and 2017 (see Figure 2 and Table 1), with Oeno reaching Bleaching Alert 1 (DHW 4–8) in all those three years (NOAA Coral Reef Watch, 2018). Pitcairn Island was the only island to experience DHWs higher than 8 (Bleaching Alert 2) during 2006, while Ducie Island did not experience thermal stress enough to trigger a bleaching alert (DHW less than 4; see Figure 2 and Table 1; NOAA Coral Reef Watch, 2018).
Table 1. Past thermal stress in each of the Pitcairn Islands showing mean SST at the start and end of a time series spanning 1985–2018 and the difference in mean SST, along with Degree Heating Weeks (DHWs) in potential bleaching years, the cumulative DHW, and the number or Alert Level 1 and 2 thermal stress events. Data obtained from NOAA Coral Reef Watch (2018).

While there is no clear evidence of coral bleaching from seawater warming in the Pitcairn Islands, there are observations of coral damage caused by unusual oceanic cold intrusions in the Ducie atoll, with up to 40% coral damage by cold-water stress found between 10–15 m depth, as explained further in the extreme weather events section (Irving & Dawson, Reference Irving, Dawson and Sheppard2013; Dawson & Zhang, Reference Dawson and Zhang2020). However, without baseline data it is not possible to accurately assess these factors or changes over time. Significant acute thermal stress (DHW 8.9) was experienced at Pitcairn Island in 2006, and Oeno Island (DHW 6) in 2006 and 2017, but otherwise the risk of acute thermal stress in the archipelago appears to remain low (Table 1). Undetected episodes of coral bleaching and mortality over the past 35 years in the Pitcairn Islands, however, remain a possibility.
Future SST projections
The Pitcairn Islands are located near the southern limit for optimal reef growth, with Ducie being the most southerly atoll island in the world. As a result of cooler conditions and slow projected warming, future SST conditions are likely to remain relatively suitable for coral growth, at least until the middle of this century (Couce et al., Reference Couce, Ridgwell and Hendy2013; Jones et al., Reference Jones, Mannion, Farnsworth, Valdes, Kelland and Allison2019). In the Pitcairn Islands and under a high emissions scenario, the onset of severe annual bleaching events is not expected until 2059, which is later than the average global onset threshold of 2043 (Van Hooidonk et al., Reference Van Hooidonk, Maynard, Tamelander, Gove, Ahmadia, Raymundo, Williams, Heron and Planes2016; Sellar et al., Reference Sellar, Jones, Mulcahy, Tang, Yool, Wiltshire, O'Connor, Stringer, Hill, Palmieri, Woodward, de Mora, Kuhlbrodt, Rumbold, Kelley, Ellis, Johnson, Walton, Abraham, Andrews, Andrews, Archibald, Berthou, Burke, Blockley, Carslaw, Dalvi, Edwards, Folberth, Gedney, Griffiths, Harper, Hendry, Hewiit, Johnson, Jones, Jones, Keeble, Liddicoat, Morgenstern, Parker, Predoi, Robertson, Siahaan, Smith, Swaminathan, Woodhouse, Zeng and Zerroukat2019). In addition, most reefs in the Pitcairn Islands are below 10 m depth (Irving & Dawson, Reference Irving, Dawson and Sheppard2013; Duffy, Reference Duffy2014; Friedlander et al., Reference Friedlander, Caselle, Ballesteros, Brown, Turchik and Sala2014), with the exception of the shallow reef flats at Oeno and Henderson and those in the Ducie lagoon, and it is expected that those deeper fore-reefs will be relatively sheltered from thermal stress (Nakamura & Van Woesik, Reference Nakamura and Van Woesik2001; Muir et al., Reference Muir, Marshall, Abdulla and Aguirre2017; Cowburn et al., Reference Cowburn, Moritz, Grimsditch and Solandt2019). Deeper areas of the outer-edge reefs on the Great Barrier Reef have been found to provide refuge from bleaching for Acropora, Pocillopora and Porites spp. (Baird et al., Reference Baird, Madin, Alvarez-Noriega, Fontoura, Kerry, Kuo, Precoda, Torres-Pulliza, Woods, Zawada and Hughes2018). In the eastern Pacific, a depth refugia has also been demonstrated for Millepora spp. (Smith et al., Reference Smith, Glynn, Maté, Toth and Gyory2014). However, the efficacy of these deeper reefs as refugia from warming will be relative, and will depend on the severity of the warming events and on the thermal sensitivity of the corals, as other evidence from the wider Pacific and from the Caribbean shows (Smith et al., Reference Smith, Gyory, Brandt, Miller, Jossart and Nemeth2016; Venegas et al., Reference Venegas, Oliver, Liu, Heron, Clark, Pomeroy, Young, Eakin and Brainard2019). The extreme clarity of the water in the Pitcairn Islands also allows for deeper irradiance, which could to some extent counteract the cooling effect at depth. At Pitcairn Island, corals grow on reefs that reach down to 75 m depth and are dominated by thermally resistant Porites spp. and Pocillopora sp. and the hydrocoral, Millepora spp., which are more likely to withstand warming conditions (Irving & Dawson, Reference Irving, Dawson and Sheppard2013; Friedlander et al., Reference Friedlander, Caselle, Ballesteros, Brown, Turchik and Sala2014). However, in similar communities in the Central and East Pacific, those same coral species were found to die in large numbers during bleaching events (Glynn, Reference Glynn1984; Pratchett et al., Reference Pratchett, McCowan, Maynard and Heron2013). This suggests that the corals of the Pitcairn Islands would still be relatively susceptible to thermal stress (Figure 2; Table 1), particularly in the shallower reefs around Ducie Island dominated by more sensitive corals such as Acropora and Montipora spp. (Loya et al., Reference Loya, Sakai, Yamazato, Nakano, Sambali and van Woesik2001).
Ocean acidification
Surface pH and aragonite saturation conditions
The increasing uptake of CO2 by the world's oceans is manifested as changes to ocean carbon chemistry particularly pH and saturation state of calcium carbonate minerals such as aragonite and calcite, conditions which are critical to the growth and survival of corals and other calcifying organisms.
The largest changes in pH are projected for the surface layer except in the subtropics, where they are expected to occur where carbonate buffering capacity is lower between 200 and 300 m (IPCC, Reference Stocker, Qin, Plattner, Tignor, Allen, Boschung, Nauels, Xia, Bex and Midgley2013). There is no long-term surface water pH data for the equatorial Pacific, making it difficult to ascertain trends (Lenton et al., Reference Lenton, Matear and Mongin2018; GOA-ON, 2020). A range of surface pH 7.91–8.12 has been previously estimated for the equatorial Pacific between 1997–2011 (Sutton et al., Reference Sutton, Feely, Sabine, McPhaden, Takahashi, Chavez, Friederich and Mathis2014), values that are lower, and appear to be declining faster, than other open-ocean regions (Johnson et al., Reference Johnson, Bell and Gupta2015; Lauvset et al., Reference Lauvset, Gruber, Landschützer, Olsen and Tjiputra2015; Wagener et al., Reference Wagener, Metzl, Caffin, Fin, Helias Nunige, Lefevre, Lo Monaco, Rougier and Moutin2018). The pH of the wider tropical Pacific is projected to decrease by 0.15 pH units by 2050 under a high emissions scenario (Johnson et al., Reference Johnson, Bell and Gupta2015). Around the Pitcairn Islands, mean surface water pH is currently estimated near 8.12 and by the end of this century could decrease between 0.08 and 0.27 units, according to low and high emissions scenarios respectively (Figure 3; NOAA Climate Change Portal, 2020).

Fig. 3. Outputs from the CMIP5 ensemble showing mean surface water pH in the eastern Indian Ocean for the period 1956–2005. Image provided by the NOAA/OAR/ESRL Physical Sciences Laboratory, Boulder, CO, USA, from their website at http://psl.noaa.gov/ (NOAA Climate Change Web Portal, 2020). The approximate location of the Pitcairn Islands is indicated by the orange star symbol.
