It has been established that intensified feeding strategies can improve future performance in dairy replacement heifers(Reference Moallem, Werner and Lehrer1–Reference Gelsinger, Heinrichs and Jones3). Overall, a high volume of liquid feed (milk or milk replacer) nutrition has been introduced to achieve this potential increase in milk yield later in life(Reference Soberon and Van Amburgh4). However, some reports confirmed that early growth rate resulting from solid feed intake is positively correlated with future milk performance(Reference Bach and Ahedo5,Reference Heinrichs and Heinrichs6) . Fat supplementation could be a solid feed intensification strategy, although the question still remains about whether starter nutrient composition during the early weeks of life could affect future milk performance.
Several studies described negative effects of fat supplementation on performance of dairy calves(Reference Fallon, Williams and Innes7,Reference Hill, Bateman and Aldrich8) ; nevertheless, other studies have reported growth rate improvements(Reference Ghasemi, Azad-Shahraki and Khorvash9). There are numerous factors that may explain the different responses to fat supplementation between studies, and these may relate to the fat supplementation level, or fatty acids (FA) type, and may also include different strategies of milk feeding as well as environmental-related factors. For example, Ghasemi et al. (Reference Ghasemi, Azad-Shahraki and Khorvash9) have shown some favourable effects regarding growth performance with soya oil supplementation in dairy calves during the cold climate. However, soya oil supplementation in another study reduced the growth rate in dairy calves, which is probably due to the inflammatory effect of a high content of linoleic acid(Reference Hill, Bateman and Aldrich8,Reference Hill, Bateman and Aldrich10) and negative effects on nutrients digestibility(Reference Hill, Bateman and Aldrich10). In contrast, anti-inflammatory effects were found with α-linolenic acid (C18 : 3n-3) in biology(Reference Patterson, Wall and Fitzgerald11). Dietary changes over the past few decades in Western pattern diets in the intake of n-6 and n-3 PUFA show increases in the n-6:n-3 ratio (about 15:1), which are associated with greater metabolism of the n-6 PUFA compared with n-3 PUFA. This increase in the ratio of n-6:n-3 PUFA increases chronic inflammatory diseases such as non-alcoholic fatty liver disease, CVD, obesity, inflammatory bowel disease, rheumatoid arthritis and Alzheimer’s disease(Reference Patterson, Wall and Fitzgerald11). n-3 FA supplementation could improve growth performance and immunity in ruminants(Reference Hill, Bateman and Aldrich12–Reference Ballou, Cruz and Pittroff14) which is similar to reports in human nutrition(Reference Patterson, Wall and Fitzgerald11). However, most starter diets of dairy calves were formulated based on maize and soyabean meal; hence, the deficiency of n-3 and high ratio (HR) of n-6:n-3, 15:1 as reported in Hill et al.(Reference Hill, Bateman and Aldrich12), are more obvious in their diets. Dairy calves are supposed to have high diarrhoea occurrence rate and immunity system concerns in early weeks of life(Reference Soberon, Raffrenato and Everett2,Reference Heinrichs and Heinrichs6) ; therefore, improving immune function could enhance rearing efficiency. Based on the discussed importance of n-3 FA in immune system improvement and the limited information available regarding the n-3 FA in suckling dairy calves due to its low concentration in conventional maize grain–soyabean meal-based diets, more research is needed to evaluate n-3 FA supplementation effects on dairy calves.
Regardless of the source and level of supplemented FA, its interaction with protein has been a neglected aspect in dairy calf nutrition. Providing more protein to dairy calves (200 v. 180 g/kg, DM basis) caused improvement in growth rate(Reference Kazemi-Bonchenari, Falahati and Poorhamdollah15); however, ruminal protein degradability effect and its interaction with fat still need more investigation in dairy calves. Schroeder et al.(Reference Schroeder, Titgemeyer and Awawdeh16) stated that more energy supplied by greater dietary FA level resulted in higher N retention and suggested that energy supplementation affects the efficiency of amino acid benefits. Feeding conjugated linoleic acid also increased N accretion in growing bulls(Reference Schiavon, Tagliapietra and Dalla Monta’17) and early lactating dairy cows(Reference Van Sossten, Meyer and Piechotta18). This may be due to some protein sparing effects found in specific FA(Reference Schiavon, Tagliapietra and Dalla Monta’17,Reference Van Sossten, Meyer and Piechotta18) . Bunting et al.(Reference Bunting, Fernandez and Fornea19) stated that supplemented fat with greater rumen undegradable protein (RUP):rumen degradable protein (RDP) ratio improved performance of growing calves averaging body weight (BW) of 195 kg during the summer. In contrast to the aforementioned positive effects of FA on protein metabolism, few studies stated that supplemented fat reduced protein digestibility and feed intake in dairy calves(Reference Fallon, Williams and Innes7). These negative effects could reduce the available amino acid in the small intestine that subsequently is detrimental for growth in dairy calves(Reference Kazemi-Bonchenari, Mirzaei and Jahani-Moghadam20) and consequently may increase undegradable protein requirements in dairy calves.
We hypothesise that linseed oil (LSO) supplementation rich in n-3 FA could improve dairy calves’ performance. Furthermore, limited information is available on how n-3 FA source might influence dairy calves’ responses to RUP:RDP ratio. Hence, the objective of the present study was to determine the effects of, and interactions between, LSO supplementation and RUP:RDP ratio on performance, blood metabolites, growth characteristics and microbial protein synthesis in Holstein dairy calves.
Experimental methods
Animal welfare
All procedures including animal welfare, management and experimental procedures and samplings were approved by the International Animal Care and Ethics Committee of Iranian Council of Animal Care(21).
