Introduction
Over 12% of extant bird species are threatened with extinction (Brooks et al. Reference Brooks, Collar, Green, Marsden and Pain2008), 58.8% of which are island species (Tershy et al. Reference Tershy, Shen, Newton, Holmes and Croll2015). Natural extinction rates are higher in island populations than in mainland populations. Sensitivity to environmental change, predation by introduced predators, and small population sizes are all common characteristics among island avifaunas; therefore, island endemic species are at an increased risk of extinction (Frankham Reference Frankham1998). Taxonomic patterns in extinction risk suggest that families containing high numbers of endemic species are more susceptible to extinction (Lockwood et al. Reference Lockwood, Brooks and Mckinney2000). Resolution of unknown taxonomic and evolutionary relationships can therefore contribute to a greater understanding of extinction vulnerabilities among bird groups (Lockwood et al. Reference Lockwood, Brooks and Mckinney2000, Johnson et al. Reference Johnson, Adler and Cherry2007).
Advances in systematic biology have allowed phylogenetic information to be combined with phenotypic data to further infer the evolutionary processes, based on the assumption that phenotypic traits within groups of species will be influenced by shared ancestry (Cressler et al. Reference Cressler, Butler and King2015, Bastide et al. Reference Bastide, Solís-Lemus, Kriebel, Sparks and Ané2018). Recently, comparative phylogenetic methods for missing data imputation have been employed to predict physiological adaptations, conservation status, and functional life history traits for data deficient species (Riek and Bruggeman Reference Riek and Bruggeman2013, Swenson Reference Swenson2014, González-del-Pliego et al. Reference González-del-Pliegoy, Freckleton, Edwards, Koo, Scheffers, Pyron and Jetz2019, James et al. Reference James, Salguero-Gómez, Jones, Childs and Beckerman2021). Predictive models are particularly useful for estimation of trait values when species are rare and elusive, locations are remote, and fiscal constraints limit field surveys (Wood et al. Reference Wood, Stillman and Hilton2018, Horswill et al. Reference Horswill, Manica, Daunt, Newell, Wanless, Wood and Matthiopoulos2021). Life history traits such as generation time, longevity, male and female maturity, clutch size, and incubation and fledging times can be used to evaluate the capability of a species to adapt to climate change and habitat fragmentation as well as being essential to estimate population trends (Mace et al. Reference Mace, Collar, Gaston, Hilton-Taylor, Akçakaya, Leader-Williams, Milner-Gulland and Stuart2008, Pearson et al. Reference Pearson, Stanton, Shoemaker, Aiello-Lammens, Ersts, Horning, Fordham, Raxworthy, Ryu, Mcnees and Akçakaya2014, Storchová and Hořák Reference Storchová and Hořák2018, Cuervo and Møller Reference Cuervo and Møller2019, Horswill et al. Reference Horswill, Manica, Daunt, Newell, Wanless, Wood and Matthiopoulos2021).
Pigeons and doves (Columbiformes; Columbidae) are one of the oldest lineages of birds, inhabiting a wide range of habitats on six of the seven continents, despite which their evolutionary history is still poorly resolved (Pereira et al. Reference Pereira, Johnson, Clayton and Baker2007, Soares et al. Reference Soares, Novak, Haile, Heupink, Fjeldså, Gilbert, Poinar, Church and Shapiro2016). Neotropical columbids may form the most ancient lineage (Johnson and Clayton Reference Johnson and Clayton2000b, Shapiro et al. Reference Shapiro, Sibthorpe, Rambaut, Austin, Wragg, Bininda-Emonds, Lee and Cooper2002) but have been little studied, with fundamental taxonomy, conservation status, ecological and life-history data still largely unknown (Brooks et al. Reference Brooks, Collar, Green, Marsden and Pain2008, Devenish-Nelson et al. Reference Devenish-Nelson, Weidemann, Townsend and Nelson2019). Therefore, gathering information about Neotropical species is essential and urgent for conservation of this diverse group of birds (Latta Reference Latta2012).
