Summation
The search identified studies that evaluated the rules of pharmacological and genetic modulation of CB1 and CB2 cannabinoid receptors in the regulation of psychostimulant responses. Most studies demonstrated that activation of CB2R and inhibition of CB1R inhibited behavioural and molecular effects induced by distinct psychostimulants.
Considerations
Although studies reported that blockade of CB1R inhibits psychostimulant effects, the incidence of serious psychiatric adverse events, limits the use of selective CB1R antagonists for treating psychostimulant addiction disorders. Drugs targeting CB2R signalling might represent a more promising approach.
Moreover, preclinical studies only have focused on male mice and rats, excluding female animals. As sexual dimorphism has been demonstrated in behavioural and molecular responses correlated to cannabinoid receptors, the role of CB1R and CB2R in regulation of psychostimulants in female animals should be explored in future studies.
Introduction
Psychostimulants are a broad class of drugs that englobe cocaine, amphetamine, and its derivatives [i.e., methamphetamine, N-methyl- 3,4-methylenedioxymethamphetamine (MDMA)]. Psychostimulant addiction is a public health issue that substantially contributes to the global burden of disease (UNODC, 2021). This chronic pathology is characterised by complex behavioural and neurobiological phenomena entailing the compulsive use of a substance (Wise and Koob, Reference Wise and Koob2014, Volkow and Morales, Reference Volkow and Morales2015). The mechanisms for the addictive properties of drugs, including psychostimulants, involves the facilitation of reward centres in the brain, particularly the mesocorticolimbic dopaminergic pathways connecting the ventral tegmental area (VTA) with various limbic structures, such as nucleus accumbens (NAcc), prefrontal cortex (PFC), and hippocampus (Koob and Volkow, Reference Koob and Volkow2010). Acute and chronic exposure to psychostimulants cause both transient and persistent adaptations in regions of the mesocorticolimbic neurocircuitry, resulting in altered behavioural responses, ultimately, leading to drug addiction (Rothman and Baumann, Reference Rothman and Baumann2003, Howell and Kimmel, Reference Howell and Kimmel2008).
Several pieces of evidence show that the endocannabinoid system modulates the reward-related effects of dopamine and that this system might be involved in the neurobiological mechanism underlying psychostimulant addiction (Wiskerke et al., Reference Wiskerke, Pattij, Schoffelmeer and De Vries2008, Manzanares et al., Reference Manzanares, Cabanero, Puente, Garcia-Gutierrez, Grandes and Maldonado2018). The endocannabinoid system comprises the endogenous ligands anandamide (AEA) and 2-arachidonoylglycerol (2-AG), the enzymes responsible for their synthesis and degradation and the cannabinoid receptors (Hillard, Reference Hillard2015). AEA and 2-AG are the main endocannabinoids, whose effects are mediated mainly by two metabotropic receptor termed CB1R and CB2R (Hillard, Reference Hillard2015, Lu and Mackie, Reference Lu and Mackie2016). Both cannabinoid receptors are expressed in mesolimbic pathways and can modulate excitability of dopaminergic neurons (Howlett et al., Reference Howlett, Bidaut-Russell, Devane, Melvin, Johnson and Herkenham1990, Onaivi et al., Reference Onaivi, Ishiguro, Gong, Patel, Perchuk, Meozzi, Myers, Mora, Tagliaferro, Gardner, Brusco, Akinshola, Liu, Hope, Iwasaki, Arinami, Teasenfitz and Uhl2006, Covey et al., Reference Covey, Mateo, Sulzer, Cheer and Lovinger2017). In accordance with their localisation, accumulating reports have pointed to the involvement of CB1R and CB2R in the main behavioural effects of psychostimulants (Wiskerke et al., Reference Wiskerke, Pattij, Schoffelmeer and De Vries2008, Vlachou and Panagis, Reference Vlachou and Panagis2014). Moreover, cannabinoid receptors also display an important role in regulating molecular responses of these drugs (Wiskerke et al., Reference Wiskerke, Pattij, Schoffelmeer and De Vries2008, Vlachou and Panagis, Reference Vlachou and Panagis2014, Parsons and Hurd, Reference Parsons and Hurd2015). Interestingly, these studies suggest that cannabinoid receptors work with opposing functions to modulate certain behavioural- and molecular-related effects of these drugs, since an activation of CB2R leads to similar results as compared to CB1R blockade.
The focus of the present review is to discuss the distinct behavioural responses induced by psychostimulants and how a differential modulation of CB1R and CB2R can regulate them. The molecular mechanisms through which CB1R and CB2R change the neuroadaptations promoted by psychostimulants will also be explored. Finally, we will propose an overarching hypothesis integrating both receptors in the pharmacological modulation of psychostimulant effects.
Psychostimulants: mechanisms of action and behavioural responses
Psychostimulants are a broad class of psychotropic substances with the capacity to stimulate various functions of the central nervous system, including attention, vigilance, alertness, arousal, and locomotion (Favrod-Coune and Broers, Reference Favrod-Coune and Broers2010). Intense hedonic feelings characterised as a “rush” are also described (Boutrel and Koob, Reference Boutrel and Koob2004, McCreary et al., Reference Mccreary, Muller and Filip2015). Most of them act by directly facilitating mesocorticolimbic terminals by either inhibiting the dopamine transporter (DAT) or facilitating the release of dopamine (Harris and Baldessarini, Reference Harris and Baldessarini1973, Nestler, Reference Nestler2004). While cocaine binds predominantly to DAT and inhibits dopamine reuptake, amphetamine has two more complementary action mechanisms to elevate dopamine levels (Sulzer et al., Reference Sulzer, Sonders, Poulsen and Galli2005). This drug can act by reversing the vesicular monoamine transporter, leading to a large release of the cytoplasmic and vesicular stores of dopamine (Robertson et al., Reference Robertson, Matthies and Galli2009). An additional mechanism of action for amphetamines is the facilitation of the output of dopamine from vesicles into the cytoplasm (Sulzer et al., Reference Sulzer, Sonders, Poulsen and Galli2005). Both mechanisms also lead to an enhanced dopaminergic signalling in the mesocorticolimbic circuitry.
The use of experimental animal models is an important strategy to obtain direct insights into the molecular and behavioural effects promoted by psychostimulants (McCreary et al., Reference Mccreary, Muller and Filip2015). Administration of cocaine and amphetamine in laboratory animals induces a dose-dependent increase in locomotor activity. Moreover, repeated exposure to these drugs leads to the development of behavioural sensitisation, which is characterised by a progressively increasing behavioural response to repeated drug exposure (Sanchis-Segura and Spanagel, Reference Sanchis-Segura and Spanagel2006, Steketee and Kalivas, Reference Steketee and Kalivas2011). This phenomenon is observed, for example, as an increase in locomotor activity, which becomes even more pronounced in animals previously exposed to single or repeated administration of the same psychostimulant (Shuster et al., Reference Shuster, Yu and Bates1977, Steketee and Kalivas, Reference Steketee and Kalivas2011).
The rewarding properties of cocaine and amphetamine are largely assessed using the conditioned place preference (CPP) paradigm (Bardo and Bevins, Reference Bardo and Bevins2000, Wiskerke et al., Reference Wiskerke, Pattij, Schoffelmeer and De Vries2008). CPP is generally performed in a box containing two distinct compartments with different contextual cues, which are equally explored by the rodents in a pre-test session. The conditioning phase is performed by administering the psychostimulants and keeping the animal confined to one compartment (the conditioned stimulus), whereas vehicle injection is paired with the other compartment, in alternate periods. After the conditioning phase, the test session is performed in the absence of the drug, and the animals can explore both sides of the box. An increase in the time exploring the drug-paired compartment, compared to time spent in the vehicle-paired side, is suggestive of the rewarding effect of the drugs (Bardo and Bevins, Reference Bardo and Bevins2000, Sanchis-Segura and Spanagel, Reference Sanchis-Segura and Spanagel2006).
