Introduction
Aphasia is an acquired communication condition that affects a person's language modalities and functions due to a focal or diffuse brain lesion (Papathanasiou & Coppens, Reference Papathanasiou and Coppens2021). Currently, there are approximately 2.5 million people in the United States who live with aphasia (Simmons-Mackie, Reference Simmons-Mackie2018). According to the 2011 US Census report, 21% of the US population speaks a language other than English at home (Ryan, Reference Ryan2011) and the number of individuals with low English proficiency increased by 80% from 1990 to 2010 (Simmons-Mackie, Reference Simmons-Mackie2018); however, most aphasia therapies are still based on monolingual English speakers. Taken together, these facts point to a pressing need for aphasia therapies that can accommodate diverse cultural and linguistic backgrounds.
Recently, there has been a push to investigate whether evidence-based anomic treatments designed for monolingual persons with aphasia (MPWA) may be adapted to effectively improve both the trained and untrained language in bilingual persons with aphasia (BPWA). This has been achieved with semantically based naming treatments across a variety of language combinations (Edmonds & Kiran, Reference Edmonds and Kiran2006; Kiran & Iakupova, Reference Kiran and Iakupova2011; Kiran & Roberts, Reference Kiran and Roberts2010; Knoph et al., Reference Knoph, Lind and Simonsen2015) and has been extended to verb retrieval using Verb Network Strengthening Treatment (VNeST) (Lerman, Reference Lerman2020; Lerman et al., Reference Lerman, Goral and Edmonds2019; Li et al., Reference Li, Li and Kiran2021).
A relatively new semantically based naming treatment that has recently been adapted for BPWA (Sandberg et al., Reference Sandberg, Zacharewicz and Gray2021) is Abstract Semantic Associative Network Training (AbSANT; Sandberg & Gray, Reference Sandberg and Gray2020). AbSANT studies have shown that training abstract words results in direct training effects and generalization to concrete words in the trained category, whereas training concrete words only results in direct training effects (Kiran et al., Reference Kiran, Sandberg and Abbott2009; Sandberg & Gray, Reference Sandberg and Gray2020). A few theoretical concepts are driving these outcomes. First, AbSANT capitalizes on the theory underlying the effectiveness of semantic feature analysis (SFA); namely, that discussing semantic features of a target concept facilitates spreading activation to the lexical form for that concept as well as semantically related concepts, thus strengthening the semantic network (Boyle & Coelho, Reference Boyle and Coelho1995; Collins & Loftus, Reference Collins and Loftus1975). Although SFA utilizes feature generation, AbSANT utilizes feature selection and verification (except during the first brainstorm session in which features are generated – see treatment protocol for details) to promote spreading activation and semantic strengthening. This is because of the differences in semantic features for abstract versus concrete words. While concrete words (e.g., pepper) have semantic features that fit nicely into semantic feature categories like use (e.g., is eaten) and physical properties (e.g., is red), semantic features for abstract words (e.g., noisy) rarely fit into SFA feature categories and even with adapted feature categories (e.g., how does it make you feel?), it is difficult to generate semantic features for abstract words due to their lack of categorical structure and sensory/perceptual features (Jones, Reference Jones1985). AbSANT also capitalizes on the complexity account of treatment efficacy (CATE; Thompson et al., Reference Thompson, Shapiro, Kiran and Sobecks2003), which posits that training more complex items promotes generalization to items that are related, but less complex. In this case, abstract words are the more complex items and concrete words are the related, less complex items. This difference in complexity can be described in a variety of ways, but the Normal Isolated Centrally Expressed theory (NICE; Newton & Barry, Reference Newton and Barry1997) captures aspects relevant to how spreading activation (an important component in the mechanism of semantically based word retrieval therapy) differs between abstract and concrete words; namely, that abstract words are weakly connected to a variety of concepts, while concrete words have strong connections to specific somatosensory experience, visual characteristics, location, etc. For a more in-depth discussion of the theoretical underpinnings of AbSANT, see Sandberg and Kiran (Reference Sandberg and Kiran2014) and Sandberg and Gray (Reference Sandberg and Gray2020).
While the majority of the studies of the efficacy of AbSANT have been focused on MPWA (Kiran et al., Reference Kiran, Sandberg and Abbott2009; Sandberg & Gray, Reference Sandberg and Gray2020; Sandberg & Kiran, Reference Sandberg and Kiran2014), recent work has extended AbSANT to bilingual individuals, resulting in BAbSANT: Bilingual Abstract Semantic Associative Network Training (Sandberg et al., Reference Sandberg, Zacharewicz and Gray2021). In this recent study, the AbSANT protocol was adapted for use in a Polish–English BPWA, who showed cross-language generalization, but in an unexpected way (which will be discussed below). The authors hypothesized an interaction between language dominance and language control. In the present study, we explore the efficacy of BAbSANT for a Russian–English BPWA, focusing specifically on language dominance and language control.
Within the past 15 years, there has been a concerted effort to better understand bilingual treatment outcomes and how cross-language generalization may be achieved. The following factors have been investigated and shown to affect cross-language generalization: age of acquisition (Goral et al., Reference Goral, Levy, Obler and Cohen2006; Kiran et al., Reference Kiran, Sandberg, Gray, Ascenso and Kester2013; Knoph et al., Reference Knoph, Lind and Simonsen2015, Reference Knoph, Simonsen and Lind2017), language dominance (Edmonds & Kiran, Reference Edmonds and Kiran2006; Kiran & Roberts, Reference Kiran and Roberts2010; Knoph, Reference Knoph2013; Kurland & Falcon, Reference Kurland and Falcon2011; Miller Amberber, Reference Miller Amberber2012), language similarity (Conner et al., Reference Conner, Goral, Anema, Borodkin, Haendler, Knoph, Mustelier, Paluska, Melnikova and Moeyaert2018; Goral et al., Reference Goral, Levy and Kastl2010; Knoph, Reference Knoph2013) and inhibitory control (Goral et al., Reference Goral, Naghibolhosseini and Conner2013; Keane & Kiran, Reference Keane and Kiran2015; Kiran et al., Reference Kiran, Sandberg, Gray, Ascenso and Kester2013; Sandberg et al., Reference Sandberg, Zacharewicz and Gray2021). It is challenging to identify the critical factors influencing cross-language generalization because they are dynamic, and combinations vary across BPWA. It is understood that two (or more) languages are simultaneously active in the bilingual brain (Ehri & Ryan, Reference Ehri and Ryan1980; Green, Reference Green1986, Reference Green1998). Further, it is accepted that two (or more) languages have bidirectional connections and that, based on proficiency, word knowledge flows between the semantic system and the two languages, with a stronger directional flow going from the less proficient language to the more proficient language (de Groot, Reference de Groot1992; Kroll et al., Reference Kroll, Van Hell, Tokowicz and Green2010; Kroll & Stewart, Reference Kroll and Stewart1994; Rice & Tokowicz, Reference Rice and Tokowicz2020). Based on these theoretical constructs, it is hypothesized that activation of the target language also activates the nontarget language, such that cross-language generalization may be observed as an effect of treatment in bilingual aphasia. When the client's languages are unbalanced, cross-language generalization is hypothesized to be stronger when the non-dominant language is trained because of the relative reliance of the non-dominant language on the dominant language for access to the semantic system (Kroll & Stewart, Reference Kroll and Stewart1994).
While some studies have shown that treating the non-dominant language results not only in improvement to the trained language, but also cross-language generalization to the dominant language (Edmonds & Kiran, Reference Edmonds and Kiran2006; Goral et al., Reference Goral, Rosas, Conner, Maul and Obler2012; Kiran & Iakupova, Reference Kiran and Iakupova2011; Kiran & Roberts, Reference Kiran and Roberts2010; Knoph, Reference Knoph2013; Kurland & Falcon, Reference Kurland and Falcon2011), other studies have shown cross-language generalization to the non-dominant language when the dominant language is trained (Goral, Reference Goral, Gitterman, Goral and Obler2012; Sandberg et al., Reference Sandberg, Zacharewicz and Gray2021), while still others have shown inconsistent results or a lack of cross-language generalization (Abutalebi et al., Reference Abutalebi, Rosa, Tettamanti, Green and Cappa2009; Croft et al., Reference Croft, Marshall, Pring and Hardwick2010; Kiran et al., Reference Kiran, Sandberg, Gray, Ascenso and Kester2013; Meinzer et al., Reference Meinzer, Obleser, Flaisch, Eulitz and Rockstroh2007; Miller Amberber, Reference Miller Amberber2012). There remains a critical need to better understand generalization based on language dominance.
Bilingual language control has also been considered when examining the efficaciousness of bilingual aphasia treatments and cross-language generalization. Various models have been proposed to account for bilingual language control; however, we will focus on the most prominent model of language control, the Inhibitory Control model (IC; Green, Reference Green1998). This model proposes that in order to access the target language, the nontarget language is inhibited via language task schemas, reducing the level of activation of all words in the non-target language (for alternate views of bilingual language control, see Blanco-Elorrieta & Caramazza, Reference Blanco-Elorrieta and Caramazza2021; Costa & Caramazza, Reference Costa and Caramazza1999). The IC model suggests a delicate balance between inhibition and activation so that lexical items in the target language are activated and retrieved, avoiding cross-language intrusions.
