The proportion of older people in the seventh census is higher than that in the sixth national census(1), indicating that China is becoming an ageing society. This is a huge challenge for China today, especially in terms of how to meet the health needs of the Chinese elderly. Dietary protein is a key nutrient for older adults, and adequate protein intake can improve function and quality of life in healthy older adults(Reference Hengeveld, de Goede and Afman2,Reference Wolfe, Miller and Miller3) .
Whether the protein requirements of elderly people are the same as that of young-middle-aged people has been controversial. In the 2013 edition of Dietary Reference Intakes for Chinese residents, the protein requirements of elderly people are consistent with those of young-middle-aged people due to a lack of local research data on the protein requirements of the elderly. The indicator amino acid oxidation (IAAO) technique is minimally invasive, relatively less demanding and more rapid than 24 h-indicator amino acid oxidation and balance (24 h-IAAO/IAAB) model, thus allowing for multiple test intakes over a wide range from deficient to excess(Reference Elango, Ball and Pencharz4). It is usually utilised to measure the response to differing levels of intake of dietary protein as well as individual essential amino acids(Reference Elango, Ball and Pencharz4). Recently, the IAAO technique has been used to determine the protein requirements in children(Reference Elango, Humayun and Ball5), young males(Reference Humayun, Elango and Ball6), pregnant females(Reference Stephens, Payne and Ball7) and the elderly(Reference Tang, McCabe and Elango8,Reference Rafii, Chapman and Elango9,Reference Rafii, Chapman and Owens10) . Therefore, in our previous study, we conducted protein requirements for fourteen elderly people using IAAO technology in 2020, and the results showed that protein estimated average requirement (EAR) and recommended nutrient intake (RNI) were determined to be 0·91 and 1·17 g/(kg·d), respectively(Reference Mao, Chen and Wang11). In order to revise protein requirements of the elderly, more research data are needed to revise the protein requirements of the elderly in China. In addition, elderly people with sarcopenia may require more protein(Reference Putra, Konow and Gage12,Reference Phillips, Chevalier and Leidy13) , which have not been excluded in previous studies. In this study, the protein requirements of elderly people were reevaluated by increasing the sample size without including patients with sarcopenia.
Materials and methods
Subjects
Eighteen healthy elderly adult volunteers (9 males and 9 females) were re-recruited in the study after undergoing a screening for sarcopenia and a routine medical examination, including complete blood count, blood chemistry and hepatic and renal functions, and two of the volunteers had participated in our previous study. The inclusion criteria of subjects included were age 65–80 years; BMI (kg/m2) 20–30; no weight loss in the previous 6 months; no acute or chronic illnesses known to affect the metabolism of proteins and amino acids, such as cancer, diabetes, renal disease or liver disease; no gastrointestinal diseases and no recent infectious diseases, surgery or antibiotic therapy within 8 weeks of the commencement of this study. Participants with hypertension were not excluded if their blood pressure was well controlled, and their antihypertensive medications were taken as prescribed by their physician. Due to the need to collect exhalation, the elderly with respiratory diseases were also excluded. Participants with sarcopenia were excluded according to the 2019 Asian Consensus on the diagnosis and treatment of sarcopenia(Reference Chen, Woo and Assantachai14). Sarcopenia was diagnosed as the presence of low muscle mass combined with either low muscle strength or poor physical performance. The appendicular skeletal muscle mass index (ASMI, kg/m2) was calculated by dividing the appendicular skeletal muscle mass (ASM) by the square of height. Upper and lower limb skeletal muscle mass were measured using bioelectrical impedance analysis (Inbody 720). Low muscle mass was defined as ASMI < 7·0 kg/m2 for males and < 5·7 kg/m2 for females. Muscle strength was assessed by measuring the power of handgrip strength using the hand dynamometer. The cut-off points for low handgrip strength were < 18 kg for females and < 28 kg for males. Six-meter gait speed (GS) or five-time chair stand test (CS-5) assessed physical performance. Low physical performance was defined as a GS < 1 m/s or CS-5 ≥ 12 s. The present study was conducted according to the guidelines in the Declaration of Helsinki and was approved by the Ethical Committee of the Institute for Nutrition and Health, Chinese Center for Disease Control and Prevention (No. 2022-016). Each subject provided signed informed consent prior to the initiation of the study. This trial was registered at the Chinese clinical trial registry as ChiCTR2200061382.
