Introduction
Through tactical selection of agronomic management practices and associated crop production technologies, dryland farming in highly variable and largely semi-arid Mediterranean regions has been adapted over many years to permit the cultivation of rainfed crops such as small grains, legumes and oilseed crops during the winter. Conventional agriculture in regions with a Mediterranean climate has led to a deterioration of the productive capability of arable soils (Debolini et al., Reference Debolini, Marraccini, Dubeuf, Geijzendorffer, Guerra, Simon, Targetti and Napoléone2018). Conservation agriculture (CA) is widely adopted in Mediterranean regions, such as western and southern Australia, and the Western Cape province of South Africa. The CA principles of minimum soil disturbance, crop rotation and permanent organic soil cover have generally been positive. Soil organic carbon (C) is sequestered or conserved, and the ecological processes that support crop production and system resilience are sustained (Friedrich et al., Reference Friedrich, Derpsch and Kassam2015). The potential of CA is however limited by certain challenges.
Mediterranean regions are characterized by cool, rainy winters and hot, dry summers. As a result, dryland production in these areas is limited to one crop per year with a summer fallow. Despite the retention of crop residues after harvest generally reducing erosion and conserving soil organic C (Ranaivoson et al., Reference Ranaivoson, Naudin, Ripoche, Affholder, Rabeharisoa and Corbeels2017), the lack of anchored, living roots in the soil over the summer months renders these soils vulnerable to damage from torrential floods that are increasingly commonplace in these regions (Michaelides et al., Reference Michaelides, Karacostas, Sánchez, Retalis, Pytharoulis, Homar, Romero, Zanis, Giannakopoulos, Bühl, Ansmann, Merino, Melcón, Lagouvardos, Kotroni, Bruggeman, López-Moreno, Berthet, Katragkou, Tymvios, Hadjimitsis, Mamouri and Nisantzi2018; Kruger et al., Reference Kruger, Mbatha and Ngwenya2022).
Moreover, due to the temporal gap between maximum temperature and irradiance (summer) and maximum water availability (winter), Mediterranean regions are predominantly unconducive to the build-up of soil organic C and are particularly susceptible to its loss (Aguilera et al., Reference Aguilera, Lassaletta, Gattinger and Gimeno2013). Coupled with a history of conventional tillage practices, this has led to the characterization of nearly 75% of Mediterranean region soils as low (⩽2%) or very low (⩽1%) organic C content (Gómez-Sagasti et al., Reference Gómez-Sagasti, Hernández, Artetxe, Garbisu and Becerril2018). Despite examples of certain regions where CA practices result in a soil organic C of more than 2% compared with 0.5% C under conventional tillage (Tshuma et al., Reference Tshuma, Rayns, Labuschagne, Bennett and Swanepoel2021), CA soils are generally still compromised by erosion, compaction and poor resource-use efficiency, all of which are problems that may be linked to low soil organic C (Blanco-Canqui et al., Reference Blanco-Canqui, Shapiro, Wortmann, Drijber, Mamo, Shaver and Ferguson2013). Also, considering the frequent occurrence of droughts in Mediterranean zones and predictions of decreasing mean precipitation attributed to climate change (Michaelides et al., Reference Michaelides, Karacostas, Sánchez, Retalis, Pytharoulis, Homar, Romero, Zanis, Giannakopoulos, Bühl, Ansmann, Merino, Melcón, Lagouvardos, Kotroni, Bruggeman, López-Moreno, Berthet, Katragkou, Tymvios, Hadjimitsis, Mamouri and Nisantzi2018), low soil organic C is a critical limitation given its key influence on soil moisture retention.
Furthermore, although the use of agrochemicals and synthetic fertilizers has underpinned the productivity of CA systems, their widespread over- or inefficient use has incurred substantial environmental costs. For example, inefficient fertilizer use has been associated with contamination of water sources, soil acidification and long-term decline in soil fertility (Dobermann et al., Reference Dobermann, Bruulsema, Cakmak, Gerard, Majumdar, McLaughlin, Reidsma, Vanlauwe, Wollenberg, Zhang and Zhang2022). Whereas, the injudicious and overuse of herbicides has led to the development of herbicide-resistant weeds, further threatening the sustainability of CA systems (Peterson et al., Reference Peterson, Collavo, Ovejero, Shivrain and Walsh2018). Pesticides use and associated loss of biodiversity has also negatively impacted ecosystem services such as pollination and biological control (LaCanne and Lundgren, Reference LaCanne and Lundgren2018). Moreover, the costs of these inputs rising disproportionately to product prices also jeopardize the economic sustainability of small-grain CA systems (Strauss et al., Reference Strauss, Swanepoel, Laker and Smith2021).
Conversely, however, organic cropping systems that omit chemical pesticides and synthetic fertilizers also face challenges including nutrient deficiencies, crop diseases and pest and weed infestation. Furthermore, organic farming systems have been reported to incur especially high yield gaps of up to 40% for wheat and barley (Seufert et al., Reference Seufert, Ramankutty and Foley2012). Thus, although organic systems may contribute positively to regulatory ecosystem services (i.e. improved soil quality), they may not adequately promote provisioning services (i.e. crop productivity benefits) necessary for small-grain production in dryland Mediterranean contexts to be profitable. For this reason, organic agriculture will not be the focus of this review.
Regenerative agriculture (RA), a concept largely unexplored in research in Mediterranean climate regions, is under scrutiny for its potential as an economically viable middle-ground between high-input CA systems and organic systems. Through complementing both CA and organic systems, RA offers perspectives for facilitating the shift away from heavy dependence on artificial inputs and addressing existing challenges of CA systems. It also aligns with the three principles of CA, however takes further measures to not merely conserve but also build soil organic C and improve soil quality, increase biodiversity, promote plant–soil interactions and foster economic sustainability (Schreefel et al., Reference Schreefel, Schulte, de Boer, Schrijver and van Zanten2020). Although this is usually achieved through a combination of agroecological practices (e.g. multi-species cover crops) and specific technologies (e.g. bioeffectors, organic amendments) adapted for a given context (LaCanne and Lundgren, Reference LaCanne and Lundgren2018), there is no clear scientific definition of the practices constituting an RA system. Nevertheless, a key distinction characterizing RA systems in the literature is the omission or minimization of agrochemicals (Rhodes, Reference Rhodes2017).
Blignaut et al. (Reference Blignaut, Knot, Smith, Nkambule, Crookes, Saki, Drimie, Midgley, De Wit, Von Loeper and Strauss2015) suggest conceptualizing RA as the end goal of a sustainability continuum (Fig. 1) that ranges from conventional management practices (considered unsustainable) to ‘sustainable’ CA, and finally to RA, which is considered not only sustainable but also regenerative due to its added restorative and ecological focus. The individual RA technologies and cultivation measures that will be evaluated in this review are not necessarily exclusively RA (Fig. 1), however they do align with the principles of agroecology and are consistent across RA systems in the literature (Rhodes, Reference Rhodes2017). Furthermore, it is when these practices are applied in concert, that this proposed RA concept may most effectively build soil organic C and restore functional ecosystem processes and healthy soils, leading to greater system resilience and crop productivity (Lal, Reference Lal2020), and thereby ultimately address the challenges of soil degradation and dependence on intensive use of agrochemicals.