The saturation states of calcium carbonate minerals naturally decrease with depth as total dissolved CO2 increases from a combination of respiration and cold temperatures, and there are indications that surface ocean aragonite saturation state in the Pacific has already declined from 4.5 to 3.8 (Feely et al., Reference Feely, Doney and Cooley2009). Aragonite saturation below 3 is considered extremely marginal for coral growth, and no major reefs currently exist at such conditions (Langdon & Atkinson, Reference Langdon and Atkinson2005; Lenton et al., Reference Lenton, Matear and Mongin2018); while unprotected shells and skeletons will begin to dissolve when surrounding seawater saturation level declines below 1 (Feely et al., Reference Feely, Doney and Cooley2009; Birchenough et al., Reference Birchenough, Williamson and Turley2017). Levels of aragonite saturation in the South Pacific are already thought to be close to the point where it can weaken coral skeletons and slow down coral growth (Dutra et al., Reference Dutra, Haywood, Singh, Piovano, Ferreira, Johnson, Veitayaki, Kininmonth and Morris2018). However, direct field observations linking acidification and ocean chemistry changes to physiological impacts on corals are still rare (Doney et al., Reference Doney, Busch, Cooley and Kroeker2020; Doo et al., Reference Doo, Kealoha, Andersson, Cohen, Hicks, Johnson, Long, Mcelhany, Mollica, Shamberger, Silbiger, Takeshita, Busch and Browman2020). It is possible that the responses of corals to more acidic waters are still within the ranges of natural variability, and such responses would also be influenced by local conditions of water quality and circulation patterns (Field et al., Reference Field, Barros, Mastrandrea, Mach, Abdrabo, Adger, Anokhin, Anisimov, Arent and Barnett2014). There are hardly any in situ studies of ocean acidification effects on corals in Pacific islands (Dutra et al., Reference Dutra, Haywood, Singh, Piovano, Ferreira, Johnson, Veitayaki, Kininmonth and Morris2018), while studies of ocean acidification impacts in other regions have found alterations in the physiology of the corals and the wider ecology of the reef, from changes in the pH of the calcifying fluid of the corals (Kubota et al., Reference Kubota, Yokoyama, Ishikawa, Suzuki and Ishii2017), to loss of skeletal density (Mollica et al., Reference Mollica, Guo, Cohen, Huang, Foster, Donald and Solow2018), to shifts in the species composition of colonies as well as increased algal cover and bioerosion (Fabricius et al., Reference Fabricius, Langdon, Uthicke, Humphrey, Noonan, De'ath, Okazaki, Muehllehner, Glas and Lough2011; Enochs et al., Reference Enochs, Manzello, Donham, Kolodziej, Okano, Johnston, Young, Iguel, Edwards, Fox, Valentino, Johnson, Benavente, Clark, Carlton, Burton, Eynaud and Price2015, Reference Enochs, Manzello, Kolodziej, Noonan, Valentino and Fabricius2016), to loss of ecological complexity and function of the reef (Mumby, Reference Mumby2009; Anthony et al., Reference Anthony, Maynard, Diaz-Pulido, Mumby, Marshall, Cao and Hoegh-Guldberg2011).
Future pH and aragonite saturation projections
ENSO dominates ocean climate in the Pacific, including ocean acidification and ocean carbon chemistry: during non-El Niño periods, ocean acidification has been observed to progress more rapidly due to the influx of upwelling deep, CO2-rich water (McPhaden et al., Reference McPhaden, Zebiak and Glantz2006; Sutton et al., Reference Sutton, Feely, Sabine, McPhaden, Takahashi, Chavez, Friederich and Mathis2014; Fiedler & Lavín, Reference Fiedler and Lavín2017). Projections of aragonite saturation state to the end of this century under high emissions scenarios for the central South Pacific surrounding the Pitcairn Islands suggest that it is likely to decrease to 2.5 (Sellar et al., Reference Sellar, Jones, Mulcahy, Tang, Yool, Wiltshire, O'Connor, Stringer, Hill, Palmieri, Woodward, de Mora, Kuhlbrodt, Rumbold, Kelley, Ellis, Johnson, Walton, Abraham, Andrews, Andrews, Archibald, Berthou, Burke, Blockley, Carslaw, Dalvi, Edwards, Folberth, Gedney, Griffiths, Harper, Hendry, Hewiit, Johnson, Jones, Jones, Keeble, Liddicoat, Morgenstern, Parker, Predoi, Robertson, Siahaan, Smith, Swaminathan, Woodhouse, Zeng and Zerroukat2019, see Figure 4), a sub-optimal level for coral calcification. But the confidence in these projections is only limited, due to the variability of the ENSO and the associated upwelling forcing, and the influence that these processes have on future ocean pH levels in the Pacific Ocean (Christensen et al., Reference Christensen, Kumar, Aldrian, An, Cavalcanti, de Castro, Dong, Goswami, Hall, Kanyanga, Kitoh, Kossin, Lau, Renwick, Stephenson, Xie, Zhou, Stocker, Qin, Plattner, Tignor, Allen, Boschung, Nauels, Xia, Bex and Midgley2013 and references therein). In addition, saturation of calcium carbonate minerals and pH can also vary at local scales depending on the habitat mosaic present (Cyronak et al., Reference Cyronak, Andersson, D'Angelo, Bresnahan, Davidson, Griffin, Kindeberg, Pennise, Takeshita and White2018; Pacella et al., Reference Pacella, Brown, Waldbusser, Labiosa and Hales2018; Bergstrom et al., Reference Bergstrom, Silva, Martins and Horta2019; Liu et al., Reference Liu, Ang, Mayfield and Lin2020) and it can be affected by biological processes like photosynthesis and respiration (Hurd et al., Reference Hurd, Lenton, Tilbrook and Boyd2018; Fabricius et al., Reference Fabricius, Neill, Van Ooijen, Smith and Tilbrook2020).

Fig. 4. Average sea surface aragonite saturation state (Ωar) for the Pitcairn Islands, showing the predicted decline in saturation under RCP2.6 (left) and RCP8.5 (right). Data from the UKESM1 forecast model, from Sellar et al. (Reference Sellar, Jones, Mulcahy, Tang, Yool, Wiltshire, O'Connor, Stringer, Hill, Palmieri, Woodward, de Mora, Kuhlbrodt, Rumbold, Kelley, Ellis, Johnson, Walton, Abraham, Andrews, Andrews, Archibald, Berthou, Burke, Blockley, Carslaw, Dalvi, Edwards, Folberth, Gedney, Griffiths, Harper, Hendry, Hewiit, Johnson, Jones, Jones, Keeble, Liddicoat, Morgenstern, Parker, Predoi, Robertson, Siahaan, Smith, Swaminathan, Woodhouse, Zeng and Zerroukat2019).
The South Pacific Circulation Gyre is expected to slow down future changes in carbonate saturation and pH in the Pitcairn Islands region compared with the wider oceanic basin (Hoegh-Guldberg & Bruno, Reference Hoegh-Guldberg and Bruno2010; Friedlander et al., Reference Friedlander, Caselle, Ballesteros, Brown, Turchik and Sala2014). However, across the tropical Pacific by mid-century under a high emissions scenario, average aragonite saturation is likely to decline to near 3, meaning a shift to sub-optimal conditions (Johnson et al., Reference Johnson, Bell and Gupta2015). This will cause a decline in coral calcification rate of about 10% (Chan & Connolly, Reference Chan and Connolly2013), which is projected to worsen as saturation continues to decline towards the end of the century.