Experimental design, treatments and animal management
The present study was conducted between 10 April and 25 June 2018 on a commercial dairy farm (Zarrin-Khooshe Agriculture and Animal Husbandry, Arak, Iran). A total of forty-eight 3-d-old female Holstein dairy calves with 41·5 (sd 2·8) kg of initial BW were randomly assigned to experimental starter diets (twelve calves per treatment) in a 2 × 2 factorial arrangement with the factors of supplemented LSO (0 or 2·5 % on DM basis) and RUP:RDP ratio (low ratio (LR) 27:73; HR 38:62). The ruminal degradability rate of protein sources had been considered based on data reported previously in dairy cows(21). The crude protein (CP) content was constant across the treatments (20 %, DM basis), and the values for the RUP:RDP ratios were based on the CP content (Table 1). Immediately after birth, calves were separated from their dams, weighed and moved to individual pens (1·2 × 2·5 m) bedded with sand, which was renewed every 24 h. The animals were fed 2·5 litres of colostrum at each of the first two feedings (i.e. within 1·5 h of life and at 12 h after the first feeding). Colostrum feeding was carried out for the first 2 d of life. Calves received 4 litres/d whole milk in galvanised tin buckets twice per d at 07.30 and 16.30 hours from day 3 to 10 and 7 litres/d from day 11 to 48 of the study followed by 3 litres/d from day 49 to 53 of the study. Whole milk samples were taken weekly and analysed for fat, CP, lactose and total solids using an infrared spectrophotometer (FOSS milk-o-scan; FOSS Electric). The average composition of offered milk was 3·17 (sd 0·09) % fat, 3·04 (sd 0·07) % CP, 4·81 (sd 0·05) % lactose and 11·8 % total solids. All the calves were weaned on day 53 of the study, but the experimental starter diets were continued 20 d thereafter (day 73 of study). Therefore, the whole study lasted 70 d (day 3–73 of age), in which the first 50 d was as pre-weaning and the last 20 d was as post-weaning periods. Experimental treatments were: (1) unsupplemented starter diet with LSO along with low RUP:RDP ratio (NLSO-LR), (2) starter diet supplemented with LSO along with low RUP:RDP ratio (LSO-LR), (3) unsupplemented starter diet LSO along with high RUP:RDP ratio (NLSO-HR) and (4) starter diet supplemented with LSO along with high RUP:RDP ratio (LSO-HR). Experimental starter concentrates were formulated to meet the current National Research Council(22) nutrients requirements. Ingredients and chemical composition of experimental diets are presented in Table 1. Starter concentrates were formulated to be iso-nitrogenous but different in RUP:RDP ratio. The supplemented n-3 FA was supplied through Ca salt of LSO (Persia Lin, Kimia Danesh Alvand Co.). It contained 9 % Ca and 85 % pure FA with following FA composition (100 % DM basis): oleic acid 21 %, stearic acid 7 %, palmitic acid 10 %, linoleic acid 18 %, α-linolenic acid 42 % and other FA 2 %. The n-6:n-3 ratio was 0·42 in this product. Substitution of fat supplement was done with maize grain, and starter concentrates had 3 % difference in energy level. Changes in RUP:RDP ratios of dietary treatments were accomplished by replacing maize gluten meal with soyabean meal. Maize gluten meal was increased in the HR diet to have similar protein level with the LR group. In addition, calcium carbonate was reduced in HR in order to have the same Ca level in the experimental diets. The formulae of the starter concentrates were kept constant within treatment, before and after weaning. Starter feed was fed ad libitum to permit at least 10 % orts (i.e. the portion of the starter not consumed over a 24-h period). Starter feed refusals were collected and recorded at 08.00 hours, and fresh starter feed was fed at 08.30 hours. The next 24-h weight of starter diet offered into calves was regulated based on the last 24-h fed starter content. The calves had free access to water throughout the experimental period.
Table 1. Experimental starter diet ingredients and chemical composition (g/kg of DM, unless otherwise stated)

CP, crude protein; RDP, rumen degradable protein; RUP, rumen undegradable protein; NDF, neutral-detergent fibre.
* Treatments were: (1) no supplementation of linseed oil with low RUP:RDP ratio (NLSO-LR); (2) no supplementation of linseed oil with high RUP:RDP ratio (NLSO-HR); (3) supplementation of linseed oil with low RUP:RDP ratio (LSO-LR) and (4) supplementation of linseed oil with high RUP:RDP ratio (LSO-HR).
† Contained 9 % Ca and 85 % pure fatty acids with the following composition (100 % basis): oleic acid 21 %, stearic acid 7 %, palmitic acid 10 %, linoleic acid 18 %, α-linolenic acid 42 % and other fatty acids 2 %.
‡ Contained per kg of supplement: 150 mg vitamin A, 150 mg vitamin D, 335 mg vitamin E, 1 g Mn, 100 g Ca, 2 g Zn, 60 g P, 12 g Mg, 10 g Na, 2 g Fe, 50 mg Co, 200 mg Cu, 40 mg I and 2 mg Se.
§ Calculated from the National Research Council(22).
|| Values were chemically analysed in laboratory.
¶ Non-fibre carbohydrate was calculated as (DM – (NDF + CP + diethyl ether extract + ash))(22).
Sample collection, chemical and biochemical analysis
BW measurements were taken at 10-d intervals starting from day 3 to day 73 of the experiment (five and two recorded weight data for the pre- and post-weaning periods, respectively). Calves were weighed before the morning meal to eliminate the effects of empty/full status of the gastrointestinal tract on BW. Feeds offered and refused were weighed daily to determine the total starter DM intake for each calf throughout the study. Pre- and post-weaning, and overall means of average daily gain (ADG) and feed efficiency (FE) (kg of BW gain/kg of total DM intake (starter DM intake + milk DM intake)) were also calculated. At 10-d intervals, samples from feeds and orts were dried in a convection oven (60°C for 48 h). Subsamples of dried feeds and orts were mixed thoroughly and ground in a mill (Ogaw Seiki Co. Ltd) to pass a 1-mm screen before chemical analysis. Diet samples were analysed for CP (method 988.05; AOAC)(23), diethyl ether extract (method 920.39; AOAC)(23) and neutral-detergent fibre without sodium sulphite, but with inclusion of α-amylase adopted by Van Soest et al.(Reference Van Soest, Robertson and Lewis24).