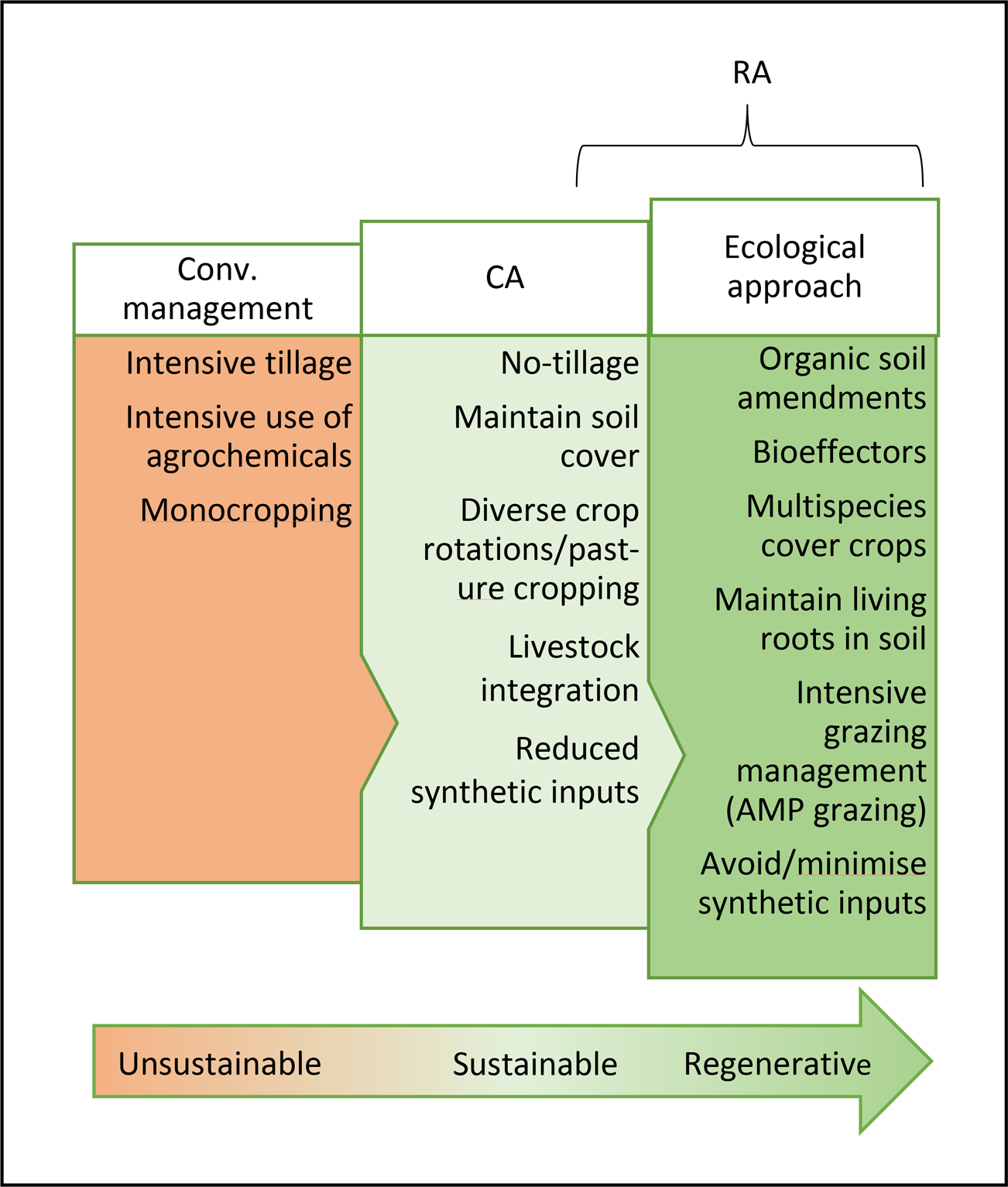
Figure 1. Basic conceptualization of a regenerative agriculture (RA) system using sustainability gradient to depict the progression from conservation agriculture (CA) to RA. AMP, adaptive multi-paddock.
The aim of this review is to describe and evaluate a set of agroecological practices comprising an RA concept, adapted for existing small-grain CA systems under dryland Mediterranean conditions. Expectations for these individual measures that produce specific ecosystem services are discussed from a soil quality, crop productivity and economic perspective, based on recent literature. The first practice is the use of organic soil amendments for the purpose of building soil organic C and improving long-term soil fertility. Secondly, the use of bio-effectors for promoting plant–soil interactions and their role in improving nutrition efficiency, crop quality and resistance to biotic and abiotic stress will be evaluated. The third practice is the growing of winter and summer multispecies cover crops, which are subjected to adaptive multi-paddock (AMP) grazing by sheep. This practice involves extended periods with living roots in the soil, leading to greater soil organic C build-up, along with the ecosystem service provision and enhancement associated with intensively grazed cover crops. Gaps in knowledge on the respective practices will also be highlighted throughout this paper.
Organic amendments for Mediterranean small-grain cropping systems
Organic amendments can be defined as raw or treated materials derived from plants (e.g. straw), animals (e.g. manure) and municipal wastes (e.g. bio-solids), for sustainable soil management and plant productivity (Chen et al., Reference Chen, Camps-Arbestain, Shen, Singh and Cayuela2018). Given the variety in sources, treatments and forms (solid, liquid), it is not surprising they have a wide variety of different properties and agronomic potentialities. However, they are mostly recognized for their enhancement of long-term soil fertility and resulting potential to replace chemical fertilizers completely or partially. Nevertheless, to feasibly apply organic amendments at appropriate quantities to reap these benefits in small-grain cropping systems, a paradigm shift towards a circular economy is necessary. Adopting ‘closed-loop’ models, in which organic waste and by-products are incorporated into the system as assets, may facilitate this shift (Gómez-Sagasti et al., Reference Gómez-Sagasti, Hernández, Artetxe, Garbisu and Becerril2018). Here follows a brief characterization of each of the most prominent categories of organic amendments, and a summary of their potential impacts on soil quality, crop productivity and farm economics within Mediterranean-based small-grain contexts.
Crop residues and mulches
The CA concept adopts an element of the circular economy paradigm through the retention of crop residues as a mulch. This has been reported to support crop production through providing protection against soil erosion, improving infiltration and conserving soil moisture, suppressing weeds and supporting beneficial biodiversity (Ranaivoson et al., Reference Ranaivoson, Naudin, Ripoche, Affholder, Rabeharisoa and Corbeels2017). Nevertheless, it also has its limitations. Residue retention has not shown significant soil fertility improvements or reductions in inorganic fertilizer use. Moreover, residue load and type may impact subsequent crop establishment and thus need to be managed at critical levels to avoid trade-offs.