Experimental design
This study was based on the minimally invasive IAAO protocol used previously in elderly adults(Reference Tang, McCabe and Elango8,Reference Rafii, Chapman and Elango9,Reference Rafii, Chapman and Owens10,Reference Mao, Chen and Wang11) . Before the studies commenced, subjects were fasted overnight (12 h) and were measured for body composition (fat-free mass and ASM) and resting energy expenditure. Body composition was measured by bioelectrical impedance analysis (Inbody 720). Resting energy expenditure was measured by continuous, open-circuit indirect calorimetry (Cortex metamax3B). Two days before the study day were the adaptation days, during which the subjects received a daily protein intake of 1·0 g/kg body weight and 1·7 × resting energy expenditure. The adaptation diet was weighted in daily portions for each subject. The diet was a standard Chinese diet, mostly consisting of high-protein meals and staple foods. The daily 1·0 g/(kg·d) protein intake was calculated for each participant according to body weight. Each individual food item in the meal was prepared separately. During the adaptation days, all participants were encouraged to consume all the food provided. Prior to and following consumption, weights were taken and recorded for each food item to ascertain actual intake. The data collection encompassed both the type and quantity of food consumed by all subjects, with detection of macronutrient concentrations (including protein, fat and carbohydrate) as well as energy content to ensure that participants’ actual protein intakes closely approximated 1·0 g/(kg·d). Participants were also provided a multivitamin and mineral tablet (Centrum; Wyeth Consumer Health Care) supplement daily for the duration of all studies.
On the study day, after the participants had fasted for 12 h, they randomly consumed 1 of 7 dietary protein intakes (0·1, 0·3, 0·6, 0·9, 1·2, 1·5 and 1·8 g/(kg·d)), with a 1-week period between doses. All participants consumed 8 hourly isoenergetic meals, and the energy was provided at 1·5 × resting energy expenditure. The experimental diet consisted of the following: lactalbumin powder, protein-free biscuits, protein-free and fried starch slices and protein-free lotus root starch. The study diet provided 33 % energy as fat and variable energy from carbohydrate (40·8 %–65·3 %) and protein (1·0 %–25·5 %) according to the test protein intake. Each protein dose provided the same amounts of phenylalanine and tyrosine, which was ensured by determination of the amino acid composition of the same batch of marketable lactalbumin powder according to the Chinese standard GB 5009·124-2016. According to the highest dose of daily protein content (1·8 g/kg), L-phenylalanine (Now Foods) and L-tyrosine (Puritan’s Pride) were added to protein doses of 0·1–1·5 g/kg. Phenylalanine was provided at an amount of 54·4 mg/(kg·d). Tyrosine was provided at 58·0 mg/(kg·d) to ensure an excess of tyrosine. Amino acid compositions of selected test protein intakes are provided in Table 1.
Table 1. Amino acid composition of reference protein and various test protein doses

* Represents lactalbumin composition.
† L-Phenylalanine intake was kept constant at 54.4 mg/(kg·d).
‡ L-Tyrosine intake was kept constant at 58.0 mg/(kg·d).
