Introduction
Dientamoeba fragilis is a pathogenic protozoan parasite which causes diarrhoea and gastrointestinal disease in humans (Barratt et al., Reference Barratt, Harkness, Marriott, Ellis and Stark2011; Stark et al., Reference Stark, Barratt, Chan and Ellis2016). It belongs to the family Trichomonadidae and close relatives are Histomonas and Trichomonas species. This organism was discovered a century ago but its life cycle is yet to be elucidated. Until recently, trophozoites were the recognized D. fragilis life cycle stage, and it degenerates quickly once passed from the host in stool. Several theories suggest how D. fragilis could survive outside the host for a short period of time to allow transmission. One possibility is that Dientamoeba is transmitted via a helminth vector, or through a resistant pseudocyst or a cyst stage (Barratt et al., Reference Barratt, Harkness, Marriott, Ellis and Stark2011; Stark et al., Reference Stark, Beebe, Marriott, Ellis and Harkness2006).
Jepps and Dobell (Reference Jepps and Dobell1918) described the fragile nature of the trophozoite stage of D. fragilis but were unable to find a cyst stage in human stool samples. It was suggested that D. fragilis may form cysts in an unidentified animal species (Jepps and Dobell, Reference Jepps and Dobell1918) indicating the potential for zoonotic transmission. Dientamoeba fragilis has been reported in several animal species including non-human primates, budgerigars, rats, cattle, sheep and pigs (Chan et al., Reference Chan, Barratt, Roberts, Phillips, Šlapeta, Ryan, Marriott, Harkness, Ellis and Stark2016; Galán-Puchades et al., Reference Galán-Puchades, Trelis, Sáez-Durán, Cifre, Gosálvez, Sanxis-Furió, Pascual, Bueno-Marí, Franco, Peracho, Montalvo and Fuentes2021; Stark et al., Reference Stark, Phillips, Peckett, Munro, Marriott, Harkness and Ellis2008; Yetismis et al., Reference Yetismis, Yildirim, Pekmezci, Duzlu, Ciloglu, Onder, Simsek, Ercan, Pekmezci and Inci2022; Yildiz and Erdem Aynur, Reference Yildiz and Erdem Aynur2022). Knowles and Das Gupta (Reference Knowles and Gupta1936) detected Dientamoeba in stool of a captive macaques using iron haematoxylin staining methods (Knowles and Gupta, Reference Knowles and Gupta1936). Another study reported Dientamoeba infections in wild monkeys in the Philippines (Hegner and Chu, Reference Hegner and Chu1930). Two studies showed the presence of Dientamoeba in the stools of western lowland gorilla by microscopy with further confirmation by polymerase chain reaction (PCR) (Lankester et al., Reference Lankester, Kiyang, Bailey and Unwin2010; Stark et al., Reference Stark, Phillips, Peckett, Munro, Marriott, Harkness and Ellis2008).
Evidence for a cyst stage has not only been elusive, but also controversial. However, several authors described long ago what appears to be a cyst-like or a pre-cyst stage of D. fragilis (Knoll and Howell, Reference Knoll and Howell1946; Piekarski, Reference Piekarski1948; Silard et al., Reference Silard, Colea, Panaitescu, Florescu and Roman1979). A pseudocyst stage was described as a small individual with a finely granular cytoplasm with distinct endosomes (Wenrich, Reference Wenrich1936). A more recent report dismissed these studies and suggested that these forms were degenerating trophozoites (Johnson et al., Reference Johnson, Windsor and Clark2004). Therefore, the general perception until recently was that a cyst stage was absent in D. fragilis (Barratt et al., Reference Barratt, Harkness, Marriott, Ellis and Stark2011). However, recent studies found cyst forms of the parasite in clinical samples, albeit at very low levels (Abd, Reference Abd2021; Garcia, Reference Garcia2016; Stark et al., Reference Stark, Garcia, Barratt, Phillips, Roberts, Marriott, Harkness and Ellis2014a).
There have been several attempts to induce experimental infections in a range of animals which have been largely unsuccessful (Dobell, Reference Dobell1940; Kean and Malloch, Reference Kean and Malloch1966; Knoll and Howell, Reference Knoll and Howell1946; Lankester et al., Reference Lankester, Kiyang, Bailey and Unwin2010; Wenrich, Reference Wenrich1944). Wenrich (Reference Wenrich1944) tried to infect laboratory rats with cultured D. fragilis trophozoites orally and via rectal inoculation; however, these attempts were unsuccessful (Wenrich, Reference Wenrich1944). Latter attempts to infect laboratory rats mention that there was damage to the underlying cells where D. fragilis attached to the caecal mucosa in which oedema was evident, however no actual ulceration was detected (Kean and Malloch, Reference Kean and Malloch1966). The failure to develop a reproducible animal model of infection with D. fragilis has hindered research into many aspects of this parasite.
Recently, it was reported that Balb/C mice can be infected orally with culture derived from trophozoites (Munasinghe et al., Reference Munasinghe, Vella, Ellis, Windsor and Stark2013). Cysts were detectable in stool samples by light microscopy and cross-transmission studies with Sprague Dawley rats showed them to be also susceptible to infection by D. fragilis (Munasinghe et al., Reference Munasinghe, Vella, Ellis, Windsor and Stark2013). Dientamoeba fragilis got its name because of the fragile nature of the trophozoite when outside their host. This fragile nature leads us to hypothesize that human-to-human transmission of the trophozoite would be impractical. Thus, we hypothesize that cysts play some role in D. fragilis transmission. As such, excystation and encystation must be features of the D. fragilis life cycle. This process must involve oral ingestion and transversal of the parasites across the hostile environment of the mammalian stomach and other parts of the gastrointestinal track since trophozoites are commonly found in stool samples. In this study, we investigated the ability of cultured trophozoites to withstand the extremes of pH and we provide evidence that trophozoites of D. fragilis are susceptible to highly acidic conditions. We also investigated further the ultrastructure of D. fragilis cysts obtained from mice and rats by transmission electron microscopy (TEM).
Materials and methods
Ethics statement
All animal-based research was approved by the Animal Care and Ethics Committee (ACEC) at the University of Technology, Sydney. Use of clinical samples derived from humans was approved by the Human Research Ethics Committee at St Vincent's Hospital Sydney.