These limitations warrant investigating the option of using nutrient-rich cover crops as a mulch following termination. Mulched winter cover crops have been shown to increase soil nitrogen and organic carbon in numerous long-term studies (Vincent-Caboud et al., Reference Vincent-Caboud, Casagrande, David, Ryan, Silva and Peigne2019; Smit et al., Reference Smit, Strauss and Swanepoel2021). However, more research is needed to better understand the link between cover crop composition, in terms of the C-to-nitrogen (N) ratio, decomposition and mineralization dynamics in the context of hot, dry Mediterranean summers. Possible implications of mulching a high C:N, grass-dominated mix are slow decomposition and nutrient immobilization. Whereas, low C:N cover crops increase N availability for the cash crop, and while their fast decomposition is less likely to jeopardize subsequent planting (i.e. seed-drill obstruction when in zero-tillage system), their soil-protective benefits may be lessened.
The method and timing of termination of a cover crop need careful consideration to prevent the resurgence of cover crop species as volunteer weeds and ensure sufficient decomposition of the cover crop residue before planting the following crop. Non-chemical termination using a roller-crimper may be used successfully in Mediterranean regions (MacLaren et al., Reference MacLaren, Swanepoel, Bennett, Wright and Dehnen-Schmutz2019b); however, its effectiveness may be influenced by a number of factors. Soils with a high stone content have shown to reduce the stem crushing effect of the roller-crimper, rendering this approach unsuitable in these contexts. In dry years, cover crops may also not produce enough biomass to be effectively terminated by a roller-crimper (Vincent-Caboud et al., Reference Vincent-Caboud, Casagrande, David, Ryan, Silva and Peigne2019). In such cases, mowing or herbicides may be necessary; however, the attendant financial and ecological costs should be considered. Furthermore, successful termination with a roller-crimper also requires timing the rolling to coincide with the growth stage when the stems are susceptible to being crushed and before seed set (MacLaren et al., Reference MacLaren, Swanepoel, Bennett, Wright and Dehnen-Schmutz2019b). This may present additional management challenges when growing a cover crop mixture comprising varying growth cycles. Overall, roller-crimping as a termination method may also have non-nutritive advantages associated with the thicker layer of intact, anchored plant material over the soil surface that may permit greater weed-suppressive and erosion-protective benefits.
Nevertheless, the option of off-field organic waste and by-products as soil amendments (i.e. manure, slurry) has been associated with larger yield increases than these plant-based amendments due to substantially higher nutrient contributions (MacLaren et al., Reference MacLaren, Mead, van Balen, Claessens, Etana, de Haan, Haagsma, Jäck, Keller, Labuschagne, Myrbeck, Necpalova, Nziguheba, Six, Strauss, Swanepoel, Thierfelder, Topp, Tshuma, Verstegen, Walker, Watson, Wesselink and Storkey2022). Thus, the option of applying exogenous organic inputs in small-grain Mediterranean systems needs further evaluation.
Animal manures and chicken litter
Due to their similar nutrient concentrations and agronomic functionalities, the implications for ‘manure’ will be representative of other animal-based amendments; including ‘chicken litter’, ‘slurry’ and ‘bio-solids’ for simplicity purposes.
Animal manures and other animal-derived amendments such as chicken litter (a mixture of chicken faeces, feathers, bedding materials, feed and water) are primarily recognized for their enhancement of soil fertility through their supply of essential macro- and micronutrients (Urra et al., Reference Urra, Alkorta and Garbisu2019). MacLaren et al. (Reference MacLaren, Mead, van Balen, Claessens, Etana, de Haan, Haagsma, Jäck, Keller, Labuschagne, Myrbeck, Necpalova, Nziguheba, Six, Strauss, Swanepoel, Thierfelder, Topp, Tshuma, Verstegen, Walker, Watson, Wesselink and Storkey2022) observed that systems that received manure applications rather than plant-based amendments not only had greater yield increases, but could have most or all the nitrogen fertilizer removed without seeing yield reductions. This suggests that nitrogen supply is an important aspect of the contribution of organic amendments to yields. However, the application of animal manure can also indirectly support long-term soil fertility through increasing short-term microbial immobilization of nutrients, thereby reducing losses to leaching, nitrification and denitrification (Gómez-Sagasti et al., Reference Gómez-Sagasti, Hernández, Artetxe, Garbisu and Becerril2018). Applying manures may reduce N losses and improve fertilizer-use efficiency, particularly on sandy textured Mediterranean soils, which are heavily reliant on N fertilizer and prone to nitrate leaching during intensive winter rainfall (Lassaletta et al., Reference Lassaletta, Sanz-Cobena, Aguilera, Quemada, Billen, Bondeau, Cayuela, Cramer, Eekhout, Garnier, Grizzetti, Intrigliolo, Ramos, Romero, Vallejo and Gimeno2021). In turn, reduced synthetic fertilizer requirements without compromising yield or grain quality may improve the environmental and economic sustainability of these systems. Aside from their nutrient value, manure-based amendments may increase soil organic C and improve soil structure, thereby improving water infiltration and retention (Urra et al., Reference Urra, Alkorta and Garbisu2019). These improved hydro-structural properties may be of particular significance in Mediterranean systems that are often jeopardized by erosion and drought stress.
However, due to the high water content of manure and the especially large volumes required for cereal-based agro-ecosystems, handling of these unprocessed waste materials can be costly and pose various environmental risks, including volatilization of ammonia, greenhouse gas emissions and nitrate leaching (Lassaletta et al., Reference Lassaletta, Sanz-Cobena, Aguilera, Quemada, Billen, Bondeau, Cayuela, Cramer, Eekhout, Garnier, Grizzetti, Intrigliolo, Ramos, Romero, Vallejo and Gimeno2021). Animal manures are also often a hazardous source of contaminants; including pathogens, heavy metals, antibiotics and antibiotic-resistant bacteria. Depending on the animal-based waste and its origin, the level of contamination and potential adverse effects on human, animal and crop health can be significant. However, processing technologies such as anaerobic digestion, composting and biochar production have been shown to suppress these contaminants (Urra et al., Reference Urra, Alkorta and Garbisu2019). Furthermore, these technologies also represent cost-effective strategies for recovering nutrients from manure, while minimizing their environmental impacts and enhancing their economic value (Azim et al., Reference Azim, Soudi, Boukhari, Perissol, Roussos and Thami Alami2018). Nevertheless, to maximize the soil quality and crop benefits of animal-derived amendments and mitigate negative impacts on human and environmental health, there is still need to optimize application methods, establish contaminant thresholds of the various types of animal-based amendments and develop more effective treatment options for reducing contaminants in these organic resources.