Tracer protocol
The participants consumed hourly meals for 4 h prior to the start of the oral tracer infusion protocol for each oxidation study day at each protein dose. Oral priming doses of 0·176 mg NaH13CO3/kg (99 atom percentage excess; Cambridge Isotope Laboratories) and 0·66 mg L-(1–13C) phenylalanine/kg (99 atom percentage excess; Cambridge Isotope Laboratories) were given with the fifth hourly meal(Reference Tang, McCabe and Elango8,Reference Mao, Chen and Wang11) . An hourly oral dose of 1·2 mg/(kg·h) of L-(1–13C) phenylalanine was commenced simultaneously with the fifth meal and continued for the remaining 3 h of the study(Reference Tang, McCabe and Elango8,Reference Mao, Chen and Wang11) . A detailed protocol is provided in Fig. 1.
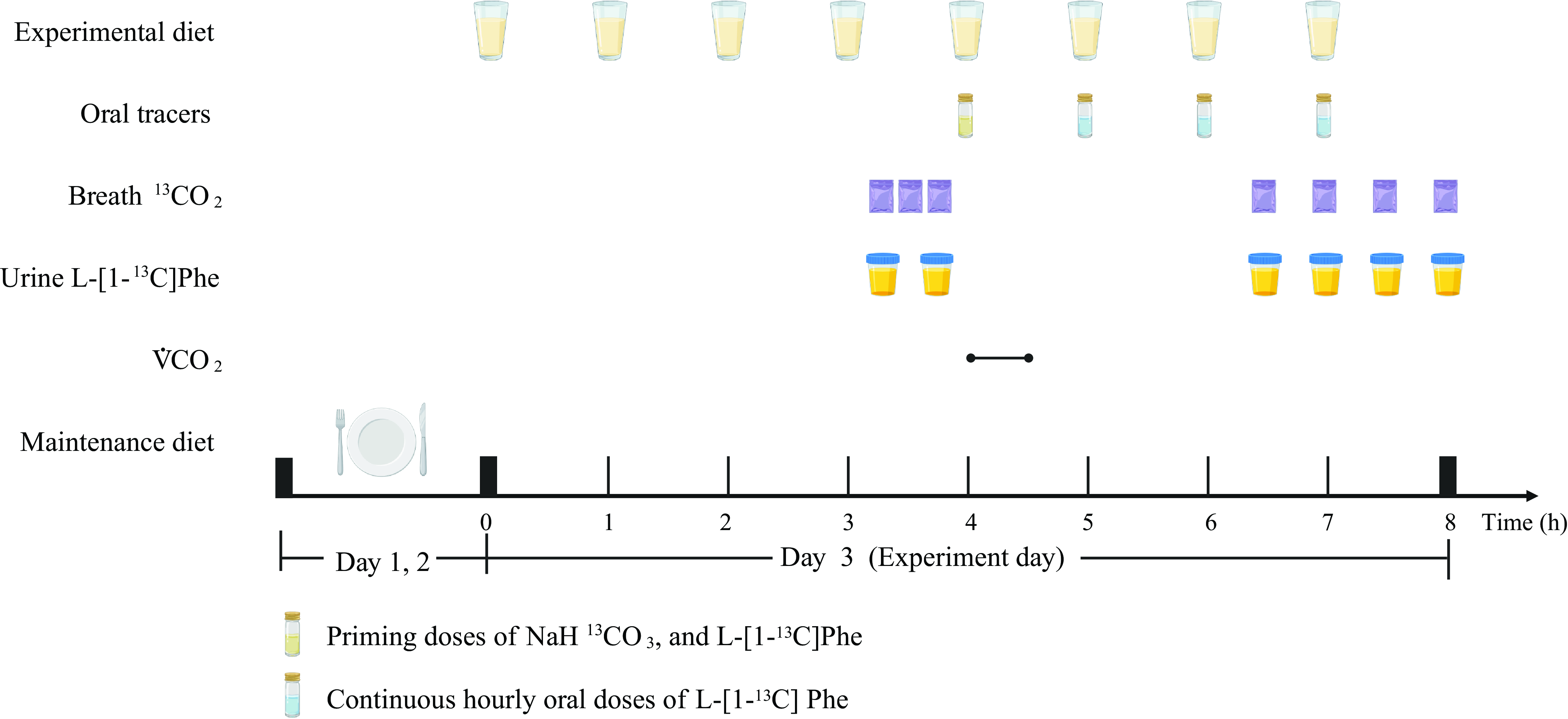
Fig. 1. Example protocol for an indicator amino acid oxidation (IAAO) study. Created with BioRender.com.
