INTRODUCTION
Foodborne disease has a significant public health impact worldwide [Reference Scallan1]. The monthly prevalence of acute gastroenteritis has been estimated to be 3·4% in Ireland, 6·4% in Australia and 7·6% in Canada and USA [Reference Scallan1]. Campylobacter and Salmonella spp. account for more than 90% of all reported cases of bacterial foodborne illness worldwide [Reference Thorns2]. Apparently healthy poultry, swine and beef carry such organisms, and contaminated products of animal origin are important sources of foodborne infections for humans [Reference Friedman3–Reference Humphrey, O'Brien and Madsen5]. While the bacterial food safety of conventional food-animal production has been extensively studied and reviewed by experts [Reference Forshell and Wierup4–Reference Callaway7], both primary research and reviews on this aspect in organic food-animal production are scarce, perhaps due to the relatively recent growth in popularity of organic food-animal production [Reference Kouba8, Reference Winter and Davis9].
Organic production is an agricultural system that emphasizes animal welfare and ecosystem sustainability while minimizing the use of off-farm inputs. Prophylactic use of antimicrobials and growth hormones is prohibited, although antimicrobials can be used to treat ill animals when all other options fail [10, 11]. Organic animals generally require daily outdoor access and must receive organic feed, but these and other requirements can differ by jurisdiction [10, 11].
Consumers often purchase organic products because they believe them to be healthier or safer than conventional products [Reference Hammitt12, Reference Williams and Hammitt13]. As consumer interest and demand for organic products increases, a better understanding of the microbial food safety of organic production is necessary. Systematic reviews are a transparent and replicable method for summarizing or synthesizing available evidence on a topic, yet are under-utilized in agri-food public health [Reference Sargeant14]. This methodology was used to compare the prevalence of bacterial enteropathogens, potentially zoonotic bacteria and bacteria resistant to antimicrobials in organic and conventional poultry, swine and beef production. The quantity, consistency and methodological soundness of all published primary research in this area was identified and evaluated. When appropriate, meta-analysis was applied on selected data subsets to generate pooled prevalence estimates.
METHODS
Literature search
An initial literature search was conducted in January 2007 and was updated in December 2007 and July 2008. No language or other restrictions were imposed. The databases used in the initial search were Agricola (1970–2007), Biological Sciences (1982–2007), BioMed Central (1997–2007), CAB Abstracts (1973–2007), Current Contents (1999–2007), Environmental Sciences and Pollution Management (1967–2007), Food Science and Technology Abstracts (1969–2007), Medline (1949–2007) and Scopus (1969–2007). The updated searches were restricted to Agricola, CAB Abstracts and Medline. Reference lists from all relevant articles (n=50) and from selected chapters of the Handbook of Organic Food Safety and Quality [Reference Cooper, Niggli and Leifert15] were hand-searched to identify any additional relevant citations that were potentially missed by the online searches.
The searches were conducted using combinations of terms covering three main question components: (1) organic production, (2) food-animal populations and (3) bacterial and antimicrobial resistance (AMR) outcomes. To increase search specificity for the updated searches, only bacterial terms were used as the outcome because all studies describing AMR were also captured with these terms, and for the most updated search the search algorithm was shortened by removing redundant terms. Citations were managed and de-duplicated using Procite 5.0 (Thomson ResearchSoft, USA) and web-based software (SRS 4.0, TrialStat! Corporation, Canada) was used to manage the systematic review.
Relevance screening, quality assessment and data extraction
Relevance screening, quality assessment and data extraction was conducted using standardized web-based forms. Each form was pre-tested by all reviewers on a sample of citations (n=50 abstracts for relevance screening, n=5 articles for quality assessment, n=2 articles for data extraction). Forms were utilized when kappa agreements exceeded 0·8 for all reviewing pairs. Relevance screening was conducted in two stages. The first stage consisted of a form with one question that was used to rapidly identify all citations that described organic food-animal production, while the second stage consisted of a form used to classify abstracts by food-animal species, outcome and sampling point (i.e. farm, processing or retail). Non-primary research studies that did not clearly define ‘organic animal production’ or that did not measure the prevalence of bacterial enteropathogens, potentially zoonotic bacteria or bacterial resistance to antimicrobials were excluded.