Faeces were scored daily before the morning milk feeding as follows: 1 = normal, 2 = soft to loose, 3 = loose to watery, 4 = watery, mucous, slightly bloody and 5 = watery, mucous and bloody(Reference Larson, Owen and Albright25,Reference Heinrichs, Erb and Rogers26) . Rectal temperature was recorded twice per week.
Body measurements including wither height (distance from the base of the front feet to wither), hip height (distance from the base of the rear feet to the hook bones), heart girth (circumference of the chest), body length (distance between the points of shoulder and rump) and body barrel (circumference of the belly before feeding) were taken at the start of the experiment (day 3), at weaning (day 53) and at the end of the study (day 73) according to the method described by Heinrichs et al.(Reference Heinrichs, Erb and Rogers26) for dairy calves.
The microbial protein synthesis was estimated through purine derivatives obtained via spot sampling technique in post-weaning calves. The spot urine sampling technique was used for the estimation of daily urine output from creatinine concentration as explained in detail by Valadares et al. (Reference Valadares, Broderick and Valadaresfilho27). Urine volumes were estimated as BW × 26·8/creatinine concentration (mg/l) in post-weaned dairy calves as reported by Dennis et al.(Reference Dennis, Suarez-Mena and Hill28). Spot urine samples were collected from each animal during the morning (between 09.00 and 11.00 hours) and during the afternoon (between 15.00 and 17.00 hours). Samples were collected when calves urinated spontaneously (approximately 10 ml). An aliquot of 5 ml of each sample was diluted immediately with 45 ml of 0·036 N sulphuric acid and then stored at –20°C for analysis. Later, urine samples were thawed at room temperature and analysed to determine the concentrations of allantoin using the HPLC method described by Chen & Gomes(Reference Chen and Gomes29). Urine samples were also analysed for creatinine and uric acid using commercial kits (555-A; Sigma Chemical Co.; 685-50 Sigma Chemical Co., respectively) according to the manufacturer’s instructions. Total excretion of allantoin and uric acid was calculated from estimated daily urine output and determined metabolite concentrations. The microbial N synthesis in the rumen was calculated from daily urinary purine derivatives output using the following equation described by Chen & Gomes(Reference Chen and Gomes29):

where X is the microbial purine absorbed (mmol/d), 70 is the N content of purines coefficient (mg N/mmol), 0·116 is the ratio of purine N:total N in mixed rumen microbes which is 11·6:100 and 0·83 is the average digestibility of microbial purines(Reference Zhao, Etherton and Martin30).
Blood samples were collected from the jugular vein into 10 ml tubes on 4 h after morning feeding on day 3 at the beginning of the experiment, day 35 and day 70. Blood samples were placed on ice immediately after collection, and within 20 min of drawing, samples were centrifuged at 3000 g for 15 min at 4°C to obtain serum. The serum was preserved at −20°C until biochemical analysis. Serum samples were analysed to determine concentrations of glucose (kit no. 93008), albumin (kit no. 9307), total protein (TP) (kit no. 9304) and blood urea N (kit no. 93013) using commercial kits in accordance with the manufacturer’s instructions (Pars Azmoon Co.). β-Hydroxybutyrate was measured with a commercial kit (Abbott Diabetes Care Ltd); the inter- and intra-assay CV % were 11·0 and 8·5, respectively. Liver function indicator enzymes, aspartate aminotransferase and alanine aminotransferase were measured using ELISA (Auto Analyzer Hitachi 717) and commercial laboratory kits (kits no. 92005 and 92004, for aspartate aminotransferase and alanine aminotransferase, respectively). Insulin concentration in serum was determined using ELISA (Auto Analyzer Hitachi 717).
Statistical analysis
Statistical analyses were performed using PROC MIXED of SAS (version 9.1; SAS Institute) with the individual calf as an experimental unit. Starter intake, ADG and FE were statistically analysed as repeated measures with period (each period was 10 d) as repeated variable using the following model:

where Y ijk is the dependent variable, µ is the overall mean, F i is the effect of LSO supplementation, RUPj is the effect of RUP:RDP ratio, T k is the effect of period, (LSO × T)ij is the interaction between LSO supplementation and period, (RUP × T)ik is the interaction between RUP:RDP ratio and period, (LSO × RUP)jk is the interaction between LSO supplementation and RUP:RDP ratio, (LSO × RUP × T)ijk is the tripartite effect of LSO supplementation, RUP:RDP ratio and period; $\beta(X_i-\bar X)$ is the covariate variable and ϵ ijk is the overall error term. According to the Akaike and Bayesian information criteria, an autoregressive (order 1) covariance structure was chosen. The data were analysed in three separate intervals (3, 53; 54, 73 and 3, 73) and divided into three periods in Results section as pre-weaning (day 3–53), post-weaning (day 54–73) and the entire period (day 3–73). The variables for structural growth, blood parameters and microbial protein synthesis were analysed using a similar model but without the effect of time. For BW and structural growth variables, the initial measurements were included as a covariate for the statistical analysis of future data. Faecal score and rectal temperature data were analysed using a multivariable logistic mixed model (GLIMMIX procedure of SAS, version 9.3) including ‘time’ effect in its statistical model. The differences among treatment means were determined using Tukey’s multiple range tests. Effects were considered to be significant when P < 0·05, and a tendency was considered when 0·05 ≤ P < 0·10. All reported values are least square means.