Sea level rise and extreme weather events
Observed sea level rise and storminess trends
Sea level has risen across the Pacific Ocean by varying amounts due to large-scale climate processes, and extreme sea levels have also been detected driven by a combination of long-term sea level rise from climate change and short-term factors such as extreme tides, storm surges and the associated wind-wave setup (Australian Bureau of Meteorology and CSIRO, 2014). There are regional differences in sea level rise across the tropical Pacific (PSMSL, 2020), with the highest rates, ~12 mm year−1, detected in the western South Pacific between 1993–2009 (Meyssignac et al., Reference Meyssignac, Becker, Llovel and Cazenave2012; Nurse et al., Reference Nurse, McLean, Agard, Briguglio, Barros, Field, Dokken, Mastrandrea, Mach, Bilir, Chatterjee, Ebi, Estrada, Genova, Girma, Kissel, Levy, MacCracken, Mastrandrea and White2014). These high sea level rise rates are thought to reflect modulations by natural phenomena such as ENSO, with lower/higher-than-average variability of sea levels during El Niño/La Niña events of the order of ±20 to 30 cm in the western tropical Pacific (Cazenave & Remy, Reference Cazenave and Remy2011; Becker et al., Reference Becker, Meyssignac, Letetrel, Llovel, Cazenave and Delcroix2012). There are no tide gauge records from the Pitcairn Islands (Woodworth & Hibbert, Reference Woodworth and Hibbert2015) but indirect estimations suggest that sea level has already risen almost 4 times the global average. It is thought that the low-lying atolls of Ducie and Oeno have persisted, and even increased in surface area and elevation by way of seasonal erosion and accretion processes following extreme events and changes in sediment supply (McLean & Kench, Reference McLean and Kench2015). This has also been confirmed for other small atoll islands in the South Pacific, where high sea level rise rates have, as yet, not caused loss of dry land area overall (Kench et al., Reference Kench, Thompson, Ford, Ogawa and McLean2015).
Storms can inflict substantial damage to shallow coral reefs. In the Pacific Ocean, the intensity of tropical cyclones appears to have increased since 1990 (Diamond et al., Reference Diamond, Lorrey and Renwick2013), although it is not clear whether there is a basin-wide trend in the number of very intense tropical cyclones across the Pacific basin (Australian Bureau of Meteorology and CSIRO, 2014; Holland & Bruyère, Reference Holland and Bruyère2014; CSIRO et al., 2015; Hoarau et al., Reference Hoarau, Chalonge, Pirard and Peyrusaubes2018; Lafale et al., Reference Lafale, Diamond and Anderson2018; Dutheil et al., Reference Dutheil, Lengaigne, Bador, Vialard, Lefèvre, Jourdain, Jullien, Peltier, Sultan and Menkès2020), and the attribution of this trend to climate change also remains in question (Collins et al., Reference Collins, Sutherland, Bouwer, Cheong, Frölicher, Jacot Des Combes, Roxy, Losada, McInnes, Ratter, Rivera-Arriaga, Susanto, Swingedouw and Tibig2019; Chand et al., Reference Chand, Dowdy, Bell and Tory2020). The trajectory and frequency of tropical cyclones in the South Pacific is typically driven by the natural variation of ENSO (Lafale et al., Reference Lafale, Diamond and Anderson2018), and severe storms are more likely linked to extreme El Niño events with an extensive eastward propagation (Santoso et al., Reference Santoso, McGregor, Jin, Cai, England, An, McPhaden and Guilyardi2013; Stephens & Ramsay, Reference Stephens and Ramsay2014). Deep ocean swells generated by extratropical cyclones in mid and high latitudes are also known to occasionally reach Pacific islands (IPCC, Reference Pachauri and Meyer2014b). For example, oceanic swells originated in the North Pacific, especially during years with a strong El Niño, have caused damage to reefs in Hawaii (Rooney et al., Reference Rooney, Fletcher, Grossman, Engels and Field2004; Fletcher et al., Reference Fletcher, Bochicchio, Conger, Engels, Feirstein, Frazer, Glenn, Grigg, Grossman, Harney, Isoun, Murray-Wallace, Rooney, Rubin, Sherman and Vitousek2008). At Henderson Island, sections of fore-reef have been eroded exposing the bedrock underneath and coral rubble has been found below 30 m depth, all of which is likely to have been caused by storm wave action (Irving & Dawson, Reference Irving, Dawson and Sheppard2013). At Ducie Island, there are observations of coral damage caused by unusual oceanic cold intrusions in the atoll. Rehder & Randall (Reference Rehder and Randall1975) documented up to 40% coral damage by cold-water stress found between 10–15 m depth recorded during an expedition in 1970. During diver surveys between 2016–2018, coral bleaching was documented between 10–15 m depth and linked to cold-water anomalies following the 2015–2016 El Niño event, confirmed by SST satellite data (Rehder & Randall, Reference Rehder and Randall1975; Irving & Dawson, Reference Irving, Dawson and Sheppard2013; Dawson & Zhang, Reference Dawson and Zhang2020). Also in Ducie Island in 1975, parts of the land were found deforested by high waves (Rehder & Randall, Reference Rehder and Randall1975). There is also evidence of transient conditions of coastal turbidity and sediment runoff at Pitcairn Island in 2012 following a major rainfall event, the heaviest since records began, although it is thought that the prevailing strong currents around the island would have dispersed the suspended sediments, mitigating the effects of turbidity and siltation (Irving & Dawson, Reference Irving, Dawson and Sheppard2013; Friedlander et al., Reference Friedlander, Caselle, Ballesteros, Brown, Turchik and Sala2014; Irving et al., Reference Irving, Dawson, Christian and Sheppard2019). Given the small size of Pitcairn Island and its population and the domestic scale of current land use practices, the low cover of live coral could be attributed to exposure to wave action, rather than as an effect of runoff and sedimentation.
Future sea level rise and storm projections
Sea levels are highly variable in the Pacific, but it is very likely that under high emissions scenarios and by the end of this century, the South Pacific in particular will experience the largest sea level rise globally, ranging 54–217 cm (Nurse et al., Reference Nurse, McLean, Agard, Briguglio, Barros, Field, Dokken, Mastrandrea, Mach, Bilir, Chatterjee, Ebi, Estrada, Genova, Girma, Kissel, Levy, MacCracken, Mastrandrea and White2014; Lafale et al., Reference Lafale, Diamond and Anderson2018; Vousdoukas et al., Reference Vousdoukas, Mentaschi, Voukouvalas, Verlaan, Jevrejeva, Jackson and Feyen2018). This presents a significant risk for small coral islands, especially when combined with a potential intensification of tropical cyclones and degraded fringe reefs (The World Bank, 2016; Lafale et al., Reference Lafale, Diamond and Anderson2018). A rise of more than 1 m by the end of the century is considered to be equivalent to a one in 100-year extreme sea level event currently (Vousdoukas et al., Reference Vousdoukas, Mentaschi, Voukouvalas, Verlaan, Jevrejeva, Jackson and Feyen2018; Kench et al., Reference Kench, Ford and Owen2018a; Masselink et al., Reference Masselink, Beetham and Kench2020; Tajima et al., Reference Tajima, Shoji and Taniguchi2020). For the Pitcairn Islands however, sea level projections are still highly uncertain (Zhang et al., Reference Zhang, Church, Monselesan and White2014) due to lack of resolution and long-term data, but there is an expectation that the rate of sea level rise is likely to accelerate and threaten the smaller low-lying islands (Kench et al., Reference Kench, Thompson, Ford, Ogawa and McLean2015), particularly as compounded with coral decalcification.