Drug self- administration has been one of the most direct approaches to study the rewarding properties of cocaine and amphetamine in experimental animals (Gardner, Reference Gardner2000). In this behavioural model, rodents are trained to perform an operant response (e.g., a lever press or nose poke) for an infusion of drug, typically accompanied by a concurrently-delivered, discrete cue such as a light and a tone. Different kinds of schedule might be used to obtain the drug, being the most largely used the fixed ratio (FR) schedule and progressive ratio (PR) schedule (Gardner, Reference Gardner2000, Farrell et al., Reference Farrell, Schoch and Mahler2018). Briefly, under a FR schedule, the psychostimulant is delivered every time that a pre-selected number of responses are completed. Conversely, under a PR schedule, the required ratio increases following a predefined progression, which usually is an arithmetic one. Breakpoints in this schedule, which reflects the motivation for the drug, can be defined as the maximum response rate achieved to obtain a single infusion of psychostimulant before the animal fails to complete the next ratio requirement (Gardner, Reference Gardner2000, Panagis et al., Reference Panagis, Mackey and Vlachou2014).
Both CPP and self-administration paradigms can be used to assess relapse, another important property of psychostimulants. Once self-administration and place preference behaviours are established, animals undergo extinction training during which they are re-exposed to the drug environment in the absence of psychostimulants. After these extinction processes, animals can be tested for reinstatement, which are often precipitated by exposure to a small priming dose of drug, experiencing acute stress, or encountering discrete or contextual cues previously paired with drug use (Shaham et al., Reference Shaham, Shalev, Lu, De Wit and Stewart2003, Farrell et al., Reference Farrell, Schoch and Mahler2018).
Over the years, the use of preclinical models has helped to elucidate the cellular and molecular aspects regarding the neurobiology of psychostimulant drugs, as well as new potential strategies for the pharmacological modulation of psychostimulant actions including, compounds targeting CB1R and CB2R.
Overview of CB1R and CB2R
Cannabis is one of the first plants to be used as a medicine and a drug of abuse by the humankind (Zuardi, Reference Zuardi2006). This plant is the source of a set of more than 100 compounds, among which is Δ9-tetrahydrocannabinol (Δ9-THC), the main responsible for the psychoactive effects of the plant (Mechoulam and Hanus, Reference Mechoulam and Hanus2000, Hanus et al., Reference Hanus, Meyer, Munoz, Taglialatela-Scafati and Appendino2016). After the identification of Δ9-THC, researchers focused their efforts in elucidating the pharmacological mechanism underlying its effects (Mechoulam and Hanus, Reference Mechoulam and Hanus2000, Pertwee, Reference Pertwee2006). Complementary studies with Δ9-THC synthetic derivatives, including radioactive ligands, provided convincing evidence regarding the existence of specific cannabinoid receptors (Devane et al., Reference Devane, Dysarz, Johnson, Melvin and Howlett1988, Pertwee, Reference Pertwee2006). Currently, two major types of receptors have been characterised and cloned, CB1R and CB2R (Devane et al., Reference Devane, Dysarz, Johnson, Melvin and Howlett1988, Munro et al., Reference Munro, Thomas and Abu-Shaar1993, Pertwee, Reference Pertwee2010).
The CB1R is one of the most abundant Gi protein alpha subunit (Gi/o) protein-coupled receptors in the brain (Howlett et al., Reference Howlett, Barth, Bonner, Cabral, Casellas, Devane, Felder, Herkenham, Mackie, Martin, Mechoulam and Pertwee2002). The activation of these presynaptic receptors leads to inhibition of neurotransmitter release by a mechanism that involves inhibition of voltage-gated calcium (Ca2+) channels and activation of inwardly rectifying potassium (K+) channels via the stimulated cyclic adenosine monophosphate-protein kinase A (cAMP-PKA) signal pathway (Kano, Reference Kano2014, Howlett and Abood, Reference Howlett and Abood2017). CB1R expression was described in distinct regions of mesocorticolimbic dopaminergic pathways, including hippocampus, PFC, and NAcc (Howlett et al., Reference Howlett, Barth, Bonner, Cabral, Casellas, Devane, Felder, Herkenham, Mackie, Martin, Mechoulam and Pertwee2002). These regions are involved in motivational and reward processes, which are modulated by endogenous and exogenous CB1R ligands (Koob and Volkow, Reference Koob and Volkow2010, Wenzel and Cheer, Reference Wenzel and Cheer2018).
Regarding the CB2R, early evidence suggested that this receptor might be absent in brain and restricted to peripheral tissues. However, after the development of more selective and sensitive tools, it was possible to identify CB2R in the central nervous system (Van Sickle et al., Reference Van Sickle, Duncan, Kingsley, Mouihate, Urbani, Mackie, Stella, Makriyannis, Piomelli, Davison, Marnett, Di Marzo, Pittman, Patel and Sharkey2005, Onaivi et al., Reference Onaivi, Ishiguro, Gong, Patel, Perchuk, Meozzi, Myers, Mora, Tagliaferro, Gardner, Brusco, Akinshola, Liu, Hope, Iwasaki, Arinami, Teasenfitz and Uhl2006). Indeed, CB2R is distributed extensively in different brain areas, such as hippocampus, PFC, amygdala, olfactory nucleus, striatum, and thalamus (Chen et al., Reference Chen, Gao, Gao, Su and Wu2017). The CB2R shares 44% homology with the CB1R and also is coupled to Gi/o protein (Howlett et al., Reference Howlett, Barth, Bonner, Cabral, Casellas, Devane, Felder, Herkenham, Mackie, Martin, Mechoulam and Pertwee2002). CB2R also modulates the activity of Ca2+ and K+ channels, and recent electrophysiological and biochemistry findings confirmed the functionality of these receptors in mesocorticolimbic pathways (Zhang et al., Reference Zhang, Gao, Liu, Bi, Li, Yang, Gardner, Wu and Xi2014, Howlett and Abood, Reference Howlett and Abood2017, Jordan and Xi, Reference Jordan and Xi2019).
This convergence of cannabinoid receptors in the central nervous system, especially in mesolimbic circuitry, is consistent with the reward effects of synthetic and natural cannabinoids (Gessa et al., Reference Gessa, Melis, Muntoni and Diana1998, Zhang et al., Reference Zhang, Gao, Liu, Bi, Li, Yang, Gardner, Wu and Xi2014, Li et al., Reference Li, Hempel, Yang, Han, Bi, Gardner and Xi2021). In addition, CB1R and CB2R are crucial mediators of synaptic plasticity in mesolimbic pathways, an important component in the control of motivated behaviour promoted by drugs that promote addiction (Xi et al., Reference Xi, Peng, Li, Song, Zhang, Liu, Yang, Bi, Li and Gardner2011, Garcia-Gutierrez et al., Reference Garcia-Gutierrez, Ortega-Alvaro, Busquets-Garcia, Perez-Ortiz, Caltana, Ricatti, Brusco, Maldonado and Manzanares2013, Zlebnik and Cheer, Reference Zlebnik and Cheer2016). Therefore, CB1R and CB2R not only underlie the rewarding effects of cannabis, but can also interact with other drugs of abuse, including psychostimulants (Wiskerke et al., Reference Wiskerke, Pattij, Schoffelmeer and De Vries2008, Zhang et al., Reference Zhang, Gao, Liu, Bi, Li, Yang, Gardner, Wu and Xi2014).
Role of CB1R in psychostimulant responses
Activation of CB1R is essential for the establishment of addiction to cannabinoid drugs (Wenzel and Cheer, Reference Wenzel and Cheer2018). Moreover, both genetic and pharmacological approaches strongly suggest a role for CB1R signalling on responses to other drugs of abuse, including psychostimulants (Wiskerke et al., Reference Wiskerke, Pattij, Schoffelmeer and De Vries2008).