Specific to bilingual aphasia, some studies have suggested that a lack of cross-language generalization or the emergence of cross-language intrusion errors in response to intervention may be related to impairments in nonverbal cognitive control (Abutalebi et al., Reference Abutalebi, Rosa, Tettamanti, Green and Cappa2009; Ansaldo et al., Reference Ansaldo, Saidi and Ruiz2010; Keane & Kiran, Reference Keane and Kiran2015; Radman et al., Reference Radman, Mouthon, Di Pietro, Gaytanidis, Leemann, Abutalebi and Annoni2016; Sandberg et al., Reference Sandberg, Zacharewicz and Gray2021). Keane and Kiran (Reference Keane and Kiran2015) investigated within- and cross-language generalization in an Amharic, English, and French trilingual individual with aphasia who presented with nonverbal cognitive control impairment. A semantic based treatment was delivered in French (phase 1) and English (phase 2). For both phases, results revealed positive effects of direct training coupled with intrusion errors from the trained language to the untrained language. The authors suggested that because this person's control network (Abutalebi & Green, Reference Abutalebi and Green2007) was disrupted, optimal inhibition required to suppress competing cross-language activation did not occur.
In another study that investigated the nature of within- and cross-language generalization in bilingual aphasia, Kiran and colleagues (Reference Kiran, Sandberg, Gray, Ascenso and Kester2013) examined the performance of 17 Spanish–English bilingual adults with aphasia who completed a semantic-based naming intervention. Optimal treatment response included within- and cross-language generalization to words that were semantically related to trained words and direct translations of trained words. Although three of the 17 participants exhibited optimal treatment response, others showed various combinations of within- and cross-language generalization to semantically related and/or direct translations, and one participant showed no generalization. Based on these patterns of generalization and participant profiles, the authors proposed an “integrative framework” that accounts for two mechanisms essential to achieving within- and cross-language generalization: spreading activation and inhibitory control. Spreading activation is responsible for increased activation of target words and semantically related words. In order for spreading activation to occur, the activation and suppression of appropriate features must occur and this relies on inhibitory control (Schnur et al., Reference Schnur, Schwartz, Brecher and Hodgson2006). Inhibitory control is also associated with the management of two languages (aka bilingual language control; Green, Reference Green1998). As discussed above, to access the target language, the nontarget language must be suppressed. These mechanisms of spreading activation and inhibitory control must be functioning properly to enable appropriate facilitation and inhibition needed for within- and cross-language generalization.
In the recent Sandberg et al. (Reference Sandberg, Zacharewicz and Gray2021) BAbSANT study, a Polish–English BPWA completed one 10-week phase of Polish (dominant language) treatment and one 10-week phase of English (non-dominant language) treatment. In both phases, abstract words were trained. The participant also completed a test of nonverbal cognitive control. It was hypothesized that in both phases, the participant would show direct training effects, within-language generalization (improved naming for concrete words in the trained language) and that when being trained in his non-dominant language, cross-language generalization would be observed. Results revealed effects of direct training and within-language generalization, but cross-language generalization was only observed when the dominant language was trained. It was suggested that the participant's poor nonverbal cognitive control may have played a role in the unexpected cross-language generalization outcome. Cross-language generalization requires spreading activation and some elements of bilingual language control (which is hypothesized to rely on inhibitory control). For this participant, these two key components may have been imbalanced, reversing the expected dominance result. Specific to the revised hierarchical model (Kroll & Stewart, Reference Kroll and Stewart1994), his impaired nonverbal cognitive control may have disrupted the way information flowed along the lexical connections between the first language (L1) and the second language (L2).
Taken together, these bilingual aphasia treatment studies propose a role for not only spreading activation, but also nonverbal cognitive control in treatment outcomes, specifically regarding the effects of cross-language generalization (Keane & Kiran, Reference Keane and Kiran2015; Kiran et al., Reference Kiran, Sandberg, Gray, Ascenso and Kester2013; Sandberg et al., Reference Sandberg, Zacharewicz and Gray2021). It is reasonable to assume that the cross-language flow of activation suggested by the models like the revised hierarchical model (Kroll & Stewart, Reference Kroll and Stewart1994), which were developed based on the behavior of neurologically intact individuals, depend on intact nonverbal cognitive control. Therefore, any hypotheses of cross-language generalization in aphasia treatment based on such models – for example, the hypothesis that training the non-dominant language will promote cross-language generalization, but not vice versa – assume intact nonverbal cognitive control of the trained individual. However, we know that nonverbal cognitive control can be impaired in aphasia (Faroqi-Shah et al., Reference Faroqi-Shah, Sampson, Pranger and Baughman2018; Green et al., Reference Green, Ruffle, Grogan, Ali, Ramsden, Schofield, Leff, Crinion and Price2011). Thus, we measure and account for the effects of cognitive control in this study and propose that this should be standard practice in studies examining cross-language generalization.
The purpose of the current study is to further explore the efficacy of BAbSANT, by expanding the treatment protocol to a Russian-dominant, Russian–English BPWA, and to specifically examine the role of cognitive control in treatment outcomes. Figure 1a illustrates the theoretical bases for the hypothesized outcomes. Following the logic of the revised hierarchical model, the lexicons for each language should be connected to a shared semantic system. The dominant lexicon should be larger than the non-dominant lexicon and should have stronger connections to the semantic system. There should be stronger connections to the dominant lexicon from the non-dominant lexicon than vice versa. As mentioned previously, this imbalance in connection strength creates a relative reliance of the non-dominant language on the dominant language for accessing the semantic system. Additionally, based on the spreading activation theory (Collins & Loftus, Reference Collins and Loftus1975), the NICE model (Newton & Barry, Reference Newton and Barry1997), and the CATE model (Thompson et al., Reference Thompson, Shapiro, Kiran and Sobecks2003), activation is more likely to spread from abstract to concrete concepts within the semantic system than vice versa. Note that because this is a semantically based treatment, we are not considering any phonologically based spreading activation that may be occurring within each lexicon.
1. Based on previous work showing generalization from abstract to concrete words within a single language in MPWA (Kiran et al., Reference Kiran, Sandberg and Abbott2009; Sandberg & Gray, Reference Sandberg and Gray2020; Sandberg & Kiran, Reference Sandberg and Kiran2014), we hypothesized that training abstract words (e.g., love) in a category (e.g., wedding) will result in a) direct training effects and b) within-language generalization to concrete words (e.g., flowers) in the same category, regardless of the trained language.
2. Based on previous work showing stronger cross-language generalization to the dominant language when training the non-dominant language in unbalanced BPWA (Edmonds & Kiran, Reference Edmonds and Kiran2006; Kiran et al., Reference Kiran, Sandberg, Gray, Ascenso and Kester2013), we hypothesized that training abstract words (e.g., health) in the non-dominant language (English) of our unbalanced Russian–English BPWA will promote cross-language generalization to abstract translated items (e.g., здоровье [health]) and concrete words (e.g., скорая помощь [ambulance]) in the same category in the untrained, dominant language (Russian). We do not expect similar cross-language generalization effects when the dominant language is trained. These hypotheses are based on the revised hierarchical model which assumes intact cognitive control.
3. We expect the dominance effect of cross-language generalization to be modulated by cognitive control. As in previous work (Abutalebi et al., Reference Abutalebi, Rosa, Tettamanti, Green and Cappa2009; Ansaldo et al., Reference Ansaldo, Saidi and Ruiz2010; Keane & Kiran, Reference Keane and Kiran2015), we hypothesize that intact nonverbal cognitive control will support the expected cross-language generalization pattern for our unbalanced Russian–English BPWA. If we observe deficits in nonverbal cognitive control, we may observe no cross-language generalization (as noted in Keane & Kiran, Reference Keane and Kiran2015) or only cross-language generalization when the dominant language is trained (as noted in Sandberg et al., Reference Sandberg, Zacharewicz and Gray2021).
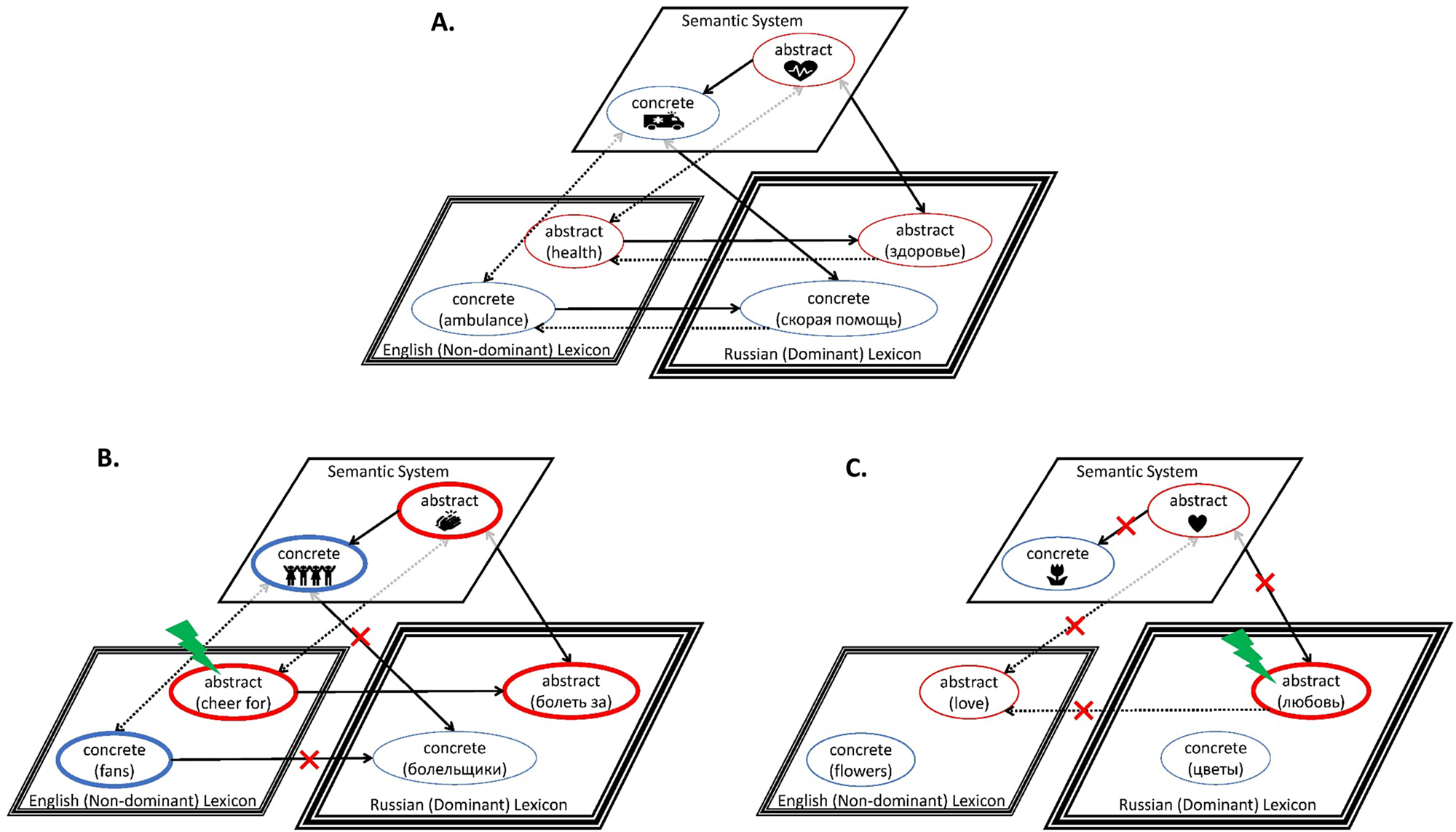
Figure 1. Illustration of the treatment effects in phase B (panel B) and phase C (panel C) based on proposed mechanisms of the spread of activation within the semantic system, between lexicons, and between the semantic system and lexicons for each language (panel A).