Results
Calf health, intake and growth performance
All the experimental calves were healthy with no observations of clinical symptoms of systemic diseases. The lowest rectal temperature in the present study was observed for LSO-HR during the pre-weaning and entire periods (P = 0·04 and P = 0·01, respectively; Table 2). A two-way interaction between time and LSO supplementation for body temperature (P = 0·03) was observed. The RUP:RDP ratio did not affect the faecal score of calves during experiment; However, LSO supplementation decreased faecal score during the first 3 weeks of life (P < 0·05; Fig. 1).
Table 2. Starter intake, average daily gain and feed efficiency in dairy calves fed supplemented linseed oil with low or high rumen undegradable protein (RUP):rumen degradable protein (RDP) ratios (n 12 calves per treatment) (Least square means with their standard errors)

DMI, DM intake.
a,b Mean values within a row with unlike superscript letters were significantly different (P < 0·05).
* Treatments were: (1) no supplementation of linseed oil with low RUP:RDP ratio (NLSO-LR); (2) no supplementation of linseed oil with high RUP:RDP ratio (NLSO-HR); (3) supplementation of linseed oil with low RUP:RDP ratio (LSO-LR) and (4) supplementation of linseed oil with high RUP:RDP ratio (LSO-HR).
† Statistical comparisons: LSO = linseed oil supplement or no supplementation; RUP, different ratios of RUP:RDP (low ratio (LR) 27:73; high ratio (HR) 38:62); LSO × RUP = interaction between linseed oil and dietary RUP:RDP ratios.
‡ Sampling time effect was: T = time effect; T × LSO = interaction of time and linseed oil supplement; T × RUP = interaction of time and different ratios of RUP:RDP.
§ kg of body weight gain/kg of total DM intake.

Fig. 1. Faecal score in calves receiving different starter diets. () No supplementation of linseed oil with low RUP:RDP ratio (NLSO-LR); (
) no supplementation of linseed oil with high RUP:RDP ratio (NLSO-HR); (
) supplementation of linseed oil with low RUP:RDP ratio (LSO-LR) and (×) supplementation of linseed oil with high RUP:RDP ratio (LSO-HR). Values are means, with standard errors represented by vertical bars. * Significant difference (P < 0·05) between groups at a given time point. RUP, rumen undegradable protein; RDP, rumen degradable protein.
Starter intake, total DM intake, ADG and FE data are presented in Table 2. In the present study, no interaction was detected between LSO supplementation and RUP:RDP ratio with respect to the starter intake, total DM intake, ADG and FE. However, calves supplemented with LSO tended to have higher ADG (724·5 v. 620·5 g/d; P = 0·05) than non-supplemented groups during the entire period. This resulted in higher weaning (72·4 v. 67·4 kg; P = 0·05) and final BW (90·1 v. 82·8 kg; P = 0·01) when LSO supplemented. The greater RUP:RDP ratio tended to increase ADG in post-weaning (P = 0·07) and increased (P = 0·03) this parameter in entire period in comparison with calves fed the LR diet. Final BW was greater in calves fed the LSO-HR diet than those fed the NLSO-LR diet. Calves supplemented with LSO had greater FE than those received no LSO diets during the pre-weaning and entire periods (P = 0·02 and P = 0·01, respectively). Further, FE tended (P = 0·08) to improve in calves fed the HR diet than those received the LR diet. All above-mentioned performance data (starter, TDM and milk intakes, ADG and FE) had been affected by time (P < 0·05), and the interaction of time and RUP:RDP ratio was significant for starter intake. There was an interaction effect between LSO supplementation and time for FE (P = 0·03); moreover, the interaction between time and RUP:RDP ratio was significant for starter intake (P = 0·04; Table 2).
Skeletal growth
The data of structural growth indices are presented in Table 3. There was no interaction for LSO supplementation and RUP:RDP ratio with respect to the skeletal growth indices in the present study. Results show that body length, body barrel and hip width were similar among treatments. LSO supplementation increased (P = 0·04) wither height and hip height and tended (P = 0·07) to enhance heart girth compared with non-supplemented calves on weaning time. Calves fed higher RUP:RDP ratio tended to have greater heart girth (P = 0·07), wither height (P = 0·06) and hip height (P = 0·05) than those fed with the LR diet at the end of the experiment. The average values for all skeletal growth indices had been influenced over time in the present study (P < 0·05).
Table 3. Growth parameters in dairy calves fed supplemented linseed oil with low or high rumen undegradable protein (RUP):rumen degradable protein (RDP) ratios (n 12 calves per treatment) (Least square means with their standard errors)

* Treatments were: (1) no supplementation of linseed oil with low RUP:RDP ratio (NLSO-LR); (2) no supplementation of linseed oil with high RUP:RDP ratio (NLSO-HR); (3) supplementation of linseed oil with low RUP:RDP ratio (LSO-LR) and (4) supplementation of linseed oil with high RUP:RDP ratio (LSO-HR).
† Statistical comparisons: LSO = linseed oil supplement or no supplementation; RUP = different ratios of RUP:RDP (low ratio (LR) 27:73; high ratio (HR) 38:62); LSO × RUP = interaction between linseed oil and dietary RUP:RDP ratios.
‡ Sampling time effect was: T = time effect; T × LSO = interaction of time and linseed oil supplement; T × RUP = interaction of time and different ratios of RUP:RDP.
Purine derivatives and microbial protein synthesis
Results show that there was no difference among treatments for allantoin, uric acid and subsequently allantoin plus uric acid as purine derivatives (Table 4). In addition, no difference was found among experimental diets for microbial protein synthesis estimated through purine derivatives in the present study.
Table 4. Purine derivatives and microbial protein production in dairy calves fed supplemented linseed oil with low or high rumen undegradable protein (RUP):rumen degradable protein (RDP) ratios (n 12 calves per treatment) (Least square means with their standard errors)

* Treatments were: (1) no supplementation of linseed oil with low RUP:RDP ratio (NLSO-LR); (2) no supplementation of linseed oil with high RUP:RDP ratio (NLSO-HR); (3) supplementation of linseed oil with low RUP:RDP ratio (LSO-LR) and (4) supplementation of linseed oil with high RUP:RDP ratio (LSO-HR).