Upon completion of relevance screening, relevant articles were procured and assessed for methodological soundness using the following five criteria: (1) comparison groups sampled from the same target population, (2) sample size explicitly justified, (3) formal systematic or random sampling method used to select the primary sampling units, (4) methods to measure the outcome described in sufficient detail to allow reproducibility of the study, and (5) statistical methods sufficiently described and appropriately used. Experimental studies were evaluated using two additional criteria: random allocation of treatment and reported use of blinding. These criteria were used for description purposes and not as exclusion criteria. Two forms, one web-based and one spreadsheet, were used to extract study design parameters (e.g. sampling and laboratory procedures) and outcome data [e.g. odds ratios (OR)] from each relevant study.
International and local collaborators assisted with translation of articles published in non-English (foreign) languages. Foreign-language articles confirmed as relevant were fully translated and reviewed using the same methods as articles published in English. Ten different reviewers conducted relevance screening for this review, while three and two of these reviewers also conducted quality assessment and data extraction, respectively. Two reviewers independently reviewed each abstract or article and disagreements were resolved by group consensus. A copy of the forms used and the list of search terms and combinations are available from the corresponding author upon request.
Statistical analysis
Data were stratified by food-animal species, outcome, sampling point, unit of analysis (e.g. flock vs. individual bird) and diagnostic test [e.g. enzyme-linked immunosorbent assay (ELISA) vs. culture]. Meta-analysis was conducted within each stratum if estimates were available from more than two studies. Crude OR and standard errors were calculated for each study, as none of the included studies provided adjusted estimates of effect, and pooled OR and forest plots were calculated for each analysis using the Mantel–Haenszel method [Reference Sutton16]. Heterogeneity was evaluated using Cochran's Q statistic and I 2 (the percentage of total variation across studies due to heterogeneity) [Reference Higgins17]. Heterogeneity was considered acceptable and pooled OR were reported when P values for the Q statistic were >0·1 [Reference Sutton16]. Pooled OR were considered statistically significant if 95% confidence intervals (CI) excluded the null. Publication bias was investigated using Begg's rank correlation test and Egger's regression test [Reference Sutton16]. All analyses were performed in Stata 10 (Stata Corporation, USA).
RESULTS
Description of studies
The results of the systematic review process are summarized in Figure 1. Ninety-one citations were considered relevant based on the title and abstract; however, after assessment of the full article, 41 studies were excluded for reasons listed in Figure 1. In 12 studies, bacterial or AMR outcomes were investigated only in organic production (i.e. no conventional comparison group was used) and were not assessed for methodological soundness due to their descriptive nature. These studies are summarized in the Appendix (available in the online version of the paper) and are not discussed further.
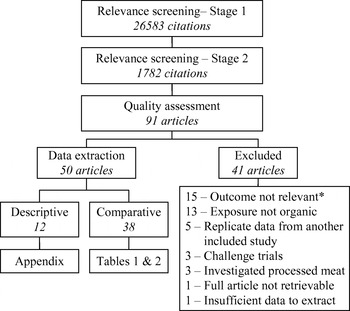
Fig. 1. Systematic review process flow-chart. * These studies did not measure the prevalence of bacterial enteropathogens, potentially zoonotic bacteria or bacterial resistance to antimicrobials in food animals, foods of animal origin or people.
In 37 studies, specific bacterial and AMR outcomes were compared between organic and conventional poultry, swine or beef production. In one additional study, organic food consumption was investigated as a risk factor in human cases of Campylobacter. Thirty-six (94·7%) of the 38 comparative studies were observational studies and two were experimental trials. Thirty-five (97·2%) of the 36 observational studies followed the principles of a cross-sectional design, five of which used longitudinal sampling, and a case-case design was used in the Campylobacter risk-factor study. Studies were published from 1991 to 2008, and 78·9% (30/38) were published between 2005 and 2008.
Sixteen studies published in seven foreign languages (Croatian, Danish, French, German, Italian, Spanish, Swedish) were investigated as potentially relevant, and four of these studies were included in the review [Reference Methner18–Reference Proietti, Passamonti and Asdrubali21].