CB1R and psychostimulant motor effects
Acute administration of cocaine or amphetamine induces a robust increase in locomotor activity (hyperlocomotion) in animals exposed to an open field. Administration of rimonabant, a CB1R antagonist/inverse agonist, dose-dependently inhibits the hyperlocomotion induced by d-amphetamine and cocaine in rodents previously exposed to the open field (Poncelet et al., Reference Poncelet, Barnouin, Breliere, Le Fur and Soubrie1999, Gobira et al., Reference Gobira, Oliveira, Gomes, Da Silveira, Asth, Bastos, Batista, Issy, Okine, De Oliveira, Ribeiro, Del Bel, Aguiar, Finn and Moreira2019). A similar effect has been observed after pharmacological blockade of CB1R by AM251, a more selective CB1R antagonist/inverse agonist (Corbille et al., Reference Corbille, Valjent, Marsicano, Ledent, Lutz, Herve and Girault2007). Accordingly, locomotor responses to cocaine were also significantly reduced in CB1R knockout mice (Li et al., Reference Li, Hoffman, Peng, Lupica, Gardner and Xi2009).
Converging evidence also supports that blockade of CB1R regulates behavioural sensitisation induced by psychostimulants. The development of single-trial cocaine- and amphetamine-induced locomotor sensitisation was impaired in CB1R knockout (KO) mice or after CB1R pharmacological blockade in wild-type mice (Corbille et al., Reference Corbille, Valjent, Marsicano, Ledent, Lutz, Herve and Girault2007, Mereu et al., Reference Mereu, Tronci, Chun, Thomas, Green, Katz and Tanda2015, Delis et al., Reference Delis, Polissidis, Poulia, Justinova, Nomikos, Goldberg and Antoniou2017, Lopes et al., Reference Lopes, Bastos, Costa, Aguiar and Moreira2019). The sensitised locomotor response to a single cocaine challenge was also reduced in rats pretreated with rimonabant (Filip et al., Reference Filip, Golda, Zaniewska, Mccreary, Nowak, Kolasiewicz and Przegalinski2006). These pharmacological studies evaluated the expression of psychostimulant locomotor sensitisation, since the blockade of CB1R was performed before the cocaine challenge. By injecting the CB1R antagonism on the first day of test, a recent work demonstrated that the acquisition of motor sensitisation also was impaired by blockade of these receptors (Lopes et al., Reference Lopes, Bastos, Costa, Aguiar and Moreira2019).
Despite this evidence, other studies have demonstrated that neither genetic silencing nor pharmacological inhibition of CB1R altered psychostimulant ability to induce motor sensitisation (Martin et al., Reference Martin, Ledent, Parmentier, Maldonado and Valverde2000, Lesscher et al., Reference Lesscher, Hoogveld, Burbach, Van Ree and Gerrits2005). In addition to distinctions in animal species and strains, these discrepancies might result from differences in the dose of psychostimulant and number of injections during acquisition phase (single or repeated drug injection). The context of CB1R antagonist administration also appears to be important in the regulation of behavioural sensitisation. For instance, Gerdeman and co-workers observed that rimonabant did not diminish the established cocaine sensitisation if delivered in the home cage, but only if the rimonabant-injected mice were exposed to activity chambers previously paired with cocaine (Gerdeman et al., Reference Gerdeman, Schechter and French2008).
In accordance with behavioural responses, CB1R also appears to be relevant in psychostimulant-activated signalling pathways. cAMP-dependent phosphorylation of glutamate receptor 1, promoted by cocaine, was altered in the striatum of CB1R -null mice (Corbille et al., Reference Corbille, Valjent, Marsicano, Ledent, Lutz, Herve and Girault2007). Moreover, phosphorylation of extracellular signal-regulated kinase (ERK) promoted by cocaine and D-amphetamine were prevented in the dorsal striatum, as well as in the NAcc core and shell of CB1R mutant mice (Corbille et al., Reference Corbille, Valjent, Marsicano, Ledent, Lutz, Herve and Girault2007). Corroborating these findings, blockade of CB1R prevented cocaine-induced increased in c-Fos expression in the shell and core portions of NAcc, and (Gobira et al., Reference Gobira, Oliveira, Gomes, Da Silveira, Asth, Bastos, Batista, Issy, Okine, De Oliveira, Ribeiro, Del Bel, Aguiar, Finn and Moreira2018). Altogether, these results provide evidences that CB1R is essential for biochemical responses to psychostimulants that are intrinsically correlated with locomotor behavioural effects.
CB1R and psychostimulant reward and reinforcement
The endocannabinoid signalling has also been implicated in the modulation of psychostimulant-induced reward, as evaluated in the CPP test. Administration of CB1R antagonist before cocaine or methamphetamine injections, in the conditioning phase, impaired dose-dependently the acquisition of CPP (Yu et al., Reference Yu, Zhou, Wang, Liu, Xue, Jiang and Lu2011, Delis et al., Reference Delis, Polissidis, Poulia, Justinova, Nomikos, Goldberg and Antoniou2017, Lopes et al., Reference Lopes, Bastos, Costa, Aguiar and Moreira2019). CB1R antagonist also decreased the expression of cocaine-induced CPP. Blockade of CB1R also prevented neuronal activation in the hippocampus of animals exposed to cocaine-CPP (Lopes et al., Reference Lopes, Bastos, Costa, Aguiar and Moreira2019). Interestingly, no effect was observed when the CB1R blockade was performed only on the test day (Chaperon et al., Reference Chaperon, Soubrie, Puech and Thiebot1998, Lopes et al., Reference Lopes, Bastos, Costa, Aguiar and Moreira2019). These data suggest that CB1R might be important in the consolidation of psychostimulant-paired memories, but is not involved in the retrieval of these memories (Lopes et al., Reference Lopes, Bastos, Costa, Aguiar and Moreira2019). Despite pharmacological findings indicating that CB1Rs are involved in psychostimulant reward memory, cocaine-induced CPP was unaffected in CB1R KO mice (Martin et al., Reference Martin, Ledent, Parmentier, Maldonado and Valverde2000, Houchi et al., Reference Houchi, Babovic, Pierrefiche, Ledent, Daoust and Naassila2005). These differences are not clear, but might be explained by compensatory changes in the CB1R KO mice, since CB1R is important during development for establishing proper neuronal connectivity in brain regions related to memory and reward (Berghuis et al., Reference Berghuis, Rajnicek, Morozov, Ross, Mulder, Urban, Monory, Marsicano, Matteoli, Canty, Irving, Katona, Yanagawa, Rakic, Lutz, Mackie and Harkany2007).
Intravenous drug self-administration is one of the most used approaches for studying drug reinforcement. Experiments using this paradigm also provide evidence that CB1Rs play a critical role in psychostimulant-induced reinforcing properties. A significant reduction in acquisition of cocaine self-administration was observed in CB1R KO mice compared with wild type (Soria et al., Reference Soria, Mendizabal, Tourino, Robledo, Ledent, Parmentier, Maldonado and Valverde2005). The number of sessions required to CB1R null mice to achieve this behaviour was increased (Soria et al., Reference Soria, Mendizabal, Tourino, Robledo, Ledent, Parmentier, Maldonado and Valverde2005). Pharmacological blockade of CB1R with SR141716A in wild-type mice promoted similar effects (Soria et al., Reference Soria, Mendizabal, Tourino, Robledo, Ledent, Parmentier, Maldonado and Valverde2005). Evidence also pointed that the maximal effort to obtain a cocaine infusion, in PR reinforcement schedule, was significantly reduced after the genetic and pharmacological ablation of CB1R (Soria et al., Reference Soria, Mendizabal, Tourino, Robledo, Ledent, Parmentier, Maldonado and Valverde2005, Xi et al., Reference Xi, Spiller, Pak, Gilbert, Dillon, Li, Peng and Gardner2008). Treatment with CB1R antagonists, in a dose-dependent manner, lowered the break point for cocaine self-administration under a PR reinforcement schedule in rats (Xi et al., Reference Xi, Spiller, Pak, Gilbert, Dillon, Li, Peng and Gardner2008). Similarly, the blockade of CB1R suppressed the intake of methamphetamine in rats trained to self-administer this drug (Vinklerova et al., Reference Vinklerova, Novakova and Sulcova2002).