† Statistical comparisons: LSO = linseed oil supplement or no supplementation; RUP = different ratios of RUP:RDP (low ratio (LR) 27:73; high ratio (HR) 38:62); LSO × RUP = interaction between linseed oil and dietary RUP:RDP ratios.
Blood metabolites, liver enzymes and insulin
The data of blood metabolites, liver enzymes and insulin concentrations in serum are presented in Table 5. No interaction was found for LSO supplementation and RUP:RDP ratio considering blood metabolites, liver enzymes and insulin in the present study. The blood glucose concentration was increased (P = 0·03) in LSO-supplemented calves compared with non-supplemented calves (4·64 v. 4·27 mmol/l) during the pre-weaning period. Total cholesterol and HDL-cholesterol were increased in higher LSO-fed calves both during the pre- and post-weaning periods. Fat supplementation reduced blood TP concentration in post-weaning period (P = 0·04). Albumin was increased in calves fed with greater dietary RUP:RDP ratio in post-weaning period (P = 0·04). The concentration of β-hydroxybutyrate and blood urea N did not change among experimental treatments. The concentration of alanine aminotransferase showed a minor reduction in LSO-supplemented calves compared with non-supplemented calves on day 35 (P = 0·09; Table 5). However, aspartate aminotransferase concentration did not influence with experimental treatments. Blood insulin concentration was increased in LSO-supplemented groups compared with non-supplemented calves both during days 35 and 70 (P = 0·03). Blood insulin concentration tended to increase when a greater RUP:RDP ratio was fed (12·2 v. 9·5 IU/l; P = 0·08). Except blood TP and albumin, all blood metabolites had been influenced over time in the present study (P < 0·05).
Table 5. Blood metabolites, liver enzymes activity and insulin concentrations in dairy calves fed supplemented linseed oil with low or high rumen undegradable protein (RUP):rumen degradable protein (RDP) ratios (n 12 calves per treatment) (Least square means with their standard errors)
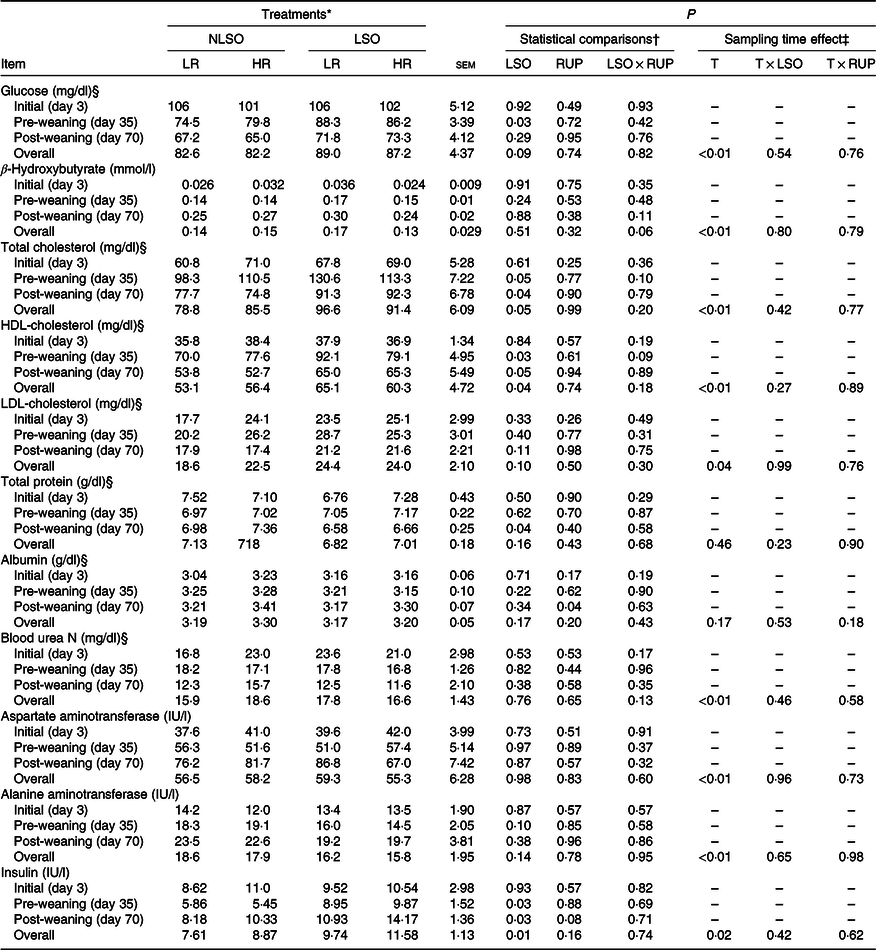
* Treatments were: (1) no supplementation of linseed oil with low RUP:RDP ratio (NLSO-LR); (2) no supplementation of linseed oil with high RUP:RDP ratio (NLSO-HR); (3) supplementation of linseed oil with low RUP:RDP ratio (LSO-LR) and (4) supplementation of linseed oil with high RUP:RDP ratio (LSO-HR).
† Statistical comparisons: LSO = linseed oil supplement or no supplementation; RUP = different ratios of RUP:RDP (low ratio (LR) 27:73; high ratio (HR) 38:62); LSO × RUP = interaction between linseed oil and dietary RUP:RDP ratios.
‡ Sampling time effect was: T = time effect; T × LSO = interaction of time and linseed oil supplement; T × RUP = interaction of time and different ratios of RUP:RDP.