Mean significant wave heights appear to have increased in regions of the Pacific and Southern Oceans, but there is as yet low confidence in these projections and the lack of data means it is challenging to discern between long-term climate trends and natural variability (Wang & Swail, Reference Wang and Swail2006; Young et al., Reference Young, Zieger and Babanin2011; Seneviratne et al., Reference Seneviratne, Nicholls, Easterling, Goodess, Kanae, Kossin, Luo, Marengo, McInnes, Rahimi, Reichstein, Sorteberg, Vera, Zhang, Field, Barros, Stocker, Qin, Dokken, Ebi, Mastrandrea, Mach, Plattner, Allen, Tignor and Midgley2012; Church et al., Reference Church, Clark, Cazenave, Gregory, Jevrejeva, Levermann, Merrifield, Milne, Nerem, Nunn, Payne, Pfeffer, Stammer, Unnikrishnan, Stocker, Qin, Plattner, Tignor, Allen, Boschung, Nauels, Xia, Bex and Midgley2013). At present however, most tropical cyclones in the South Pacific basin are formed in the region of the Vanuatu archipelago (Diamond et al., Reference Diamond, Lorrey and Renwick2013), strengthening as they move southwards, and so the Pitcairn Islands tend to miss the most severe events (Lafale et al., Reference Lafale, Diamond and Anderson2018). Instead, the impact of long-distance ocean swells from remote low-pressure systems such as the Southern Ocean could be more significant for the Pitcairn Island corals.
Other human pressures in the Pitcairn Islands
In spite of their isolation and the small size of the resident human community, non-climatic anthropogenic pressures – some transboundary – may be impacting the marine environment of the Pitcairn Islands, and particularly the corals.
Fishing
Prior to 2016, most non-commercial fishing took place around Pitcairn Island, with occasional (every 4 to 5 years) trips to 40-Mile Reef to the east-south-east of the island (Irving and Dawson, Reference Irving and Dawson2012). There is no mandatory recording of catches at present and only intermittent fisheries monitoring, but reconstructed data indicate that landings by this small-scale fishery have been higher than the data reported by the Food and Agriculture Organization of the United Nations on behalf of the Pitcairn Islands, although they declined to 4 tonnes year−1 overall by 2014 (Sea Around Us, 2016; Coghlan et al., Reference Coghlan, White, Dawson, Irving, Zeller and Palomares2017). Licenced commercial fishing declined sharply after 2005 as fleets withdrew to international waters outside the EEZ (Irving & Dawson, Reference Irving and Dawson2012). All forms of fishing have been banned within the Pitcairn Islands' MPA since its designation in 2016, apart from artisanal fishing by the local community which is allowed within the coastal conservation areas. There have been some concerns of overfishing targeting groupers, sharks and lobsters (Irving & Dawson, Reference Irving and Dawson2012; Coghlan et al., Reference Coghlan, White, Dawson, Irving, Zeller and Palomares2017) which could have negative consequences for the reef since some of these species, sharks particularly, play an important role in its resilience (Roff et al., Reference Roff, Doropoulos, Rogers, Bozec, Krueck, Aurellado, Priest, Birrell and Mumby2016). A Fisheries Management Plan was introduced in 2017 for the management of the local fishery, particularly around Pitcairn Island (Irving et al., Reference Irving, Dawson, Christian and Sheppard2019; Dawson & Irving, Reference Dawson and Irving2020). The management plan brought in minimum catch sizes for a number of species of fish and shellfish and also banned the fishing of sharks.
Marine litter
Ocean-borne litter is an example of transboundary pressure in the Pitcairn Islands. Henderson Island is reported to have one of the highest levels of marine plastic litter on the planet despite it being uninhabited (Lavers & Bond, Reference Lavers and Bond2017; Forrest & Hindell, Reference Forrest and Hindell2018; Irving et al., Reference Irving, Dawson, Christian and Sheppard2019; Ryan, Reference Ryan2020; Ryan & Schofield, Reference Ryan and Schofield2020). This island is on the edge of the South Pacific Gyre, which collects litter from distant Pacific shores and deposits it on the island's shores (UK Gov Marine Developments, 2019). On the beaches of Henderson below the accumulated plastic, temperature within the sand was found to be +2.5°C above the daily maximum and dropped down by −1.5°C below the daily minimum, compared with areas with no plastic debris (Lavers et al., Reference Lavers, Rivers-Auty and Bond2021). Quantities of plastic items were also found tangled on the surrounding reefs (S. Archer-Rand, personal communication).
Anchoring
Anchoring from visiting cruise ships and yachts and from supply vessels is another concern (Irving et al., Reference Irving, Dawson, Christian and Sheppard2019). Anchors and anchor warps can destroy large swathes of seabed as they settle and when retrieved (Giglio et al., Reference Giglio, Ternes, Mendes, Cordeiro and Ferreira2017). Hard corals are especially susceptible to the impacts of anchoring, but the scale of damage and recovery timeframes remains unknown.
Non-native species
The presence of invasive non-native species, even on land, as well as coral diseases, remain important threats to coral reefs. Invasive rats on Henderson Island have caused dramatic declines of the biomass of resident seabirds, including endemic or endangered species (BirdLife International, 2020) and this could cause an indirect negative effect on the surrounding reefs as found in other oceanic coral islands, where declines in nesting seabird populations caused losses in reef productivity due to the loss of guano nutrient inputs (Graham et al., Reference Graham, McClanahan, MacNeil, Wilson, Cinner, Huchery and Holmes2017, Reference Graham, Wilson, Carr, Hoey, Jennings and MacNeil2018; Benkwitt et al., Reference Benkwitt, Wilson and Graham2019, Reference Benkwitt, Gunn, Le Corre, Carr and Graham2021). The eradication of invasive rats should be important for the sustainable management of the natural environment of these islands (Graham et al., Reference Graham, McClanahan, MacNeil, Wilson, Cinner, Huchery and Holmes2017; Benkwitt et al., Reference Benkwitt, Wilson and Graham2019; BirdLife International, 2020; Perez-Correa et al., Reference Perez-Correa, Carr, Meeuwig, Koldewey and Letessier2020). Corallivorous crown-of-thorns starfish have been reported in the Pitcairn Islands (Birkeland, Reference Birkeland1989; Irving, Reference Irving1995), as well as coral diseases.
Discussion
Corals can recover following bleaching and other climate change impacts such as storm damage, and healthy and well-balanced reefs hold the key to this resilience. Herbivorous reef-fish will for example encourage recruitment of new corals by grazing on the algae and preventing their encroachment on the reef (Mumby et al., Reference Mumby, Steneck, Adjeroud and Arnold2016; Steneck et al., Reference Steneck, Arnold, Boenish, de León, Mumby, Rasher and Wilson2019). Good water quality is also particularly important to help recovery following bleaching and to reduce susceptibility to disease outbreaks and invasive species (Carlson et al., Reference Carlson, Foo and Asner2019; MacNeil et al., Reference MacNeil, Mellin, Matthews, Wolff, McClanahan, Devlin, Drovandi, Mengersen and Graham2019). Large MPAs are effective options for reef resilience, as they minimize local stresses and support a wide range of species and functional groups, particularly when they include replicates of representative habitats, ensuring reef connectivity, and encompass different thermal regimes (Carpenter et al., Reference Carpenter, Abrar, Aeby, Aronson, Banks, Bruckner, Chiriboga, Cortés, Delbeek, DeVantier, Edgar, Edwards, Fenner, Guzmán, Hoeksema, Hodgson, Johan, Licuanan, Livingstone, Lovell, Moore, Obura, Ochavillo, Polidoro, Precht, Quibilan, Reboton, Richards, Rogers, Sanciangco, Sheppard, Sheppard, Smith, Stuart, Turak, Veron, Wallace, Weil and Wood2008; McLeod et al., Reference McLeod, Anthony, Mumby, Maynard, Beeden, Graham, Heron, Hoegh-Guldberg, Jupiter, MacGowan, Mangubhai, Marshall, Marshall, McClanahan, McLeod, Nyström, Obura, Parker, Possingham, Salm and Tamelander2019). In the Pitcairn Islands MPA in particular, the deeper, cooler fore-reefs could act as areas of refugia from the effects of warming to corals and other species (Kleypas et al., Reference Kleypas, McManus and Meñez2010; Friedlander et al., Reference Friedlander, Caselle, Ballesteros, Brown, Turchik and Sala2014).