Composts and biodigestates
Compost represents the most utilized form of recycled organic matter as a soil amendment. It is the stable end-product of aerobic decomposition of organic materials, typically agro- or municipal waste (crop residues, animal manures or sewage sludge). The composting conditions (i.e. temperature, oxygen and moisture) and the type of raw materials have the greatest effects on maturity, stability, nutrient availability and ultimately the quality of the compost (Sayara et al., Reference Sayara, Basheer-salimia, Education and Hawamdeh2020). Composts derived from nutrient-rich raw materials such as manure may supply significant amounts of essential macro- and micro-nutrients, directly improving soil fertility. Composts are also recognized for improving soil fertility through indirect means such as improved soil structure and associated nutrient retention, as well as improved nutrient cycling through enhanced microbial processing (Urra et al., Reference Urra, Alkorta and Garbisu2019). Although composting helps to reduce transportation costs and facilitates handling through reducing water content of organic wastes, its use in dryland small-grain systems is still limited by low cost-efficiency. Reeve et al. (Reference Reeve, Endelman, Miller and Hole2012), however, report on high rates of composted cattle manure having a long legacy effect on dryland winter wheat yield and soil quality. By inference, a similar strategy of applying high rates of compost at a lower frequency could contribute to the long-term economic feasibility of their use in Mediterranean small-grain CA systems due to lower transport and applications costs. However, the nutritive and non-nutritive carryover effects vary widely depending on compost composition, climate and soil type, and thus extensive context-specific research is needed to evaluate this approach for Mediterranean settings.
Conversely, digestates are produced through the anaerobic digestion of organic materials. They are also typically plant-derived and are relatively low in essential nutrients. Thus, their value is mainly tied to their provision of beneficial microbes that enhance plant growth and nutrient uptake through various mechanisms such as phosphorous and potassium solubilization, and nitrogen fixation (Sulok et al., Reference Sulok, Ahmed, Khew, Zehnder, Jalloh, Musah and Abdu2021). Nevertheless, anaerobic digestion of manure or sewage sludge presents emerging options for increasing and manipulating relative nutrient concentrations of these products, facilitating their handling, and reducing the load of potential human pathogens and pollutants. However, the economic feasibility of this approach for on-farm production is unlikely due to expensive equipment and production costs.
Biochar
Biochar is a highly stable carbon-rich product obtained from the thermal decomposition (pyrolysis) of organic material (feedstock) under low oxygen conditions. The pyrolysis conditions and the feedstock type can be optimized to generate ‘tailored’ biochar that has selective properties which address soil physiochemical constraints common to arable soils in Mediterranean regions. For instance, biochar derived from carbon-rich raw materials such as wheat straw, under relatively fast and high temperature (>400°C) pyrolysis, raises soil pH and soil organic C, and has surface characteristics that improve nutrient retention and create a favourable habitat for microorganisms (Gao et al., Reference Gao, Yang, Liu, Gu, Han, Li, Li, Liu, An, Dai, Liu and Han2021). The agronomic value of such a biochar in Mediterranean CA systems may be in addressing the pertinent issue of soil acidification, serving as a long-acting liming agent (Zhang et al., Reference Zhang, Yan, Niu, Liu, van Zwieten, Chen, Bian, Cheng, Li, Joseph, Zheng, Zhang, Zheng, Crowley, Filley and Pan2016), while also reducing leaching losses, and improving soil nutrient dynamics. Conversely, slow pyrolysis at low temperature (<400°C) of a nutrient-rich feedstock such as manure favours the production of biochar with more labile carbon and nutrients, and thus greater potential to directly improve soil fertility (Lehmann et al., Reference Lehmann, Gaunt and Rondon2006).
However, despite the many reported positive impacts that biochar can have when applied to soils (Basak et al., Reference Basak, Sarkar, Saha, Sarkar, Mandal, Biswas, Wang and Bolan2022), there is a need for long-term research evaluating the effects of different biochars on soil quality and crop productivity under Mediterranean dryland farming constraints. This should be coupled with research in identifying easily accessible and suitable waste materials in these production regions for the efficient upscaling of this technology. Furthermore, biochar's expensive nature brings its economic feasibility into question, particularly in cereal-based agroecosystems. Thus, a long-term economic assessment of its impacts in these systems is called for. There is also a need for developing biochar application strategies (e.g. top-dressing v. incorporation, optimum application rate and co-application with fertilizer or other organic amendments) that will optimize the efficiency of its use (Basak et al., Reference Basak, Sarkar, Saha, Sarkar, Mandal, Biswas, Wang and Bolan2022).
Bioeffectors for Mediterranean small-grain cropping systems
Plant growth-promoting bioeffectors are natural substances and/or microorganisms applied to living plants or soil to enhance nutrient-use efficiency, crop quality, growth and stress tolerance (Hamid et al., Reference Hamid, Zaman, Farooq, Fatima, Sayyed, Baba, Sheikh, Reddy, Enshasy, Gafur and Suriani2021). Although they may improve the ability of crops to utilize nutrients from both artificial and natural fertilizers (i.e. organic amendments), plant bioeffectors differ from fertilizers in that they work regardless of the presence of nutrients in the product. Similarly, they differ from pesticides and other plant protection products in that they do not take direct action against pests or diseases but rather defend plants by competition or antimicrobial properties. Furthermore, in a meta-analysis conducted by Li et al. (Reference Li, Van Gerrewey and Geelen2022), bioeffectors showed the strongest crop yield effects under low precipitation conditions and in poor quality soils, particularly acidic soils with low soil organic C and fertility. Given that these abiotic stress factors are often characteristic of Mediterranean small-grain systems, bioeffectors may serve as a promising tool in safeguarding crop yield under these suboptimal growing conditions.
Although most trials have focused on their ultimate effect on crop performance, the link with improved soil quality and other regulating services still needs strengthening. Furthermore, general ‘easy-to-use’ approaches, applicable over a wide range of environmental conditions, are not available (du Jardin, Reference du Jardin2015). Thus, for bioeffectors to be applied effectively in small-grain Mediterranean systems, more context-specific and quantitative evaluations of the various bioeffector types, combinations and application methods are called for. Evaluations should go beyond crop performance and place more emphasis on regulating services such as climate stress alleviation, nutrient cycling and pest regulation to gain a fuller understanding of their potential to improve yield stability in the given context.
Bioeffectors can be broadly divided into those of microbial origin and those of non-microbial origin. Here follows a brief overview of these two categories in terms of their potential impacts on soil quality, crop performance and whole-farm economics in the context of small-grain cropping systems under a Mediterranean climate.
Microbial bioeffectors
Microbial bioeffectors can be further categorized into beneficial fungi, such as arbuscular mycorrhizal fungi or Trichoderma spp., and beneficial bacteria – primarily rhizospheric or endophytic. The representative genera of plant growth-promoting bacteria (PGPB) include nitrogen-fixing Rhizobium, Azotobacter spp., Azospirillum spp., Pseudomonas spp. and Bacillus spp. Through numerous and often synergistic mechanisms, PGPB applied directly into soil with a carrier material or via inoculated seed could successfully colonize plant roots and positively enhance plant growth and fitness. Plant growth promotion and resilience can be obtained by their release of growth-stimulating compounds and the improvement of nutrient uptake (e.g. iron and phosphorous solubilization and nitrogen fixation). Furthermore, some PGPB may indirectly promote plant growth through control of pathogens, and the synthesis of antibiotics and secondary metabolites that induce systemic resistance (Vimal et al., Reference Vimal, Singh, Arora and Singh2017).