Parasite culture
Strain F of D. fragilis was isolated and cultured from a symptomatic patient at St. Vincent's Hospital Sydney. The trophozoites were grown microaerophilically at 37 °C in McCartney Bottles containing Loeffler's slopes overlaid with phosphate-buffered saline (PBS) as described (Barratt et al., Reference Barratt, Banik, Harkness, Marriott, Ellis and Stark2010). The presence of D. fragilis was initially determined in the symptomatic patient and the parasite culture using the EasyScreenTM Enteric Protozoan Detection Kits (Genetic Signatures, Australia) according to the manufacturer's instructions (Stark et al., Reference Stark, Roberts, Ellis, Marriott and Harkness2014b). Identification of parasites in the culture was confirmed by Sanger Sequencing a region of the small subunit ribosomal DNA (SSU rDNA) at the Australian Genome Research Facility using the novel primers SSUF3 5′-CCG GGT AGT TCC TAC CTT AAA C-3′ and SSUR3 5′-GGA CAT CAC GGA CCT GTT ATT-3′ (Genbank: OR826038). Cycling conditions were denaturation at 95 °C, annealing at 60 °C and extension at 72 °C each for 1 min for a total of 40 cycles.
Resistance of trophozoites to pH conditions
Six solutions of PBS were adjusted using concentrated HCl to cover a pH range of 1–6. PBS (pH 7.0) was used as the negative control with and without a Loeffler's slope. Dientamoeba fragilis trophozoites from the log phase culture (48 h post inoculation of cultures) were centrifuged at 500 g for 10 min. The supernatant was removed and the cell pellet containing the trophozoites was resuspended in the pH-adjusted PBS (pH 1–7). The trophozoite (pH 1–7) suspensions were sampled at time intervals: 0, 2, 4 and 6 h and at each sampling time, the trophozoites resistance to treatment was determined using cell counts with a viability stain (1% trypan blue). These data were used to calculate a total cell count relative to time point 0 (T0), viability (percentage) and viable cell count relative to T0.
Animal experiments
Six-week-old specific pathogen-free Balb/C female mice and Sprague Dawley rats were obtained from the Animal Resource Centre (Perth, WA) and housed in filter top cages in separate rooms. The mice were given sterile rodent chow as standard diet and sterile water to drink ad libitum. The cage bedding was changed every day to avoid any possible cross-contamination. Prior to infection with D. fragilis trophozoites, the animals were surveyed for the presence of intestinal parasites. Two methods were used. Feces was tested daily for 7 days by light microscopy using a modified iron haematoxylin stain as previously described (Stark et al., Reference Stark, Barratt, Roberts, Marriott, Harkness and Ellis2010) to identify any enteric parasites. PCR was also undertaken using D. fragilis-specific primers as previously described (Stark et al., Reference Stark, Barratt, Roberts, Marriott, Harkness and Ellis2010). A tandem multiplex RT-PCR for the detection of Cryptosporidium spp., D. fragilis, Entamoeba histolytica and Giardia intestinalis (Stark et al., Reference Stark, Al-Qassab, Barratt, Stanley, Roberts, Marriott, Harkness and Ellis2011) was also used as a final screen to confirm these animals were free of D. fragilis (along with other protozoa) prior to experimental infection. All these methods showed that the animals were free of any protozoal parasites, including D. fragilis, prior to study.
Mice and rats were infected with approximately100 μL PBS (pH7.0) containing 103–1010 trophozoites orally using a 1 mL syringe. Fecal pellets were collected every day from each mouse into vials containing sodium acetic acid formalin and the presence of D. fragilis was investigated by light microscopy of iron haematoxylin stained fecal smears. The weight, stool consistency and the data on the pattern of shedding were recorded every day during the study (summarized in Supplementary information).
Sprague Dawley rats were given (by a 1 mL syringe) a fecal suspension containing D. fragilis cysts obtained from infected Balb/C mice. The fecal suspension was prepared from feces of infected Balb/C mice where the presence of cysts was confirmed via iron haematoxylin staining. Fecal samples containing D. fragilis cysts were suspended in PBS and thoroughly mixed into a suspension that was administered orally to rats. Fecal samples of infected rats were collected every day post infection, pooled and used for TEM (described below).
Transmission electron microscopy
The methods used are as follows (Munasinghe et al., Reference Munasinghe, Vella, Ellis, Windsor and Stark2013). Pooled fecal pellets from infected mice or rats were collected and resuspended in PBS. Fecal debris was removed by centrifugation at 500 g for 10 min. The supernatant containing cysts was centrifuged at 1500 g for 10 min to pellet the cysts which were washed 3 times with PBS by centrifugation and resuspension in PBS. The pellets containing cysts were fixed in 3% glutaraldehyde in PBS (pH 7.2) at room temperature overnight from all animals where shedding of D. fragilis could be confirmed by light microscopy of iron haematoxylin stained fecal smears. The fixed fecal specimens containing cysts were centrifuged at 500 g for 10 min. The fixatives were removed and PBS added. This washing step with PBS was repeated 3 times for 15 min for each wash. Cell pellets were then embedded in 2% low melt agarose. The agar blocks were cut into 1 mm cubes and post fixed in 1% osmium tetroxide in PBS for 1 h at room temperature. Following post fixation, the samples were washed in distilled water 3 times for 15 min each and subsequently immersed in 2% aqueous uranyl acetate for 30 min. The pellets were dehydrated through a graded series of ethanols (50–100%), infiltrated in mixtures of ethanol and L.R. White Resin (London Resin) and subsequently embedded in LR White Resin. Semi-thin and ultra-thin sections were cut using a Reichert Ultracut S ultramicrotome (Leica Microsystems, North Ryde, NSW, Australia). Semi-thin sections (1 μm thickness) were stained with 1% methylene blue and observed by transmitted light microscopy (Olympus BH2, Olympus, Japan). Ultra-thin sections (70 nm) were mounted onto pioloform coated, 300-mesh, thin-bar copper grids, stained with saturated aqueous uranyl acetate (7.7%) for 30 min and Reynold's lead citrate (Reynolds, Reference Reynolds1963) for 4 min, rinsed with milliQ H2O after each stain. Ultrathin sections were examined using a Philips CM10 transmission electron microscope (Eindhoven, Netherlands).
Results
Resistance of trophozoites to acid pH
Infection of mice with D. fragilis trophozoites led to the presence of cysts in stool. These observations suggest that cultured trophozoites could survive the pH conditions found in the mouse stomach which is typically pH 3 (fed) and pH 4 (fasted) and higher than that found in the human stomach (McConnell et al., Reference McConnell, Basit and Murdan2008). In order to investigate the ability of trophozoites to withstand exposure to extremes of pH, cultured trophozoites were resuspended in PBS adjusted to different pH levels and incubated for periods of time up to 6 h. After incubation, the viability of the cells was assessed by cell counts with a trypan blue viability stain. Our main conclusion was that trophozoites were unable to withstand acidic pH conditions below pH 3 for 2 h, as judged by viable cell counts (Fig. 1). The susceptibility of trophozoites to extremes of pH suggests that D. fragilis cannot easily survive the pH conditions found in the human which typically range from 1 to 3 (Hong et al., Reference Hong, Jiao, Hu, Zhang, Liu, Fu, Shen, Xia and Chang2005).