§ To convert glucose in mg/dl to mmol/l, multiply by 0·0555. To convert cholesterol in mg/dl to mmol/l, multiply by 0·0259. To convert protein in g/dl to g/l, multiply by 10. To convert albumin in g/dl to g/l, multiply by 10. To convert blood urea N in mg/dl to mmol/l, multiply by 0·357.
Discussion
Fat supplementation as an intensified feeding strategy in starter diets could enhance energy provision to dairy calves during the early weeks of life(Reference Ghasemi, Azad-Shahraki and Khorvash9). Previous reports clarified that higher RUP:RDP ratio could possibly provide greater available amino acid in the small intestine(Reference Kazemi-Bonchenari, Mirzaei and Jahani-Moghadam20). We hypothesised that LSO supplementation which is a rich source of n-3 FA along with greater RUP:RDP ratio could improve growth performance in dairy calves. Growth rate tended to improve and FE increased in LSO-supplemented calves during the pre-weaning period; however, the greater RUP:RDP ratio improved aforementioned parameters during the post-weaning period. This clarifies that differences in dairy calves’ responses to fat supplementation and RUP:RDP ratio might be influenced by the physiological and metabolic development of the rumen. Some reports believe that rumen is not fully functional during the pre-weaning period; therefore, providing higher RUP:RDP ratio may have a minimal effect on the amount or composition of amino acids received in the small intestine(Reference Kazemi-Bonchenari, Mirzaei and Jahani-Moghadam20,22) . We found that feeding prilled SFA sources along with greater RUP level did not influence dairy calves’ performance in our previous work(Reference Kazemi-Bonchenari, Mirzaei and Jahani-Moghadam20). However, the growth rate tended to be greater, and the FE was improved in calves which received LSO in the present study. Fat supplementation in dairy calves has resulted in improved(Reference Ghasemi, Azad-Shahraki and Khorvash9,Reference Zhao, Etherton and Martin30) or decreased growth performance(Reference Hill, Bateman and Aldrich8,Reference Kuehn, Otterby and Linn31) . Ghasemi et al. (Reference Ghasemi, Azad-Shahraki and Khorvash9) reported that inclusion of soya oil in the starter diet of dairy calves increased ADG up to 91 and 273 g/d in contrast to control and mixed fat sources supplemented calves, respectively. The difference of metabolisable energy between NLSO and LSO starter diets was 0·50 MJ/kg (12·22 v. 12·72 MJ/kg for NLSO and LSO, respectively) which could partly influence gain and efficiency in dairy calves which is in line with previous reports(Reference Ghasemi, Azad-Shahraki and Khorvash9). Regardless of the fat level effects in starter diets on the performance of dairy calves, FA composition could also influence the growth and immunity(Reference Hill, Bateman and Aldrich10). Some studies indicated that fat sources rich in α-linolenic acid improved growth performance of dairy calves(Reference Hill, Bateman and Aldrich10,Reference Kadkhoday, Riasi and Alikhani32) . Generally, the mechanism for performance improvement in animals fed n-3 FA such as α-linolenic acid has been related to immune system enhancement(Reference Garcia, Shin and Schaefli33). Furthermore, consistent with previous reports on sparing effect of some FA on protein in ruminants(Reference Schiavon, Tagliapietra and Dalla Monta’17,Reference Van Sossten, Meyer and Piechotta18) , it seems that n-3 FA source feeding has the potential to have such an effect in dairy calves fed greater RUP:RDP ratio.
In the present study, the calves receiving the higher RUP:RDP ratio recorded greater ADG than those fed on a LR diet during the entire period, which resulted in greater final BW. Similarly, greater growth rate in dairy calves was reported in calves being fed diets containing higher RUP:RDP ratio(Reference Bunting, Fernandez and Fornea19). However, McCoy et al. (Reference McCoy, Ruppert and Hutjens34) reported that calves fed extruded soyabean did not gain as much as calves fed the soyabean-based starter. It is notable that regardless the RUP:RDP ratio in dairy calves, the source of RUP incorporated in starter diet could influence animal performance per se. For instance, xylose-treated soyabean meal is an RUP source that was replaced instead of unprocessed soyabean meal in dairy calves resulted in intake reduction that could be due to negative processing effect on animal intake(Reference Kazemi-Bonchenari, Alizadeh and Tahriri35). Another way is to replace a feedstuff containing a high RUP level such as maize gluten meal or fishmeal instead of the protein source in the basal diet(Reference Amirabadi Farahani, Amanlou and Kazemi-Bonchenari36). Gathering together the results obtained in previous works(Reference Bunting, Fernandez and Fornea19,Reference Kazemi-Bonchenari, Mirzaei and Jahani-Moghadam20,Reference McCoy, Ruppert and Hutjens34,Reference Kazemi-Bonchenari, Alizadeh and Tahriri35,Reference Chagas, Ferreira and Faciola37) , discrepancies among studies for the effects of RUP:RDP ratios on dairy calves’ growth rate responses might be attributed to the differences in the source and level of RUP feeding and different amino acid profiles of experimental feedstuffs included in the starter diet which could influence dairy calves’ performance.
All the above-mentioned performance traits responded to time throughout the study. This indicates that intake level, ADG and FE are influenced by animal age, which is mostly related to whole-body metabolism of the animal at different ages(Reference Chegini, Kazemi-Bonchenari and Khaltabadi-Farahani38). Zhao et al.(Reference Zhao, Gordon and O’Connell39) clarified that younger animals have better nutrients efficiency in comparison with older animals, which could influence whole-body metabolism towards better efficiency. Furthermore, different feed efficiencies before and after weaning may be partly related to greater milk feeding efficiency in comparison with solid feed which improves growing animal’s whole-body efficiency(Reference Kazemi-Bonchenari, Falahati and Poorhamdollah15,Reference Kazemi-Bonchenari, Mirzaei and Jahani-Moghadam20) .