Note: The size of the box indicates the size of the lexicon. The border style around lexicons indicates parallel activation. The size of the border indicates the relative strength of language activation. Solid arrows represent stronger links, dotted arrows represent weaker links. The arrow direction indicates direction of spreading activation. In panels B and C, the lightning bolt represents training. The thickened circle lines represent activation of a lexical or semantic entry. A red x indicates the lack of spreading activation in a pathway.
Methods
Participant
The participant, referred to hereafter as “Dmitri,” is a Russian immigrant, who moved to the United States at the age of 40 with his wife and son. Dmitri experienced a left-sided cerebrovascular accident at the age of 56. At the time of injury, Dmitri was diagnosed with global aphasia and oral and verbal apraxia of speech. He received inpatient speech and language services and some outpatient services when discharged. At the time of the current study, Dmitri was 68 years old and 150 months post-stroke. He presented with Broca's aphasia and apraxia of speech in Russian and English. It was also determined that Dmitri is right-handed, and although not formally assessed, his hearing was not a concern at the time of the treatment. Dmitri gave consent to participate in this study and all procedures were approved by the San Francisco State University IRB.
Language profile
The Language Use Questionnaire (LUQ; Kastenbaum et al., Reference Kastenbaum, Bedore, Peña, Sheng, Mavis, Sebastian-Vaytadden, Rangamani, Vallila-Rohter and Kiran2019) was administered to determine Dmitri's language use and exposure across his lifetime (see Table 1a). His wife assisted with responses. The LUQ consists of specific questions regarding L1 and L2 abilities pertaining to Age of Acquisition; Daily Exposure, which captures L1 and L2 heard and spoken throughout Dmitri's pre-stroke and post-stroke weekday and weekend routines; Lifetime Exposure, which is indexed by age increments, averaged across speaking, reading, and writing and expressed as a ratio between L1 and L2; Confidence, which is indexed by age increments and averaged across speaking, reading, and writing for L1 and L2; Family Proficiency, which identifies language proficiency for Dmitri's immediate family (parents and siblings only), is presented as an average for L1 and L2; Education History, which accounts for language of instruction and preferred language in elementary school, high school and university and is presented as a ratio between L1 and L2; and Pre- and Post-stroke Language Ability Rating (LAR), which is a self-assessment of language skill that captures reading, writing, listening, and speaking skills across formal and informal environments. The LAR is obtained using a 1-5 Likert scale (1 represents language skill at the word level and 5 represents native like fluency) and expressed as an average for L1 and L2. In order to identify a bilingual individual's post-stroke language loss profile (e.g., parallel, differential, etc.), it is imperative to know their pre-stroke language dominance, and while past language ability cannot be measured objectively in the present, the LAR has been identified as a reliable measure of pre-stroke language dominance (Gray & Kiran, Reference Gray and Kiran2013; Reference Gray and Kiran2015; Kiran et al., Reference Kiran, Balachandran and Lucas2014; Peñaloza et al., Reference Peñaloza, Barrett and Kiran2020).
Table 1ab. Language Use Questionnaire (LUQ) Data and Diagnostic Data
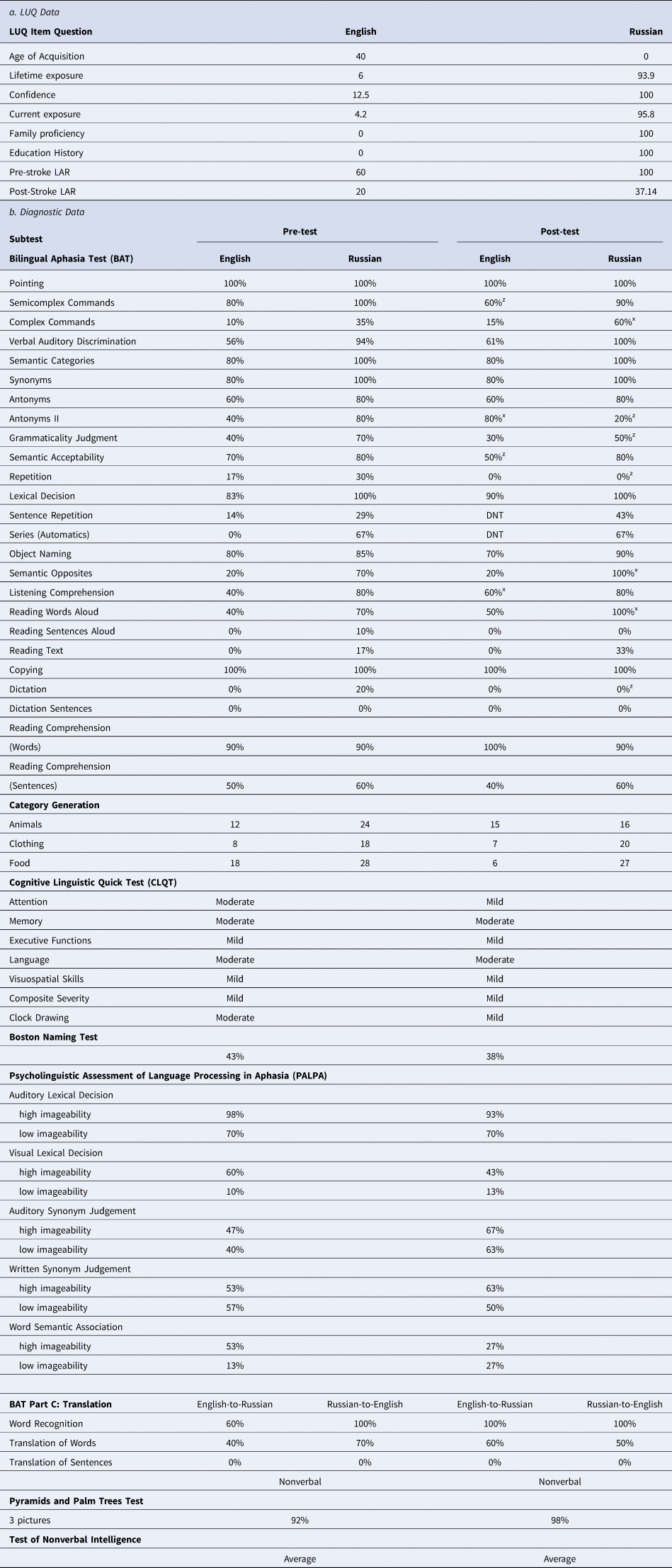
Note: Lifetime exposure and Confidence are indexed by age increments and averaged across speaking, reading, and writing for English and Russian. Language Ability Rating (LAR) accounts for reading, writing, listening and speaking in formal and informal contexts. It is presented as an average based on a 1-5 Likert rating scale (“1” is non-fluent and “5” is fluent). x = at least a 20% increase from pre- to post-treatment assessment. z = at least a 20% decrease from pre- to post-treatment assessment.
Dmitri was born in Russia to monolingual Russian-speaking parents. He acquired all of his education in Russian, including elementary school, middle school, high school, bachelor's degree, and master's degree, and worked as an engineer in the Soviet Union/Russia. Dmitri was not exposed to English until moving to the United States at the age of 40. After relocating to the United States, Dmitri started taking English classes and eventually worked at a maintenance firm. While performing his duties as a maintenance worker, Dmitri used English at work, but he spoke Russian at home with his family and during family and community events. Thus, his overall exposure to English was limited but his confidence to speak, read and write English increased over time. After his stroke, Dmitri discontinued his duties as a maintenance worker due to physical and communication challenges, dedicating his time to improving his speech and physical well-being. In his post-stroke lifestyle, he primarily uses Russian throughout the week, and practices English when he attends communication classes at local community groups. The LAR shows that Russian was Dmitri's pre- and post-stroke dominant language and that he lost language skill in both English and Russian as a consequence of his stroke.