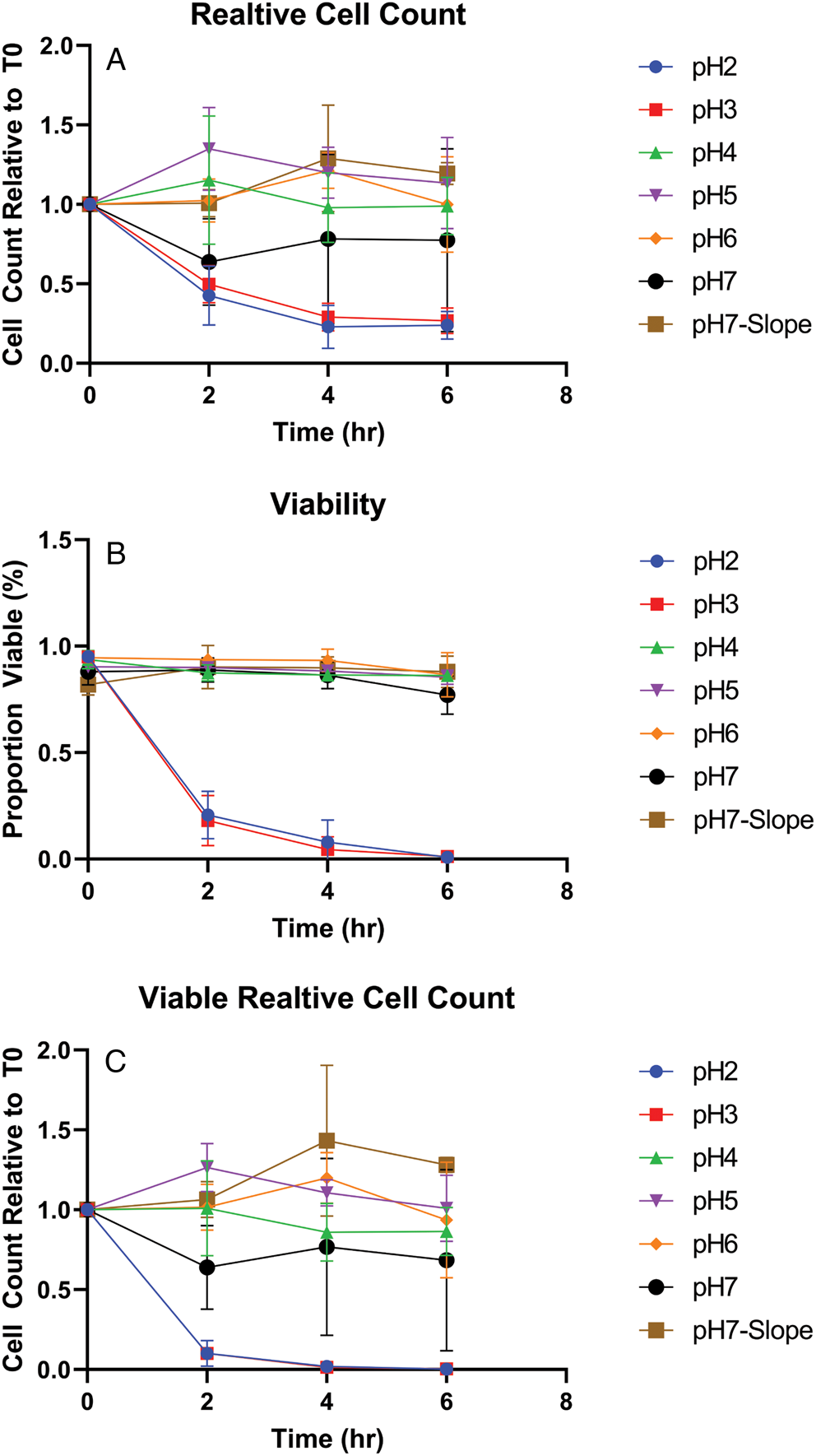
Figure 1. Viability of D. fragilis in-vitro following incubation at acid pH. Dientamoeba fragilis trophozoites were incubated in PBS at different pH levels (2–7) and negative control condition with a Loeffler slope (pH7-Slope) for 0–6 h. The graphs show the resulting total cell counts relative to T0 (a), viable proportion (b) and relative viable cell counts (c). The error bars represent standard error of mean cell counts, performed in triplicate.
Transmission electron microscopy of cysts
The ultrastructure of the cell wall and other organelles including nuclei and flagellar axonemes was examined. Dientamoeba fragilis cysts had a well-developed cyst wall and the parasite was enclosed within. Twenty D. fragilis cysts from the rats were measured and the cyst diameter fell within range 4–6 μm. The cyst diameter of the 5 cysts from the mice was 4–5 μm, with the range found in the rat model.
Nuclei
Cysts contained either 1 (Fig. 2A; 80% of cysts seen) or 2 nuclei (Fig. 2A; 20% of cysts seen) in the TEM planes analysed. The nuclear membrane was not visible in some cysts (Fig. 2A). The nucleolus was located centrally in the nucleus (Figs 2A and 3A).