Intriguingly, these reports are countered by studies which demonstrated that pharmacological and genetic inactivation of CB1R were ineffective to modulate cocaine and amphetamine self-administration under FR schedules (Cossu et al., Reference Cossu, Ledent, Fattore, Imperato, Bohme, Parmentier and Fratta2001, Lesscher et al., Reference Lesscher, Hoogveld, Burbach, Van Ree and Gerrits2005). Besides the differences in ratio schedule, the extension in the period of cocaine self-administration also appears to be important to the effect of CB1R in modulation of drug-intake. For example, blockade of CB1R reduces the breakpoint for cocaine self-administration in rats that had 6 h to access the drug. On the other hand, a lower efficacy of CB1R antagonist was observed in rats that access cocaine only 1 h daily (Orio et al., Reference Orio, Edwards, George, Parsons and Koob2009). In accordance with those findings, the levels of both phosphorylated and total CB1R protein were increased only in the NAcc of rats given extended daily access to cocaine (Orio et al., Reference Orio, Edwards, George, Parsons and Koob2009). In the extended access regimen, the intake of the drug gradually increases over days, on the other hand the consume of cocaine remains stable in animals under short access protocol (Ahmed and Koob, Reference Ahmed and Koob1998, Wee et al., Reference Wee, Specio and Koob2007). This escalated drug intake also is associated with increased breakpoints or responding for cocaine under a progressive ratio (PR) schedule of reinforcement, both processes that demonstrated pivotal role of CB1R in modulating psychostimulant behaviour (Wee et al., Reference Wee, Specio and Koob2007). Therefore, this evidence suggested that the capacity of CB1R to regulate the rewarding properties of psychostimulants might influence the motivation to obtain these drugs.
Increases in dopamine extracellular levels in the NAcc have been related to the primary reinforcing effects of psychostimulants (Di Chiara, Reference Di Chiara1998). Similar to observed in the modulation of self-administration, the importance of silencing of CB1R in regulation of levels of dopamine in the NAcc also appears to be complex. Striatal extracellular dopamine response to acute cocaine was reduced in CB1R KO mice (Li et al., Reference Li, Hoffman, Peng, Lupica, Gardner and Xi2009). A similar result was obtained after the pharmacological blockade of these receptors in wild-type mice (Li et al., Reference Li, Hoffman, Peng, Lupica, Gardner and Xi2009). Although this is consistent with findings that rimonabant inhibits cocaine- and amphetamine-induced dopamine release in rats (Cheer et al., Reference Cheer, Wassum, Sombers, Heien, Ariansen, Aragona, Phillips and Wightman2007, Covey et al., Reference Covey, Bunner, Schuweiler, Cheer and Garris2016), both basal and cocaine-induced increase in extracellular levels of dopamine in the NAcc were unaffected in CB1R KO mice (Soria et al., Reference Soria, Mendizabal, Tourino, Robledo, Ledent, Parmentier, Maldonado and Valverde2005) or after treatment with CB1R antagonist (Caille and Parsons, Reference Caille and Parsons2006). Differences in the genetic background of the KO animals and methods to evaluate dopamine levels might explain these discrepant results. For example, while no changes in cocaine-enhanced dopamine release were observed in KO mice from CD1 background (Soria et al., Reference Soria, Mendizabal, Tourino, Robledo, Ledent, Parmentier, Maldonado and Valverde2005), alterations in dopamine levels following cocaine injections were obtained in CB1R -null mice with a C57BL/6J genetic background (Li et al., Reference Li, Hoffman, Peng, Lupica, Gardner and Xi2009).
A recent study, using modern molecular tools to selectively ablate CB1R on specific subtypes of neurons, provided interesting novel insights that clarified the role of these receptors in the regulation of dopamine levels in the NAcc in animals submitted to self-administration paradigm. A lower training dose was required to acquire cocaine self-administration for the mutant mouse lines with CB1R deletion targeted in forebrain GABAergic (GABA-CB1-KO) neurons, suggesting an increased sensitivity to the aversive effect of high unit drug doses (Martin-Garcia et al., Reference Martin-Garcia, Bourgoin, Cathala, Kasanetz, Mondesir, Gutierrez-Rodriguez, Reguero, Fiancette, Grandes, Spampinato, Maldonado, Piazza, Marsicano and Deroche-Gamonet2016). Conversely, at low doses, GABA-CB1R-KO mice self-administered more than the wild type, confirming an increased sensitivity to the positive reinforcing effects of cocaine (Martin-Garcia et al., Reference Martin-Garcia, Bourgoin, Cathala, Kasanetz, Mondesir, Gutierrez-Rodriguez, Reguero, Fiancette, Grandes, Spampinato, Maldonado, Piazza, Marsicano and Deroche-Gamonet2016). A dopaminergic mechanism appears to be involved in this behavioural response, since naïve GABA-CB1R-KO mice showed increased cocaine-induced dopamine release in the NAcc (Martin-Garcia et al., Reference Martin-Garcia, Bourgoin, Cathala, Kasanetz, Mondesir, Gutierrez-Rodriguez, Reguero, Fiancette, Grandes, Spampinato, Maldonado, Piazza, Marsicano and Deroche-Gamonet2016). Authors also observed that silencing of cortical glutamatergic neurons did not change cocaine’s primary reinforcing effects as revealed by the similar dose-response curves for cocaine self-administration in this genotype compared to wild type (Martin-Garcia et al., Reference Martin-Garcia, Bourgoin, Cathala, Kasanetz, Mondesir, Gutierrez-Rodriguez, Reguero, Fiancette, Grandes, Spampinato, Maldonado, Piazza, Marsicano and Deroche-Gamonet2016).
Overall, blockade of CB1R might curb behavioural and dopaminergic responses correlated to psychostimulant reward. However, these effects are sensitive to variations in the experimental protocol, such as dose of psychostimulant, ratio schedule, and the extension in the period of drug self-administration. Moreover, recent evidence demonstrated that CB1R located on glutamatergic and in GABAergic neurons contribute differentially to the effect of psychostimulants.