This study suggests that, in the Pitcairn Islands, thermal stress is likely to remain low compared with other tropical reefs globally, particularly in the deeper fore-reefs, although those deeper coral communities appear dominated by sensitive species which may heighten their vulnerability to thermal stress should it occur. The geographic isolation of these reefs is another challenge in terms of supply of healthy larvae, meaning these reefs would rely on self-seeding to boost recolonization and recovery (Robinson et al., Reference Robinson, New, Popova, Srokosz and Yool2017; Romero-Torres et al., Reference Romero-Torres, Treml, Acosta and Paz-García2018). On the other hand, the low level of other local detrimental pressures in these islands such as nutrient inputs or pollution, can help corals better withstand thermal stress (Wiedenmann et al., Reference Wiedenmann, D'Angelo, Smith, Hunt, Legiret, Postle and Achterberg2013). The additional protection granted by the MPA is expected to promote recovery following bleaching (Sheppard et al., Reference Sheppard, Harris and Sheppard2008; Gilmour et al., Reference Gilmour, Smith, Heyward, Baird and Pratchett2013), although there is evidence that severe bleaching and mortality can still occur in highly protected reefs, as demonstrated by the Aldabra Atoll in the Western Indian Ocean (Cerutti et al., Reference Cerutti, Burt, Haupt, Bunbury, Mumby and Schaepman-Strub2020), BIOT (Head et al., Reference Head, Bayley, Rowlands, Roche, Tickler, Rogers, Koldewey, Turner and Andradi-Brown2019), Jarvis Island in the central Equatorial Pacific (Vargas-Ángel et al., Reference Vargas-Ángel, Huntington, Brainard, Venegas, Oliver, Barkley and Cohen Brainard2019) and the Great Barrier Reef (Hughes et al., Reference Hughes, Kerry, Álvarez-Noriega, Álvarez-Romero, Anderson, Baird, Babcock, Beger, Bellwood, Berkelmans, Bridge, Butler, Byrne, Cantin, Comeau, Connolly, Cumming, Dalton, Diaz-Pulido, Eakin, Figueira, Gilmour, Harrison, Heron, Hoey, Hobbs, Hoogenboom, Kennedy, Kuo, Lough, Lowe, Liu, McCulloch, Malcolm, McWilliam, Pandolfi, Pears, Pratchett, Schoepf, Simpson, Skirving, Sommer, Torda, Wachenfeld, Willis and Wilson2017).
There are two main approaches for enhancing climate resilience of ecosystems: management of local pressures and implementation of adaptation and resilience-building actions. Efforts are increasing globally to understand coral recovery and promote adaptation using coordinated ecosystem-based management approaches (Obura & Grimsditch, Reference Obura and Grimsditch2009; NOAA Coral Reef Conservation Programme, 2014; Belokurov et al., Reference Belokurov, Baskinas, Biyo, Clausen, Dudley, Guevara, Lumanog, Rakotondrazafy, Ramahery, Salao, Stolton and Zogib2016; Harvey et al., Reference Harvey, Nash, Blanchard and Edwards2018; Roche et al., Reference Roche, Williams and Turner2018; McLeod et al., Reference McLeod, Anthony, Mumby, Maynard, Beeden, Graham, Heron, Hoegh-Guldberg, Jupiter, MacGowan, Mangubhai, Marshall, Marshall, McClanahan, McLeod, Nyström, Obura, Parker, Possingham, Salm and Tamelander2019). The goal of these adaptation strategies is to ‘maintain the ecosystem in a healthy, productive, and resilient condition so that it can continue to provide the services that society wants and needs’ (NOAA Coral Reef Conservation Programme, 2014).
Here, we discuss potential options for enhancing the climate resilience of coral reefs in the Pitcairn Islands, based on examples from other reefs. Intervention and management actions should be underpinned by scientific evidence wherever possible, and include monitoring plans, review periods and adaptive management approaches (Belokurov et al., Reference Belokurov, Baskinas, Biyo, Clausen, Dudley, Guevara, Lumanog, Rakotondrazafy, Ramahery, Salao, Stolton and Zogib2016). Decisions and actions should also be undertaken in consultation with and with the full involvement of the local community, as custodians and direct beneficiaries of these reefs, so they can contribute their valuable knowledge. An example of a step-by-step process that could be followed to develop an Action Plan for building and enhancing climate change adaptation and resilience of the coral reefs of the Pitcairn Islands is represented in Figure 5.
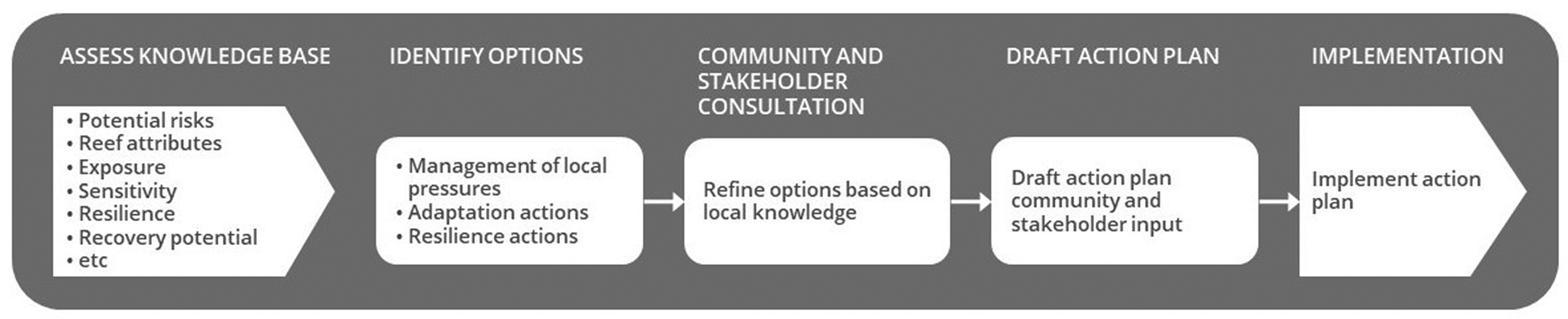
Fig. 5. Conceptual diagram for the development of a climate change adaptation and resilience action plan for the coral reefs of the Pitcairn Islands, adapted from Obura & Grimsditch (Reference Obura and Grimsditch2009) and Belokurov et al. (Reference Belokurov, Baskinas, Biyo, Clausen, Dudley, Guevara, Lumanog, Rakotondrazafy, Ramahery, Salao, Stolton and Zogib2016).
Next, we discuss a number of considerations to develop a plan of climate adaptation and resilience for the Pitcairn Islands' reefs, that could potentially be incorporated into the framework of the Pitcairn Islands MPA.