Bacterial prevalence and mean count data
Bacterial prevalence or count data were reported in 34 studies (Table 1). Five studies investigated the prevalence of Campylobacter spp. in organic and conventional broiler chickens at slaughter in the USA [Reference Luangtongkum22, Reference Lund23], Belgium [Reference Overbeke 24, Reference Tuyttens25] and Denmark [Reference Heuer26]. In three of these studies, a higher prevalence of Campylobacter was reported in organic broiler chickens [Reference Luangtongkum22, Reference Overbeke 24, Reference Heuer26], while Campylobacter was not isolated in one study and no difference in prevalence was observed in another study [Reference Lund23, Reference Tuyttens25]. Meta-analysis was precluded as only graphical data were reported in one study [Reference Overbeke 24] and level of analysis differed between the other two studies (flock vs. individual bird) [Reference Luangtongkum22, Reference Heuer26]. The prevalence of Campylobacter spp. in retail chicken was investigated in four studies conducted in the USA [Reference Cui27–Reference Price29] and UK [Reference Soonthornchaikul30], and a meta-analysis of their prevalence estimates is shown in Figure 2. A low to moderate amount of heterogeneity (I 2=37·3%, P=0·172) and a non-significant pooled OR of 1·33 (95% CI 0·92–1·92) were observed, indicating no significant difference in prevalence between organic and conventional retail chicken.
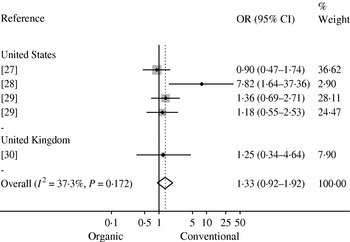
Fig. 2. Mantel–Haenszel meta-analysis of the prevalence of Campylobacter in organic and conventional retail chicken. Studies are stratified by country and estimates of effect are presented as odds ratios (OR) with 95% confidence intervals (CI). The P value refers to the Q statistic test for heterogeneity. Reference 29 was included twice since it provided estimates for two sampling periods (2004 and 2006).
Table 1. Summary of 34 studies comparing the prevalence or mean counts of bacterial enteropathogens and potentially zoonotic bacteria between organic and conventional poultry, swine and beef production
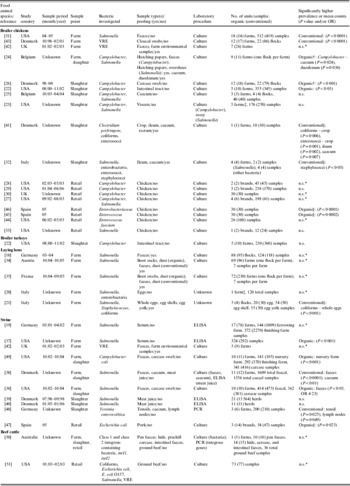
CI, Confidence interval; ELISA, enzyme-linked immunosorbent assay; intI1, class 1 integrase; intI2, class 2 integrase; n.s., no significant difference reported; OR, odds ratio; PCR, polymerase chain reaction; VRE, vancomycin-resistant enterococci.
* No statistical comparisons conducted.
† Flock-level analysis.
‡ Experimental study where the same farm(s) housed both the organic and conventional production groups.
§ Comparison of mean counts of bacteria.
In four studies researchers investigated Salmonella spp. in broiler chickens on farms or at slaughter in the USA [Reference Lund23, Reference Siemon, Bahnson and Gebreyes31], Belgium [Reference Tuyttens25] and Italy [Reference Proietti32], and found very few or no positive samples in both organic and conventional populations. However, a higher prevalence of Salmonella spp. was reported in organic retail chicken in two other studies [Reference Cui27, Reference Izat, Kopek and McGinnis33]. Insufficient data were available for meta-analysis. The prevalence of Salmonella spp. was investigated in laying hens in five studies conducted in Italy [Reference Castellini20, Reference Proietti, Passamonti and Asdrubali21], Austria [Reference Much34], France [Reference Mahe35] and Germany [Reference Methner18], but Salmonella spp. were identified in only three studies [Reference Methner18, Reference Much34, Reference Mahe35] reporting a higher prevalence in conventional laying hen flocks. Meta-analysis of estimates from these studies revealed significant heterogeneity (I 2=79·5%, P=0·008) and a pooled estimate is not reported.