Sample collection and analysis
Breath and urine samples were collected on all oxidation study days. Three baseline breath samples (45, 30 and 15 min before), and two baseline urine samples (45 and 15 min before) were obtained before the participants consumed the fifth hourly meal containing the indicator amino acid. Four plateau breath and four plateau urine samples were collected at isotopic steady state every 30 min beginning 2·5 h after the fifth hourly meal.
Breath samples were obtained and maintained at room temperature for further analysis in disposable expiratory bags (Shenzhen Zhonghe Headway Bio-Sci & Tech Co., Ltd). The enrichment of 13CO2 in breath samples was analysed using a 13C-breath test device (HeliFANplus, Fischer ANalysen Instrumente GmbH). Prior to analysis, urine samples were kept at −80°C. Using a TSQ triple quadrupole mass spectrometer (Thermo Fisher Scientific) in positive electrospray ionization mode, as described previously(Reference Tian, Liu and Zhang15), the enrichment of L-(1–13C) phenylalanine in urine samples was measured. Isotopic enrichment was expressed as mole percentage excess and was calculated from peak area ratios at the isotopic steady state at baseline and at plateau.
Tracer kinetics
Isotope kinetics were calculated as previously described(Reference Tang, McCabe and Elango8,Reference Mao, Chen and Wang11,Reference Matthews, Motil and Rohrbaugh16) . The 13CO2 rate in breath (F13CO2, μmol/(kg·h)) after the oxidation of ingested L-(1–13C) phenylalanine was calculated by using a factor of 0·82 to account for carbon dioxide retained in the body’s bicarbonate pool(Reference Hoerr, Yu and Wagner17).
Whole-body phenylalanine flux (μmol/(kg·h)) was calculated from the dilution of orally administered L-(1–13C) phenylalanine into the plasma pool (at isotopic steady state) by using enrichment of L-(1–13C) phenylalanine in urine.
Statistical analysis
Statistical analyses were performed by using the Statistical Analysis Systems 9.4 software. All of the results are expressed as means ± SD. A value of P < 0·05 was considered statistically significant.
ANOVA was used to test for differences in the body weight, fat-free mass, ASMI and phenylalanine flux, and if there was a general difference, a post hoc analysis was performed by using the Student Newman–Keuls test for multiple comparisons. The mean protein requirement was estimated by applying a nonlinear mixed-effects model (PROC NLMIXED, SAS Institute) to the F13CO2 data as previously described(Reference Tang, McCabe and Elango8,Reference Mao, Chen and Wang11) .
The SAS Procedure NLMIXED used in our analysis is able to provide estimates for arbitrary functions of the parameters with CI. Using this approach, we obtained an estimate with 95 % CI for the EAR. CI were obtained by following the standard asymptotic theory of the maximal likelihood estimation, but the method narrowed the 95 % CI(Reference Hayamizu, Kato and Hattori18). Therefore, to determine RNI, we further combined sd (EAR plus 1·96 sd)(Reference Elango, Ball and Pencharz4,19) or CV with protein EAR (1·25 times the EAR)(19,20) .
Results
Subject characteristics
Subject characteristics are presented in Table 2. Each subject was randomly assigned to receive a concentration of 0·1, 0·3, 0·6, 0·9, 1·2, 1·5 and 1·8 g protein/kg body weight, for which two subjects were missing the 0·1 and 1·8 g/kg doses and two subjects were missing the 0·1 g/kg doses. ASM measurements were performed weekly to better monitor the subject’s muscle mass. When ASMI was below normal, patients with sarcopenia were excluded in combination with handgrip strength, gait speed or five chair stand tests. One female participant diagnosed with sarcopenia during the trial period was excluded. Another subject withdrew from the study in the third week due to personal reasons. Therefore, it was ultimately sixteen subjects who completed this study. There was no significant difference in body weight and ASMI of subjects at different doses of dietary protein in Table 3.