Enhancing resilience through management of local pressures
Reducing pressures from human activities and regulating the uses of the marine environment may not entirely prevent coral bleaching, or coral mortality, but boost the resilience of reefs and their chances of recovery (Nyström et al., Reference Nyström, Graham, Lokrantz and Norström2008; IPCC, Reference Barros, Field, Dokken, Mastrandrea, Mach, Bilir, Chatterjee, Ebi, Estrada, Genova, Girma, Kissel, Levy, MacCracken, Mastrandrea and White2014a; UNEP, 2017). Approaches of this kind can help delay the onset of mass loss of corals, allowing time for emissions mitigation measures to take effect in limiting global warming (IPCC, Reference Barros, Field, Dokken, Mastrandrea, Mach, Bilir, Chatterjee, Ebi, Estrada, Genova, Girma, Kissel, Levy, MacCracken, Mastrandrea and White2014a, Reference Pörtner, Roberts, Masson-Delmotte, Zhai, Tignor, Poloczanska, Mintenbeck, Nicolai, Okem, Petzold, Rama and Weyer2019b). There are strict regulations as part of the designation of the Pitcairn Islands MPA, a highly protected marine area and one of the largest in the world at 841,910 km2 (UNEP-WCMC & IUCN, 2021). They include a 5-year Management Plan to limit local pressures as well as long-term monitoring of the coral reefs to assess reef health. This vast MPA hosts a network of shallow and deep reefs across a range of different thermal regimes, likely to offer taxonomic overlap and a well-connected reef system with areas of refugia and therefore appears to meet reef resilience management requirements (McLeod et al., Reference McLeod, Anthony, Mumby, Maynard, Beeden, Graham, Heron, Hoegh-Guldberg, Jupiter, MacGowan, Mangubhai, Marshall, Marshall, McClanahan, McLeod, Nyström, Obura, Parker, Possingham, Salm and Tamelander2019). Given their isolated location and low population, the Pitcairn Islands are exposed to fewer pressures compared with other similar coral atoll islands, but nevertheless the following pressures have been identified in the Pitcairn MPA Management Plan: Illegal, Unreported and Unregulated (IUU) fishing by foreign fishing vessels; pollution including oil spills and marine plastics; anchor damage; erosion and run-off from land; and invasive non-native species.
Fishing
The implementation of large, highly protected marine areas such as the Pitcairn Islands MPA can be an effective climate resilience option for the corals and other marine communities (Hughes et al., Reference Hughes, Baird, Bellwood, Card, Connolly, Folke, Grosberg, Hoegh-Guldberg, Jackson, Kleypas, Lough, Marshall, Nystrom, Palumbi, Pandolfi, Rosen and Roughgarden2003; McClanahan et al., Reference McClanahan, Cinner, Maina, Graham, Daw, Stead, Wamukota, Brown, Ateweberhan, Venus and Polunin2008; Coghlan et al., Reference Coghlan, White, Dawson, Irving, Zeller and Palomares2017; Hays et al., Reference Hays, Koldewey, Andrzejaczek, Attrill, Barley, Bayley, Benkwitt, Block, Schallert, Carlisle, Carr, Chapple, Collins, Diaz, Dunn, Dunbar, Eager, Engel, Embling, Esteban, Ferretti, Foster, Freeman, Gollock, Graham, Harris, Head, Hosegood, Howell, Hussey, Jacoby, Jones, Pilly, Lange, Letessier, Levy, Lindhart, McDevitt-Irwin, Meekan, Meeuwig, Micheli, Mogg, Mortimer, Mucciarone, Nicoll, Nuno, Perry, Preston, Rattray, Robinson, Roche, Schiele, Sheehan, Sheppard, Sheppard, Smith, Soule, Spalding, Stevens, Steyaert, Stifel, Taylor, Tickler, Trevail, Trueba, Turner, Votier, Wilson, Williams, Williamson, Williamson, Wood and Curnick2020). The Pitcairn Islands MPA limits local fishing activities to coastal conservation areas (Pitcairn Government, 2017). A Fisheries Management Plan regulates the inshore artisanal fishery including gear restrictions and minimum landing sizes (Irving et al., Reference Irving, Dawson, Christian and Sheppard2019; Dawson & Irving, Reference Dawson and Irving2020), and a separate Compliance and Enforcement Strategy covers the surveillance and prosecution of IUU fishing offences. An immediate ecological benefit to the reef results from allowing healthy stocks of herbivorous reef-fish and invertebrates to remain and graze on the algae that otherwise could occupy the reef, freeing up substrate for coral recruitment (Mumby et al., Reference Mumby, Hastings and Edwards2007; Steneck et al., Reference Steneck, Arnold, Boenish, de León, Mumby, Rasher and Wilson2019). There is evidence in the Caribbean region, where measures such as this enabled recovery of damaged reefs following hurricane impacts and bleaching (Steneck et al., Reference Steneck, Arnold, Boenish, de León, Mumby, Rasher and Wilson2019).
Predatory fish are also important to keep smaller, corallivorous fish, and other trophic groups in check and therefore preserve a healthy functional reef. Previous fish assessments indicate that whilst fish communities in the Pitcairn Islands are relatively healthy, numbers of sharks and groupers have been locally impoverished due to targeted catches (Friedlander et al., Reference Friedlander, Caselle, Ballesteros, Brown, Turchik and Sala2014; Coghlan et al., Reference Coghlan, White, Dawson, Irving, Zeller and Palomares2017; Dawson & Irving, Reference Dawson and Irving2020; Duffy et al., Reference Duffy, Letessier, Koldewey, Dawson and Irving2021).
Pollution and litter
Improving water quality can also promote climate resilience in corals. Ridge-to-reef coastal management approaches help control and reduce land sources of pollution and disturbance (Carlson et al., Reference Carlson, Foo and Asner2019). Moreover, coral monitoring in the Great Barrier Reef show that improving water quality helps coral recovery following bleaching and it also reduces susceptibility to crown-of-thorns starfish outbreaks and diseases (MacNeil et al., Reference MacNeil, Mellin, Matthews, Wolff, McClanahan, Devlin, Drovandi, Mengersen and Graham2019). On Pitcairn Island, macroalgae are more abundant here compared with the other three islands, possibly as a combination of runoff (Irving et al., Reference Irving, Dawson and Wowor2017) and wave action. Changes in land use and soil erosion issues on Pitcairn Island have led to excess runoff inputs into coastal waters during heavy rain events resulting in short-term sedimentation turbidity (Friedlander et al., Reference Friedlander, Caselle, Ballesteros, Brown, Turchik and Sala2014). However, coastal waters around all four islands are unusually clear overall suggesting oligotrophic conditions with low concentrations of dissolved inorganic nutrients. Preserving land vegetation and encouraging sustainable agricultural practices would help prevent soil erosion and maintain good water quality.
A more noticeable issue in terms of marine pollution in the case of the Pitcairn Islands is plastic litter, with Henderson Island declared to have some of the highest levels of ocean-borne plastic litter on the planet (Forrest & Hindell, Reference Forrest and Hindell2018; Irving et al., Reference Irving, Dawson, Christian and Sheppard2019; Ryan, Reference Ryan2020; Ryan & Schofield, Reference Ryan and Schofield2020). Marine plastics are known to endanger many marine animals and although a specific direct link to the corals themselves (other than by physical damage from large-size items such as entangled fishing nets) is not overly clear, it has been suggested that the proliferation of plastic litter increases the risk of coral diseases (Lamb et al., Reference Lamb, Willis, Fiorenza, Couch, Howard, Rader, True, Kelly, Ahmad, Jompa and Harvell2018).
Invasive species and coral diseases
Other important threats to corals are diseases and the presence of invasive and non-native species, even on land. A dramatic example is the introduction of rats to small islands, not just in the Pitcairn Islands but also in the British Indian Ocean Territory. As the rats caused a crash in the population of breeding seabirds, this resulted in a detrimental knock-on effect to coastal reefs following the loss of nutrients inputs from the birds’ guano (Graham et al., Reference Graham, McClanahan, MacNeil, Wilson, Cinner, Huchery and Holmes2017, Reference Graham, Wilson, Carr, Hoey, Jennings and MacNeil2018; Benkwitt et al., Reference Benkwitt, Wilson and Graham2019). Eradication of rats should therefore be a conservation priority for the islands as it could have positive effects on the nearshore corals if seabird populations are able to re-establish their numbers to previous levels (BirdLife International, 2020), and the inputs of guano nutrients to the reef areas are restored (Graham et al., Reference Graham, McClanahan, MacNeil, Wilson, Cinner, Huchery and Holmes2017; Benkwitt et al., Reference Benkwitt, Wilson and Graham2019; BirdLife International, 2020; Perez-Correa et al., Reference Perez-Correa, Carr, Meeuwig, Koldewey and Letessier2020). Finally, although very challenging given the vast size and remoteness of the Pitcairn Islands MPA, early warning, monitoring or screening surveys (for example, by applying novel techniques such as detection of environmental DNA) could help detect outbreaks of crown-of-thorns and coral diseases, both of which can have equally devastating consequences for reefs (Birkeland, Reference Birkeland1989; Irving, Reference Irving1995; Galloway et al., Reference Galloway, Bruckner and Woodley2009).