Of the fungal-based bioeffectors, mycorrhizal fungi inoculations forming extensive hyphal root networks that facilitate water and nutrient availability to plants represent an attractive symbiosis in dryland Mediterranean soils that are often compromised by drought stress and consequently low nutrient availability (Lassaletta et al., Reference Lassaletta, Sanz-Cobena, Aguilera, Quemada, Billen, Bondeau, Cayuela, Cramer, Eekhout, Garnier, Grizzetti, Intrigliolo, Ramos, Romero, Vallejo and Gimeno2021). Mycorrhizal fungi may also improve soil water- and nutrition efficiency through their formation of stable aggregates and solubilization of phosphate and micronutrients (du Jardin, Reference du Jardin2015). Distinct from the mycorrhizal species, Trichoderma spp. belong to a class of plant growth-promoting fungi that have been used successfully for biological control of plant pathogens and nematodes. There is also evidence that Trichoderma-based products may increase wheat growth under nutrient-poor and moderate drought conditions through altering the plant hormone balance (López-Bucio et al., Reference López-Bucio, Pelagio-Flores and Herrera-Estrella2015).
However, despite numerous reports of microbial bioeffectors enhancing crop performance and allowing nutrient savings, these are mainly in potting experiments under controlled greenhouse conditions, and there are limited studies demonstrating measurable benefits in field conditions (Herrmann et al., Reference Herrmann, Wang, Hartung, Hartmann, Zhang, Nkebiwe, Chen, Müller and Yang2022). The inability to exclude environmental stress factors and native microbial populations that may impede establishment of plant–microbial interactions leads to unreliable outcomes, limiting their practical use – and adoption in field conditions (Tabacchioni et al., Reference Tabacchioni, Passato, Ambrosino, Huang, Caldara, Cantale, Hett, Del Fiore, Fiore, Schlüter, Sczyrba, Maestri, Marmiroli, Neuhoff, Nesme, Sørensen, Aprea, Nobili, Presenti, Giovannetti, Giovannetti, Pihlanto, Brunori and Bevivino2021). Furthermore, the higher application costs for effective inoculation due to larger soil volumes in small-grain cropping systems, coupled with lower market prices of the final products as compared with horticultural production, further limits their viability in these systems. Nevertheless, the options of localized application of microbial bioeffectors for product-saving (e.g. in-furrow placement) and combined application with selected fertilizers, organic amendments or bioactive compounds as carrier materials offer perspectives for more efficient and cost-effective inoculation under field conditions (Vinci et al., Reference Vinci, Cozzolino, Mazzei, Monda, Spaccini and Piccolo2018). This may be explained by the positive influence of these inputs on viability and survival of novel microorganisms in the soil, along with synergistic interactions that further improve stress resistance and nutrient acquisition of crops. Nevertheless, these strategies still require further research and site-specific adaptations for their potential in small-grain Mediterranean agroecosystems to be realized.
Non-microbial bioeffectors
Non-microbial bioeffectors comprise a variety of bioactive natural compounds from plants or animals, including humic substances, amino acids and extracts from seaweeds and other plants. Relative to microorganisms, these bioactive compounds are less influenced by edaphic and environmental factors after application, and thus may offer options for more efficient and cost-effective applications in small-grain agroecosystems (Tabacchioni et al., Reference Tabacchioni, Passato, Ambrosino, Huang, Caldara, Cantale, Hett, Del Fiore, Fiore, Schlüter, Sczyrba, Maestri, Marmiroli, Neuhoff, Nesme, Sørensen, Aprea, Nobili, Presenti, Giovannetti, Giovannetti, Pihlanto, Brunori and Bevivino2021). Also, despite microbial and non-microbial bioeffectors showing common modes of action towards improving growth, nutrient acquisition and stress tolerance of crops, non-microbial bioeffectors have been most widely recognized for their protection against abiotic stress under field conditions. Exploiting these stress-protective functions may be of value for reducing production risks and yield fluctuations in Mediterranean-based small-grain systems. The potential of common non-microbial bioeffectors and their respective modes of actions, particularly relating to stress mitigation, are thus reviewed.
Seaweed and plant extracts
Seaweeds and plant extracts can directly enhance crop production through their release of bioactive compounds that alter plant metabolism, increasing root growth and nutrient acquisition, seed germination, crop quality and yield (Stamatiadis et al., Reference Stamatiadis, Evangelou, Jamois and Yvin2021). They may also indirectly influence crop production through improving soil growing conditions and nutrient availability for plants. Causal mechanisms may include promoting the function of beneficial fungi (i.e. mycorrhization) and altering soil water holding capacity (Halpern et al., Reference Halpern, Bar-Tal, Ofek, Minz, Muller and Yermiyahu2015). Relative to other microbial and non-microbial bioeffectors, extracts had the largest effect on nitrogen and phosphorous uptake and use efficiency in small-grain and legume crops, as shown in Herrmann et al.'s (Reference Herrmann, Wang, Hartung, Hartmann, Zhang, Nkebiwe, Chen, Müller and Yang2022) global meta-analysis. This may account for the superior biomass production and crop quality benefits observed under extract treatments (Herrmann et al., Reference Herrmann, Wang, Hartung, Hartmann, Zhang, Nkebiwe, Chen, Müller and Yang2022).
However, despite a lack of convincing evidence on the effectiveness of extracts on yield response of small grains under field conditions, their activation of adaptive stress responses may contribute toward their well-established drought and salinity stress-protective functions under field conditions (Stamatiadis et al., Reference Stamatiadis, Evangelou, Jamois and Yvin2021). Considering this, along with their relatively low prices and their cost-saving seed dressing options or low-dose foliar application possibilities, extracts may be a viable technology for improving resilience and yield stability of small-grain cropping systems in Mediterranean environments.
Humic substances
Humic substances, including humic and fulvic acids, are final components of the natural or biochemical decay of organic matter and are therefore stable and resistant to further decomposition. They are most successfully applied as foliar sprays, or directly to the soil. Of the non-microbial bioeffectors, humic substances are mainly recognized for improving soil quality; particularly water holding capacity, nutrient retention, microbial activity and soil aggregation. Humic substances are thus reported to optimize the interplay in the soil between the organic matter, microbes and plant roots, thereby indirectly promoting crop growth and yield. However, they are also reported to enhance crop growth directly through physiological and nutritional effects. Under low pH conditions, humic substances may alleviate aluminium and iron toxicity through forming metal–humic complexes, which leads to improved root growth, phosphorous solubility and ultimately improved nutrient uptake (Halpern et al., Reference Halpern, Bar-Tal, Ofek, Minz, Muller and Yermiyahu2015). Furthermore, humic substances elicit direct physiological effects on plants through functioning as natural plant hormones that enhance germination, lateral root development and fitness and protection against drought and salinity stress (Canellas et al., Reference Canellas, Olivares, Aguiar, Jones, Nebbioso, Mazzei and Piccolo2015).