Table 2. Characteristics of the elderly Chinese adults administered different protein doses*

ASMI, appendicular skeletal muscle mass index; FFM, fat-free mass; REE, resting energy expenditure.
* Results are expressed as means ± SD.
Table 3. Body weight of elderly Chinese adults administered different protein doses in 7 week*

ASMI, appendicular skeletal muscle mass index; FFM, fat-free mass.
* Results are expressed as means ± SD.
There was no significant difference in subject body weights, ASMI and FFM.
Dietary nutrient intakes
During the 2 d before the tracer protocol, the diets with different protein doses were consistent, and the designed protein doses were maintained at 1·0 g/(kg·d). The actual main macronutrient and energy intakes of the subjects for the 7 dietary protein doses were 1·00 ± 0·05 g/(kg·d) for protein, 0·82 ± 0·06 g/(kg·d) for fat, 5·45 ± 0·39 g/(kg·d) for carbohydrate and 33·5 ± 2·15 kcal/(kg·d) for energy. The dietary macronutrient and energy intakes of older males and older females on the adaptation days are shown in Supplementary Table 1.
Breath 13CO2 excretion
The influence of protein intake on production of 13CO2 from phenylalanine oxidation (F13CO2) in older adults is shown in Fig. 2. F13CO2 decreased as protein intake increased, consistent with an increase of L-(1–13C) phenylalanine incorporation into the protein. This increase in incorporation of labeled phenylalanine continued until the protein intake reached the protein requirement, and there was no further incorporation of labeled phenylalanine into the protein, with the model-derived equation as follows:



Fig. 2. The relation between protein intake and breath F13CO2 production in elderly Chinese adults administered protein at seven different doses. The data points represent the 13CO2 excretion of individual subjects at each protein intake dose. The breakpoint represents the estimated mean protein requirement, which is 0·94 g/(kg·d).
where x ij is the protein intake for subject i at protein dose j; y ij is the F13CO2 for subject i at protein dose j; 1·30 is the intercept; –0·71 the slope of the linear part of the model and 0·94 is the breakpoint of the piecewise linear function (r 2 = 0·52).
Nonlinear mixed-effects model analysis of the F13CO2 data resulted in the identification of a breakpoint for the mean protein requirement of 0·94 g/(kg·d). The protein EAR was 0·93 g/(kg·d) and 0·94 g/(kg·d) for male and female older adults, respectively. Calculated on the basis of fat-free mass, the protein requirement was estimated to be 1·47 g/(kg·d) for older females and 1·31 g/(kg·d) for older males. The RNI by determining the upper 95 % CI of the breakpoint was at 1·13 g/(kg·d) with a lower 95 % CI of 0·75 g/(kg·d). Combining the sd resulted in the RNI of 1·36 g/(kg·d), and extrapolating the protein RNI using the CV of the protein EAR resulted in the RNI of 1·18 g/(kg·d).
Phenylalanine flux
Phenylalanine flux was not affected (P = 0·9451) within each individual by differences in protein intake as required by the IAAO technique. The phenylalanine flux was 25·8 ± 8·1 (males: 26·4 ± 8·5, females: 24·9 ± 7·7) μmol/(kg·h). Thus, the precursor pool of phenylalanine was consistent across trials, which is a requirement for the reliable estimation of the recommended protein intake by F13CO2 analysis.