Diagnostic assessment
A battery of standardized and non-standardized assessments was administered to assess Dmitri's language and cognitive skills before and after treatment. The Bilingual Aphasia Test (BAT; https://www.mcgill.ca/linguistics/research/bat) and category generation (animals, food, clothing) task were administered in both Russian and English to compare Dmitri's post-stroke language ability in each language. In line with the participant's report of Russian language dominance, Dmitri scored higher in Russian compared to English on the BAT and category generation tasks, indicative of a parallel language loss profile. According to the BAT and considering pre-stroke language proficiency, Dmitri presented with moderate English and Russian language loss.
Some testing could only be administered in English, due to unavailability of these tests in Russian. The Cognitive Linguistic Quick Test (CLQT; Helm-Estabrooks, Reference Helm-Estabrooks2001) assesses language and cognitive functioning. Dmitri presented with mild impairment on executive functions, visuospatial skills, and the composite severity. He presented with moderate impairment on attention, memory, language, and clock drawing subtests. CLQT cognitive testing requires some language; therefore, these results may be confounded by Dmitri's English proficiency. The Boston Naming Test (BNT; Kaplan et al., Reference Kaplan, Goodglass, Weintraub, Segal and Loon-Vervoorn2001) measures confrontational naming ability at the word level. Dmitri exhibited impaired naming. Selected subtests from the Psycholinguistic Assessment of Language Processing in Aphasia (PALPA; Kay et al., Reference Kay, Lesser and Coltheart1992) were administered to assess Dmitri's ability to process abstract and concrete words using lexical decision and synonym judgement tasks. Overall, Dmitri scored higher on high imageability words compared to low imageability words, with the exception of pre-treatment written synonym judgements (< 5% difference) and post-treatment word semantic association where there was no difference.
Two tests were language-free. The Test of Nonverbal Intelligence (TONI; Brown et al., Reference Brown, Sherbenou and Johnsen2010) is a nonverbal intelligence test that requires reasoning strategies to solve problems presented as novel abstract figures. Based on TONI results, Dmitri exhibited average intelligence. While the TONI does not directly assess attention and memory, note that this language-free test resulted in an average score, rather than suggesting a mild-moderate deficit as did the English-dependent CLQT. The Pyramids and Palm Trees Test Picture Version, 3-picture version (PPT; Howard & Patterson, Reference Howard and Patterson1992) nonverbally assesses the integrity of the semantic system. Dmitri performed within normal limits suggestive of intact nonverbal semantic processing, despite potential cultural differences in the picturable stimuli. See Table 1b for diagnostic scores.
In addition to formal and informal diagnostic testing, two nonverbal cognitive control tasks were administered: an inhibitory control task and a task-switching task. While both tasks measure resistance to distractor interference (e.g., the ability to suppress and overcome interfering stimuli), the task-switching task measures sustained control mechanisms (e.g., conflict and response monitoring) in the form of mixing costs and transient control mechanisms (e.g., resolving conflict between competing tasks) in the form of switch costs (Braver et al., Reference Braver, Reynolds and Donaldson2003). Resistance to distractor interference closely aligns with language control: in order to access the target language, the non-target language must be inhibited, and speakers must switch between languages. Both nonverbal cognitive control tasks used in the current study are well-established (Calabria et al., Reference Calabria, Hernández, Branzi and Costa2012, Reference Calabria, Branzi, Marne, Hernández and Costa2015; Gray, Reference Gray2020; Gray & Kiran, Reference Gray and Kiran2019; Peng & Gray, Reference Peng and Gray2021) and were administered on ePrime (Psychology Toolkit; E-Prime, 2012).
In the inhibitory control task, participants are presented with three shapes (circle, triangle, square) that vary by color (red, blue, green). A cue instructs participants to match two of the three shapes by color or shape, requiring the inhibition of the non-target matching dimension (e.g., shape if the cue is color). On congruent trials, stimuli are mapped within univalent constructs (e.g., all stimuli are the same color if shape is the target matching dimension), whereas incongruent stimuli are mapped within bivalent constructs (e.g., color needs to be inhibited if shape is the target matching dimension). Therefore, compared to congruent trials, incongruent trials require more effort to inhibit nontarget stimuli and are expected to evoke lower accuracy and/or slower response times. A total of 160 trials (80 congruent and 80 incongruent) were presented across 5 blocks (32 trials each) and pseudorandomized to ensure that repeat trials never exceeded five consecutive trials.
The nonverbal task-switching task comprises the incongruent stimuli from the previously described inhibitory control task and was constructed using a sandwich design: two single blocks (one matching by color and one matching by shape), followed by three mixed blocks that include matching by color and shape (comprised of “repeat” and “switch” trials), concluding with two single blocks (one matching by color and one matching by shape). It is expected that switch costs (repeat trials are faster and/or more accurate than switch trials) and mixing costs (trials in the single blocks are faster and/or more accurate than repeat trials in the mixed blocks) will be observed. In the task-switching task, each single block included 18 trials, and each mixed block included 24 incongruent color and 24 incongruent shape trials. In mixed blocks, repeat trials never exceeded five consecutive trials. Due to technical problems, data from the third mixed block were not included in the analysis.
Results from the two nonverbal cognitive control tasks revealed that Dmitri exhibited the hypothesized effects of suppressing and overcoming interfering stimuli. Specifically, on the inhibitory control task, Dmitri was faster and more accurate on congruent conditions compared to incongruent conditions. On the task-switching task, Dmitri's results were indicative of intact sustained control mechanisms (aka mixing costs); however, evidence of intact transient control mechanisms (aka switch costs) was not observed. See Figure 2ab for stimuli design and Table 2ab for results.
Table 2abcd. Nonverbal Cognitive Control Tasks, Effect Size Interpretations and Effect Size Results
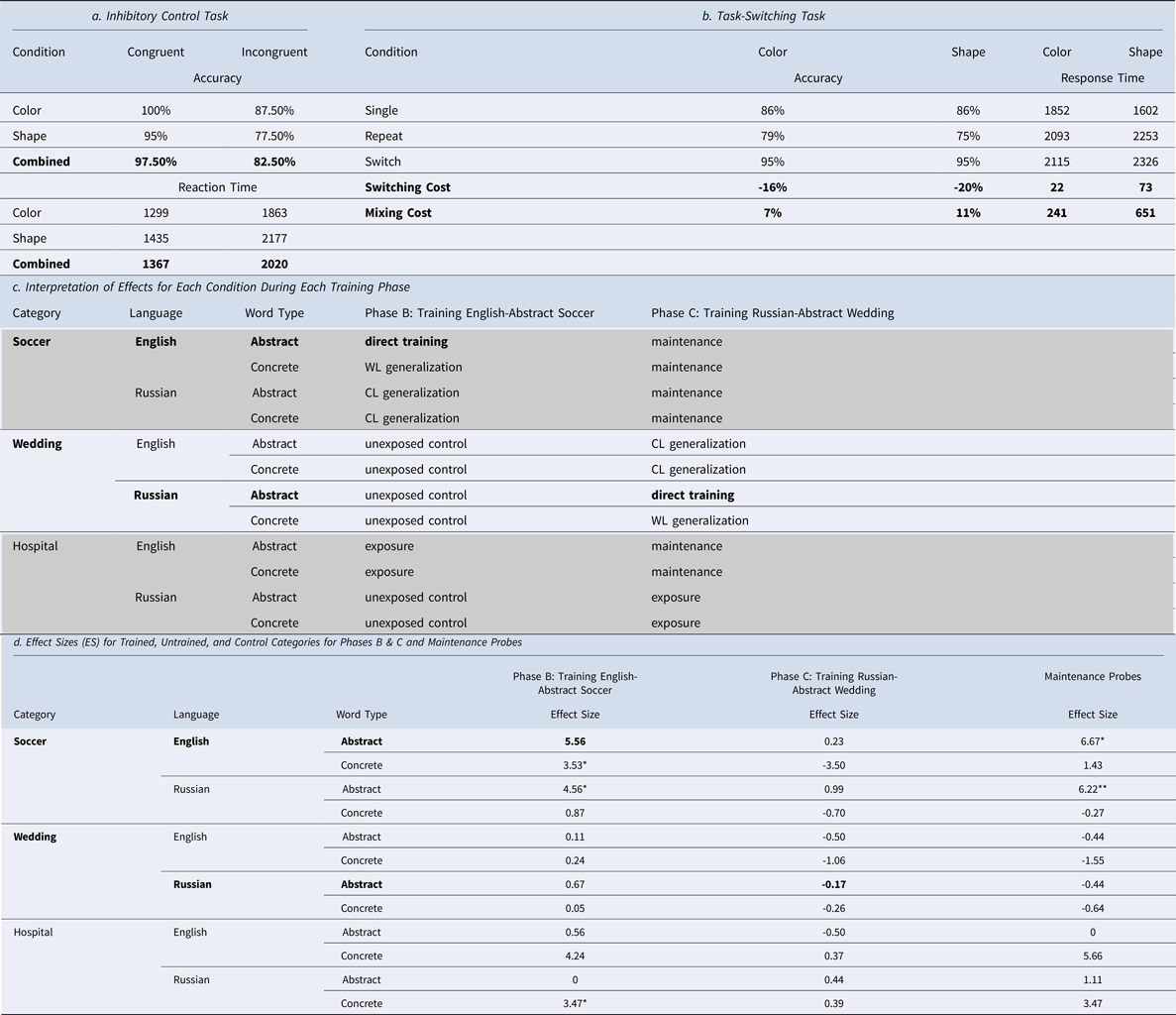
Note. CL = cross-language, WL = within-language; Asterisks indicate strength of direct training and generalization effect sizes (ES) based on Beeson and Robey (2008); *** = large, ** = medium, * = small. Direct training: 9.5 = large, 8 = medium, 6.5 = small; Generalization: 8 = large, 5 = medium, 2 = small. Direct training indicated in bold. For exposure effects, direct training guidelines are used.