The Neotropical columbid genus Leptotila comprises 11 morphologically similar species, of which one, the Grenada Dove L. wellsi, is ‘Critically Endangered’ (BirdLife International 2018). Endemic to Grenada in the eastern Caribbean Sea, the Grenada Dove is found in two seemingly isolated populations in the west and south-west of the island (Rusk et al. Reference Rusk, Temple, Wege, Wallace, Blockstein, Ledec and Sorenson2008, Rusk Reference Rusk2017). The most recent population assessment estimated that 160 ± 30 individuals remain of this species and showed a decreasing population trajectory (Rivera-Milán et al. Reference Rivera-Milán, Bertuol, Simal and Rusk2015, BirdLife International 2018). Important details about the species’ biology, such as longevity and incubation period, are currently unreported. The Grenada Dove was once considered a subspecies of the Grey-fronted Dove L. rufaxilla until reclassified as a distinct species based on morphological and acoustic evidence (Blockstein and Hardy Reference Blockstein and Hardy1989). However, its genetic distinctiveness and relationships have never been investigated. Anecdotal evidence indicates that the Grenada Dove is closely related to the Caribbean Dove L. jamaicensis due to morphological similarities (Anthony Jeremiah, Head of Forest and National Parks Department, Government of Grenada, pers. comm.).
By investigating both the evolutionary and life history of the Grenada Dove, and by estimating its phylogenetic placement, we were able to predict currently unreported life history traits. We extracted DNA from Grenada Dove samples and obtained sequences from three mitochondrial DNA markers: Cytochrome oxidase I (COI), NADH dehydrogenase 2 (ND2) and Cytochrome b (Cyt b); and one nuclear marker: β-Fibrinogen intron 7 (β-FIB). We used these markers first to infer the evolutionary history of this species amongst other Neotropical columbid species. We then used phylogenetic comparative methods for missing data imputation, based on this evolutionary history, to predict unknown life history trait values to inform conservation management decisions.
Methods
DNA Extraction and PCR
Non-invasively collected eggshell and feather samples (n = 2) were collected in 2018 and 2017, respectively. Samples were transported by airmail to the UK in June 2018 where they were cleaned with 70% ethanol and stored at −20°C. DNA barcoding was used to confirm species identification for the samples using methods outlined in Patel et al. (Reference Patel, Waugh, Millar and Lambert2010). As this is the first time Grenada dove DNA has been obtained, there is no reference available for this species. However, as it is the only member of its genus resident on Grenada, where all of our samples were collected, we conducted a BLAST® search with the resulting data and found that sequences obtained for this study were consistent with previously obtained sequences for Leptotila. We used the optimised method for DNA extraction, using the QIAGEN DNeasy® Blood and Tissue kit and the primerless PCR protocol, outlined in Peters et al. (Reference Peters, Nelson, Rusk and Muir2019).
The substrate from the primerless PCR process was used to amplify the mitochondrial markers Cyt b (882 bp), COI (613 bp) and ND2 (949 bp) and the nuclear marker β-FIB (902 bp). Primer sequences and the corresponding annealing temperatures are given in Supporting Information 1. PCR cycling parameters for all except the COI region were: initial denaturation at 95°C for 5 min, 45 cycles of 95°C for 30 s, 50–60°C (primer specific, see Appendix S1 in the online supplementary material) for 30 s, 72°C for 60 s and a final extension at 72°C for 5 min; and for the COI region: 95°C for 5 min, 10 cycles of 95°C for 30 s, 55°C for 30 s, 72°C for 60 s, 35 cycles of 95°C for 30 s, 50°C for 30 s, 72°C for 60 s and a final extension at 72°C for 5 min. Where regions could not be amplified using a single primer set, additional primers were designed using Primer3 (Untergasser et al. Reference Untergasser, Cutcutache, Koressaar, Ye, Faircloth, Remm and Rozen2012) allowing a small amplicon strategy to be used where regions were amplified in smaller overlapping sections (as per Peters et al. Reference Peters, Nelson, Rusk and Muir2019).
Prior to sequencing, samples were purified as per the manufacturer’s protocol using the QIAquick PCR Purification kit (QIAGEN Inc., Crawley). Preparation and submission of samples were carried out according to the Eurofins Genomics guidelines for the Mix2Seq kit (Eurofins Genomics, Luxembourg) where sequencing was performed using Sanger sequencing methods. As the primerless PCR technique has the potential to increase the chance of sequencing error, amplification and sequencing were performed in triplicate in order to obtain consensus data for each region (Peters et al. Reference Peters, Nelson, Rusk and Muir2019, Weber et al. Reference Weber, Stewart, Garza and Lehman2000). Sequence reconstruction and alignments were conducted using Sequencher 5.4.6 (Gene Codes Corporation 2017).