Conflicting results were reported in six studies that examined the prevalence of Salmonella spp. in swine on farms and at slaughter in the USA [Reference Gebreyes, Thakur and Morrow36, Reference Gebreyes37], Denmark [Reference Bonde, Sorensen and Niggli38–Reference Zheng, Bonde and Sorensen40] and Germany [Reference Meyer, Beilage and Krieter19]. Meta-analysis was not conducted in these data subsets due to different diagnostic testing procedures and levels of analysis between these studies (see Table 1).
Several other bacterial outcomes were identified in this review [Reference Castellini20, Reference Proietti, Passamonti and Asdrubali21, Reference Proietti32, Reference Bjerrum41–Reference LeJeune and Christie51] (see Table 1); however, these outcomes were investigated in only a small number of studies, precluding the use of meta-analysis.
Antimicrobial resistance data
Bacterial AMR or multidrug resistance (MDR) data were extracted from 18 studies (Table 2). In seven studies researchers investigated AMR in Campylobacter spp. isolated from broilers chickens at slaughter or retail chicken [Reference Luangtongkum22, Reference Heuer26–Reference Soonthornchaikul30, Reference Sapkota52]. A meta-analysis was conducted on five estimates from four studies [Reference Cui27–Reference Soonthornchaikul30] that examined the prevalence of ciprofloxacin-resistant Campylobacter spp. in organic and conventional retail chicken (Figure 3). No evidence of heterogeneity was observed (I 2=0·0%, P=0·416) and a pooled OR of 9·62 (95% CI 5·67–16·35) was obtained, indicating a higher prevalence of ciprofloxacin-resistant Campylobacter spp. in conventional chicken. Bacterial AMR was also investigated in 11 other studies [Reference Siemon, Bahnson and Gebreyes31, Reference Much34, Reference Gebreyes, Thakur and Morrow36, Reference Kieke44–Reference Miranda47, Reference Thakur and Gebreyes49, Reference LeJeune and Christie51, Reference Bunner53, Reference Nulsen, Mor and Lawton54] (see Table 2). However, a meta-analysis was not attempted due to the limited data available.
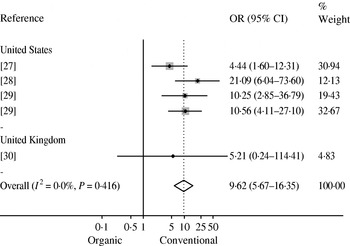
Fig. 3. Mantel–Haenszel meta-analysis of the prevalence of ciprofloxacin-resistant Campylobacter isolated from organic and conventional retail chicken. Studies are stratified by country and estimates of effect are presented as odds ratios (OR) with 95% confidence intervals (CI). The P value refers to the Q statistic test for heterogeneity. Reference 29 was included twice since it provided estimates for two sampling periods (2004 and 2006).
Table 2. Summary of 18 studies comparing the bacterial resistance to antimicrobials between organic and conventional poultry, swine and beef production
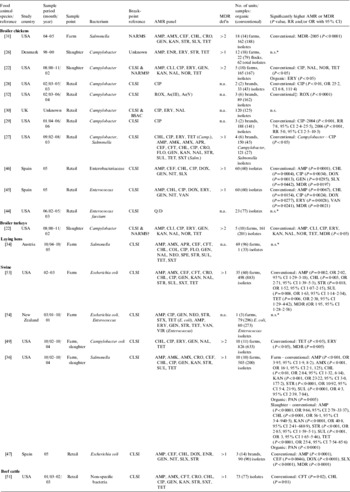
AMP, Ampicillin; AMR, antimicrobial resistance; AMX, amoxicillin; APR, apramycin; BSAC, British Society for Antimicrobial Chemotherapy; CEF, cephalothin; CFT, ceftiofur; CHL, chloramphenicol; CI, confidence interval; CIP, ciprofloxacin; CLI, clindamycin; CLSI, Clinical Laboratory Standards Institute; CRO, ceftriaxone; COL, colistin; DOX, doxycycline; ENR, enrofloxacin; ERY, erythromycin; FLO, florfenicol; GEN, gentamycin; KAN, kanamycin; MDR, multidrug resistance; n.a., not applicable (no multidrug resistance data provided); n.s., no significant difference reported; NAL, naladixic acid; NARMS, National Antimicrobial Resistance Monitoring System; NEO, neomycin; NIT, nitrofurantoin; NOR, norfloxacin; OR, odds ratio; PAN, pansusceptible (susceptible to all antimicrobials tested); Q/D, quinupristin/dalfopristin; RR, relative risk; SPE, spectinomycin; STR, streptomycin; SUL, sulfamethoxazole; SLX, sulfisoxazole; TET, tetracycline; SXT, trimethoprim-sulfamethoxazole; VAN, vancomycin; VIR, virginiamycin.