Anchor damage
Anchoring can damage corals and other benthic communities (Irving et al., Reference Irving, Dawson, Christian and Sheppard2019). Some measures have been taken towards limiting anchoring of cruise ships, yachts and supply vessels to certain areas, but the seabed around these locations should be monitored regularly, so that further measures can be put in place if necessary (Lewis et al., Reference Lewis, Day, Wilhelm, Wagner, Gaymer, Parks, Friedlander, White, Sheppard, Spalding, San Martin, Skeat, Taei, Teroroko and Evans2017; Sheppard & Sheppard, Reference Sheppard and Sheppard2019). For example, permanent moorings can help reduce the impacts of anchoring.
Promoting coral recovery through adaptation and restoration
Reducing future global greenhouse gas emissions is, at present, the only feasible pathway to controlling future global warming and therefore effectively limiting climate change impacts on coral reefs (Bruno et al., Reference Bruno, Côté and Toth2019). Tangible and targeted management measures and regular monitoring can help in determining what, if any, active steps are required to further promote coral resilience and adaptation. These measures are likely to involve an array of interventions, from improving protection through MPA management, to reducing local pressures, and to restoring degraded and damaged reefs (Abelson, Reference Abelson2020).
Recent coral planting and seeding initiatives have had some local success in remediating coral reefs elsewhere (Doropoulos et al., Reference Doropoulos, Vons, Elzinga, ter Hofstede, Salee, van Koningsveld and Babcock2019; IPCC, Reference Pörtner, Roberts, Masson-Delmotte, Zhai, Tignor, Poloczanska, Mintenbeck, Nicolai, Okem, Petzold, Rama and Weyer2019b). Planting or seeding of corals can draw on thermally resistant species or strains (Dance, Reference Dance2019), and so while this might change the diversity or species composition of the corals in an area, it can enhance the overall resilience of the reef. Coral recruitment can also be enhanced by collecting gametes from the sea during spawning, and following fertilization returning settled larvae to the sea a few days later (Dance, Reference Dance2019). Research is underway in Australia to breed heat-tolerant coral species and strains (Cornwall, Reference Cornwall2019). Coral husbandry also allows testing if corals can adapt to warming temperatures, and genetic manipulation of the bacteria or zooxanthellae in the corals, or even the corals themselves, may allow the creation of new heat-tolerant mixtures (Crabbe, Reference Crabbe2019). However, coral restoration is usually only targeted at reefs that reach a critical stage of damage, to aid recovery, which is not the case in the Pitcairn Islands at this moment. Furthermore, coral restoration interventions are expensive and labour-intensive, and would be logistically challenging on remote reefs as these. Reducing bleaching during heatwaves by using artificial shading at times of high sea temperatures has also been proposed (Belokurov et al., Reference Belokurov, Baskinas, Biyo, Clausen, Dudley, Guevara, Lumanog, Rakotondrazafy, Ramahery, Salao, Stolton and Zogib2016); again, this may not be a practical choice of intervention in the Pitcairn Islands' reefs.
All these potential interventions can be very labour intensive and costly, and often involve complex decisions (Abelson, Reference Abelson2020; Anthony et al., Reference Anthony, Helmstedt, Bay, Fidelman, Hussey, Lundgren, Mead, McLeod, Mumby, Newlands, Schaffelke, Wilson and Hardisty2020) and are not without uncertainties and controversy as to their efficacy. Their implementation across large and remote areas such as the Pitcairn Islands MPA presents big challenges.
Further options for coral action on the Pitcairn Islands
Monitoring the reefs and other vulnerable benthic habitats around Pitcairn, Henderson, Oeno and Ducie Islands would help identify any impacts. It would be important to adopt a strategic approach to any monitoring, optimized for example by gathering additional data to support the Fisheries Management Plan, and by coordinating efforts and maximizing coverage of the MPA, and this is already contemplated as part of a Monitoring and Research Plan associated to the Pitcairn Islands MPA Management Plan.
Knowledge gaps and future research needs
Habitat mapping and reef vulnerability assessments
There remain large data gaps on the coral communities of the Pitcairn Islands (Friedlander et al., Reference Friedlander, Caselle, Ballesteros, Brown, Turchik and Sala2014) making it difficult to determine a pre-bleaching baseline, and therefore habitat mapping of coral reefs and other benthic communities across the MPA appears to be an important and necessary step. Regular coral monitoring of key ecological indicators could also be implemented, including, but not limited to: coral cover; species composition; disease screenings; occurrence of non-native invasive species such as crown-of-thorns; coral spawning and recruitment studies; water quality; changes to macroalgal cover; and fish groups counts. A smart and targeted approach to data acquisition would ensure that the information is ecologically relevant and provide the necessary evidence to support adaptive management measures.
A coral vulnerability assessment (Belokurov et al., Reference Belokurov, Baskinas, Biyo, Clausen, Dudley, Guevara, Lumanog, Rakotondrazafy, Ramahery, Salao, Stolton and Zogib2016; UNEP, 2017) would be useful to characterize exposure and sensitivity to climate change or other pressures across the MPA and inform decisions on interventions. Coral monitoring can also be used to identify more resistant species or colonies (Belokurov et al., Reference Belokurov, Baskinas, Biyo, Clausen, Dudley, Guevara, Lumanog, Rakotondrazafy, Ramahery, Salao, Stolton and Zogib2016), so they can be prioritized for protection and research.
Finally, further investigations on the deeper reefs looking at factors such as temperature profiles, light attenuation, coral community composition, and connectivity to shallow reefs, would help determine their potential as climate refugia.
Coral conservation interventions
While some of the emerging intervention techniques such as planting, seeding and genetic engineering of coral and zooxanthellae strains may appear promising in other global reef regions, some of those methods are still debatable and require further research and at this point would be too labour-intensive and expensive to be practical or even necessary in the case of the Pitcairn Islands. It has been proposed that some of the healthier reefs in the Pitcairn Islands have the potential to become coral seed banks for use in coral conservation and restoration projects in other degraded reefs in the wider Pacific. Given the remoteness of the Pitcairn Islands, this would present important logistical challenges; however as trials in the Great Barrier Reef show it remains a possibility. In any case it would require further research, not the least because unlike corals from the Red Sea and the Persian or Arabian Gulf, which are exposed to higher levels of environmental stress, the Pitcairn Islands corals are likely to be thermally naïve and therefore sensitive.
Ocean acidification
It is important to improve our understanding of ocean carbon chemistry and coral adaptation in the Pitcairn Islands, given the high and immediate risk posed by ocean acidification. These efforts could be integrated as part of the Global Ocean Acidification Observing Network (GOA-ON) monitoring network expansion, ideally by encouraging capacity in location. Given that this is a data-poor region, and taking into consideration the potential severity of calcium carbonate minerals saturation suggested by future projections, ocean acidification research in the Pitcairn Islands deserves urgent attention. The use of autonomous sensor technology could be a feasible, cost-effective option worth considering.
Research of ocean acidification on corals focuses on single-species responses. Increasingly, experiments now combine multiple climatic and non-climatic stressors on single species, which are revealing how other stressors interact with ocean acidification. It is not yet clear to what extent corals and other marine organisms can acclimatize and adapt to ocean acidification, however. In situ studies are required to build an understanding of effects in single species, and extrapolate these responses to impacts on whole reef systems and functions, including food webs and competition.