Phylogenetics
Sequences from 24 Neotropical columbids with Common Crane Grus grus as an outgroup were obtained for all markers from Genbank (Benson et al. Reference Benson, Cavanaugh, Clark, Karsch-Mizrachi, Lipman, Ostell and Sayers2013; Table 1). Phylogenetic tree construction was performed using MEGAX 10.2.4 (Kumar et al. Reference Kumar, Stecher, Li, Knyaz and Tamura2018). We used Maximum Likelihood to fit 24 different nucleotide substitution models and used Akaike information criterion (AIC) and Bayesian information criterion (BIC) to select the best models (Schwarz Reference Schwarz1978, Akaike Reference Akaike1987, Nei and Kumar Reference Nei and Kumar2000).
Table 1. List of species and their Genbank accession number for all sequences used in phylogenetic analysis.

Sources:
1 (Clayton et al. Reference Clayton, Bush, Goates and Johnson2003)
2 (Ericson et al. Reference Ericson, Anderson, Britton, Elzanowski, Johansson, Källersjö, Ohlson, Parsons, Zuccon and Mayr2006)
3 (Johnson & Clayton, Reference Johnson and Clayton2000a)
4 (Johnson & Clayton, Reference Johnson and Clayton2000b)
5 (Johnson et al., Reference Johnson, De Kort, Dinwoodey, Dinwoodey, Mateman, Cate, Lessells and Clayton2001)
6 (Johnson & Weckstein, Reference Johnson and Weckstein2011)
7 (Kerr et al. Reference Kerr, Stoeckle, Dove, Weigt, Francis and Hebert2006)
8 (Kerr et al. Reference Kerr, Stoeckle, Dove, Weigt, Francis and Hebert2007)
9 (Kerr et al. Reference Kerr, Lijtmaer, Barreira, Hebert and Tubaro2009)
10 (Krajewski et al. Reference Krajewski, Sipiorski and Anderson2010)
11 (Li et al. Reference Li, Shi and Shi2015)
12 (Miller et al. Reference Miller, Bermingham and Winker2008)
13 (Monceau et al. Reference Monceau, Wattier, Moreau, Motreuil and Cezilly2012)
14 (Monceau et al. Reference Monceau, Cézilly, Moreau, Motreuil and Wattier2013)
15 (Pacheco et al. Reference Pacheco, Battistuzzi, Lentino, Aguilar, Kumar and Escalante2011)
16 (Pereira et al. Reference Pereira, Johnson, Clayton and Baker2007)
17 (Prychitko and Moore Reference Prychitko and Moore2003)
18 (Qu Reference Qu2018)
19 (Santiago-Alarcon et al. Reference Santiago-Alarcon, Rodríguez-Ferraro, Parker and Ricklefs2014)
20 (Schindel et al. Reference Schindel, Stoeckle, Milensky, Trizna, Schmidt, Gebhard and Graves2011)
21 (Shapiro et al. Reference Shapiro, Sibthorpe, Rambaut, Austin, Wragg, Bininda-Emonds, Lee and Cooper2002)
22 (Soares et al. Reference Soares, Novak, Haile, Heupink, Fjeldså, Gilbert, Poinar, Church and Shapiro2016)
23 (Sorenson et al. Reference Sorenson, Oneal, García-Moreno and Mindell2003)
24 (Stoeckle Reference Stoeckle2011)
25 (Sweet and Johnson Reference Sweet and Johnson2015)
26 (Tavares et al. Reference Tavares, Gonçalves, Miyaki and Baker2011)
The Hasegawa-Kishino-Yano (HKY) model using a discrete Gamma distribution (+G) was the best-supported model for the combined mitochondrial and nuclear DNA dataset of 3,346 bp and thus evolutionary analyses were performed using this model with 500 bootstrap replicates (Hasegawa et al. Reference Hasegawa, Kishino and Yano1985). In order to check the consistency of this tree we also built the tree using the *BEAST approach implemented in BEAST v2.6.6 (Heled and Drummond Reference Heled and Drummond2010, Bouckaert et al. Reference Bouckaert, Vaughan, Barido-Sottani, Duchêne, Fourment, Gavryushkina, Heled, Jones, Kühnert, De Maio, Matschiner, Mendes, Müller, Ogilvie, Du Plessis, Popinga, Rambaut, Siveroni, Suchard, Wu, Xie, Zhang, Stadler and Drummond2019) and the resulting species tree provided the same topology as the concatenated tree (for details see Appendix S2). As mtDNA tends to have a larger proportion of variable bases than nDNA (Allio et al. Reference Allio, Donega, Galtier and Nabholz2017), we also inferred the position of the Grenada Dove within the Leptotila genus based on the mtDNA and nDNA separately, to provide a comparison between the two marker types, using the following best-supported models as per AIC and BIC: General Time Reversible model ([+I], 38.01% sites), for a total of 2,444 bp of mtDNA (Tavaré Reference Tavaré1986), and Tamura 3-parameter model, for a total of 902 bp of nDNA (Nei and Kumar Reference Nei and Kumar2000). We also inferred the position of the Grenada Dove in relation to L. jamaicensis, using a reduced genetic dataset using the Hasegawa-Kishino-Yano model (+G, parameter = 0.2496) for a total of 2,720 bp of ND2, Cyt b and β-FIB.