The aforementioned benefits of humic substances are of high relevance to Mediterranean CA systems, which are typically characterized by and subject to degraded soils and environmental stresses, respectively. However, despite its promising soil quality and crop productivity benefits for small-grain agroecosystems, most humic substances are currently derived from non-renewable resources such as coal and peat (Canellas et al., Reference Canellas, Olivares, Aguiar, Jones, Nebbioso, Mazzei and Piccolo2015). Thus, for the adoption of this technology to be economically feasible in these cropping systems, the development of alternative sources of humic products (e.g. organic wastes) may be necessary.
Protein hydrolysates
Commercially available protein hydrolysates are mostly mixtures of different amino acids and signalling peptides. They are usually derived from the hydrolysis of protein that is of microbial, plant or animal origin, which are often agricultural and industrial wastes (du Jardin, Reference du Jardin2015). Protein hydrolysates are most effectively applied as foliar sprays, and to a lesser extent as a soil drench or seed treatment (Herrmann et al., Reference Herrmann, Wang, Hartung, Hartmann, Zhang, Nkebiwe, Chen, Müller and Yang2022). They have been reported to enhance germination, plant growth/yield and quality of small-grain crops, primarily through their direct effects on nitrogen metabolism and induction of hormonal-like activities that promote root and shoot growth. However, they may also indirectly support crop productivity through enhancing nutrient uptake and efficiency. This is primarily associated with modifications of root architecture for spatial nutrient acquisition, and their provision of amino acids that facilitate microbial mineralization of insoluble nutrients (Halpern et al., Reference Halpern, Bar-Tal, Ofek, Minz, Muller and Yermiyahu2015). With estimations of approximately only 50% N fertilizer-use efficiency and 20% phosphorous fertilizer-use efficiency in certain Mediterranean regions (Lassaletta et al., Reference Lassaletta, Sanz-Cobena, Aguilera, Quemada, Billen, Bondeau, Cayuela, Cramer, Eekhout, Garnier, Grizzetti, Intrigliolo, Ramos, Romero, Vallejo and Gimeno2021), protein hydrolysates may play a significant role in improving the uptake of immobile phosphorous, or easily leached and volatilized nitrate, or urea-based fertilizers in these contexts.
However, there is a growing safety concern of using the most common, animal by-product-derived protein hydrolysates due to potential phytotoxicity and human/animal health risks (du Jardin, Reference du Jardin2015). Nevertheless, enzymatically processed plant-based products are a recent development, which may elicit similar biostimulant potential to their animal counterparts, with additional stress-protectant benefits against salinity, drought and heavy metals (Colla et al., Reference Colla, Rouphael, Canaguier, Svecova and Cardarelli2014). Foliar applications of plant-derived protein hydrolysates offer promising perspectives in the given context and warrant more context-specific trials.
Combination strategies
In recent years, considerable research has gone into the development and adaptation of existing microbial and non-microbial bioeffectors for enhanced field efficiency. Through identifying product-specific modes of actions and designing combinations of microorganisms and non-microbes for targeted and complementary functions, these new products have achieved substantially larger yield increases to any single variants (Herrmann et al., Reference Herrmann, Wang, Hartung, Hartmann, Zhang, Nkebiwe, Chen, Müller and Yang2022). Nevertheless, there is still potential for developing synergistic combination options through better understanding the functional mechanisms underlying different bioeffectors.
Additionally, the simultaneous application of bioeffectors with special nitrogen fertilizers (e.g. stabilized ammonium) has improved their field efficiency through triggering mechanisms for nutrient acquisition (Zimmermann et al., Reference Zimmermann, Claß-Mahler, von Cossel, Lewandowski, Weik, Spiller, Nitzko, Lippert, Krimly, Pergner, Zörb, Wimmer, Dier, Schurr, Pagel, Riemenschneider, Kehlenbeck, Feike, Klocke, Lieb, Kühne, Krengel-Horney, Gitzel, El-Hasan, Thomas, Rieker, Schmid, Streck, Ingwersen, Ludewig, Neumann, Maywald, Müller, Bradáčová, Göbel, Kandeler, Marhan, Schuster, Griepentrog, Reiser, Stana, Graeff-Hönninger, Munz, Otto, Gerhards, Saile, Hermann, Schwarz, Frank, Kruse, Piepho, Rosenkranz, Wallner, Zikeli, Petschenka, Schönleber, Vögele and Bahrs2021). Foliar applications of selected seaweed, plant extracts and humic acids combined with stabilized ammonium were also able to reduce drought stress (Zimmermann et al., Reference Zimmermann, Claß-Mahler, von Cossel, Lewandowski, Weik, Spiller, Nitzko, Lippert, Krimly, Pergner, Zörb, Wimmer, Dier, Schurr, Pagel, Riemenschneider, Kehlenbeck, Feike, Klocke, Lieb, Kühne, Krengel-Horney, Gitzel, El-Hasan, Thomas, Rieker, Schmid, Streck, Ingwersen, Ludewig, Neumann, Maywald, Müller, Bradáčová, Göbel, Kandeler, Marhan, Schuster, Griepentrog, Reiser, Stana, Graeff-Hönninger, Munz, Otto, Gerhards, Saile, Hermann, Schwarz, Frank, Kruse, Piepho, Rosenkranz, Wallner, Zikeli, Petschenka, Schönleber, Vögele and Bahrs2021). Similarly, micronutrient fertilizers such as silicon, zinc and manganese, with functions in stress defence, synergistically improved the stress-protective performance of various microbial and non-microbial bioeffectors in cereal crops (du Jardin, Reference du Jardin2015). These results highlight the importance of further research toward enhancing host plant adaptations for optimizing the efficacy and/or efficiency of bioeffector applications. Long-term context-specific field trials monitoring yield development will also help to better quantify the potential benefits to yield assurance and ultimately whole-farm economics in the Mediterranean small-grain setting.
Cover crops
A cover crop can be defined as a crop that is planted between two cash crop seasons/phases for a purpose other than harvesting (MacLaren et al., Reference MacLaren, Swanepoel, Bennett, Wright and Dehnen-Schmutz2019b). Cover crops therefore do not directly provide an income, but rather are intended to provide or enhance ecosystem services that ultimately improve the sustainability and productivity of a cropping system (Wendling et al., Reference Wendling, Büchi, Amossé, Jeangros, Walter and Charles2017). Despite the name ‘cover crop’, their functions go beyond simply providing cover and protection for the soil. Due to their versatile contribution to the system, cover crops are also referred to as ‘service crops’, ‘green manures’, ‘catch crops’, ‘living mulch’ and ‘forage crops’ (Kaspar and Singer, Reference Kaspar and Singer2011). In farming regions where double cropping is practiced, cover crops are often planted in the fallow season. However, in Mediterranean regions with a poor rainfall distribution, their cultivation generally implies forfeiting a cash crop or pasture in the single winter growing season, incurring financial losses. Thus, their utilization as fodder is necessary to justify their inclusion in these rotations financially (Sanderson et al., Reference Sanderson, Archer, Hendrickson, Kronberg, Liebig, Nichols, Schmer, Tanaka and Aguilar2013; Smit et al., Reference Smit, Strauss and Swanepoel2021).