Considering interaction of time with LSO, LSO supplementation proved to be more effective in early weeks of life (pre-weaning) than the post-weaning period in obtaining suitable faecal score (Fig. 1) as well as higher FE (Table 2), which is probably related to greater susceptibility of dairy calves in this critical period of life. In addition, significant interaction of time and RUP level for intake (Table 2) may also be related to the developing rumen in young calves which had been different responses into various RUP:RDP ratios in growing calves(Reference Kazemi-Bonchenari, Mirzaei and Jahani-Moghadam20).
The rectal temperatures of the calves in all treatments were within the normal range in this study. The synchronous feeding of greater RUP accompanied with LSO supplementation caused the least rectal temperature in the present study. Hill et al.(Reference Hill, Bateman and Aldrich10) stated that inclusion of a blend FA in starter diet (butyrate, medium-chain FA and α-linolenic acid) lessens the inflammatory effects of vaccination in dairy calves. Two-way interaction between time and LSO for body temperature may indicate the role of n-3 FA to prevent diseases and consequently low body temperature during the pre-weaning period. Three-way interactions (time × LSO × RUP) had no effect on any response observed in animals fed different experimental treatments; hence, this effect was not discussed furthermore.
Considering the faecal scores, results proved that LSO supplementation improved faecal score of dairy calves in pre-weaning period especially in the first 3 weeks of experiment (1·89 v. 1·55 for NLSO and LSO groups, respectively) (Fig. 1). Lower faecal scores for calves supplemented with LSO suggest that n-3 FA feeding has the potential to reduce diarrhoea in dairy calves during the pre-weaning period. Favourable effects of n-3 sources on dairy calves health and immunity were observed when n-3 source was fed in milk replacer(Reference Ballou and DePeters13,Reference Ballou, Cruz and Pittroff14) or in starter diet(Reference Hill, Bateman and Aldrich10). The basal starter diet in the present study was based on maize grain and soyabean meal of which both are deficient in α-linolenic acid(Reference Hill, Bateman and Aldrich10,Reference Ballou and DePeters13) . The neonatal calves are more susceptible in early weeks of life with respect to scouring and inflammation, and the present study findings showed the improvement of health and performance of dairy calves fed n-3 source in this critical period. The anti-inflammatory effects of n-3 FA have been previously reported(Reference Hill, Bateman and Aldrich12,Reference Ballou and DePeters13) . Beside some negative reports of fat supplementation in dairy calves performance(Reference Hill, Bateman and Aldrich8,Reference Kazemi-Bonchenari, Mirzaei and Jahani-Moghadam20) , notable positive responses of individual FA inclusion in dairy calf diets on immune system and performance also occur(Reference Hill, Bateman and Aldrich10,Reference Hill, Bateman and Aldrich12–Reference Ballou, Cruz and Pittroff14) which confirms that individual FA requirement of dairy calves especially during the pre-weaning period needs to be re-evaluated. Regardless of the experimental diet effects, faecal scores influenced with time and were lower in early weeks of life (Fig. 1). This was consistent with some of previous reports that calves had more diarrhoea incidences in the first few weeks after birth(Reference Kazemi-Bonchenari, Mirzaei and Jahani-Moghadam20,Reference Kadkhoday, Riasi and Alikhani32) .
The improvements in skeletal growth indices for wither height and hip height with LSO supplementation were observed on the weaning day. Results regarding skeletal growth found in the present study are in agreement with those reported by Heinrichs et al. (Reference Heinrichs, Erb and Rogers26) who showed a positive correlation between growth rate and skeletal growth indices. Similar to growth rate and FE status in the present study, skeletal growth indices had a positive influence with supplemented LSO in pre-weaning period. It seems that greater energy content in starter diet supplied by LSO caused an improvement in growth performance. n-3 FA supplementation on growth quality and its accretion in animal tissues related to human nutrition require more research. All skeletal growth indices were affected by time. Because the calves were in the growing stages throughout the experiment, all measured skeletal indices improved by age at weaning and thereafter.
Neither supplemented fat nor changed RUP:RDP ratio in dairy calves changed microbial protein production in the present study. To the best of our knowledge, the present study is the first report regarding the interaction effect of fat and protein (RUP:RDP ratio) on microbial protein synthesis in post-weaned calves. According to findings reported in dairy cows studies, total DM intake could be a main factor on microbial protein synthesis by providing organic matter for microbial fermentation(Reference Clark, Klusmeyer and Cameron40). Solid feed intake was similar among treatments in the present study; therefore, organic matter provision for microbial fermentation could be the same. More studies are needed to evaluate the different nutrients effect on microbial protein synthesis in dairy calves.
Glucose was increased in the LSO-supplemented calves during the post-weaning period probably due to the sparing effect of fat for glucose which has been previously explained in dairy calves(Reference Ghasemi, Azad-Shahraki and Khorvash9). However, in another study(Reference Kazemi-Bonchenari, Mirzaei and Jahani-Moghadam20), glucose did not change in saturated free FA-supplemented starter diet, while fat addition reduced starter feed intake. In fact, it seems that blood glucose concentration is related to changes in total starter feed intake as well as sparing effect of supplemented fat on glucose metabolism. Total cholesterol as well as HDL-cholesterol increased in the present study similar to the most studies those reported greater blood cholesterol concentration in calves received fat-supplemented starter diets(Reference Ghasemi, Azad-Shahraki and Khorvash9,Reference Kazemi-Bonchenari, Mirzaei and Jahani-Moghadam20) . Some veterinary findings suggest that the calves with deficient cholesterol status are more susceptible to diarrhoea and illness(Reference Duff, Passant and Wessels41). Supplementing starter diet with fat sources could stimulate cholesterol synthesis in the liver of dairy calves(Reference Drackley, Burrin and Mersmann42). We assume that improvement in gain, growth and FE of pre-weaned calves supplemented with LSO in the present study might partly be related to the increased health status of the calves through HDL-cholesterol enhancement in these calves.