* No statistical comparisons conducted.
† CLSI break-points were used for norfloxacin only.
‡ Comparison of minimum inhibitory concentrations (MICs).
In 10 studies researchers compared MDR or pansusceptibility (i.e. susceptibility to all antimicrobials tested) in bacterial isolates from organic and conventional broiler chickens or turkeys in the USA [Reference Luangtongkum22, Reference Siemon, Bahnson and Gebreyes31], retail chicken in the USA [Reference Cui27] or Spain [Reference Miranda45, Reference Miranda46], swine in the USA [Reference Gebreyes, Thakur and Morrow36, Reference Thakur and Gebreyes49, Reference Bunner53], retail pork in Spain [Reference Miranda47], or retail ground beef in the USA [Reference LeJeune and Christie51]. In seven studies bacterial isolates from conventional production were found to be significantly more likely to be resistant to multiple antimicrobials [Reference Luangtongkum22, Reference Siemon, Bahnson and Gebreyes31, Reference Miranda45–Reference Miranda47, Reference Thakur and Gebreyes49, Reference Bunner53], and in one study bacterial isolates from organic production were found to be significantly more likely to be pansusceptible [Reference Gebreyes, Thakur and Morrow36]. No significant difference was found in the other two studies [Reference Cui27, Reference LeJeune and Christie51].
Human risk-factor study
UK researchers examined different exposures in household case clusters of Campylobacter jejuni and found that cases who had at least one other family member ill within a week of their onset of symptoms were more likely to have eaten organic meats in the winter (OR 6·86, 95% CI 1·49–31·69) compared to cases who did not have other family members ill within a week of their onset of symptoms [Reference Gillespie55].
Assessment of study methodological soundness
The number of studies that met each of the criteria for methodological soundness is shown in Table 3. Only one study met all five criteria [Reference Gillespie55], and one of the eight studies that did not use statistical methods to compare organic and conventional production met the other four criteria [Reference Mahe35]. Of the two experimental trials [Reference Castellini20, Reference Lund23], only one used a random allocation of treatment and neither reported the use of blinding.
Table 3. Summary of the methodological assessment of 38 comparative studies
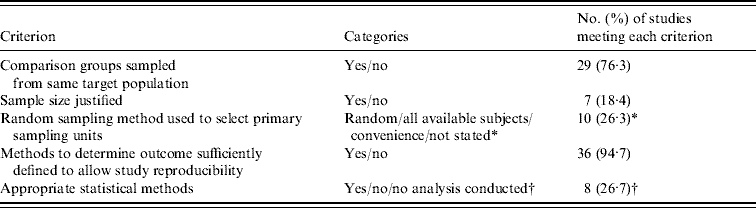
* Random (or systematic) sampling and selection of all available subjects in a database were considered ‘yes’, while convenience sampling or failure to state the sampling method were considered ‘no’.
† Reasons for selecting ‘no’ included failure to adjust for longitudinal or hierarchical clustering or failure to adequately specify statistical methods; n=30 for this criterion since eight studies did not conduct a statistical comparison on data of interest.