Sea level
It is important to better understand the processes by which coral islands adjust to sea level rise (Barnett & O'Neill, Reference Barnett and O'Neill2012; Kench et al., Reference Kench, Thompson, Ford, Ogawa and McLean2015). Rising sea level is expected to cause coastal erosion, remobilize sediments and destabilize islands, but there is debate about the likely physical changes to atolls in the future (Kench et al., Reference Kench, Thompson, Ford, Ogawa and McLean2015, Reference Kench, McLean, Owen, Tuck and Ford2018b; Storlazzi et al., Reference Storlazzi, Gingerich, van Dongeren, Cheriton, Swarzenski, Quataert, Voss, Field, Annamalai, Piniak and McCall2018; Ford et al., Reference Ford, Kench, Owen and Hua2020). Further research into atoll coastal processes in response to sea level rise and reef erosion in the Pitcairn Islands, particularly at Ducie, Henderson and Oeno, would be important.
Implementation of fisheries management and collection of catch records
There is a need for an effective management of the local artisanal fishery (Bell et al., Reference Bell, Johnson, Ganachaud, Gehrke, Hobday, Hoegh-Guldberg, Le Borgne, Lehodey, Lough, Pickering, Pratchett and Waycott2011; Coghlan et al., Reference Coghlan, White, Dawson, Irving, Zeller and Palomares2017; Dawson & Irving, Reference Dawson and Irving2020). Data and information gathered as part of the fisheries management and catch recording should be integrated and coordinated with marine monitoring activities across the wider MPA, including corals. There are plans to address this as part of the MPA Management Plan and associated Monitoring and Research Plan.
Summary and conclusions
The Pitcairn Islands are some of the most remote and best preserved oceanic island environments (Readman et al., Reference Readman, Deluna, Ebinghaus, Guzman, Price, Readman, Sheppard, Sleight, Sturm, Thompson, Tonkin, Wolschke, Wright and Sheppard2013; Moritz et al., Reference Moritz, Vii, Long, Tamelander, Thomassin and Planes2018) where the low level of other human pressures could allow them to persist longer into the future (UNEP, 2017). To date, no significant changes in average SST have been detected, although marine heatwaves have been observed (in 1995, 2006 and 2017). There is, so far, no evidence of coral bleaching from seawater warming in the Pitcairn Islands, but the reefs' species composition suggests that they would likely be sensitive to thermal stress should that occur. Also, the geographic isolation of these reefs is an added challenge in terms of larval recruitment. Although recruitment of juvenile corals has yet to be assessed, the isolation of these islands, being further than 100 km away from each other and even further removed from any other reefs, indicates limited connectivity and high reliance on self-seeding (Swearer et al., Reference Swearer, Caselle, Lea and Warner1999; Wood et al., Reference Wood, Paris, Ridgwell and Hendy2014). Furthermore, even if these reefs persist their composition and structural complexity is likely to change (Sheppard et al., Reference Sheppard, Sheppard and Fenner2020). Ocean acidification appears to be a significant and more immediate risk to these reefs, likely to lead to coral de-calcification by the end of the century under a high emissions scenario.
Future climate change is also likely to bring about a host of combined pressures, feedbacks and indirect impacts on coral reefs, some of which are unknown. The knowledge and appreciation of such cumulative impacts are growing rapidly (Ateweberhan et al., Reference Ateweberhan, Feary, Keshavmurthy, Chen, Schleyer and Sheppard2013; Cinner et al., Reference Cinner, Pratchett, Graham, Messmer, Fuentes, Ainsworth, Ban, Bay, Blythe, Dissard, Dunn, Evans, Fabinyi, Fidelman, Figueiredo, Frisch, Fulton, Hicks, Lukoschek, Mallela, Moya, Penin, Rummer, Walker and Williamson2016; Bruno et al., Reference Bruno, Côté and Toth2019). Even among the direct impacts, there are major differences in the understanding and confidence surrounding impact pathways, that are partly attributable to differences in the timing of impacts: for example while impacts from warming SST and storms are already being observed, those from sea level rise and ocean acidification will take time to emerge (Cinner et al., Reference Cinner, Pratchett, Graham, Messmer, Fuentes, Ainsworth, Ban, Bay, Blythe, Dissard, Dunn, Evans, Fabinyi, Fidelman, Figueiredo, Frisch, Fulton, Hicks, Lukoschek, Mallela, Moya, Penin, Rummer, Walker and Williamson2016). As research in these fields continues, it is expected that new impact pathways will be found (Cinner et al., Reference Cinner, Pratchett, Graham, Messmer, Fuentes, Ainsworth, Ban, Bay, Blythe, Dissard, Dunn, Evans, Fabinyi, Fidelman, Figueiredo, Frisch, Fulton, Hicks, Lukoschek, Mallela, Moya, Penin, Rummer, Walker and Williamson2016). For example, ocean acidification is likely to increase susceptibility of corals to storm damage because a lower seawater pH will weaken coral skeleton density and strength (Hoegh-Guldberg et al., Reference Hoegh-Guldberg, Mumby, Hooten, Steneck, Greenfield, Gomez, Harvell, Sale, Edwards, Caldeira, Knowlton, Eakin, Iglesias-Prieto, Muthiga, Bradbury, Dubi and Hatziolos2007; Madin et al., Reference Madin, O'Donnell and Connolly2008). De-calcification may also be exacerbated by warming ocean temperature, as is the case for coralline algae (Martin & Gattuso, Reference Martin and Gattuso2009). To date, research on how synergies between key climate drivers affect coral reef systems is very limited (Ban et al., Reference Ban, Graham and Connolly2014) and therefore assessments of climate change impacts usually focus on individual drivers, rather than on cumulative effects (Cinner et al., Reference Cinner, Pratchett, Graham, Messmer, Fuentes, Ainsworth, Ban, Bay, Blythe, Dissard, Dunn, Evans, Fabinyi, Fidelman, Figueiredo, Frisch, Fulton, Hicks, Lukoschek, Mallela, Moya, Penin, Rummer, Walker and Williamson2016). Latest IPCC projections for warm-water coral reefs globally indicate that increased water depth due to combined coral loss, reef erosion and loss of structural complexity will exacerbate the risk of flooding to reef-fringed shorelines and islands and will cause declines of reef fish populations (IPCC, Reference Pörtner, Roberts, Tignor, Poloczanska, Mintenbeck, Alegría, Craig, Langsdorf, Löschke, Möller, Okem and Rama2022).
Owing to their isolation and low levels of human impacts, further studies of the Pitcairn Islands' reefs can help disentangle these synergies and provide answers to many of these scientific questions, for the benefit of this as well as other reef areas around the world.
Acknowledgements
We are very grateful for the constructive and insightful comments received from two anonymous reviewers, which helped us improve the final version of this article.
Author contributions
Susana Lincoln: Writing – Original Draft Lead Author, Review and Editing, Supervision; Benjamin Cowburn: Writing – Draft Sea Temperature Change Section; Ella L. Howes: Writing – Draft Ocean Acidification Section; Silvana Birchenough: Review – Draft Ocean Acidification Section, Review and Editing; John K. Pinnegar: Review; Stephen Dye: Writing – Draft Sea Level Rise Section; Paul Buckley: Review and Editing; Charles Sheppard: Review; Colette C. C. Wabnitz: Review; Leo X. C. Dutra: Review; Jennifer Graham: Review – Draft Sea Level Rise Section; Simeon Archer-Rand: Review; Emily Hardman: Review; Georg H. Engelhard: Review and Editing; Bryony L. Townhill: Writing – Original Draft Building Resilience to Climate Change and Knowledge Gaps and Future Research Needs, Review and Editing.
Financial support
This study was supported by the UK Government Blue Belt Programme (www.gov.uk/government/publications/the-blue-belt-programme).
Conflict of interest
The authors declare that they have no known conflict of interests or personal relationships that could have appeared to influence the work reported in this paper.