Time-calibrated phylogenies were reconstructed to infer molecular-based estimates of divergence by applying the RelTime-ML method following the protocol outlined in Mello (Reference Mello2018). Fossil calibrations are widely used for molecular dating, but no fossil records are available for the taxa used in this study (Ksepka et al. Reference Ksepka, Parham, Allman, Benton, Carrano, Cranston, Donoghue, Head, Hermsen, Irmis, Joyce, Kohli, Lamm, Leehr, Patané, Polly, Phillips, Smith, Smith, Van Tuinen, Ware and Warnock2015, Peters and McClennen Reference Peters and McClennen2015). We therefore used confidence intervals of minimum and maximum boundary estimations from the TimeTree database, which provides divergence time estimates from published studies integrated with a geological timescale, to calibrate divergence time (Kumar et al. Reference Kumar, Stecher, Suleski and Hedges2017, Mello Reference Mello2018, Tamura et al. Reference Tamura, Battistuzzi, Billing-Ross, Murillo, Filipski and Kumar2012, Reference Tamura, Tao and Kumar2018).
Life history trait imputation
To predict life history traits for the Grenada Dove we used multivariate phylogenetic comparative methods for missing data imputation implemented in the Rphylopars package version 0.3.0 (Goolsby et al. Reference Goolsby, Bruggeman and Ané2017) in R version 3.6.2 (R Core Team 2020). This approach uses a multivariate procedure of phylogenetic covariance across species to predict differences in life history traits (Goolsby et al. Reference Goolsby, Bruggeman and Ané2017, James et al. 2020). We imputed phylogenetic covariance across 24 Neotropical columbid species. Twelve life history traits - clutch size, clutches per year, incubation time, hatching weight, fledging age, longevity, generation length, male and female maturity, no-sex body mass (unreported sex; Myhrvold et al. Reference Myhrvold, Baldridge, Chan, Sivam, Freeman and Ernest2015), and male and female body mass - were obtained for each of these species, where available, from primary and grey literature (Appendix S3; Myhrvold et al. Reference Myhrvold, Baldridge, Chan, Sivam, Freeman and Ernest2015, HBW Alive 2020).
Available life history trait values for the Grenada Dove were a clutch size of two eggs (Rusk, unpubl. data), an imputed generation length of 4.2 years calculated using the IUCN generation length calculator (BirdLife International 2018), a fledging age of 14 days from focal nest observations and an estimate of two or three nesting attempts per year (Rusk, unpubl. data). We imputed these traits to determine whether multivariate phylogenetic comparative methods generated similar values. We fitted evolutionary models and used AIC and BIC as well as assessing model parameters to select the best fitting model. Phylogenetic signals were calculated using a maximum-likelihood approach to quantify the relationship between life history trait values and phylogenetic signal in order to ascertain model precision per life history trait (Penone et al. Reference Penone, Davidson, Shoemaker, Marco, Brooks, Young, Graham, Costa, Di Marco, Rondinini, Brooks, Young, Graham and Costa2014, James et al. Reference James, Salguero-Gómez, Jones, Childs and Beckerman2021). We tested the accuracy of the RPhylopars method by predicting known values, which we removed individually from the dataset. We focused on the genus Columbina as this genus contained the most known data, which allowed us to sequentially remove a total of 20% of these known values for each trait with minimal decrease in the accuracy of predictions (Penone et al. Reference Penone, Davidson, Shoemaker, Marco, Brooks, Young, Graham, Costa, Di Marco, Rondinini, Brooks, Young, Graham and Costa2014). We compared the predicted values from RPhylopars to the true value to assess the accuracy of predictions. We also calculated the median values, used in some life-history imputation without explicit phylogenetic information (e.g. Bird et al. Reference Bird, Martin, Akçakaya, Gilroy, Burfield, Garnett, Symes, Taylor, Şekercioğlu and Butchart2020), for fledging age and longevity.