DISCUSSION
The largest body of evidence pertained to the investigation of Campylobacter prevalence in organic and conventional broiler chickens at slaughter and retail. While it was previously thought that access to outdoor runs in organic production might result in a higher prevalence of Campylobacter in organic broiler chickens [Reference Bull56], a recent molecular and behavioural longitudinal study of 64 free-range broiler chicken farms in the UK has disputed this claim [Reference Colles57]. In this review, a higher prevalence of Campylobacter was reported in organic broiler chickens at slaughter in three studies [Reference Luangtongkum22, Reference Overbeke 24, Reference Heuer26]. Slower-growing breeds are used in organic production, and differences in average broiler age at slaughter (81 days in organic vs. 40 days in conventional) might, at least partially, explain these findings, as slaughter age is a recognized risk factor for the prevalence of Campylobacter in broiler chicken flocks [Reference Arsenault58]. No discernible differences were noticed among potential sources of heterogeneity, including study country, study quality parameters, sample size and sampling procedures, between these studies and two others [Reference Luangtongkum22, Reference Lund23] in which Campylobacter was either not recovered or no differences between organic and conventional production were observed. In contrast to the findings at slaughter, no difference in the prevalence of Campylobacter was observed in organic and conventional retail chicken. Processing and post-processing conditions can affect the prevalence of Campylobacter on broiler chicken carcases [Reference Berrang59], and it is possible that any difference in prevalence between organic and conventional chickens on farms or at slaughter is altered by these conditions.
The consumption of organic meats was an identified significant risk factor for household case clusters of C. jejuni in one study [Reference Gillespie55]. However, this study compared cases of C. jejuni to other cases, and should be verified by future case-controls studies before the consumption of organic meats is considered a risk factor for this illness.
A higher prevalence of Salmonella in organic retail chicken, reported in only two studies, must be interpreted with caution and clearly indicates that more research in this area is necessary. In laying hens, a higher prevalence of Salmonella was reported in conventional flocks, although significant heterogeneity was observed. All three studies [Reference Methner18, Reference Much34, Reference Mahe35] were conducted in Europe, where organic requirements are similar, and the final sample size for each study was comparable. However, observed heterogeneity might be due to random sampling being utilized in only one study [Reference Mahe35], sample size being justified in only two studies [Reference Much34, Reference Mahe35] or differences in sampling methodology. It should also be noted that each study reported a much higher average flock size on conventional farms, a significant risk factor for Salmonella prevalence in laying hens [Reference Mollenhorst60]. Therefore, it is possible that the higher prevalence observed in conventional flocks in each study may be confounding a true relationship between flock size and prevalence, as no analysis was conducted in these studies to control for this factor.
Differences were observed among the studies investigating the prevalence of Salmonella on organic and conventional swine farms. Geographic location is the most likely source of this heterogeneity as a higher on-farm prevalence of Salmonella in organic pigs was reported in two USA studies [Reference Gebreyes, Thakur and Morrow36, Reference Gebreyes37] while a higher on-farm prevalence in conventional pigs was reported in two studies in Germany and Denmark [Reference Meyer, Beilage and Krieter19, Reference Bonde, Sorensen and Niggli38]. These differences might be due to different management and antimicrobial use practices in conventional and organic production in the USA and Europe [Reference Sorensen61]. Variation in sampling and diagnostic procedures can also lead to differences in the prevalence of Salmonella in swine due to differences in test sensitivity and specificity [Reference Sanchez62]. These factors may also explain some of the observed difference, as an ELISA test was used in two studies (each with a different cut-off value) [Reference Meyer, Beilage and Krieter19, Reference Gebreyes37] and culture in two studies [Reference Gebreyes, Thakur and Morrow36, Reference Bonde, Sorensen and Niggli38]. Study design may be another contributory factor, as sample size was justified in only one study [Reference Meyer, Beilage and Krieter19], random sampling was conducted in only one study [Reference Bonde, Sorensen and Niggli38], and in the three studies where a statistical analysis was conducted [Reference Gebreyes, Thakur and Morrow36–Reference Bonde, Sorensen and Niggli38] methods were used that did not account for hierarchical clustering.
Several studies investigated AMR or MDR in commensal bacteria in broiler chickens, retail chicken, laying hens, swine, pork or ground beef. Commensal bacteria such as Enterococcus spp. can serve as a reservoir for the transfer of resistance genes from bacteria in foods of animal origin to bacteria in humans [Reference Angulo, Nunnery and Bair63], and resistant enterococci such as vancomycin-resistant enterococci (VRE) are a recognized cause of nosocomial infections in humans [Reference Rice64]. Resistance to quinupristin/dalfopristin, one of the treatment options for infections caused by VRE, is of increasing concern given the use of virginiamycin (an analog of quinupristin/dalfopristin) in food-animal production [Reference Angulo, Nunnery and Bair63]. In two studies in this review, higher prevalence of resistance to either virginiamycin or quinupristin/dalfopristin was reported in Enterococcus spp. isolates from conventional rather than organic swine in New Zealand [Reference Nulsen, Mor and Lawton54] and retail chicken in the USA [Reference Kieke44], respectively. However, while the USA study reported E. faecium, the New Zealand study only reported unspeciated enterococci, which could have included E. faecalis, a species known to carry natural resistance to this antimicrobial [Reference Delgado65].