Discussion
This study assessed the protein needs of Chinese elderly without sarcopenia by using the IAAO technique and the results of this study suggest that the protein EAR of Chinese elderly aged 65–80 years was 0·94 g/(kg·d), and the RNI ranged from 1·13 to 1·36 g/(kg·d). These results are higher than the current recommendations, 0·88 g/(kg·d) for EAR and 0·98 g/(kg·d) for RNI.
In the last decade, the IAAO technique has been the most commonly used to study human protein requirements. The results of studies using the IAAO technique to study protein requirements in the elderly indicated that estimated protein EAR and RNI of 0·94 and 1·24 g/(kg·d) in older males (n = 6)(Reference Rafii, Chapman and Elango9) and 0·96 and 1·29 g/(kg·d) in older females (n = 12)(Reference Rafii, Chapman and Owens10). The protein EAR of the elderly (> 65 years) in the present study, as determined by the IAAO technique, was comparable to that in the above study(Reference Rafii, Chapman and Elango9,Reference Rafii, Chapman and Owens10) , whereas the upper 95 % CI (RNI) of 1·13 g/(kg·d) was lower.
In the current study, the protein RNI of 1·36 g/(kg·d) obtained by combining sd was relatively large. Between- and within-subject variation may be the main reason for the large sd, especially for elderly subjects(Reference Nguyen, Moodie and Forget21,Reference Ferrucci and Kuchel22) . Body composition changes considerably with age(Reference Cruz-Jentoft, Landi and Schneider23), and in the present study, the participants spanned a wide range of ages (range: 66–79 years), which may have made more variation in the results. The approximate of the RNI using 1·25 times the EAR was 1·18 g/(kg·d). It was recently shown that the inter-individual variability using the change-point regression model was estimated to be a CV of about 20 % based on seven IAAO studies(Reference Hayamizu, Aoki and Izumo24). The CV of the protein requirements determined by IAAO technique was wider than the ordinary CV obtained from the nitrogen balance test, which may account for the small number of breakpoints obtained and the different study conditions (especially with athletes). The weighted mean of CV of three studies (conducted at steady state among adults or the elderly without exercise load) was 19·0 %(Reference Hayamizu, Aoki and Izumo24). The use of this CV (19·0 %) to infer the protein RNI resulted in the RNI of 1·30 g/(kg·d). However, these studies are limited and individual differences in elderly may be large, which requires further data to determine whether a CV of 19 % is appropriate.
Due to limited direct researches on the protein requirements of elderly people, most countries have the same EAR and RNI for the elderly as for young and middle-aged people. In the last decade, however, some countries have already set higher RNI for older adults. For example, the Germany, Austria and Switzerland in 2017 recommend 1·0 g/kg of protein for people ≥ 65 years and 0·8 g/(kg·d) for young and middle-aged people(Reference Richter, Baerlocher and Bauer25). In 2012, the recommended amount of protein for people ≥ 65 years in Northern Europe was 1·1–1·3 g/(kg·d)(Reference Becker, Anderssen and Fogelholm26). In 2017, the recommended amount of protein for people > 70 years in Australia was 1·07 g/(kg·d) for men and 0·94 g/(kg·d) for women, both 25 % higher than the recommended amount for young and middle-aged people(27). Furthermore, experts recommend a protein intake of 1·2–2·0 g/(kg·d) or higher for the elderly(Reference Wolfe, Miller and Miller3,Reference Baum, Kim and Wolfe28) .