CB1R and psychostimulant reinstatement
A major feature of psychostimulants use disorder is the risk of relapse in drug use even after long periods of withdrawal (Le Moal and Koob, Reference Le Moal and Koob2007, Wise and Koob, Reference Wise and Koob2014). Reinstatement episodes might be triggered by re-exposure to the drug itself or even to previously drug-associated contextual cues, as well as exposure to stressful stimuli (Shaham et al., Reference Shaham, Shalev, Lu, De Wit and Stewart2003, Steketee and Kalivas, Reference Steketee and Kalivas2011). De Vries and co-workers provided evidence for a pivotal role of CB1R signalling in psychostimulant reinstatement. They found that a single injection of the CB1R agonist HU-210 reinstated drug-seeking following the extinction of cocaine self-administration, an effect reversed by co-administration of a CB1R antagonist (De Vries et al., Reference De Vries, Shaham, Homberg, Crombag, Schuurman, Dieben, Vanderschuren and Schoffelmeer2001). The authors also showed that rimonabant by itself prevented drug-induced reinstatement of cocaine seeking (De Vries et al., Reference De Vries, Shaham, Homberg, Crombag, Schuurman, Dieben, Vanderschuren and Schoffelmeer2001). These findings were replicated by other studies using AM251 (Xi et al., Reference Xi, Gilbert, Peng, Pak, Li and Gardner2006, Adamczyk et al., Reference Adamczyk, Miszkiel, Mccreary, Filip, Papp and Przegalinski2012) and ORG 27,569, a CB1R negative allosteric modulator (Jing et al., Reference Jing, Qiu, Zhang and Li2014). Similarly, methamphetamine- and MDMA-induced reinstatement were prevented by both CB1R antagonism and by allosteric modulation of these receptors (Jing et al., Reference Jing, Qiu, Zhang and Li2014, Nawata et al., Reference Nawata, Kitaichi and Yamamoto2016). Accordingly, CB1R antagonism also impaired cocaine and methamphetamine-induced reinstatement in the CPP paradigm (Yu et al., Reference Yu, Zhou, Wang, Liu, Xue, Jiang and Lu2011).
CB1R has also been found to play a critical role in mediating reinstatement of previously extinguished drug-seeking behaviour upon re-exposure to the drug-associated cues. The increase in operant self-administration response induced by re-exposure to cues previously paired with methamphetamine, MDMA, and cocaine infusion was blocked by CB1R antagonist (Anggadiredja et al., Reference Anggadiredja, Nakamichi, Hiranita, Tanaka, Shoyama, Watanabe and Yamamoto2004, Ward et al., Reference Ward, Rosenberg, Dykstra and Walker2009, Adamczyk et al., Reference Adamczyk, Miszkiel, Mccreary, Filip, Papp and Przegalinski2012, Nawata et al., Reference Nawata, Kitaichi and Yamamoto2016). Reinstatement to psychostimulant-seeking induced by different types of stressors was also inhibited by blockade of CB1R. For instance, forced swim or restraint stress-induced reinstatement of extinguished cocaine-CPP was suppressed by systemic CB1R antagonism (Vaughn et al., Reference Vaughn, Mantsch, Vranjkovic, Stroh, Lacourt, Kreutter and Hillard2012, Tung et al., Reference Tung, Lu, Lee, Yu, Lee, Leishman, Bradshaw, Hwang, Hung, Mackie, Zimmer and Chiou2016, Guzman et al., Reference Guzman, Avalos, De Giovanni, Euliarte, Sanchez, Mongi-Bragato, Rigoni, Bollati, Virgolini and Cancela2021). Moreover, restraint stress-induced cocaine seeking was not observed in CB1R-deficient mice (Tung et al., Reference Tung, Lu, Lee, Yu, Lee, Leishman, Bradshaw, Hwang, Hung, Mackie, Zimmer and Chiou2016). Reinstatement to cocaine-seeking promoted by injection of pharmacological stressor corticotrophin-releasing factor also was prevented by blockade of CB1R (Kupferschmidt et al., Reference Kupferschmidt, Klas and Erb2012). The exposure to various types of stress events potentiated other relapse-promoting stimuli (e.g., cues, drug re-exposure), augmenting their proneness to elicit drug seeking (Mantsch et al., Reference Mantsch, Baker, Funk, Le and Shaham2016, McReynolds et al., Reference Mcreynolds, Doncheck, Li, Vranjkovic, Graf, Ogasawara, Cravatt, Baker, Liu, Hillard and Mantsch2018). CB1R played an important role in both stress- and drug-induced reinstatement, blockade of these receptors prevented the ability of stress to potentiate low-dose cocaine-induced reinstatement (McReynolds et al., Reference Mcreynolds, Doncheck, Vranjkovic, Ganzman, Baker, Hillard and Mantsch2016). In addition, a similar modulatory role of CB1R has consistently been found with respect to cocaine- and amphetamine reinstatement induced by exposure to cues previously associated with these drugs.
Although it is recognised that CB1Rs are important to the behavioural effects of psychostimulants-seeking, few studies have focused on understanding the neural substrates involved in these processes. The NAcc is an important neuroanatomical locus of the reinstatement-preventing effects of CB1R antagonists. Local injections of this CB1R antagonist into the NAcc inhibited cocaine-induced reinstatement of drug-seeking behaviour (Xi et al., Reference Xi, Gilbert, Peng, Pak, Li and Gardner2006). The antagonism of CB1R in the portion core of the NAcc, but not in the shell, dose-dependently prevented restraint stress-induced reinstatement of cocaine-CPP, while activation of CB1R potentiated this behaviour (Guzman et al., Reference Guzman, Avalos, De Giovanni, Euliarte, Sanchez, Mongi-Bragato, Rigoni, Bollati, Virgolini and Cancela2021). Alteration of glutamate release within NAcc appears to be involved in these effects of CB1R, since pharmacological modulation of CB1R in the NAcc regulates extracellular levels of this neurotransmitter under cocaine-reinstatement conditions (Xi et al., Reference Xi, Gilbert, Peng, Pak, Li and Gardner2006, Guzman et al., Reference Guzman, Avalos, De Giovanni, Euliarte, Sanchez, Mongi-Bragato, Rigoni, Bollati, Virgolini and Cancela2021). CB1R expressed in VTA and in prelimbic (PL) cortex also appear to be involved on stress-induced cocaine reinstatement. CB1R antagonist microinjected bilaterally into the VTA inhibited the capacity of the restraint stress to reinstate extinguished cocaine CPP (Tung et al., Reference Tung, Lu, Lee, Yu, Lee, Leishman, Bradshaw, Hwang, Hung, Mackie, Zimmer and Chiou2016). The activation of CB1R inhibits GABA release leading to VTA dopaminergic disinhibition and reinstatement of cocaine CPP (Tung et al., Reference Tung, Lu, Lee, Yu, Lee, Leishman, Bradshaw, Hwang, Hung, Mackie, Zimmer and Chiou2016). Regarding PL, both stress- and corticosterone-potentiated cocaine reinstatement were prevented by intra-PL administration of the CB1R antagonist in this region (McReynolds et al., Reference Mcreynolds, Doncheck, Li, Vranjkovic, Graf, Ogasawara, Cravatt, Baker, Liu, Hillard and Mantsch2018). Similarly, to observed in the VTA, a CB1R-dependent attenuation of GABAergic neurotransmission in the PL seems to be involved in this process (McReynolds et al., Reference Mcreynolds, Doncheck, Li, Vranjkovic, Graf, Ogasawara, Cravatt, Baker, Liu, Hillard and Mantsch2018).
In summary, although the effect of CB1R blockade in modulation of psychostimulant reward is still controversy, more robust behavioural and molecular evidence indicate that CB1R is a required element in the ability of drug, stress and cue to reinstate psychostimulants seekingbehaviour.
Role of CB2R in psychostimulant responses
Early evidence suggested that expression of CB2R could be absent in encephalic structures and restricted to peripheral tissues (Munro et al., Reference Munro, Thomas and Abu-Shaar1993). More recently, their expression and function were detected in the brain through molecular, genetic, behavioural, and pharmacological approaches (Gong et al., Reference Gong, Onaivi, Ishiguro, Liu, Tagliaferro, Brusco and Uhl2006, Jordan and Xi, Reference Jordan and Xi2019). Among other regions, CB2Rs have been identified in the cell bodies of dopaminergic neurons in mesocorticolimbic pathway, indicating that these receptors might modulate the effects of psychostimulant drugs (Gong et al., Reference Gong, Onaivi, Ishiguro, Liu, Tagliaferro, Brusco and Uhl2006, Chen et al., Reference Chen, Gao, Gao, Su and Wu2017). Indeed, the presence of the CB2R in mesocorticolimbic neurocircuitry is in conformity with findings that modulation of these receptors regulates behavioural and molecular responses to cocaine and amphetamine (Xi et al., Reference Xi, Peng, Li, Song, Zhang, Liu, Yang, Bi, Li and Gardner2011, Canseco-Alba et al., Reference Canseco-Alba, Schanz, Sanabria, Zhao, Lin, Liu and Onaivi2019, Jordan and Xi, Reference Jordan and Xi2019). Interestingly, the roles for CB2R in the effects of psychostimulants seem to be opposite to those ascribed to CB1R.