The prevalence of ciprofloxacin-resistant Campylobacter spp. was found to be higher in conventional compared to organic retail chicken (OR 9·62). Ciprofloxacin is a fluoroquinolone that is often used to treat severe human cases of Campylobacter infection [Reference Nelson66]. The World Health Organization has placed fluoroquinolones in the ‘critically important antimicrobial’ category in terms of importance to human medicine [67]. Until 2005, fluoroquinolones were approved for use in the USA commercial broiler chicken industry for the flock-level treatment of infection [Reference McEwen and Fedorka-Cray68, Reference McDermott69]. In the UK, therapeutic use of fluoroquinolones has been approved for use in poultry since 1993 [Reference Taylor70]. As a result of increasing evidence that such practices were contributing to fluoroquinolone-resistant Campylobacter infections in humans, the U.S. Food and Drug Administration proposed a ban on all use of fluoroquinolones in the broiler industry in 2000, which was enacted in 2005 [Reference Nelson66, Reference McEwen and Fedorka-Cray68]. It should be noted that the USA studies included in this review were conducted before or during this time, and if the ban indeed results in reduced levels of resistance in Campylobacter isolates from conventional production, the observed difference would be expected to diminish over time.
In eight studies, significantly higher MDR was reported in bacterial isolates from conventional than organic broiler chickens, retail chicken, swine and pork, which suggests that antimicrobial use practices on conventional farms are more selective of MDR than those on organic farms. However, other management factors that differ between organic and conventional production, such as flock/herd size, type of feed and use of biosecurity practices may also contribute to this difference in selection pressure. Some bacterial isolates from organic production showed resistance to certain antimicrobials, such as ampicillin, erythromycin, streptomycin and tetracycline, in the apparent absence of selection pressure from growth-promoting or prophylactic antimicrobial use. However, some therapeutic use of antimicrobials is permitted in organic production in Europe [10], while in the USA, animals that are treated with antimicrobials cannot be marketed as organic [11]. These environments may provide sufficient selection pressure to retain some level of resistance, albeit at lower prevalence, in bacterial populations. Moreover, it is known that once resistance determinants are acquired by bacterial populations, they may in some instances be retained at the same or reduced prevalence for considerable periods of time, particularly if the encoding resistance genes are linked to other genes for which selection pressure remains [Reference Aarestrup71, Reference Maynard72]. Resistance to chloramphenicol, for example, remains in faecal Escherichia coli populations in pigs and cattle decades after the drug was banned from food-animal production in North America [Reference Maynard72, Reference O'Connor, Poppe and McEwen73]. Co-selection by other antimicrobials still used in food-animal production is believed to be responsible [Reference Maynard72, Reference O'Connor, Poppe and McEwen73]. Similarly, research in Europe has shown that although there was a decline in the prevalence of resistance in bacterial isolates to certain antimicrobials after the discontinued use of antimicrobials for growth promotion in food animals [Reference Aarestrup71, Reference Boerlin74], there was some persistence of resistance observed [Reference Heuer75, Reference Sorum76]. AMR surveillance programmes should target bacterial isolates from organic animal production to better understand AMR in this environment.
This review used a rigorous search strategy to identify all potentially relevant published literature and used a search verification strategy of hand-searching the reference lists of all relevant articles captured by the review. For the meta-analyses presented in this review, both Begg's and Egger's tests revealed no statistical evidence of publication bias. However, these tests suffer from a lack of power in meta-analyses that include only a small number of studies, and the existence of publication bias cannot be ruled out [Reference Sutton16]. Moreover, no attempts were made to identify government or organizational research reports (e.g. AMR surveillance reports) that may not have been indexed in the included databases, nor were attempts made to identify unpublished studies, as unpublished literature is often incomplete and previous attempts to gather additional information from authors have proven to be very time consuming and largely unsuccessful [Reference Sargeant77]. Therefore, it is possible that unpublished studies have been conducted that are not included in this review.