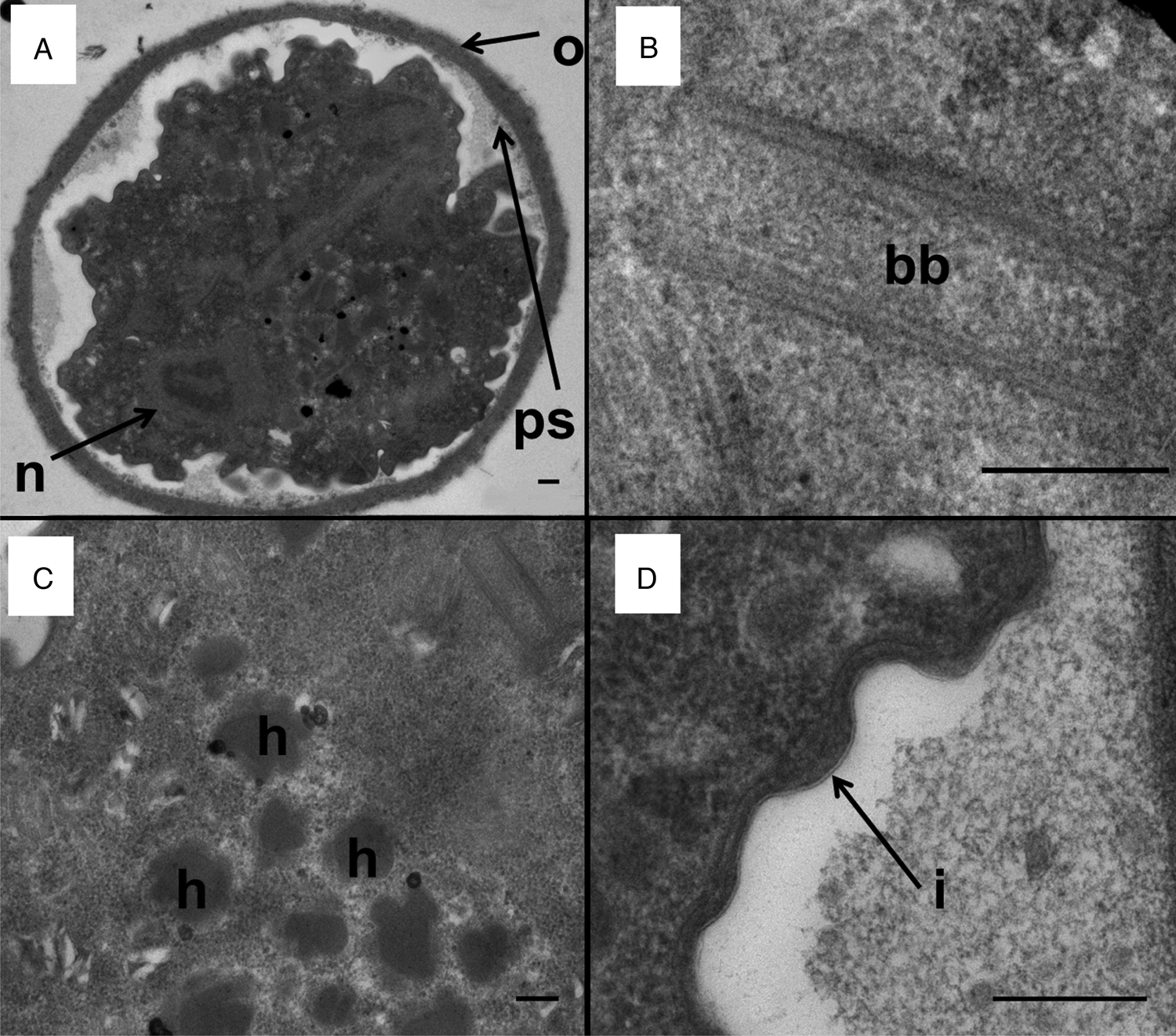
Figure 2. Transmission electron micrographs of a D. fragilis cyst showing the cyst wall and other organelles. (A) Transverse section across a mononucleated cyst showing the outer fibrillar cyst wall (o), peritrophic space (ps) and the nucleus of the encysted parasite (n). (B) Basal body structure (bb). (C) Developing hydrogenosomes which contain a dense core and a less electron-dense outer layer (h). (D) The inner membrane of the cyst wall (i). Scale bar represents 200 nm.
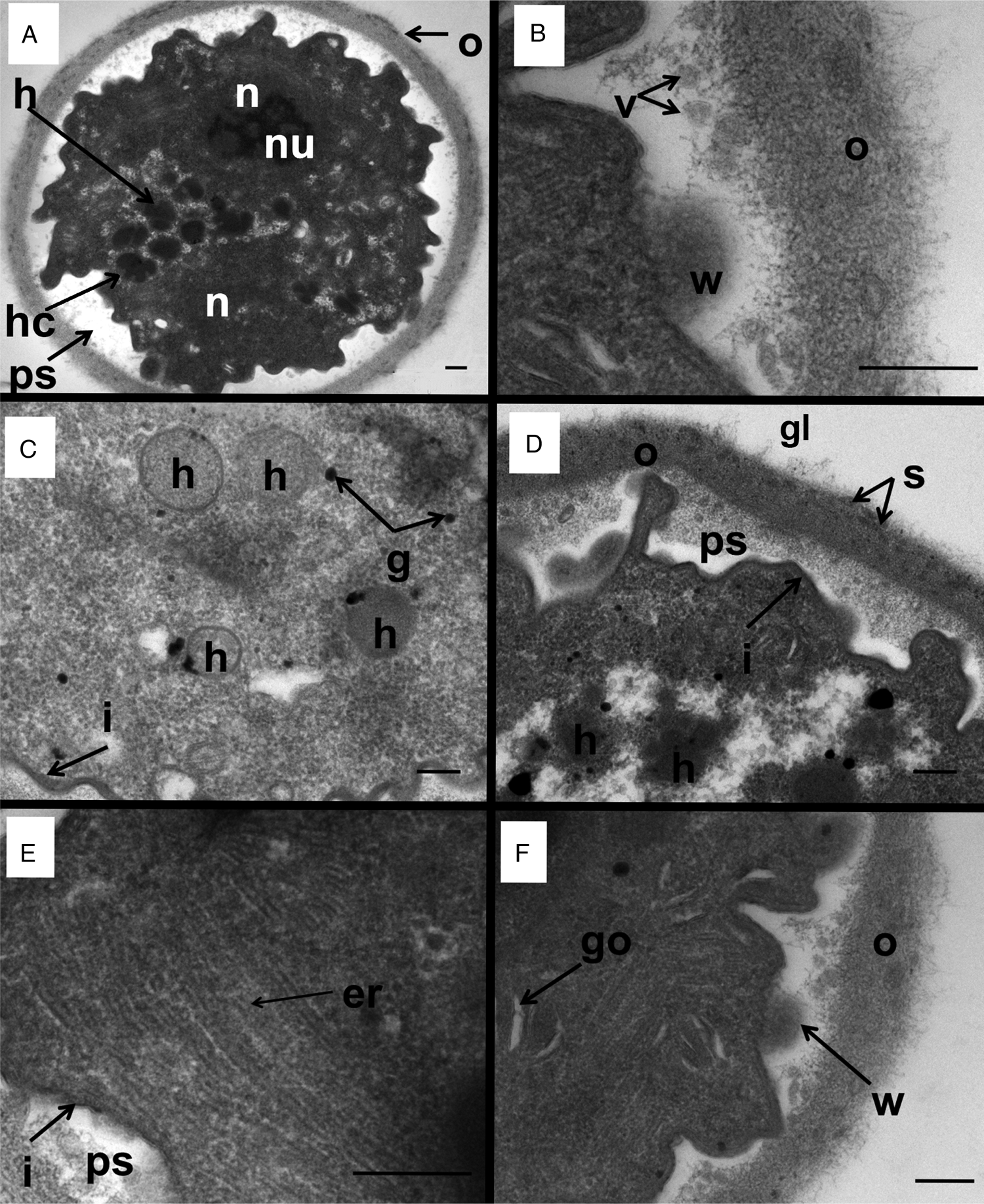
Figure 3. Transmission electron micrographs of a D. fragilis cyst showing the whole cyst and other organelles and structures. (A) Transverse section across a binucleated cyst showing the outer cyst wall (o), the peritrophic space (ps), the 2 nuclei (n) nucleolus (nu). Note the hydrogenosomes (h) and the ‘cauliflower-like’ hydrogenosomes (hc) that lie in the space between the margins of the 2 nuclei. (B) A closer view of the outer cyst wall (o). Note the weakened area of the inner membrane (w) lying directly next to the ESV-like structures (v). (C) Hydrogenosomes (h), glycogen granules (g) and the inner membrane of the cyst (i). (D) Electron micrograph of the outer cyst wall (o) showing the dark striations (s) and coated in a glycocalyx (gl), the inner membrane (i), the peritrophic space (ps). Note the developing hydrogenosomes with the darker inner core and less electron-dense outer layer (h). (E) Smooth endoplasmic reticulum (er), inner cyst membrane (i) and peritrophic space (ps). (F) Golgi complex of D. fragilis cyst: weakening of the inner membrane (w): outer cyst wall (o).