Results
Phylogenetics
There were no polymorphisms observed between the two Grenada Dove sequences. There were 27 nucleotide polymorphisms across 3,346 bp that were unique to the Grenada Dove and not present in any other Leptotila species used in this investigation, consisting of 21 transition mutations, two transversion mutations and one transition/transversion mutation (depending on the Leptotila species) in the mtDNA and three transitional mutations in the nDNA β-FIB gene. The phylogenetic tree constructed using concatenated sequence data - Cyt b (882 bp), COI (613 bp), ND2 (949 bp), and β-FIB (902 bp) - for Neotropical columbids indicates that the Grenada Dove falls within the monophyletic group formed by Leptotila species (Figure 1a.). The Grenada Dove shared a most recent common ancestor with its sister group containing L. cassinii and L. plumbeiceps approximately 2.53 million years ago (mya) (Figure 2a). The smallest genetic distance (P = 0.0303) where 100 nucleotides were polymorphic (Table 2) was identified between the Grenada Dove and L. cassinii. The phylogenetic tree built with the reduced data and containing L. jamaicensis (Figure 3) still shows a sister relationship between the Grenada Dove and L. cassinii and L. plumbeiceps.

Figure 1. Phylogenetic analysis for 24 Neotropical columbid species and outgroup using the Maximum Likelihood method. Grenada Dove sequences indicated by the circular symbol; a. all mitochondrial and nuclear sequence data estimated using the Hasegawa-Kishino-Yano model (+G, parameter = 0.2281); b. mtDNA using General Time Reversible model ([+I], 38.01% sites); c. β-fibrinogen using the Tamura 3-parameter model. The trees are drawn to scale, with branch lengths measured in the number of substitutions per site and nodes supported by bootstrap values of >80%.