The potential need for more dietary protein in older adults is due in part to the declining anabolic response to protein intake, as well as the need for more protein to counteract the inflammatory and catabolic conditions associated with chronic and acute diseases that typically occur with aging(Reference Baum, Kim and Wolfe28). The protein EAR obtained in this study for older males does not differ from the estimates in young males(Reference Humayun, Elango and Ball6). But on the basis of fat-free mass, the current estimated protein requirement for older males was 1·31 g/(kg·d), which was significantly higher compared with the estimated protein requirement for young males of 1·14 g/(kg·d)(Reference Humayun, Elango and Ball6). One study found that older males required more protein than younger males to maximally stimulate myofibrillar protein synthesis after adjusting for body weight or lean mass(Reference Moore, Churchward-Venne and Witard29). In addition, many older adults have a reduced appetite and do not consume enough protein(Reference Volpi, Campbell and Dwyer30). However, a study based on nitrogen balance data indicated that the protein requirements of older adults are not different from those of younger adults and that the recommended of 0·8 g/(kg·d) protein is adequate to meet the minimum dietary requirements of virtually all older adults(Reference Campbell, Johnson and McCabe31). Nonetheless, previous metabolic and epidemiologic studies suggested that protein requirements may increase with aging(Reference Moore, Churchward-Venne and Witard29,Reference Franzke, Neubauer and Cameron-Smith32,Reference Deutz, Bauer and Barazzoni33) . There is not sufficient evidence from the IAAO technique to indicate whether the protein requirement of the elderly is higher than that of young people. We think that using the IAAO technique to study the protein requirements of middle-aged and elderly people at the same time would provide stronger evidence.
A current and frequently discussed area of protein nutrition in elderly people is ‘sarcopenia’, the age-related loss of skeletal muscle mass and consequent decline in muscle strength(Reference Ren, Zhang and He34). Accumulating evidence suggests that dietary protein intakes greater than the current RNI may be beneficial in reducing age-related muscle loss(Reference Putra, Konow and Gage12,Reference Phillips, Chevalier and Leidy13,Reference Paddon-Jones, Short and Campbell35,Reference Wolfe36) . In recent years, it has been widely accepted that older adults with sarcopenia may need more protein, with multiple consensus statements and opinion articles recommending a protein intake of at least 1·0–1·5 g/(kg·d)(Reference Volpi, Campbell and Dwyer30,Reference Morley, Argiles and Evans37,Reference Fielding, Vellas and Evans38) . Therefore, the current study is distinct from previous studies in that patients with sarcopenia were excluded and if there were newly diagnosed sarcopenia patients during the study, they were also excluded.
The participants in this study were not above the age of 80, and the protein EAR of 0·94 g/(kg·d) for older females was greater than the protein EAR of 0·88 g/(kg·d) in a study of females over the age of 80(Reference Tang, McCabe and Elango8). However, with a decline in social or economic status and physiological function results from aging, the oldest old (≥ 80 years) are at a significant risk of nutritional deficiencies in particular(Reference Rivlin39,Reference Lv, Yuan and Mao40) . More studies that include research the oldest old are warranted.
In summary, we reevaluated the dietary protein requirements of healthy Chinese elderly adults without sarcopenia by using the IAAO technique. Our results suggest the EAR in this study is 6·8 % higher than the currently recommended EAR. The protein RNI determined using various derivation approaches ranged from 1·13 to 1·36 g/(kg d), which is higher than the current RNI.
Acknowledgements
The authors specifically thank Glenda Courtney-Martin Ph.D., Assistant Professor, University of Toronto, The Hospital for Sick Children, Toronto, ON, Canada, for assistance with the guidance about experimental design.
This research was funded by Active Health and Aging Technologic Solutions Major Project of National Key R&D Program – Study of Diet and Nutrition Assessment and Intervention Technology (No. 2020YFC2006300) – Study on the Key Nutrients Requirement of Chinese Population (No. 2020YFC2006302).
The authors’ responsibilities were as follows—W. W. and M. L.: designed the research; Y. Z., W. W., H. M., J. L., F. C., H. Z., S. N., Y. Y., R. W., W. Y., L. Y. and M. L.: conducted the research; W. W. and M. L.: performed the statistical analysis; W. W. and M. L.: wrote the paper; W. W., Y. Z., L. Y. and M. L.: had primary responsibility for the final content and all authors: read and approved the final manuscript.
There are no conflicts of interest.
Supplementary material
For supplementary material/s referred to in this article, please visit https://doi.org/10.1017/S0007114523002611