The nucleus as far as it existed in the cutting plane showed an ovoid or an irregular shape. In all cysts with a single nucleus observed, no nuclear membrane was visible (Fig. 2A) which could be due to the difficulty in distinguishing the membrane from the often dense cytoplasmic contents. In contrast, cysts with 2 observed nuclei contained a nuclear membrane that was visible as described previously (Munasinghe et al., Reference Munasinghe, Vella, Ellis, Windsor and Stark2013). A clear nucleolus was seen which is electron dense, fragmented, irregular in shape and lacks a membrane.
Cyst wall
The cyst wall consisted of an outer layer and an inner layer (Munasinghe et al., Reference Munasinghe, Vella, Ellis, Windsor and Stark2013). The outer cyst wall contained fibrillar elements which appeared to be dense and had a uniform thickness (approximately 0.2 μm) (Figs 2A and 3A). The outer cyst wall was coated by a glycocalyx (Fig. 3D). There were dark striations along the outer fibrillar cyst wall (Fig. 3D). The inner cyst wall consists of 2 layers which collectively appear as a dark margin outlining the parasite (Fig. 2D).
Encystation specific vesicles (ESVs)
The presence of ESV-like structures in the cyst was described previously (Munasinghe et al., Reference Munasinghe, Vella, Ellis, Windsor and Stark2013). A weakening in the inner membrane of the encysted parasite occurs which is surrounded by vesicles in the peritrophic space (Fig. 3B, F). These weakened areas of the inner cyst membrane resemble areas which liberate the ESV-like structures from the encysted parasite into the peritrophic space. This is similar to that process which occurs in Giardia cysts where the ESVs are exported from inside the cyst to the periphery of the cell for deposition (Carpenter et al., Reference Carpenter, Assaf, Gourguechon and Cande2012).
Cytoplasm
Other organelles were not as visible in the cyst as in the trophozoite stage of D. fragilis, although the endoplasmic reticulum (ER) and structures that are morphologically similar to Golgi bodies were easily identifiable (Fig. 3E, F). Electron-dense particles that measure 0.1–0.2 μm could be seen scattered across the cytoplasm which are similar in appearance to glycogen granules reported in the trophozoites of D. fragilis (Banik et al., Reference Banik, Birch, Stark and Ellis2012) and other trichomonads (Munsch et al., Reference Munsch, Lotfi, Hafez, Al-Quraishy and Mehlhorn2009) (Fig. 3C). ER was scattered around the cytoplasm (Fig. 3E).
Hydrogenosomes
Hydrogenosomes are double layered membrane bound, electron-dense organelles located in the cytoplasm of the D. fragilis cyst. They ranged in size from 0.3 to 0.4 μm and the number present in cysts ranged from 10 to 12 (Fig. 3C). The hydrogenosomes in some cysts were spherical in shape, bound with a double membrane, electron dense and located in the cytoplasm. In some cysts, the hydrogenosomes had an appearance of a ‘cauliflower shape’, the size ranging from 0.33 to 0.38 μm (n = 6) and the number present varied from 4 to 20 (Fig. 2C). These ‘cauliflower-type’ hydrogenosomes possessed an outer layer which was less electron dense and an inner core which was more electron dense. Some of these had an outer membrane (Fig. 2C). The hydrogenosomes in some cysts contained a homogeneous matrix (Fig. 3C). Glycogen granules were also often observed near both types of hydrogenosomes.
Basal body structure
A structure that looks similar to a basal body was present in D. fragilis cysts (Fig. 2B). The cysts also possessed an axostyle, flagellar axonemes, pelta and a costa (Munasinghe et al., Reference Munasinghe, Vella, Ellis, Windsor and Stark2013).
Discussion
To our knowledge, our report of a cyst stage in the life cycle of D. fragilis and the successful establishment of an animal model to study infection and transmission was important in providing evidence that transmission of D. fragilis may occur through a fecal–oral process via a cyst stage (Munasinghe et al., Reference Munasinghe, Vella, Ellis, Windsor and Stark2013). In this study, we extend these observations by showing that trophozoites are susceptible to acid exposure, and so are unlikely to be able to withstand the extremes of pH found in the human gastrointestinal tract (discussed below). We also provide a description of the cyst stage as studied by TEM. These studies also complement and extend those of the trophozoite stage of D. fragilis (Banik et al., Reference Banik, Birch, Stark and Ellis2012).