The impact of language bias was reduced by utilizing translators to assist in interpreting relevant foreign-language articles, and this provided a more complete assessment of the currently available research in two European countries (Germany and Italy). The studies provided enough additional data to allow a meta-analysis of the prevalence of Salmonella in laying hens, demonstrating the advantage of including foreign-language studies in systematic reviews and meta-analyses, especially when data are scarce. Although article translation requires significant time and resources, the use of international collaborative reviewers might be an efficient way to overcome these obstacles.
Most studies included in this review were observational cross-sectional studies, which can only provide evidence for an association and cannot establish causation due to a lack of temporal evidence. In addition, cross-sectional studies are subject to selection, misclassification and confounding bias, which can lead to distorted results and conclusions [Reference Dohoo, Martin and Stryhn78]. Furthermore, many of the studies were based on a small sample size, and their purpose is most suitable for generating rather than testing hypotheses. They are a valuable source of initial evidence on new and emerging topics but do not provide high-quality evidence for systematic reviews or meta-analyses.
The assessment of study methodological soundness revealed that most studies did not explicitly justify their sample size, and to a lesser extent, state whether a formal random sampling method was used to select the primary sampling units. In addition, many of the studies sampled animals or food products from multiple herds, farms or retail locations and used statistical methods that did not account for the hierarchical structure of the data. The lack of adjustment for clustering might have resulted in underestimated standard errors, increasing the chance of rejecting the null hypothesis when it is true [Reference Dohoo, Martin and Stryhn78].
Within most strata there were too few studies with sufficient data for a meta-analysis or significant heterogeneity was identified. The exceptions were the prevalence of Campylobacter and ciprofloxacin-resistant Campylobacter in organic and conventional retail chicken. However, these estimates should be interpreted with the aforementioned caveats in mind, as calculations were based on crude OR calculated post hoc from a small number of studies, and without adjustment for potential confounders or clustering. The fixed-effect Mantel–Hanszel method was chosen over the random-effects model since no significant heterogeneity was identified, and a sensitivity analysis showed little difference between results from both methods. Meta-regression is one approach that can be used to investigate potential sources of between-study heterogeneity [Reference Sutton16]; however, the use of this method in this review was prohibited due to the small number of studies. Once more primary research of sufficient quality is generated, the precision of the pooled estimates presented in this paper can be improved and potential sources of heterogeneity such as study country, study quality parameters and laboratory procedures can be further investigated in meta-regression models.
CONCLUSION
Meta-analysis revealed no difference in the prevalence of Campylobacter in organic and conventional retail chicken, while Campylobacter isolates from conventional retail chicken were significantly more likely to be ciprofloxacin-resistant. Limited or inconsistent research was identified in studies examining the prevalence of bacterial enteropathogens and potentially zoonotic bacteria in other food-animal species. Bacterial isolates from conventional broiler chicken, turkey and swine production exhibited more AMR and MDR than isolates from organic production; however, the presence of some resistant strains in organic animal production was also identified. There is a need for future research of sufficient quality in this area, so that more accurate pooled estimates can be generated and potential sources of heterogeneity can be investigated using more advanced techniques such as meta-regression.
ACKNOWLEDGEMENTS
The authors thank Janet Harris for developing search terms, conducting the original literature search and relevance screening, Vi Nguyen for procuring articles, conducting one of the updated searches and relevance screening, Judy Grieg, Karen Robertson and Ashley Farrar for relevance screening, Claudia Schmidt, Natalia Cernicchiaro Martinez, Mats Lindblad, Juliana Ruzante and Mette Cornelisse for their assistance with translation of foreign articles, and the Laboratory for Foodborne Zoonoses, Public Health Agency of Canada, National Collaborating Centre for Environmental Health, Saskatchewan Agriculture and Ontario Graduate Scholarship Program for providing funding for this project.
NOTE
Supplementary material accompanies this paper on the Journal's website (http://journals.cambridge.org/hyg).
DECLARATION OF INTEREST
None.