Figure 2. Time-calibrated phylogenies for Leptotila species and outgroup using the RelTime-ML method a. all mitochondrial and nuclear sequence data estimated using the Hasegawa-Kishino-Yano model (+G, parameter = 0.1547), b. mtDNA General Time Reversible model ([+I], 35.97% sites) c. β-fibrinogen using the Tamura 3-parameter model. Number scale indicates million years ago (mya). Nodes indicate 7 estimated divergence time calibration points of estimated divergence time ranges in million years ago (mya) obtained using the TimeTree resource (Kumar et al. Reference Kumar, Stecher, Suleski and Hedges2017).
Table 2. The number of substitutions per site (p-distance) and the number of base differences (polymorphic sites) between sequences for a total of 3,346 bp positions in the final dataset. *2,720 bp positions in the final dataset.
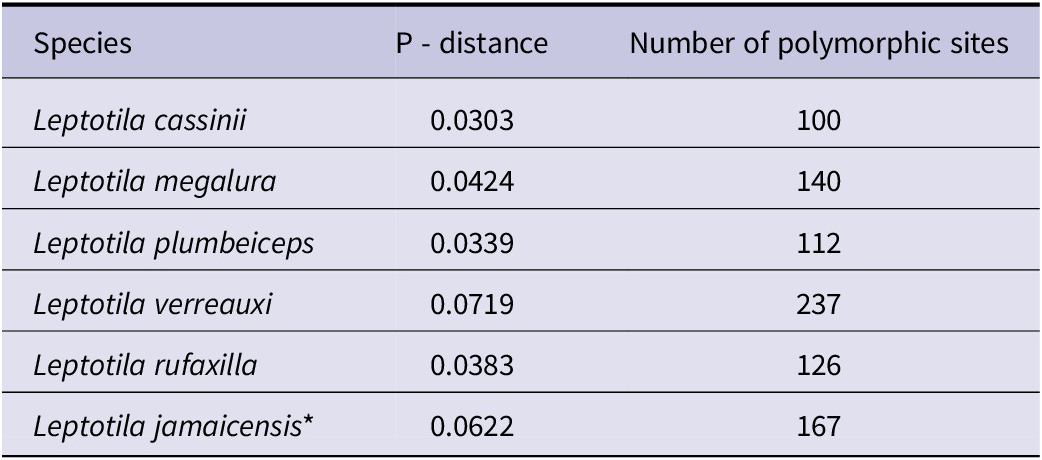
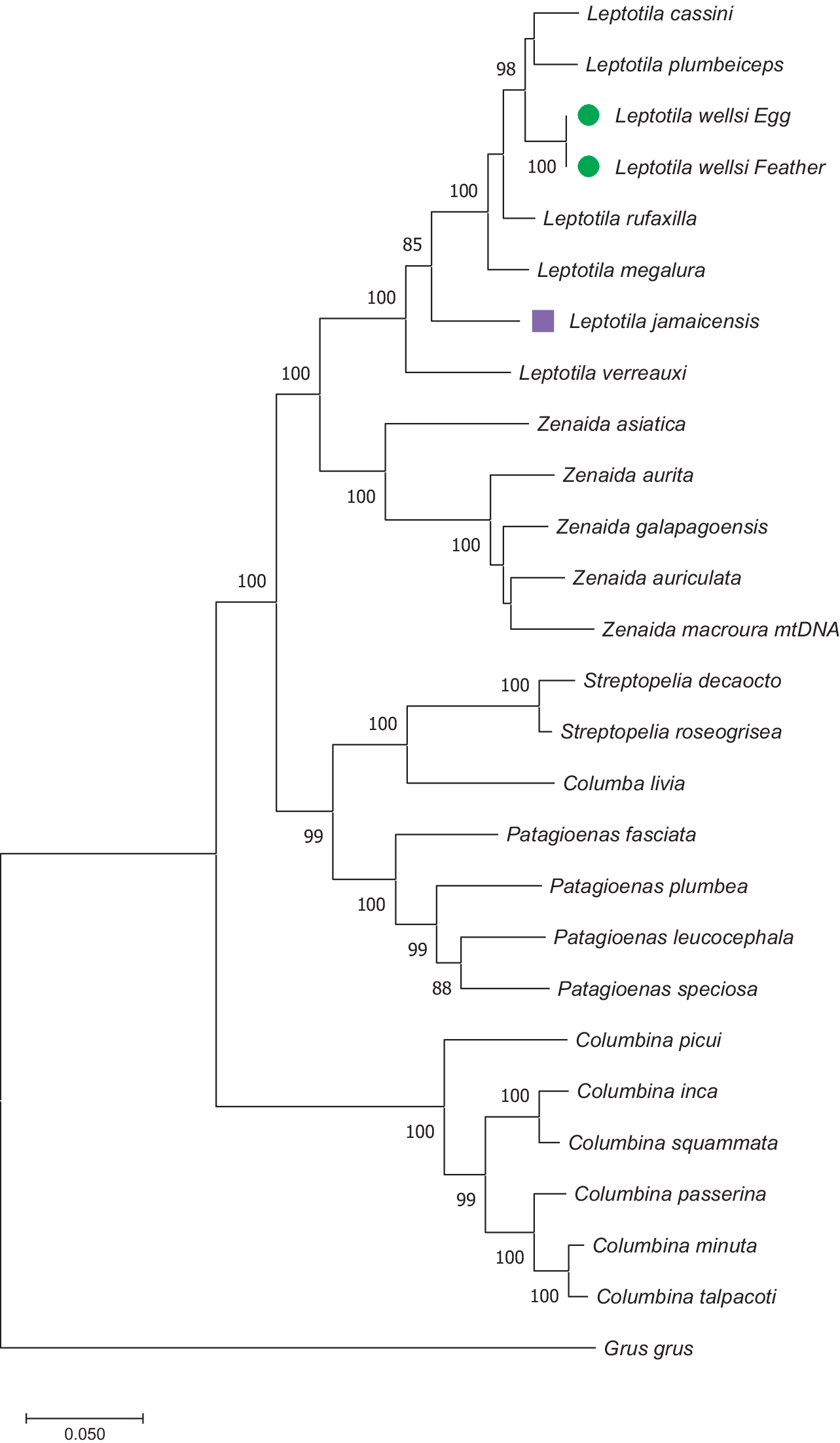
Figure 3. Phylogenetic analysis for 25 Neotropical columbid species and outgroup using the Maximum Likelihood method. Grenada Dove sequences indicated by the circular symbol and Leptotila jamaicensis are indicated by a square using the Hasegawa-Kishino-Yano model (+G, parameter = 0.2496). Tree drawn to scale, with branch lengths measured in the number of substitutions per site and nodes supported by bootstrap values of >80%.