Systemic administration of the CB2R agonist, JWH133, dose-dependently inhibited cocaine-enhanced locomotion in wild-type mice, but not in CB2R KO animals (Xi et al., Reference Xi, Peng, Li, Song, Zhang, Liu, Yang, Bi, Li and Gardner2011, Gobira et al., Reference Gobira, Oliveira, Gomes, Da Silveira, Asth, Bastos, Batista, Issy, Okine, De Oliveira, Ribeiro, Del Bel, Aguiar, Finn and Moreira2019, Lopes et al., Reference Lopes, Bastos, Costa, Aguiar and Moreira2019). Local administration of CB2R agonist into NAcc also resulted in attenuation of cocaine hyperlocomotion, confirming that this effect is mediated by activation of brain CB2R (Xi et al., Reference Xi, Peng, Li, Song, Zhang, Liu, Yang, Bi, Li and Gardner2011). Consistently with these pharmacological data, findings obtained with genetically modified mice also support the importance of CB2R to regulate psychostimulant responses. Transgenic mice overexpressing CB2R were less responsive to cocaine-induced motor hyperactivity than wild-type mice (Aracil-Fernandez et al., Reference Aracil-Fernandez, Trigo, Garcia-Gutierrez, Ortega-Alvaro, Ternianov, Navarro, Robledo, Berbel, Maldonado and Manzanares2012). Corroborating these findings, specific deletion of CB2R in dopamine neurons increased the responsivity to acute administration of amphetamine, methamphetamine, and cocaine (Canseco-Alba et al., Reference Canseco-Alba, Schanz, Sanabria, Zhao, Lin, Liu and Onaivi2019).
CB2R also is involved in regulation of behavioural sensitisation induced by psychostimulants. A decrease in motor sensitisation to cocaine was observed in mice overexpressing the CB2R and after treatment with an agonist of these receptors during the acquisition phase of sensitisation (Aracil-Fernandez et al., Reference Aracil-Fernandez, Trigo, Garcia-Gutierrez, Ortega-Alvaro, Ternianov, Navarro, Robledo, Berbel, Maldonado and Manzanares2012, Delis et al., Reference Delis, Polissidis, Poulia, Justinova, Nomikos, Goldberg and Antoniou2017, Lopes et al., Reference Lopes, Bastos, Costa, Aguiar and Moreira2019). Similarly, when a CB2R agonist was injected on the test day, the expression of cocaine sensitization in both mice and rats also was inhibited (Delis et al., Reference Delis, Polissidis, Poulia, Justinova, Nomikos, Goldberg and Antoniou2017). Interestingly, compared to the wild type, mice with a selective deletion of CB2R in dopamine neurons did not develop behavioural sensitisation when exposed to repeated treatment with cocaine, amphetamine, and methamphetamine (Canseco-Alba et al., Reference Canseco-Alba, Schanz, Sanabria, Zhao, Lin, Liu and Onaivi2019).
CB2R seems to be also involved in the regulation of psychostimulant-rewarding responses. Transgenic mice overexpressing CB2R show an impairment in the acquisition of cocaine self-administration (Aracil-Fernandez et al., Reference Aracil-Fernandez, Trigo, Garcia-Gutierrez, Ortega-Alvaro, Ternianov, Navarro, Robledo, Berbel, Maldonado and Manzanares2012). Similarly, both acquisition and expression of cocaine-induced CPP were inhibited by previous pharmacological treatment with CB2R agonist (Delis et al., Reference Delis, Polissidis, Poulia, Justinova, Nomikos, Goldberg and Antoniou2017, Lopes et al., Reference Lopes, Bastos, Costa, Aguiar and Moreira2019). These effects, were inhibited by blockade of CB2R, supporting the involvement of these receptors in regulation of cocaine rewarding (Lopes et al., Reference Lopes, Bastos, Costa, Aguiar and Moreira2019).
Intriguingly, some studies have observed opposite results. For example, systemic blockade of CB2R inhibited intravenous cocaine self-administration and shifted cocaine dose-response curves downward in rats and wild type, but not in CB2R KO, mice (Jordan et al., Reference Jordan, Feng, Galaj, Bi, Xue, Liang, Mcguire, Xie and Xi2020). Similarly pharmacological silencing of CB2R reduced the reinstatement of cocaine-seeking behaviour (Adamczyk et al., Reference Adamczyk, Miszkiel, Mccreary, Filip, Papp and Przegalinski2012). Although the reasons for these discrepancies remain unclear, species difference in CB2R expression could play a role. For instance, activation of CB2R inhibited cocaine self-administration under a FR in mice, but not in rats (Zhang et al., Reference Zhang, Bi, Li, Li, Qu, Zhang, Li, Onaivi, Gardner, Xi and Liu2015). However, under a PR schedule of reinforcement, activation of CB2R increased breakpoint for cocaine self-administration in rats (Zhang et al., Reference Zhang, Bi, Li, Li, Qu, Zhang, Li, Onaivi, Gardner, Xi and Liu2015). Beyond differences between species, the multifaceted pattern of CB2R suggested by these studies may be due to the doses and the pattern of psychostimulants administration as well as by the differences in behavioural protocol used in the experiments.
Evidence from molecular assays suggests that brain CB2R modulates the effects of psychostimulants by a dopaminergic mechanism. Indeed, while activation of these receptors reduces the cocaine-induced enhancement of dopamine levels in the NAcc, the blockade of CB2R elevated basal extracellular dopamine levels in this brain region (Xi et al., Reference Xi, Peng, Li, Song, Zhang, Liu, Yang, Bi, Li and Gardner2011, Zhang et al., Reference Zhang, Gao, Liu, Bi, Li, Yang, Gardner, Wu and Xi2014). Moreover, electrophysiological studies showed that treatment with CB2R agonists leads to a decrease in VTA’s dopamine neuronal firing (Zhang et al., Reference Zhang, Gao, Liu, Bi, Li, Yang, Gardner, Wu and Xi2014). Finally, a reduction in dopamine active transporter gene expression and enhanced in tyrosine hydroxylase activity were observed in the midbrain after selective deletion of CB2R in dopamine neurons (Canseco-Alba et al., Reference Canseco-Alba, Schanz, Sanabria, Zhao, Lin, Liu and Onaivi2019).
In summary, the data reviewed here provided evidence that CB2R modulates behavioural and molecular responses to psychostimulants. Considering the important limitation for the therapeutic development of CB1R antagonists, which cause unwanted serious psychiatric adverse events, modulation of CB2R might be an interesting target to treat psychostimulant addiction (Moreira and Crippa, Reference Moreira and Crippa2009).
Integrating CB1R and CB2R functions in the modulation of psychostimulant effects
As discussed throughout this review, either CB1R blockade or CB2R activation inhibits the molecular and behavioural responses to psychostimulant drugs. Their diametrically opposite roles might be explained by differences in the expression patterns in mesolimbic pathways modulating drug reward and reinforcement. CB1Rs are expressed in GABAergic neurons and glutamate presynaptic terminals in the VTA, while CB2Rs are located direct in dopaminergic VTA neurons (Kortleven et al., Reference Kortleven, Fasano, Thibault, Lacaille and Trudeau2011, Zhang et al., Reference Zhang, Gao, Liu, Bi, Li, Yang, Gardner, Wu and Xi2014, Wang et al., Reference Wang, Treadway, Covey, Cheer and Lupica2015). This differential expression leads to a distinct regulation in the function of dopamine neurons. CB1R might fine-tune GABA and glutamate inputs onto mesolimbic dopaminergic neurons, predominantly increasing dopaminergic activity, whereas CB2R might directly inhibit VTA neurons and reduce dopamine release (Zhang et al., Reference Zhang, Gao, Liu, Bi, Li, Yang, Gardner, Wu and Xi2014, Wang et al., Reference Wang, Treadway, Covey, Cheer and Lupica2015).