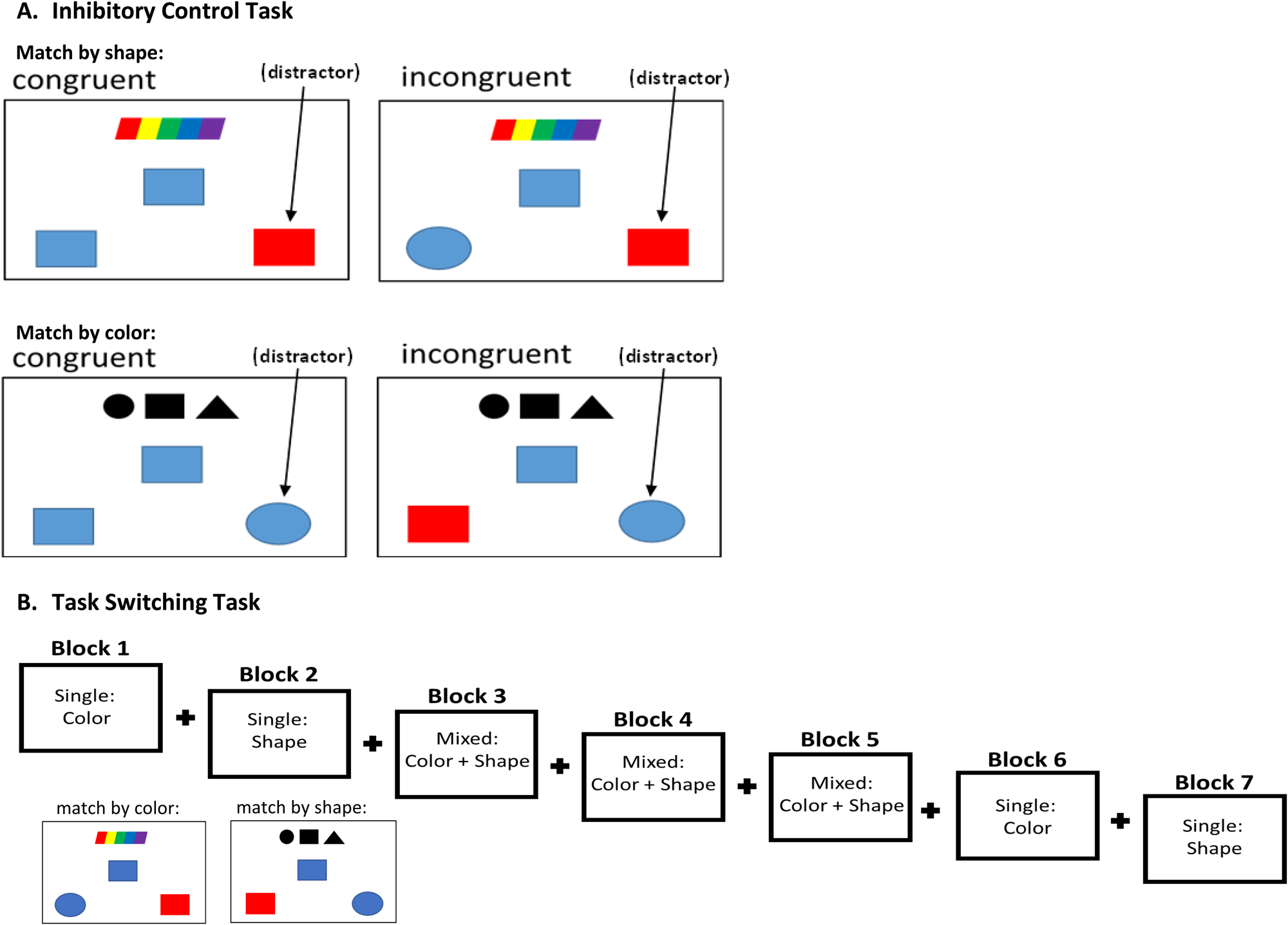
Figure 2ab. Nonverbal Cognitive Control Tasks
Note: A. Inhibitory Control Task: The cue (three black shapes or rainbow) indicates to match by shape or color. The participant indicates with a button press whether the shape in the left or right lower corner matches the shape in the middle of the screen (aka the given item). On congruent trials, all shapes are the same color (when matching by shape) or the same shape (when matching by color). On incongruent trials, the distractor matches the given item on the dimension (color or shape) that must be ignored which creates interference that must be suppressed.
B. Task Switching Task: Trials consist of incongruent color and shape triads from the nonverbal control task. The single blocks include single trials and the mixed blocks include repeat and switch trials.
Treatment
Experimental design
This study used a single-subject multiple baseline design with a A1BA2CA3A4 format (Beeson & Robey, Reference Beeson and Robey2006). Treatment was administered in both the participant's nondominant language (phase B English) and in his dominant language (phase C: Russian). Each treatment phase lasted approximately 10 weeks. Treatment was administered twice per week, for 2 hours each session, for a total of 20 sessions, equating to approximately 40 treatment hours, in each language. Maintenance probes were administered two months after post-treatment probes were completed.
Stimulus development: Category selection
An integral aspect of this study is that treatment stimuli were normed with native Russian speakers to create word lists that are culturally and linguistically appropriate. JP, a native Russian speaker, interviewed 15 native Russian speakers, asking each person to name as many items as possible within 2 minutes for eleven thematic categories (i.e., beach, school, holidays, restaurant, vacation, hospital, house/home, wedding, soccer, pharmacy, and shopping center). In addition to the generative naming task, each of these native Russian speakers rated each category in terms of its relevance to Russian culture, using a 1-5 scale where 1 is not relevant and 5 extremely relevant.
All responses for each thematic category were analyzed to determine three appropriate categories for treatment. Based on cultural relevance, number of abstract and concrete words produced, and minimal overlap across categories, soccer, wedding, and hospital categories were selected for treatment. Soccer was chosen to train abstract words in English, wedding was chosen to train abstract words in Russian, and hospital was chosen as the control category for both English and Russian training phases.
Stimulus development: Words
For a word to be included in treatment, at least two respondents had to have named it and cognates were excluded. To determine whether a word was abstract or concrete, the Brysbaert English database (Brysbaert et al., Reference Brysbaert, Warriner and Kuperman2014) was used to obtain a concreteness rating for each word, where 1 is non-imageable (abstract; e.g., spirituality) and 5 is highly imageable (concrete; e.g., alarm-clock). The concreteness rating threshold for target abstract words in each category was set to less than 3 (less imageable) and was set to 4.5 or higher (highly imageable) for concrete words. Based on the concreteness rating and frequency of response (i.e., if more norming respondents said a word, it was more likely to be chosen for treatment), 10 abstract words were chosen to be trained in each trained category, 10 concrete words were chosen in each trained category as generalization targets, and 10 abstract and 10 concrete words were chosen in the control category. For soccer, the average concreteness rating for abstract and concrete words was 2.67 and 4.6, respectively; for wedding, the average concreteness rating for abstract and concrete words was 2.49 and 4.64, respectively; and for hospital, the average concreteness rating for abstract and concrete words was 2.58 and 4.64, respectively. Abstract and concrete word lists from all categories were translated into English. For each category, abstract and concrete word lists were matched on frequency (all ps > .05) and concreteness (all ps < .05), and word lists across categories did not differ in frequency or concreteness (all ps > .05). See Appendix S1 for a list of all stimuli.
Stimulus development: Features
As part of the treatment protocol, 45 semantic features were required for the feature selection and verification steps. Thirty of the 45 features were acquired from Sandberg and Kiran (Reference Sandberg and Kiran2014), where 15 features represented a general description that could apply to any abstract word (e.g., is a feeling or emotion), and 15 features served as distractors that were not related to any abstract word (e.g., lives in trees). The last 15 of the 45 features were generated by the participant during a “brainstorm” session. In this first treatment session (one time for phase B and one time for phase C), the participant was asked to provide as many descriptions for each of the 10 target abstract words in the trained category as possible (soccer in English phase B and wedding in Russian phase C). For example, one description the participant provided for the target abstract word disappointment from the category soccer was “when something good doesn't happen.” These brainstormed descriptions were fashioned into features such that each could be used as a predicate for one or more trained words. Preference was given to features that were in the participant's own words and that were neither too specific (so they could potentially be used for more than one word) nor too general (so they were still more relevant to the trained items than potentially any abstract word). See Appendix S2 for a list of features for each category trained.
Probes
A category generation task was used to measure improvement resulting from the BAbSANT intervention. These probes were given before, during, and after each training phase. During each probe, Dmitri was instructed to name as many abstract and concrete words as possible in each category (soccer, wedding, hospital) in two minutes. All three categories were tested in one language and then the other language. The order of languages (Russian, English) and order of the three categories (trained, untrained, and control) were randomized across probe sessions. When switching languages, the clinician would chat with Dmitri for 5-10 minutes in the language they were going to use next, to help him make the switch from one language to the other. Five probes were completed in the pre-treatment phase A1, 10 probes were completed during the English treatment phase B, four probes were completed in the interim phase A2, 10 probes were completed during the Russian treatment phase C, four probes were completed in the post-treatment phase A3, and two probes were completed in the A4 maintenance phase. During the treatment phases B and C, a probe was acquired every other session (i.e., one time per week).