Cover crops v. monoculture pastures
The integration of monoculture pasture phases into Mediterranean-climate crop rotations and the associated income diversification through supporting a livestock enterprise has helped in dampening fluctuations in income as a result of both grain price and climate variability (Bell et al., Reference Bell, Moore and Kirkegaard2013). Monoculture pastures are typically legume based, consisting of either annual medics (Medicago spp.), clovers (Trifolium spp.) or lupines (Lupinus spp.), which are grown in single season rotations with cash crops, or of lucerne (Medicago sativa), which is grown in long phases of up to 7 years and then followed by 5–7 years of cash crops. Whereas grass-based pasture phases often include oats (Avena sativa) or barley (Hordeum vulgare) that is grown during the winter growing season. These pasture phases have also further optimized the beneficial characteristics of CA, particularly via their capacity to improve weed control and break pest and disease cycles, sequester soil carbon, increase biodiversity and improve nutrient cycling (Planisich et al., Reference Planisich, Utsumi, Larripa and Galli2021). However, the increased diversity that cover crops offer may provide superior soil quality, crop productivity and ultimately economic benefits compared to monoculture pastures.
Perhaps the most significant advantage of multi-species cover crops compared to monoculture pastures is that they do not only offer temporal diversity (through a crop rotation sequence), but also offer spatial diversity. Thus, through utilizing a combination of cover crop species that excel at different services, this may sustain a greater number of agroecological functions simultaneously. Importantly, however, it is the selection for functional diversity of the cover crop that is of more importance for ecosystem functioning than diversity (species richness) per se (Finney and Kaye, Reference Finney and Kaye2017). For instance, given the poor organic matter content of soils in Mediterranean-type environments, cover crops are often selected for functionally diverse traits that mediate nitrogen-related services such as sequestering, scavenging and supplying of nitrogen to the main crop. This could be achieved through combining legumes and cereal species for their respective nitrogen fixing and retention functions, whilst also providing significant carbon input and conservation benefits (Blanco-Canqui and Jasa, Reference Blanco-Canqui and Jasa2019). Nevertheless, selecting for cover crop multi-functionality may also cause trade-offs for service provision. For example, selecting for a highly weed-suppressive mix depends on individual species competitiveness rather than functional diversity. By implication, such a mix will typically have a relative abundance of competitive non-legumes, resulting in lower inorganic nitrogen availability and potentially reduced cash crop yields (MacLaren et al., Reference MacLaren, Swanepoel, Bennett, Wright and Dehnen-Schmutz2019b).
Furthermore, this diversity in space may increase resilience of the ecosystem services cover crops provide, as different plant types contribute to particular services in different ways and under different conditions. This enables a higher overall capacity for a cover crop to consistently perform a given function, whether it is providing more consistent, high-quality forage material and reducing the risk of feed gaps (Bell et al., Reference Bell, Moore and Thomas2018), improving resistance against various biotic or abiotic stresses (Isbell et al., Reference Isbell, Adler, Eisenhauer, Fornara, Kimmel, Kremen, Letourneau, Liebman, Polley, Quijas and Scherer-Lorenzen2017) or extending time with crop cover for erosion prevention and enhanced soil functioning (Garland et al., Reference Garland, Edlinger, Banerjee, Degrune, García-Palacios, Pescador, Herzog, Romdhane, Saghai, Spor, Wagg, Hallin, Maestre, Philippot, Rillig and van der Heijden2021). Nevertheless, a potential drawback of this spatial diversity is an increased susceptibility to harbouring insect pests and diseases and forming a ‘green bridge’ between cover crop and cash crop phases, exacerbating pest populations in crops. Although this is generally not considered a concern in Mediterranean regions due to a relatively dry summer, it warrants further investigation.
To illustrate the importance of permanent living plant material, in these regions where low or erratic rainfall limits plant productivity in the summer months and presents fodder flow and erosion issues, strategically designed cover crops may serve a particularly important role in increasing the proportion of time with living roots in the soil. Through over-sowing a winter cover crop or perennial lucerne pasture with a combination of hardy grass species such as annual teff (Eragrostis tef) or forage sorghum (Sorghum spp.) in spring, this may provide short-lived crop cover extending into the summer months and superior herbage production to perennial lucerne. This, in turn, may lead to improved fodder flow, reduced erosion and leaching, and the development of carbon-rich roots that enhance soil fertility, soil structure and microbial health, ultimately improving crop production (Garland et al., Reference Garland, Edlinger, Banerjee, Degrune, García-Palacios, Pescador, Herzog, Romdhane, Saghai, Spor, Wagg, Hallin, Maestre, Philippot, Rillig and van der Heijden2021).
Chen and Weil (Reference Chen and Weil2010) report that cover crops with deep taproots such as radish can directly alleviate soil compaction through their roots serving as bio-drills that penetrate compacted layers. Although this phenomenon is still disputed in the literature, it is well established that cover crops do reduce the susceptibility of the soil to compaction through improving soil aggregation and decreasing bulk density (Blanco-Canqui et al., Reference Blanco-Canqui, Claassen and Presley2012). Additionally, the inclusion of plants with deep tap roots can create root channels/macro-pores as they decompose, thereby improving water infiltration and the subsequent cash crops’ root growth.
Adaptive multi-paddock grazing v. continuous grazing
The integration of continuously grazed cover crop phases in CA crop rotations of a Mediterranean climate has shown to maintain yields at reduced fertilizer rates (MacLaren et al., Reference MacLaren, Storkey, Strauss, Swanepoel and Dehnen-Schmutz2019a). Set stocking in these systems has also shown to facilitate reductions in chemical plant protectants required for satisfactory weed, pest and disease management, providing not only ecological benefits but also improved economic performance (MacLaren et al., Reference MacLaren, Storkey, Strauss, Swanepoel and Dehnen-Schmutz2019a; Strauss, Reference Strauss, Dent and Boincean2021). However, the alternative use of AMP grazing management is under scrutiny for potentially superior ecosystem service delivery, crop productivity and profitability benefits.
Although numerous publications have dealt with the integration of crops and livestock, there is almost no information comparing continuous to AMP grazing in integrated crop–livestock systems, let alone in Mediterranean climatic contexts. Thus, despite substantial anecdotal evidence from producers in support of AMP grazing on multi-species covers v. continuous grazing, there is still a lack of consensus in the scientific literature on the purported advantages. Nevertheless, based on studies from different farming systems and agroclimatic regions, benefits may still be inferred for crop–livestock systems with a Mediterranean climate.