The treatment of trophozoites with PBS of pH less than 7 was carried out to determine the effect of extreme acid pH on the survival of D. fragilis trophozoites. The results showed that D. fragilis trophozoites were unable to survive for 2 h in pH 3 or less. This represents an important finding since it demonstrates that D. fragilis is not tolerant of environmental extremes, supporting the long-held dogma of the ‘fragile’ nature of this organism. After ingestion via the fecal–oral route, D. fragilis must travel through the stomach into the gut where it has to survive an acidic pH which is usually 1.8–2.0 in a healthy human (Hong et al., Reference Hong, Jiao, Hu, Zhang, Liu, Fu, Shen, Xia and Chang2005) for 1–6 h depending on stomach content (Feldman et al., Reference Feldman, Smith and Simon1984; Meyer et al., Reference Meyer, Elashoff, Porter-Fink, Dressman and Amidon1988; Pal et al., Reference Pal, Brasseur and Abrahamsson2007). Trophozoites were infrequently found in the feces of infected mice and rats compared to the relative abundance of cysts, suggesting that D. fragilis cysts may play a role in zoonotic transmission of this organism. Alternatively, this could indicate that the development of cysts is upregulated when conditions are not favourable for the multiplication of D. fragilis, such as finding itself in the wrong host. In contrast, in humans the major morphological forms found are trophozoites (Stark et al., Reference Stark, Barratt, Roberts, Marriott, Harkness and Ellis2010). Precystic forms are rarer and cyst forms almost never seen (Stark et al., Reference Stark, Garcia, Barratt, Phillips, Roberts, Marriott, Harkness and Ellis2014a). Recent studies confirmed the identification of cysts from human clinical samples which are morphologically similar to the cysts that were found in this study (Abd, Reference Abd2021; Garcia, Reference Garcia2016; Stark et al., Reference Stark, Garcia, Barratt, Phillips, Roberts, Marriott, Harkness and Ellis2014a). The frequency in which cysts are reliably found in human clinical samples leads us to believe that human-to-human transmission may not be the dominant form of transmission for D. fragilis and that transmission from animals may be a significant contributor to human infection.
The observation that the trophozoite of D. fragilis is unable to resist acidic conditions might appear to be in contradiction to the establishment of a D. fragilis model in mice using cultured trophozoites (Munasinghe et al., Reference Munasinghe, Vella, Ellis, Windsor and Stark2013). It is important to note, that cultured trophozoites were unable to establish infections in rats; in contrast, a suspension of mouse feces containing cysts was able to result in infection. After the establishment of the mouse model, a study determined that a dose of 105 trophozoites per mouse was required for a 37% chance to establish infections in mice using the oral route and showed a 100% infection with a dose of 4 × 106 trophozoites per mouse (El-Gayar et al., Reference El-Gayar, Mokhtar and Hassan2016). This is higher than in our experiments as the minimum infectious dose was 105 and 103 in our second experiment. This impact could be a result of how D. fragilis adapts to growing in culture. The isolate that we selected for this study was the one that we were culturing which grew most readily (data not shown). Compared to the infective dose of other gastrointestinal protozoa, this is potentially higher: greater than 1 cyst for Entamoeba coli (Rendtorff, Reference Rendtorff1954), greater than 1000 cysts for E. histolytica (Ryan et al., Reference Ryan, Kenneth, Ray and Sherris2004), 30–1000 oocysts for Cryptosporidium parvum (DuPont et al., Reference DuPont, Chappell, Sterling, Okhuysen, Rose and Jakubowski1995) and 10–10 000 cysts for G. intestinalis (Schaefer et al., Reference Schaefer, Johnson, Hsu and Rice1991). Compared to the healthy pH range of 1.8–2.0 in the stomach of humans (Hong et al., Reference Hong, Jiao, Hu, Zhang, Liu, Fu, Shen, Xia and Chang2005), the pH range of the stomach in a mouse is 3.0–4.0 (McConnell et al., Reference McConnell, Basit and Murdan2008). It is important to note that the pH range of the rat stomach is 3.2–3.9, which is similar to a mouse, so the pH of the stomach cannot solely explain the failure to establish an infection in a rat using trophozoites (McConnell et al., Reference McConnell, Basit and Murdan2008). Mice have a shorter gut transit time than rats which may limit the exposure of D. fragilis trophozoites to acid in their stomach in comparison (Giron et al., Reference Giron, Perez-Garcia and Abalo2016). We speculate these differences have allowed for the establishment of the mouse model.
Our observations that trophozoites cannot withstand acid pH treatments are consistent with the mechanisms of transmission involving either the pre-cyst, cyst stage or E. vermincularis vector. Evidence for the role of E. vermincularis as a vector of D. fragilis infection includes detecting D. fragilis DNA inside pinworm ova (Clark et al., Reference Clark, Röser and Stensvold2014; Ögren et al., Reference Ögren, Dienus, Löfgren, Iveroth and Matussek2013; Roser et al., Reference Roser, Nejsum, Carlsgart, Nielsen and Stensvold2013). While these results are certainly compelling, this still does not indicate that the organisms within are viable or transmissible, as previously suggested (Clark et al., Reference Clark, Röser and Stensvold2014). In previous studies on the use of hypochlorite to destroy DNA, a 2.5–3% solution (w/v) was recommended for at least 15–40 min (Greenstone et al., Reference Greenstone, Weber, Coudron, Payton and Hu2012; Kemp and Smith, Reference Kemp and Smith2005). It is likely that this sterilization procedure employed in those studies on D. fragilis may have been insufficient to remove DNA (Ögren et al., Reference Ögren, Dienus, Löfgren, Iveroth and Matussek2013; Roser et al., Reference Roser, Nejsum, Carlsgart, Nielsen and Stensvold2013). A final step of DNAase treatment appears preferable to ensure that all exogenous DNA had been completely removed. Indeed, a similar experiment which employed DNAse treatment found that DNA of D. fragilis was not detected within E. vermicularis ova (Menghi et al., Reference Menghi, Makiya, Gatta and Méndez2005). Additionally, E. vermicularis is a human-specific Oxyurid nematode, with rare identifications in captive chimpanzees (Murata et al., Reference Murata, Hasegawa, Nakano, Noda and Yanai2002). The identification of D. fragilis in a variety of non-human hosts (Chan et al., Reference Chan, Barratt, Roberts, Phillips, Šlapeta, Ryan, Marriott, Harkness, Ellis and Stark2016; Galán-Puchades et al., Reference Galán-Puchades, Trelis, Sáez-Durán, Cifre, Gosálvez, Sanxis-Furió, Pascual, Bueno-Marí, Franco, Peracho, Montalvo and Fuentes2021; Hegner and Chu, Reference Hegner and Chu1930; Knowles and Gupta, Reference Knowles and Gupta1936; Lankester et al., Reference Lankester, Kiyang, Bailey and Unwin2010; Stark et al., Reference Stark, Phillips, Peckett, Munro, Marriott, Harkness and Ellis2008; Yetismis et al., Reference Yetismis, Yildirim, Pekmezci, Duzlu, Ciloglu, Onder, Simsek, Ercan, Pekmezci and Inci2022; Yildiz and Erdem Aynur, Reference Yildiz and Erdem Aynur2022) indicates that a model of transmission using E. vermilcularis as a vector responsible for its transmission is unlikely. In our study, rodents were not infected with pinworm. Notably, rodent pinworms belong either to the Syphacia and Aspiculuris genera, not Enterobius like the human pinworm (Meade and Watson, Reference Meade and Watson2014). For the helminth vector model to remain, a non-species-specific helminth must be involved which has yet to be identified, or the mechanism by which D. fragilis enter the ova must not be species-specific. The variety of D. fragilis hosts supports the importance of the cyst model of transmission. Further studies are required to ascertain the exact life cycle and mode of transmission of this parasite.