Accuracy was determined based on the predetermined 10-item abstract and 10-item concrete word list for each category in the language being tested. Responses provided in the non-target language were counted as incorrect. See Sandberg and Gray (Reference Sandberg and Gray2020) for a complete description of probe administration and scoring procedures for AbSANT.
Although acceptable other responses were tracked (i.e., words that were named by at least two native speakers during norming, but were not included in the target list), appreciable change in these items was not observed and thus the effect sizes for these items will not be reported.
Treatment protocol
The treatment protocol for BAbSANT (Sandberg et al., Reference Sandberg, Zacharewicz and Gray2021) is identical to that of AbSANT (Sandberg & Gray, Reference Sandberg and Gray2020). Each treatment session included five steps for training abstract words: 1) category sorting, 2) feature selection, 3) feature verification, 4) concreteness judgement, synonyms, and recall, and 5) free generative naming. The amount of target abstract words discussed every session varied between 3 and 6 words, depending on the session's pace and other external factors (e.g., participant's level of engagement or fatigue).
Step 1: Category sorting
Dmitri sorted a deck of 40 cards consisting of 20 words (10 abstract and 10 concrete) from the target category (phase B soccer; phase C wedding) and 20 words (10 abstract and 10 concrete) from the control category (wedding). If Dmitri placed words in the wrong category the clinician discussed with Dmitri why it was incorrect, and he moved the card to the appropriate category. Category sorting was conducted once at the beginning of each treatment session.
Step 2: Feature selection
Once the sorting was completed, the clinician presented an individual target abstract word card to Dmitri and a stack of 45 printed semantic feature cards. Dmitri was asked to choose six semantic features that were most applicable to the targeted word. During the first few sessions, the clinician assisted with reading and used communication supports to promote full comprehension of each feature. After six features were selected, Dmitri produced six sentences using the target word with each semantic feature (e.g., “rules are associated with competition”, where “rules” was the target abstract word in the category soccer, and “associated with competition” was one of the 45 features for that category). The feature selection step was conducted for each item that was trained during treatment sessions.
Step 3: Feature verification
After feature selection, feature verification was completed. Dmitri was asked 15 yes/no questions about the target word using the same 45 semantic features. The questions were distributed so that 5 of the 15 questions were acceptable semantic features (based on clinician opinion) and were expected to elicit a “yes” response; another 5 out of 15 questions were expected to elicit a “no” response, being unacceptable semantic features (based on clinician opinion); and the last group of 5 out of 15 questions contained unrelated semantic features and expected a “no” response as well. All 15 questions were asked in a random order to avoid predictability. If Dmitri gave an unexpected answer, the clinician requested an explanation and accepted his answer if Dmitri justified his choice. The remaining questions were adjusted extemporaneously to maintain the 5 yes, 5 no, and 5 distractor composition. The feature verification step was administered for each abstract word trained during the session.
Step 4: Concreteness judgement, synonym, and recall
After feature verification, Dmitri was asked to determine if the target word was abstract or concrete. Then he was instructed to think of a synonym for the target word. For example, regulation is a synonym for the target word rule. If Dmitri had difficulties with finding a synonym, the clinician gave him a choice of two possibilities, with the semantic distance between the possibilities adjusted to provide more or less support, as needed (e.g., easy: regulation vs. sadness; hard: regulation vs. control). Finally, Dmitri was asked to recall the word that he worked on without looking at the card. Step 4 was completed for every abstract word discussed during the session.
Step 5: Free generative naming
Finally, Dmitri was asked to name as many words as he could, including the words from the current session, in the trained category without a time limit. The clinician provided Dmitri with cues and reinforcements as needed to generate as many target abstract words as possible. This step was the last step of each two-hour session and completed only once each session.
Effect size calculation
The direct training effect, within language generalization, and cross-language generalization outcomes were determined by calculating effect sizes (ES). ES were calculated based on Beeson and Robey (Reference Beeson and Robey2006) as the difference between the mean of the post-treatment probes and the mean of the baseline probes, divided by the standard deviation (SD) of the baseline probes. In cases where the SD was 0, we used the value 0.45, which is the lowest possible nonzero SD for 5 probes. The Beeson and Robey (Reference Beeson and Robey2006) guidelines for direct training and generalization ES magnitudes were followed. For direct training, a small ES is 6.5 or higher, a medium ES is 8 or higher, and a large ES is 9.5 or higher. For within-language and cross-language generalization, a small ES is 2 or higher, a medium ES is 5 or higher, and a large ES is 8 or higher.
The ES calculations for phase B (English-abstract-soccer) used probes from A1 (N = 5) and A2 (N = 4). Probes from A2 (N = 4) and A3 (N = 5) were used to calculate the ES for phase C (Russian-abstract-wedding). The effect sizes for the maintenance probes were calculated using the A1 (N = 5) and A4 (N = 2) probes.
Reliability and fidelity
Reliability for the generative naming probes and treatment fidelity were conducted by two trained raters, one of whom was a native Russian speaker. Reliability was conducted on 23% of the Russian probes and 57% of the English probes. Interrater agreement was high, with a Gwet's AC1 of .96 for English probes and .93 for Russian probes. This measure of interrater agreement above chance is preferable to Cohen's Kappa because it does not penalize for extremely high agreement, which should be expected for well-trained raters on straightforward tasks such as this (Gwet, Reference Gwet2008). Treatment fidelity could only be conducted for half of the English phase treatment sessions (25% of total treatment sessions) due to the unavailability of the native Russian-speaking rater during the Russian phase. Overall treatment fidelity was calculated at 97%, with 7 of the 10 rated sessions exhibiting 100% adherence to the protocol. Of the three rated sessions with missing protocol steps, one may have been due to the video being cut short.
Results
An interpretation of effects for all phases and categories is provided in Table 2c. All ESs are listed in Table 2d. The probe results across all study timepoints (i.e., pre-treatment, phase B and C, and post-treatment) are shown in Figure 3.
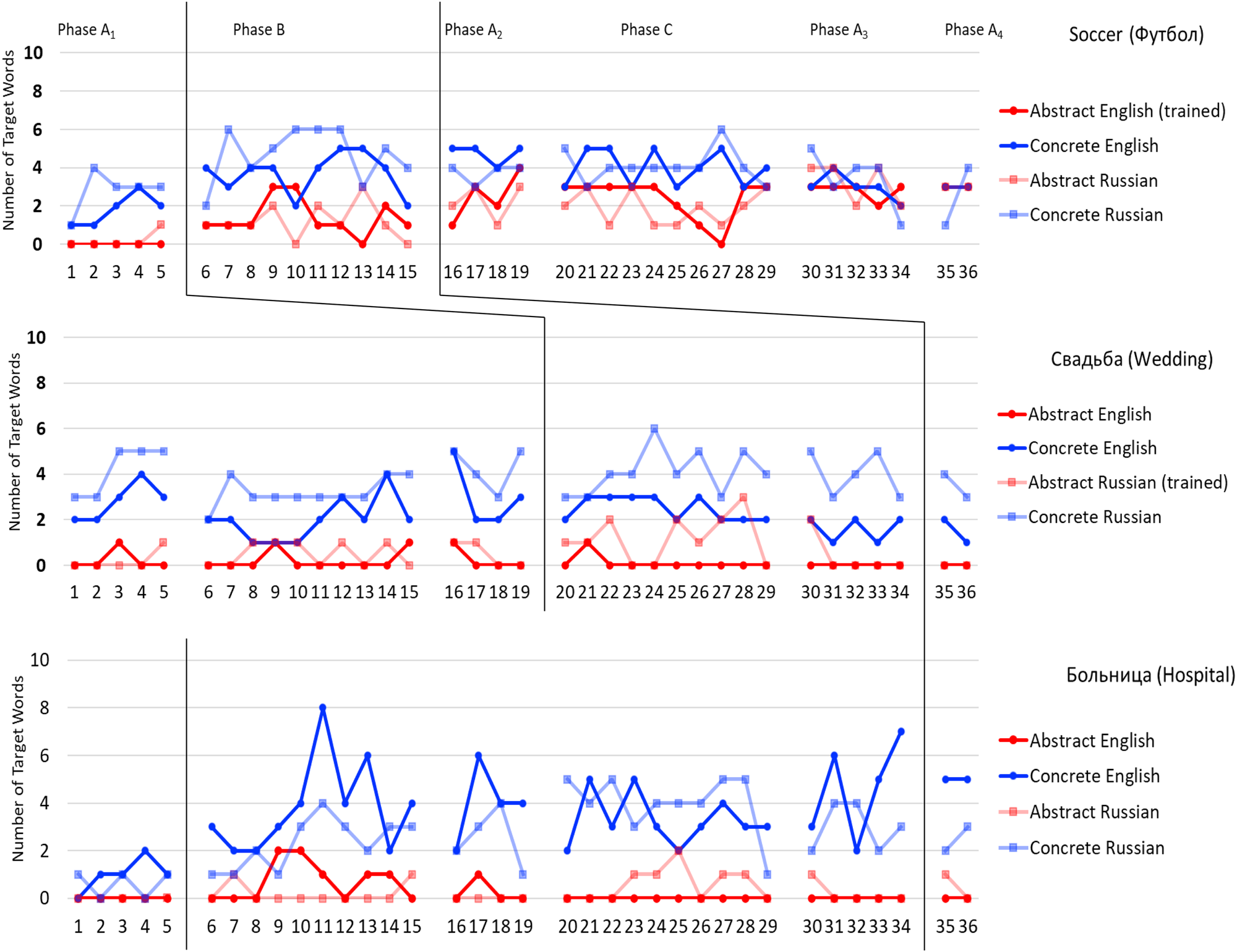
Figure 3. Generative Naming Accuracy for Abstract and Concrete Words in Russian and English Within Each Context-Category for Each Phase of Treatment.