AMP grazing is an observant approach, such that animal numbers, grazing intensity and recovery periods are adaptively adjusted to match available forage and ensure sufficient post-grazing plant residual for optimal regrowth. Several studies suggest AMP livestock management not only sustains greater forage production and quality than continuous grazing, but also synergistically enhances cover crop service delivery (Kleppel, Reference Kleppel2020). This sustaining of the functional value of cover crops may be largely attributed to the adaptive nature of AMP grazing. Other advantages of AMP grazing are linked to the short and intense nature of this high stock-density approach, which ensures even and non-selective utilization of plant material (Teague and Kreuter, Reference Teague and Kreuter2020). This may exert a greater selection pressure on weeds compared with low-density grazing and prevent less palatable plant types from dominating as a result of selective grazing. Importantly, however, for effective and sustainable weed control, this strategy should be integrated with contrasting selection pressures such as strategic low-use of herbicides and/or mechanical control (MacLaren et al., Reference MacLaren, Storkey, Strauss, Swanepoel and Dehnen-Schmutz2019a). The high utilization of vegetation associated with the high stocking density also implies greater root turnover, thereby stimulating more carbon sequestration and improving soil structure (Kleppel, Reference Kleppel2020). Furthermore, the high stocking density leads to the formation of a uniform layer of manure and plant litter that is trampled into the soil, enriching it with organic matter and enhancing nutrient cycling and soil fertility (Peel and Stalmans, Reference Peel and Stalmans2018).
There are also potential drawbacks of AMP grazing. Particularly when stocking densities are beyond the optimum and/or grazing duration is too long, this approach may increase the risk of overgrazing and soil compaction (Wang et al., Reference Wang, Richard Teague, Park and Bevers2018). Another limitation of AMP grazing is that its intensive and complex nature implies higher levels of organizational skills, knowledge and commitment are required for it to be effectively executed (Sanderson et al., Reference Sanderson, Archer, Hendrickson, Kronberg, Liebig, Nichols, Schmer, Tanaka and Aguilar2013). For instance, the selection of plant species to form a complementary mixture (spatially and temporally) in a specific context and the grazing management thereof is particularly complex. This is because optimal grazing times for maintaining forage quality and productivity vary for grasses and forage herbs due to their different growth patterns, and therefore trade-offs are inevitable with a mixture (Sanderson et al., Reference Sanderson, Archer, Hendrickson, Kronberg, Liebig, Nichols, Schmer, Tanaka and Aguilar2013).
Furthermore, a potential limitation of AMP grazing is that it may not ensure sufficient biomass is consistently maintained for cover crops to maintain their functional value to deliver specific ecosystem services to the cropping system (Sanderson et al., Reference Sanderson, Archer, Hendrickson, Kronberg, Liebig, Nichols, Schmer, Tanaka and Aguilar2013). Kelly et al. (Reference Kelly, Schipanski, Tucker, Trujillo, Holman, Obour, Johnson, Brummer, Haag and Fonte2021) suggest judicious cover crop utilization with low-intensity rotational grazing may be the best strategy to maintain cover crops’ dual-purpose of forage and service provision. However, in the South African Mediterranean climate region, Smit et al. (Reference Smit, Strauss and Swanepoel2021) found that high-density grazing (fundamentally similar to AMP) of cover crops did not affect subsequent wheat grain yield, while it did improve soil quality, and in particular nitrogen content when compared to a no grazing control. These findings suggest there is still a need for further research into the role of grazing intensity on cover crop ecosystem provision.
Research is still needed to identify and better quantify the specific services that different cover crop traits provide, as well as determine the species abundances required to achieve them. Knowledge gaps also exist around the interdependence and trade-offs among these services. Addressing these gaps will help farmers to strategically design multifunctional cover crops based on their specific objectives/constraints. Similarly, for producers to consider adopting AMP grazing of their cover crops, it needs to be validated in context-specific grazing studies that investigate its relative contribution to ecosystem service delivery, compared with conventional set-stocking approaches.
Recommendations for future research
In the face of emerging agroecological and economic challenges that threaten the sustainability of small-grain farming systems in Mediterranean climatic regions, the development of RA cropping systems adapted for these conditions offers promising perspectives. It is evident that technologies such as organic inputs, bioeffectors and cultivation of multi-species cover crops with AMP grazing have potential to improve soil quality, crop productivity and farm economics while allowing for reduced synthetic inputs. However, these improvements can be highly variable and difficult to predict. Thus, to validate their potential in Mediterranean small-grain CA systems, context-specific research needs to be carried out (Table 1). For organic amendments and bioeffectors, there is need for evaluating different product types, combinations, application rates and methods. Importantly, being multi-functional in nature, these technologies should be evaluated based not only their direct but also indirect contributions to soil quality, crop productivity and related ecosystem services, as well as their resource savings in the short and long-term. The RA approach may also provide an alternative to the current high-input, linear model of CA farming systems. The circular economy model for utilizing organic wastes as soil amendments was explored as an option for reducing the heavy reliance on expensive chemical inputs – offsetting the economic and agroecological costs associated with their intensive use. Given the large-scale nature of commercial small-grain croplands, this represents a cost-effective and more realistic option for attaining appropriate quantities of organic inputs for these systems. Despite a range of potential plant growth-promoting and stress priming functions, the viability of microbial bioeffectors seems to be limited in small-grain agroecosystems due to the difficulty of successful establishment of plant–microorganism interactions under stressed conditions. Nevertheless, non-microbial bioeffectors, and various combinations, offer options for more efficient and cost-effective applications in these systems, with particularly promising perspectives for abiotic stress resistance. Implications for small-grain CA systems under Mediterranean conditions may include reduced production risks and yield fluctuations, especially when subjected to drought stress, low soil organic C and low pH conditions. This, along with their potential to improve resistance to biotic stress and enhance the effectiveness of mineral fertilizers, while reducing their use through improved resource use efficiency, may further motivate the use of this technology in these cropping systems. Finally, the option of integrating cover crops subjected to AMP grazing in crop rotations shows promise as a tool for leveraging natural ecological processes to support soil quality, crop productivity and whole-farm economics. There are also, however, potential drawbacks associated with the intensive and complex nature of this approach and to accurately evaluate the potential of this practice in Mediterranean crop–livestock systems, more context-specific research is needed. Nevertheless, for the value of the RA concept to be accurately explored for these systems, all the associated benefits and trade-offs in provisioning and regulating services need to be considered holistically, and thus a systems research approach is recommended. For instance, where certain services may be compromised (e.g. yield reductions), others could compensate (e.g. reduced inputs costs and long-term fertility), thereby still achieving desired agroecological and economic objectives.
Table 1. Summary of research findings and recommendations for selected regenerative agriculture practices in small-grain conservation agriculture systems

Conclusion
The sustainability of dryland Mediterranean-based small-grain CA systems is threatened by inherently low soil organic C, poor and unpredictable rainfall regimes, and a legacy of conventional farming practices that have deteriorated the productive capability of soils. The use of organic amendments, bioeffectors, and multi-species cover crops subjected to AMP grazing show promise as innovative strategies for leveraging ecosystem services to sustain crop production with fewer agrochemicals. In the face of volatile markets and increasing climate variability and unpredictability, the potential of these technologies for improving resilience against environmental and financial stresses are of particular significance. Some of these conclusions are, however, based on inferences from different agroecological contexts, thus, to accurately validate the potential of these technologies in the given context, more long-term and context-specific research is required.
Author contributions
G. A. Musto wrote the article. P. A. Swanepoel and J. A. Strauss made substantial contributions to the conception and drafting of the study and made critical revisions.
Financial support
Western Cape Department of Agriculture and Stellenbosch University are acknowledged for funding the project and offering a bursary.
Conflict of interest
None.
Ethical standards
Not applicable.