A major challenge consists in tying together studies focusing on each cannabinoid receptor to postulate an integrative hypothesis on endocannabinoid modulation of psychostimulant effects. Recently, we found that CB1R antagonists and CB2R agonists prevent the hyperlocomotion and the CPP induced by cocaine in mice, as expected. More importantly, the ameliorating effects of CB1R antagonism could be reversed by previous administration of CB2R antagonist. Therefore, CB1R antagonists could inhibit cocaine effects possibly because endocannabinoid actions are diverted predominantly to CB2R. Moreover, although inhibition of the endocannabinoid hydrolysing enzymes FAAH (fatty acid amide hydrolase) and MAGL (monoacylglycerol lipase) failed to interfere with cocaine effects, inhibition of MAGL, which preferentially hydrolysis 2-AG, did prevent cocaine hyperlocomotion when combined with a low, ineffective dose of a CB1R antagonist (Gobira et al., Reference Gobira, Oliveira, Gomes, Da Silveira, Asth, Bastos, Batista, Issy, Okine, De Oliveira, Ribeiro, Del Bel, Aguiar, Finn and Moreira2019). Accordingly, cocaine inhibition of norepinephrine uptake stimulates 2-AG release in the VTA, with subsequent inhibition of GABAergic terminals and facilitation of dopaminergic activity (Wang et al., Reference Wang, Treadway, Covey, Cheer and Lupica2015). Moreover, modulation of 2-AG levels in VTA and in prelimbic cortex also regulate cocaine-related responses (Tung et al., Reference Tung, Lu, Lee, Yu, Lee, Leishman, Bradshaw, Hwang, Hung, Mackie, Zimmer and Chiou2016, McReynolds et al., Reference Mcreynolds, Doncheck, Li, Vranjkovic, Graf, Ogasawara, Cravatt, Baker, Liu, Hillard and Mantsch2018). In summary, a distinct functional localisation of cannabinoid receptors in the mesocorticolimbic system might explain how CB1R blockade and CB2R activation exert opposite effects upon cocaine responses. In addition, the ameliorating effects of CB1R antagonists might occur by redirecting 2-AG effects to predominantly facilitate CB2R signalling (Fig. 1).
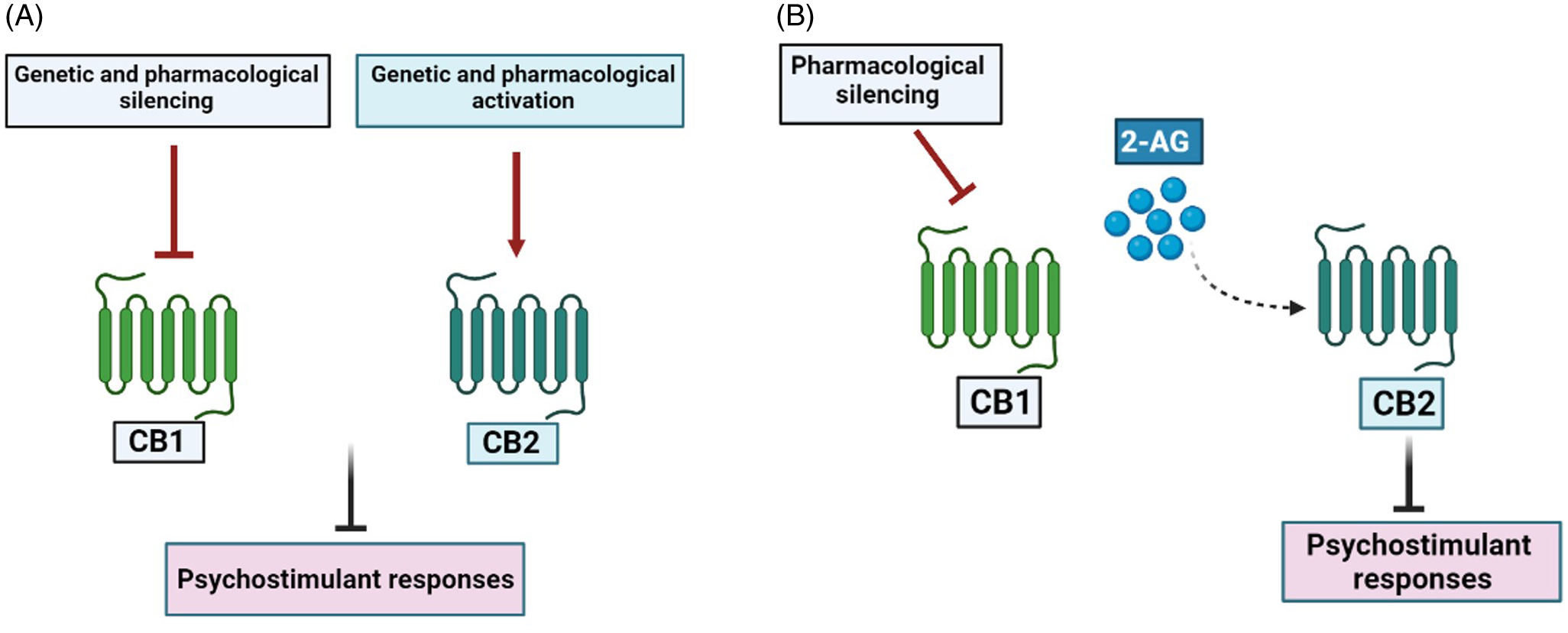
Fig. 1. CB1 and CB2 receptors differentially regulate the effects of psychostimulant drugs. Both CB1R blockade and CB2R activation inhibits the molecular and behavioural responses to psychostimulant drugs (Panel A). Blockade of CB1R redirects 2-AG effects to predominantly facilitate CB2R signalling (Panel B).
The integrative response promoted by CB1R blockade and CB2R activation in modulation of other drugs of abuse have not been performed yet. However, the individual role of cannabinoid receptors in decreasing the effects induced by distinct classes of addictive drugs has already been demonstrated (Manzanares et al., Reference Manzanares, Cabanero, Puente, Garcia-Gutierrez, Grandes and Maldonado2018). For instance, pharmacological and genetic silencing of CB1R reduced the hyperlocomotion and rewarding effects induced by alcohol, opiates, and nicotine (Navarro et al., Reference Navarro, Carrera, Fratta, Valverde, Cossu, Fattore, Chowen, Gomez, Del Arco, Villanua, Maldonado, Koob and Rodriguez De Fonseca2001, Houchi et al., Reference Houchi, Babovic, Pierrefiche, Ledent, Daoust and Naassila2005, Simonnet et al., Reference Simonnet, Cador and Caille2013, Marinho et al., Reference Marinho, Oliveira-Lima, Santos, Hollais, Baldaia, Wuo-Silva, Yokoyama, Takatsu-Coleman, Patti, Longo, Berro and Frussa-Filho2015, Guegan et al., Reference Guegan, Cebria, Maldonado and Martin2016). CB1R also modulates the dopamine release in the NAcc elicited by these prototypical drugs (Cheer et al., Reference Cheer, Wassum, Sombers, Heien, Ariansen, Aragona, Phillips and Wightman2007, Parsons and Hurd, Reference Parsons and Hurd2015). Moreover, similarly to observed with psychostimulants, the activation of CB2R reduced ethanol consumption and the ethanol-induced CPP (Al Mansouri et al., Reference Al Mansouri, Ojha, Al Maamari, Al Ameri, Nurulain and Bahi2014). Treatment with CB2R-agonist also decreases the responses promoted by opiates (Zhang et al., Reference Zhang, Dong, Zou, Li, Li, Wang and Li2018, Iyer et al., Reference Iyer, Slivicki, Thomaz, Crystal, Mackie and Hohmann2020). Together these studies suggested that modulation of both cannabinoid receptors seems to be a common mechanism underlying the molecular and behavioural properties of different classes of drugs.