TEM of the D. fragilis cysts showed they had a spherical to ovoid shape with a thick cyst wall that is filamentous in appearance. The overall structure of the cysts observed in this paper and our previous study shows remarkable similarity to the cysts of Trichomitus batrachorum and Trichomonitus sanguisugae including the presence of a peritrophic space and axoneme (Brugerolle, Reference Brugerolle1973; Munasinghe et al., Reference Munasinghe, Vella, Ellis, Windsor and Stark2013). The cyst wall contained 2 layers: an outer dense fibrillar layer and a double-membrane bilayer enclosing the encysted parasite. Thus, the cyst wall of D. fragilis is similar to that of T. batrachorum, T. sanguisugae, Honigbergiella sp. and Giardia which also has an outer fibrillar layer and an inner double layer which is continuous with the plasma membrane of the trophozoite (Brugerolle, Reference Brugerolle1973; Coggins and Schaefer, Reference Coggins and Schaefer1986; Hampl et al., Reference Hampl, Cepicka, Flegr, Tachezy and Kulda2007). The outer cyst wall consisted of a meshwork of fibrillar elements whereas the inner bilayer encloses the parasite. There is a space between the cyst wall and the encysted parasite which is similar to the peritrophic space reported in Giardia cysts (Coggins and Schaefer, Reference Coggins and Schaefer1986). Similar to the cyst of Honigbergiella sp., the outer cyst wall is coated with a glycocalyx (Hampl et al., Reference Hampl, Cepicka, Flegr, Tachezy and Kulda2007). This space shrinks in mature D. fragilis cysts where the plasma membrane is closely attached to the cyst wall as seen in Entamoeba species (García-Zapién et al., Reference García-Zapién, González-Robles and Mora-Galindo1999).
Despite these similarities, there were some structural differences in the cyst wall of Giardia and D. fragilis. The cyst wall of Giardia contains a filamentous outer layer and 2 cell membranes separated from the plasma membrane of the parasite by a peritrophic space (Chávez-Munguía et al., Reference Chávez-Munguía, Cedillo-Rivera and Martínez-Palomo2004). In D. fragilis, the cyst wall contains an outer filamentous portion and an inner bilayer membrane enclosing the parasite. The peritrophic space was present between the outer layer and the inner bilayer. Fibrillar material is also present in the cyst wall of Entamoeba species (García-Zapién et al., Reference García-Zapién, González-Robles and Mora-Galindo1999). In Entamoeba invadens, the cyst wall is a single continuous fibrillar layer which is closely associated with the plasma membrane (Chávez-Munguía et al., Reference Chávez-Munguía, Omaña-Molina, González-Lázaro, González-Robles, Cedillo-Rivera, Bonilla and Martínez-Palomo2007). In Acanthamoeba castellanii which is a free-living amoeba, the cyst wall is a double-layered structure which includes the ectocyst and endocyst (Chávez-Munguía et al., Reference Chávez-Munguía, Omaña-Molina, González-Lázaro, González-Robles, Cedillo-Rivera, Bonilla and Martínez-Palomo2007) which is not seen in D. fragilis. The cyst wall of D. fragilis was different to the precyst stage described in Histomonas meleagridis which is a close relative of D. fragilis (Barratt et al., Reference Barratt, Harkness, Marriott, Ellis and Stark2011) where a fibrillar-dense cyst wall was absent instead it contained only an outer amorphous zone (Zaragatzki et al., Reference Zaragatzki, Hess, Grabensteiner, Abdel-Ghaffar, Al-Rasheid and Mehlhorn2010).
Immediately beneath the cyst wall as well as inside the peritrophic space, there were numerous double-membrane vesicles which are similar to the ESVs described in Giardia cysts (Chávez-Munguía et al., Reference Chávez-Munguía, Omaña-Molina, González-Lázaro, González-Robles, Cedillo-Rivera, Bonilla and Martínez-Palomo2007). Some of these vesicles were also seen embedded in the cyst wall. It has been suggested that in Giardia cysts, these ESVs contain material that forms the fibrillar component of the cyst wall (Reiner et al., Reference Reiner, McCaffery and Gillin1990). These ESVs were described as spherical and electron dense in Giardia which contain condensed material that goes on to form the cyst wall (Hehl and Marti, Reference Hehl and Marti2004). Formation and maturation of large ESVs (up to 1 μm in diameter) is functionally linked to the regulation of cyst wall proteins and evidence suggests that ESVs represent a novel Golgi equivalent in Giardia (Hehl et al., Reference Hehl, Marti and Köhler2000; Marti et al., Reference Marti, Li, Schraner, Wild, Köhler and Hehl2003). In Entamoeba species, similar forms of small vesicles are present close to the plasma membrane which is involved in a process of depositing granular material to the cell wall (Chávez-Munguía and Martínez-Palomo, Reference Chávez-Munguía and Martínez-Palomo2011). In Acanthamoeba, vesicles with a similar appearance are present in the cytoplasm of mature cysts and the fibrillar content inside these vesicles resembles the appearance of the endocyst and ectocyst wall (Chávez-Munguía et al., Reference Chávez-Munguía, Omaña-Molina, González-Lázaro, González-Robles, Bonilla and Martínez-Palomo2005). Overall, we speculate that ESVs are present in the D. fragilis cyst. We also speculate that the mechanisms of cyst wall formation are probably Giardia-like, mainly because of the morphological similarities seen by TEM between this species and D. fragilis.
The enclosed parasite within the cyst wall was amoeboid in appearance. There were small spherical particles that appeared across the cytoplasm which are deemed to be free ribosomes. These are also apparent in Histomonas pseudocysts (Mielewczik et al., Reference Mielewczik, Mehlhorn, Al-Quraishy, Grabensteiner and Hess2008). The ER was scattered throughout the cytoplasm and around the nuclear region and glycogen granules were present which has also been described in H. meleagridis (Munsch et al., Reference Munsch, Lotfi, Hafez, Al-Quraishy and Mehlhorn2009). Although the nuclear membrane was often not visible, a clear nucleolus was present at the centre of the nucleus.