Fat addition reduced blood TP concentration in the present study. The reduction of blood TP concentration might be related to the blood lipid transport system. Fats are transported in bovine blood as α- and β-lipoproteins and as chylomicrons produced in the small intestine wall(Reference Chandler, McCarthy and Kesler43). It seems that because of similar protein content of the experimental diets in the present study, increased demand for lipoprotein synthesis in fat-supplemented calves negatively affected total amino acid pool for albumin synthesis and consequently decreases the level of blood TP concentration. Previously, reduced TP content of blood was reported by Kazemi-Bonchenari et al.(Reference Kazemi-Bonchenari, Mirzaei and Jahani-Moghadam20) when feeding 6 v. 3 % dietary fat level in dairy calves. None of the blood metabolites except blood albumin during the post-weaning period was influenced by RUP:RDP ratio in the present study. It seems that greater RUP:RDP ratio did not influence blood metabolites at pre-weaning period. However, the greater RUP:RDP ratio increased blood albumin concentration at post-weaning period. During the pre-weaning period, rumen is still under-developing and rumen microbial and physiological functions are not as the same as to ruminants(Reference Kazemi-Bonchenari, Mirzaei and Jahani-Moghadam20,Reference Chandler, McCarthy and Kesler43) . Contrarily, calf feeding relies only on starter intake and not on milk or milk replacers during the post-weaning period.
Blood insulin concentration increased at pre- as well as post-weaning periods in calves supplemented with n-3 FA from LSO. Our results confirmed that supplemented LSO in dairy calves’ starter diet caused greater insulin concentration in the blood (9·25 v. 12·55 IU/l for LSO and NLSO groups, respectively). This was found probably by supplying greater available energy for the animal in LSO-supplemented diets compared with NLSO diets (12·22 v. 12·72 MJ/kg for NLSO and LSO, respectively; Table 1). The other probable mechanism may be related to increased blood insulin concentration in response to supplemented some individual FA which was reported previously in beef cows fed with polyunsaturated plant FA(Reference Williams and Stanko44). Furthermore, sparing effect of FA for amino acid and glucose could increase these metabolite concentrations in the blood(Reference Ghasemi, Azad-Shahraki and Khorvash9) that consequently both glucose(Reference Kazemi-Bonchenari, Salem and Lopez45) and amino acids(Reference Lal and Chungh46) had potential to increase blood insulin concentration. The young calves are highly sensitive to insulin similar to non-ruminants(Reference Leat and Phillipson47), and long-term effect of insulin concentration in dairy calves on future insulin sensitivity status may be a controversial issue. Yunta et al. (Reference Yunta, Terré and Bach48) studied this concept through performing a glucose tolerance test, 4-h postprandial on 42, 86 and 300 d of life in replacement heifers that were fed 2, 3 or 4 litres meals of milk replacer twice per d and weaned on 63 d of life. They concluded that feeding an elevated plane of milk replacer (8 litres/d) is associated with a decrease in insulin sensitivity pre-weaning, but there would be no carry-over effect in the post-weaning period. Bach et al. (Reference Bach, Domingo and Montoro49) also cleared that there is responsiveness of insulin by level of milk replacer offered to young calves. As far as the authors know, it is currently unknown in dairy calves whether pre-weaning nutrition associated with altered (increased or decreased) insulin sensitivity has a carry-over effect in the post-weaning period. Regardless the long-term effect of blood insulin concentration on dairy calves, the positive effects of insulin concentration on growth performance of ruminants have been previously proved(Reference Wester, Lobley and Birine50). More researches need to clarify the short- and long-term effects on blood insulin concentration in dairy calves on future insulin sensitivity status. Most of the blood metabolites were influenced over time in the present study. Previous studies clarified that as pre-weaned ruminant aged, the blood glucose concentration decreased and blood β-hydroxybutyrate concentration increased(Reference Soltani, Kazemi-Bonchenari and Khaltabadi-Farahani51) which is correlated with the greater solid feed intake(Reference Ferreira and Bittar52) and consequently rumen development. Overall, we found that there was no interaction between LOS supplementation and RUP:RDP ratios on growth performance of dairy calves showing individual mode of action for each of them in young dairy calves’ metabolism.
Conclusion
The present study findings suggest that supplementing LSO, rich in α-linolenic acid, in starter diet (2·5 %, DM basis) might improve FE especially during the pre-weaning period. Contrary, greater RUP:RDP ratio might be recommendable in the post-weaning period of diary calves. No interaction was found regarding growth performance of dairy calves between LSO supplementation and dietary RUP:RDP ratios. More research with different types of fat and protein sources and RUP:RDP ratios may give more insight into the underlying mechanisms, which can be used to optimise fat and protein feeding strategies in dairy calves.
Acknowledgements
Special thanks to Dr A. Fazlali (deputy of research and technology of Arak University) and Dr Y. Payandeh for their assistances with performing the experiments. Deep appreciations to Dr Moshayedi, manager in-chief and Mr Sadeghi, technical manager of the Zarrin-Khoosheh commercial dairy farm as well as its helpful staff for their cooperation in conducting the study. The authors thank Dr H. Sadri (Institute of Animal Science, University of Bonn, Germany) for valuable comments related to insulin metabolism in dairy calves.
Costs for the project were mainly financed by Ministry of Science Research and Technology of Iran. Authors appreciate for partial financial supports done by Kimia Danesh Alvand Company.
M. K.-B. was the main leader of the current project from the beginning until the paper submission. M. D.-B. gave consultations in performing the experiment. F. F. collected some valuable literature for writing the paper. A. S.-B. collected the data and performed laboratory analysis. M. J.-M. collected the data. M. M. carried out some valuable literature reviews for the team.
The authors declare that there is no conflict of interest regarding the publication of this paper.