Concluding remarks and future perspectives
Preclinical studies implicate cannabinoid receptors in the modulation of behavioural and molecular responses induced by psychostimulant drugs. The consistent results showing that genetic and pharmacological blockade of CB1R inhibits cocaine effects and could encourage the use of selective antagonists for treating psychostimulant addiction disorders. However, the incidence of serious psychiatric adverse events, such as anxiety and depression, limits the use of these compounds. In this context, drugs targeting CB2R might represent a more promising approach. Thus, an increasing number of studies has focused on the effects of CB2R agonists in modulation of psychostimulants responses. Combining inhibition of 2-AG hydrolysis with low doses of CB1R antagonists and therefore favouring endocannabinoid facilitation of CB2R signalling could also represent a new approach.
Despite this substantial evidence demonstrating the important roles of CB1R and CB2R in regulation of psychostimulant responses, so far the studies have focused on male mice and rats, excluding in female animals. Sexual dimorphism has been demonstrated in behavioural and molecular responses correlated to CB1R and CB2R. For example, expression and functionality of CB1R were observed in the VTA and PFC of females compared to male animals, possibly providing a neural substrate for the existing sex differences to the rewarding effects of cannabinoids (Llorente-Berzal et al., Reference Llorente-Berzal, Assis, Rubino, Zamberletti, Marco, Parolaro, Ambrosio and Viveros2013, Castelli et al., Reference Castelli, Fadda, Casu, Spano, Casti, Fratta and Fattore2014). In fact, females self-administered more WIN55,212-2, a non-selective cannabinoid agonist, than male rats(Fattore et al., Reference Fattore, Spano, Altea, Fadda and Fratta2010). Regarding responses correlated to CB2R, Onaivi and co-workers demonstrated that treatment with CB2R agonist alters mouse spontaneous locomotor activities in a sex-dependent fashion (Onaivi et al., Reference Onaivi, Ishiguro, Gong, Patel, Perchuk, Meozzi, Myers, Mora, Tagliaferro, Gardner, Brusco, Akinshola, Liu, Hope, Iwasaki, Arinami, Teasenfitz and Uhl2006). In the face with this evidence, the role of CB1R and CB2R in regulation of psychostimulants in female animals should be explored in future studies.
Table 1. Cannabinoid receptors influence on psychostimulant-related behaviours
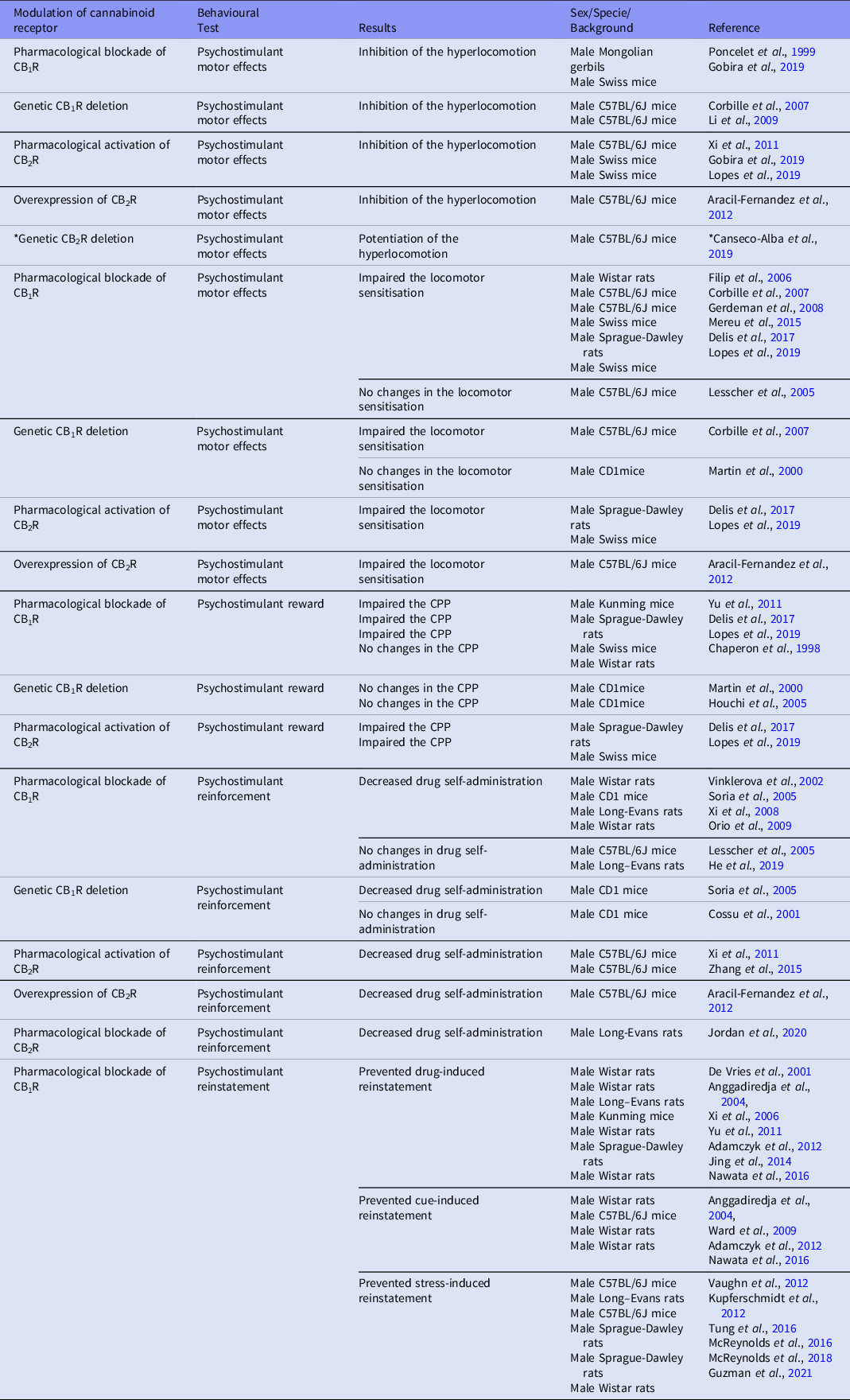
*DAT-Cnr2 conditional knockout, ** Deleted CB1R in cortical glutamatergic neurons.
Acknowledgments
Pedro Henrique Gobira received a postdoctoral fellowship from Fundação de Amparo à Pesquisa do Estado de São Paulo (FAPESP; no. 2017/19284-0). SJ received a productivity fellowship from Conselho Nacional de Desenvolvimento Científico e Tecnológico (CNPq; no. 304780/2018-9 and 406122/2016-4). FM received a productivity fellowship from Conselho Nacional de Desenvolvimento Científico e Tecnológico (CNPq; no. 303123/2020-6). This work was funded by Coordenação de Aperfeiçoamento de Pessoal de Nível Superior (CAPES; Finance Code 001), FAPESP (no. 2017/24304-0), Aarhus University Research Foundation (AUFF-E-2020-7-1 9) and Fundação de Amparo à Pesquisa do Estado de Minas Gerais (no. APQ-02064-15)
Conflicts of interest
The authors declare no conflict of interest.