Hydrogenosomes are found in several trichomonad species and other anaerobic protozoa living in oxygen-poor environments. The hydrogenosome contains enzymes that participate in pyruvate metabolism and formation of ATP (Benchimol, Reference Benchimol2009). The gene for pyruvate : ferredoxin oxidoreductase was identified in the transcriptome of D. fragilis along with other hydrogenosomal ATP synthases (Barratt et al., Reference Barratt, Cao, Stark and Ellis2015). Therefore, the hydrogenosomes are the main energy-producing organelles in D. fragilis cysts. Hydrogenosomes were present in all the cysts observed. Some of these were spherical in shape which was similar to those seen in the trophozoite stage (Banik et al., Reference Banik, Birch, Stark and Ellis2012). In some cysts, hydrogenosomes were irregular in shape (‘cauliflower-like’) with irregular inclusions. These irregular-shaped hydrogenosomes do not appear to have been reported before in any other protozoa. Other irregular hydrogenosomes have been identified in Tritrichomonas foetus, having a ‘heart’-shaped morphology (Benchimol, Reference Benchimol2009). We suggest the unusual appearance of the hydrogenosomes we describe is linked to a reorganization of membranous structures that occur during cyst development or a division process similar to that described in Benchimol (Reference Benchimol2009).
A basal body structure containing an axostyle and costa was observed in D. fragilis trophozoites. Additionally, the cyst of D. fragilis contains a pelta and flagella which were not found in the trophozoite (Banik et al., Reference Banik, Birch, Stark and Ellis2012). Previous phylogenetic studies resulted in the inclusion of D. fragilis in the Parabasalia purely on the presence of hydrogenosomes (Cepicka et al., Reference Cepicka, Hampl and Kulda2010; Silberman et al., Reference Silberman, Clark and Sogin1996). The loss of the pelta axostyle complex was seen as a derived trait. The results presented here and in Munasinghe et al. (Reference Munasinghe, Vella, Ellis, Windsor and Stark2013) of the presence of internal flagella and pelta in the encysted parasite are therefore important. The pelta is a crescent-shaped sheet which could be found along the periphery of the nucleus (Munasinghe et al., Reference Munasinghe, Vella, Ellis, Windsor and Stark2013). The pelta apparently supports the periflagellar canal from which the flagella emerge in the trichomonads (Benchimol et al., Reference Benchimol, Diniz and Ribeiro2000). The costa is found only in trichomonads which possess an undulating membrane and it is assumed that its function is to provide mechanical support to the undulating membrane (Benchimol, Reference Benchimol and de Souza2010). Although the costa and pelta structure are visible in D. fragilis, no external flagella, undulating membrane or axonemes are seen in the peritrophic space. Therefore, although these structures may be remnants derived from an evolutionary ancestor, they do provide further ultrastructural evidence for D. fragilis to be classified as a trichomonad. In the H. meleagridis life cycle, however, an amoeboid stage, cyst-like stage and a flagellated stage have been identified (Munsch et al., Reference Munsch, Lotfi, Hafez, Al-Quraishy and Mehlhorn2009). As D. fragilis is a close relative of Histomonas sp., we wonder whether there is a further life cycle stage of D. fragilis waiting to be discovered.
During encystment, many biochemical and cellular changes occur in the cell including the production of a cyst wall (Chávez-Munguía et al., Reference Chávez-Munguía, Omaña-Molina, González-Lázaro, González-Robles, Cedillo-Rivera, Bonilla and Martínez-Palomo2007). In E. invadens, Giardia and Acanthamoeba species that have been studied extensively, encystation can be divided into several phases. The observations reported here suggest that D. fragilis undergoes similar reorganization of its internal structures. For example, the loss of the nuclear membranes and the presence of hydrogenosomes with unusual shape and structure suggest that cyst formation involves extensive cellular remodelling, which includes reorganization of membrane-bound organelles. In Giardia cysts, a similar process of remodelling occurs where the nuclei membranes fuse and disappear and exchange genetic information during encystation (Carpenter et al., Reference Carpenter, Assaf, Gourguechon and Cande2012). We presume similar processes may be occurring in D. fragilis.
The identification of cysts containing 1 or 2 nuclei suggests the cyst probably contains a single cell. The most prominent change that occurs during the encystation of protozoan parasites is the synthesis of cyst wall components and their transport to the cell membrane via ESVs which release their contents to the cell surface through exocytosis (Chávez-Munguía et al., Reference Chávez-Munguía, Cristóbal-Ramos, González-Robles, Tsutsumi and Martínez-Palomo2003). ESVs do not occur in non-encysting trophozoites of Giardia (Benchimol, Reference Benchimol2002). In Giardia, ESVs fuse with the plasma membrane and release their contents to the exterior leaving behind empty vesicles that are found in the peritrophic space (Hehl and Marti, Reference Hehl and Marti2004). In D. fragilis, numerous ESV-like structures were present in the peritrophic space. These preliminary observations of a cyst wall and the presence of ESV-like structures suggest a mechanism for cyst wall formation in D. fragilis similar to that in Giardia.
Conclusion
The current study demonstrated that D. fragilis trophozoites cannot withstand extreme pH conditions present in the human stomach, showing that fecal–oral transmission via the trophozoite to humans is possible but improbable. This highlights the need for further studies into both the cyst stage and Enterobius vermicularis ova vector models of transmission. Cyst forms are the dominant morphological form found in experimentally infected rodent feces; it is possible that the cyst form plays an important role in the zoonotic transmission. The transmission electron microscopic studies revealed the close similarity of the cysts ultrastructure of D. fragilis and other trichomonads, especially Histomonas which further highlights the presence of a cyst stage in the life cycle. All these findings have important implications for dientamoebiasis and advance our knowledge on the recurrent nature and transmission of the disease.
Supplementary material
The supplementary material for this article can be found at https://doi.org/10.1017/S0031182024000076
Data availability statement
The authors confirm that the data supporting the findings of this study are available within the article and its supplementary materials.
Acknowledgements
We thank Debra Birch (Macquarie University) for her advice and assistance in specimen processing and image interpretation. This work was supported by Sydpath, St. Vincent Hospital, Darlinghurst and the University of Technology Sydney. This research was performed by L. M. H. and V. S. M. in partial fulfilment of a PhD at UTS. The graphical abstract was created in BioRender under agreement number ED26486CAW.
Author contributions
L. M. H.: methodology, data curation, investigation, writing – original draft, review and editing. V. S. M.: conceptualization, investigation, writing – original draft. N. G. F. V.: investigation, supervision. J. T. E.: conceptualization, methodology, supervision, writing – review and editing. D. S.: conceptualization, supervision, writing, resources
Financial support
The research was supported by St Vincent's Hospital, SydPath, Department of Microbiology.
Competing interest
None.
Ethical standards
These experiments were completed to the standards of the Australian Research Council Codes and Guidelines. HREC approval was obtained through the HREC at St Vincent's Hospital Sydney for the establishment of cultures from clinical specimens. ACEC approval was obtained through UTS.