Note. In Phase B, abstract English words were trained in the category soccer. Generalization to within language concrete words and cross-language generalization to Russian abstract words was observed. In Phase C, abstract Russian words were trained in the category wedding. Direct effects of treatment and within- or cross-language generalization effects were not observed.
Phase B (English training in soccer) effects
In phase B, Dmitri was trained on abstract words in English in the thematic category soccer. Although he improved his abstract word retrieval by 25% in English, this resulted in a less-than-small-threshold direct training effect. Dmitri still showed a small within-language generalization effect to concrete words, and a small cross-language generalization effect to the translated abstract words in Russian. Although Russian concrete words appeared to improve during therapy, performance decreased back to baseline levels at post-test, so no cross-language generalization to translated concrete words was observed. Interestingly, during maintenance probes, the direct training effect increased past the small threshold and the cross-language generalization effect increased past the medium threshold. However, the observed within-language generalization was not maintained.
In the control category hospital, which was exposed during the category sorting step, Dmitri showed a small gain in concrete words in English, but this exposure effect did not reach the small direct training threshold. Interestingly, although the Russian translations of these words were not exposed during this phase, they also improved, resulting in a small cross-language generalization effect of exposure.
Importantly, Dmitri did not demonstrate any spontaneous improvements in the category wedding during this phase, indicating a stable baseline for phase C.
Phase C (Russian training in wedding) Effects
In phase C, Dmitri was trained on abstract words in Russian in the thematic category wedding. Although performance was sloping upward for the directly trained abstract words during therapy, it returned to baseline levels at post-treatment. During this phase, Dmitri did not demonstrate within-language generalization to concrete words in Russian, nor cross-language generalization to abstract and concrete words in English. No changes were observed during the maintenance phase.
Additionally, although exposure effects of the control category hospital were observed during phase B, no exposure effects were observed during phase C.
Pre-treatment vs. post-treatment diagnostic testing
There were some pre- to post-treatment changes observed on diagnostic testing scores. On the BAT, a few Russian and English subtests increased by at least 20% (Russian: Complex Commands, Semantic Opposites and Reading Words Aloud; English: Antonyms, II and Listening Comprehension), and some subtests decreased by at least 20% for pre- vs. post-treatment testing (Russian: Antonyms II, Repetition, Dictation; English: Semi-complex Commands and Semantic Acceptability). On the BAT Part C Translation, Word Recognition subtest scores for recognizing words from English to Russian increased by 40%; Translation of Sentences (into English and into Russian) remained at 0% at pre- and post-treatment timepoints. On the CLQT, the attention and clock drawing scores improved from moderate impairment to mild impairment. On the PALPA, three scores improved by at least 20% (Visual Lexical Decision high imageability and Auditory Synonym Judgement high and low imageability), whereas Word Semantic Association high imageability decreased by more than 20%. Other test scores remained similar across pre- and post-treatment timepoints.
Discussion
This study examined within- and cross-language generalization in Dmitri, a Russian–English BPWA who received abstract word retrieval training in his non-dominant English language (phase B) and in his dominant Russian language (phase C). We hypothesized that a) regardless of the language in which training took place, training abstract words would result not only in direct training effects, but also generalization to concrete words in the same thematic category, b) training in the non-dominant language would result in more cross-language generalization than training in the dominant language, and c) cognitive control ability would modulate cross-language generalization effects. These hypotheses were partially confirmed. In general, we found that in a Russian–English unbalanced BPWA with intact cognitive control, training abstract words in the non-dominant language is more beneficial than training abstract words in the dominant language. We will first discuss the results of training in the non-dominant language, then the results of training in the dominant language.
Training abstract words in the non-dominant language promoted both within- and cross-language generalization
Dmitri received treatment in English (non-dominant language) for abstract words in the thematic category of soccer. He exhibited within-language generalization to concrete words, replicating the results of AbSANT studies showing generalization to concrete words (Kiran et al., Reference Kiran, Sandberg and Abbott2009; Sandberg & Gray, Reference Sandberg and Gray2020; Sandberg & Kiran, Reference Sandberg and Kiran2014). Extending these results, we observed cross-language generalization to abstract words in the dominant language (Russian) when the non-dominant language (English) was trained. This is consistent with the literature that shows cross-language generalization when the non-dominant language is trained (Edmonds & Kiran, Reference Edmonds and Kiran2006; Goral et al., Reference Goral, Rosas, Conner, Maul and Obler2012; Kiran et al., Reference Kiran, Sandberg, Gray, Ascenso and Kester2013). However, cross-language generalization to concrete words in the dominant language (Russian) was not observed. This was unexpected, but may be explained by the effect of distance on spreading activation, which can be visualized in Figure 1, Panel B. This illustration shows the assumed effects of phase B training on the activation of lexical forms and concepts, and the spread of activation among lexical forms and concepts.
When English abstract words (such as cheer) were trained, the lexical form was activated, as well as the conceptual representation in the semantic system (activation denoted by thickened circles). Activation could have spread to the semantic system via either the weak link from the English lexicon or via the two strong links to first the Russian lexicon (болеть за [cheer for]), then to the semantic system. The latter scenario, in which semantic access is mediated by activation of the Russian lexicon, would promote cross-language generalization. Once the concept in the semantic system is activated and strengthened, activation can flow to related concepts, including concrete concepts (e.g., fans), as well as to both English and Russian lexicons. This accounts for the observed generalization to concrete words in English, and perhaps some reinforcement of generalization to direct translations. However, Dmitri did not show cross-language generalization to concrete words in Russian (e.g., болельщики [fans]). This may be an effect of cognitive control mechanisms, distance effects of spreading activation, or a combination. Let us first explore cognitive control.
It has been proposed that cross-language generalization is achieved when there is an optimal balance between spreading activation and nonverbal cognitive control (e.g., Kiran et al., Reference Kiran, Sandberg, Gray, Ascenso and Kester2013). Previous studies have found that BPWA who do not show cross-language generalization when trained in the non-dominant language also exhibit poor nonverbal cognitive control (Keane & Kiran, Reference Keane and Kiran2015; Sandberg et al., Reference Sandberg, Zacharewicz and Gray2021). To explore the effects of nonverbal cognitive control, we administered an inhibitory control task and a task-switching task. On the inhibitory control task, Dmitri exhibited intact nonverbal cognitive control, indicating that he is able to suppress and overcome interfering stimuli. On the task-switching task, which is associated with mechanisms of monitoring and resolving conflict, Dmitri exhibited intact mixing costs, but he did not exhibit intact switch costs. These results are in line with previous findings that show BPWA and age-matched bilingual adults without aphasia or neurological disease are observed to exhibit mixing costs but switch costs are not observed (Peng & Gray, Reference Peng and Gray2021). Capturing and interpreting the meaning of mixing costs and switch costs across verbal and/or nonverbal cognitive control tasks can be elusive (Cattaneo et al., Reference Cattaneo, Calabria, Marne, Gironell, Abutalebi and Costa2015; Declerck et al., Reference Declerck, Koch, Duñabeitia, Grainger and Stephan2019; Timmer et al., Reference Timmer, Calabria and Costa2019), raising the question of how these indices of control (measured across a variety of receptive and expressive experimental paradigms) map on to bilingual language control. Some studies have shown that comprehension studies that examine language switching do not consistently evoke switch costs (Declerck et al., Reference Declerck, Koch, Duñabeitia, Grainger and Stephan2019), whereas other findings suggest that both mixing costs and switch costs may be affected by trial-specific manipulations (Mosca & Clahsen, Reference Mosca and Clahsen2016). In sum, because Dmitri showed effects of mixing costs and exhibited patterns indicative of intact cognitive control on the inhibitory control task, we are confident to interpret Dmitri's nonverbal cognitive control as being intact.
Because Dmitri presents with intact nonverbal cognitive control, it is reasonable to consider that mechanisms of spreading activation within and between semantic and lexical levels may play a pivotal role in the process of cross-language generalization. Turning our attention back to Figure 1, Panel A, note that for Dmitri to achieve cross-language generalization to concrete words in Russian (when trained on abstract words in English), two routes for activation flow are possible: 1) abstract concept in semantic system -> concrete concept in semantic system -> Russian concrete lexical entry or 2) abstract concept in semantic system -> concrete concept in semantic system -> English concrete lexical entry -> Russian concrete lexical entry. In the second route, activation of the Russian concrete lexical form may have simply been one step too far, since spreading activation is known to decrease with distance (at least within the semantic system; Collins & Loftus, Reference Collins and Loftus1975). In the first route, activation of the concrete concept appears to have failed to directly activate the Russian lexical form. If cross-language generalization occurs solely due to shared concept activation, we should see similar results for both lexicons (i.e., since concrete words improved in English, they should also improve in Russian). However, we suspect that the cross-language generalization effect we see with direct translations of the trained abstract words is an effect of mediation of initial semantic access by the dominant language.
Training abstract words in the dominant language resulted in null effects
Dmitri received treatment in Russian (dominant language) in the thematic category of wedding. Dmitri did not demonstrate any cross-language generalization to abstract or concrete words in English (nondominant language). This result was in line with our hypotheses. However, Dmitri did not show any direct effect on trained abstract words in Russian nor any within-language generalization to concrete words in this trained language (see Figure 1, Panel C). These results were not expected and need to be further investigated as well as compared with other communication profiles.
Lack of a direct training effect to the trained words and within-language generalization to untrained words has been previously observed in the literature. Kiran et al. (Reference Kiran, Sandberg, Gray, Ascenso and Kester2013) examined within- and cross-language generalization in 17 Spanish–English BPWA. A semantically based intervention was delivered twice a week for two hours. While receiving therapy, UT20 did not show any improvement of the trained and/or semantically related items in her dominant language (Spanish). Unlike Dmitri (who was 68 years old, acquired English at age 40 and was 150 months post onset of stroke), UT20 was an 85.6-year-old bilingual female, who had acquired English at the age of 69 and was 41 months post onset of stroke. However, at the time study participation, both Dmitri and UT20 reported low confidence in their non-dominant L2 English (at 12.5% and 2%, respectively) and stronger confidence in their L1s (Russian and Spanish at 100%). In addition, Dmitri and UT20 are matched on their lifetime exposure in L2 (at 6% and 5%) and in their L1s (at 93.9% and 95%). Dmitri's aphasia was less severe than UT20s; however, during pre-treatment testing, Dmitri and UT20 were stronger at identifying L1 into L2 word translations compared to L2 into L1 word translations, which is contradictory to how healthy brains manage activation flow between two languages and the conceptual system. If access to the conceptual system is breaking down in BPWA, this has the potential to negatively affect how BPWA respond to intervention. Even though Dmitri and UT20's profiles slightly differ, the outcomes of the therapy in their native languages follow the same trend and might depend on the strength of bilingual lexical connections and AoA, as well as lifetime exposure which is associated with AoA.
Dmitri's motivation and attention may have also affected his performance during phase C. Throughout study participation, Dmitri voiced his preference in receiving therapy in English because it was more impaired than Russian. Dmitri completed phase C in Russian but appeared bored and uninterested, evidenced by his asking when the session would be over. He also repeatedly asked the clinician to repeat herself, appearing as though he were not fully attending. Motivation, attention and engagement have been associated with treatment outcomes (Hunting-Pompon et al., Reference Hunting-Pompon, Kendall and Moore2011; Riley & Owora, Reference Riley and Owora2020) and impaired language performance (Roelofs & Piai, Reference Roelofs and Piai2011). In a recent study, Riley and Owora (Reference Riley and Owora2020) investigated the relationship between behavioral engagement as measured by fluctuations in attention and PWA performance on language tasks. Results revealed that lower levels of attention were associated with more errors on language tasks. It could be that Dmitri's lack of interest and attention during treatment delivered in Russian negatively influenced his performance in phase C. These qualitative observations lend support to the importance of prioritizing BPWA preferences when deciding on what language to target in therapy.
Exposure effects
Because we included a category that was exposed during the category sorting step, but was never trained, we have the opportunity to examine the effects of exposure alone. During phase B, both abstract and concrete English words in the category hospital were exposed, and during phase C, both abstract and concrete Russian words in the category hospital were exposed. Interestingly, we found that the exposed English concrete words improved slightly (but did not reach the small threshold) during phase B, as did their Russian translations. This effect appears to be a less-robust version of the direct training and cross-language generalization effects that we observed during phase B. However, during phase C (Russian training), no improvements on the exposed items were observed. Again, aligning with the general lack of improvement observed in phase C. Taken together, the exposure results suggest that a) exposure can improve concrete (but not abstract) word retrieval, albeit slightly, b) generalization from exposure can occur and most likely results from lexical mediation of the dominant language for semantic access (since exposure is not a focused semantic training), and c) Dmitri's performance in phase C was universally depressed, potentially providing further support for the role of motivation and attention.
Generalization to assessment
In an observation pertaining to Dmitri's diagnostic data, various changes in performance observed on the BAT Part C pre-treatment to post-treatment diagnostic testing may suggest that BAbSANT treatment enhances lexical connections between L1 and L2 and the conceptual system. Dmitri's pre-treatment BAT Part C Word Recognition and Word Translation subtest values were stronger when translating from L1 (Dmitri's dominant language) to L2 (Dmitri's non-dominant language). However, based on the revised hierarchical model (Kroll & Stewart, Reference Kroll and Stewart1994) the opposite trend is expected because bilingual healthy adults have stronger activation flow when translating from their non-dominant language into their dominant language. Dmitri's post-treatment testing revealed that he improved when translating from L2 (non-dominant) into L1 (dominant). Specifically, before participating in BAbSANT, Dmitri's performance on the BAT Part C Word Recognition subtest (reading a word in English and identifying its translation in Russian) was at 60% accuracy. After the treatment, his results improved to 100% accuracy. On BAT Part C Translation of Words subtest (hearing a word in English and generating its Russian equivalent), Dmitri's pre-treatment score was at 40% accuracy and his post-treatment score improved to 60% accuracy. In other words, after participating in BAbSANT, the lexical weights between L1 and L2 and the conceptual system had changed to be more in line with bilingual language processing in neurologically intact brains, suggesting that BAbSANT helps improve bilingual language processing. It was observed that on the BAT Part C Translation of Sentences (into English and into Russian), pre- and post-treatment scores remained at 0%. However, translating sentences is more challenging than recognizing words or translating words, so although we speculate that BAbSANT improves bilingual language processing, we are reminded that we must consider the difficulty of the task when examining these trends in the data.
BAbSANT: Comments on lexical and semantic connections
Sandberg et al. (Reference Sandberg, Zacharewicz and Gray2021) adapted AbSANT to BAbSANT and examined its efficacy in a Polish dominant Polish–English BPWA. Although effects of direct training and within-language generalization were observed, cross-language generalization was achieved only when the dominant language was trained. This finding was unexpected because based on the logic of the revised hierarchical model and previous bilingual aphasia treatment studies (Goral et al., Reference Goral, Rosas, Conner, Maul and Obler2012; Kiran et al., Reference Kiran, Sandberg, Gray, Ascenso and Kester2013), we would expect cross-language generalization to the dominant language. Notably, this participant also presented with impaired nonverbal cognitive control, an element required to achieve cross-language generalization. Thus, the findings of Sandberg et al. (Reference Sandberg, Zacharewicz and Gray2021) suggest that the impaired nonverbal cognitive control observed in their participant may have disrupted the way information flowed along the lexical connections between L1 and L2 (although the semantic connections appeared unaffected). In contrast, in the current study, Dmitri's nonverbal cognitive control was unimpaired, and he showed cross-language generalization to the dominant language when the less dominant language was trained, indicative of balanced facilitation and inhibition along both the lexical connections between L1 and L2 and semantic connections.
Conclusion
This study investigated the effects of training abstract words within thematic categories in a Russian-dominant, Russian–English BPWA. When English was trained, direct training effects and within- and cross-language generalization was observed. These findings replicated previous studies (Edmonds & Kiran, Reference Edmonds and Kiran2006; Kiran et al., Reference Kiran, Sandberg and Abbott2009; Sandberg & Kiran, Reference Sandberg and Kiran2014) and are supported by theoretical models of language processing (Kroll & Stewart, Reference Kroll and Stewart1994; Thompson et al., Reference Thompson, Shapiro, Kiran and Sobecks2003). However, when Russian was trained, no improvement was observed. This result could have been due to lack of motivation and interest in receiving treatment in Russian. In this study, the role of nonverbal cognitive control in treatment outcomes was also explored. Our findings align with the notion that intact nonverbal cognitive control supports the advantage of training the non-dominant language for cross-language generalization in an unbalanced BPWA (e.g., Kiran et al., Reference Kiran, Sandberg, Gray, Ascenso and Kester2013), offering support for the relationship between nonverbal cognitive control and bilingual language control (Abutalebi & Green, Reference Abutalebi and Green2007). This finding also suggests that accounting for nonverbal cognitive control should be standard practice in research that examines cross-language generalization in bilingual aphasia.
Because the majority of people in the world are bilingual, there is a need to develop evidence-based interventions designed for BPWA. BAbSANT (Sandberg et al., Reference Sandberg, Zacharewicz and Gray2021), VNeST (Lerman et al., Reference Lerman, Goral and Edmonds2019, Reference Lerman, Goral, Edmonds and Obler2022; Li et al., Reference Li, Li and Kiran2021), and other studies adapting semantically based interventions for BPWA (Edmonds & Kiran, Reference Edmonds and Kiran2006; Kiran & Roberts, Reference Kiran and Roberts2010) are on the forefront of this burgeoning exploration, and future work should systematically expand these therapies across a variety of language combinations. In addition to the investigation of adapting evidence-based monolingual treatments for BPWA, the investigation of leveraging particular word types, e.g., cognates, has been used to explore the advancement of language recovery in bilingual aphasia secondary to stroke (Kohnert, Reference Kohnert2004; Kurland & Falcon, Reference Kurland and Falcon2011) and bilingual primary progressive aphasia (Grasso et al., Reference Grasso, Peña, Kazemi, Mirzapour, Neupane, Bonakdarpour, Gorno-tempini and Henry2021). Continuing to expand these lines of inquiry will help us to better understand bilingual aphasia, how bilingual language control relates to treatment outcomes, and how to best maximize language rehabilitation outcomes for BPWA.
Acknowledgments
The authors would like to thank Dmitri and his family for their time and commitment to participating in BAbSANT. The authors also acknowledge that SF State occupies the ancestral land of the Ohlone and Coast Miwok Peoples and Penn State University campuses are located on the original homelands of the Erie, Haudenosaunee (Seneca, Cayuga, Onondaga, Oneida, Mohawk, and Tuscarora), Lenape (Delaware Nation, Delaware Tribe, Stockbridge-Munsee), Shawnee (Absentee, Eastern, and Oklahoma), Susquehannock, and Wahzhazhe (Osage) Nations.
Supplementary material
For supplementary material accompanying this paper, visit https://doi.org/10.1017/S1366728923000500