The mitochondrial phylogenetic trees shown in Figures 1b and 2b suggests that the Grenada Dove shared its most recent common ancestor with L. cassinii and L. plumbeiceps approximately 2.18 mya, which is consistent with the phylogenetic tree constructed with the concatenated data set in Figure 1a. The nuclear phylogenetic trees shown in Figures 1c and 2c suggest that the Grenada Dove shared its most recent common ancestor with L. megalura and L. ruffaxilla approximately 2.75 mya, dissimilar to the most recent common ancestor shown by the phylogenetic trees constructed with the combined (Figure 1a and 2a) and mtDNA (Figure 1b and 2b) datasets. Analysis of the β-FIB gene relevels an indel (Appendix S4) in L. cassinii and L. plumbeiceps sequences which is not shared by the Grenada Dove or any of the other Leptotila species included in this study.
Life history trait imputation
Strong phylogenetic signals were revealed for clutch size, no-sex body mass, and male and female body mass, whereas weak phylogenetic signal was detected for clutches per year, incubation time, hatching weight, fledging age, longevity, and generation length (Appendix S5). Model validation (Figure 4) showed lower variation relative to each trait around seven of the twelve life history trait predictions including clutch size, clutches per year, incubation time, hatching weight, fledging age, longevity, and generation length and were therefore considered accurate. However, the remaining five life history trait predictions including male and female maturity, no-sex body mass, and male and female body mass showed a high level of variation from the true value and therefore were discounted. Despite suggested trait accuracy from the model validation for fledging age and longevity, we report large standard error values in addition to weak phylogenetic signal, and as such, genus medians were considered a more accurate representation for these traits. Known values for generation length for Leptotila are themselves imputed using the IUCN generation length calculator, and thus we discounted this trait from our results. Overall, six life history trait values were predicted for the Grenada Dove with confidence (Figure 5) using multivariate and single imputation procedures: a. clutch size = 2 (±0.09 SE) eggs, b. clutches per year = 1.4 (±0.81 S.E.), c. incubation time=14.2 (±0.75 SE) days. d. hatching weight = 3.8 g (± 1.05 SE) grams, fledging age = 15.5 days (genus median) days, f. longevity = 8.6 years (genus median).

Figure 4. Plot displaying the squared difference between the eb model predicted value and the true value divided by the trait median using the genus Columbina along with Normalized Root Mean Squared Error [NRMSE (Penone et al. Reference Penone, Davidson, Shoemaker, Marco, Brooks, Young, Graham, Costa, Di Marco, Rondinini, Brooks, Young, Graham and Costa2014, James et al. Reference James, Salguero-Gómez, Jones, Childs and Beckerman2021)]. Raw data are represented by the ° and the first, second and third quartile are represented by the box plot. Mean Squared Error per life history trait.

Figure 5. Predicted trait values with upper and lower 95% confidence intervals for the Grenada Dove Leptotila wellsi in comparison with other Leptotila species for the following lift history traits using the EB model: a. clutch size, b. clutches per year, c. incubation time, d. hatching weight; and median estimates: e. fledging age, f. longevity. Species of interest highlighted red. Known trait values represented by individual grey point.
Discussion
This study reports the first genetic data obtained for the Grenada Dove and supports a sister relationship with Grey-chested Dove L. cassinii along with Grey-headed Dove L. plumbeiceps, whose ranges span Central America into Colombia (BirdLife International 2021). We provide estimations for six previously unpublished life history traits for the Grenada Dove some of which, such as clutch size and clutches per year, have been found to be correlated with extinction risk (Parlato et al. Reference Parlato, Armstrong and Innes2015).
Leptotila cassinii was the species with the smallest genetic distance from the Grenada Dove (P = 0.0303) with 100 bp nucleotide polymorphisms based on concatenated sequence data and phylogenetic reconstruction. The most recent common ancestor was shared approximately 2.53 mya between L. cassinii, L. plumbeiceps, and the Grenada Dove. However, when the mtDNA and nDNA are considered separately our results show disparity. The mtDNA shows the same relationship displayed by the concatenated data described above, but nDNA analysis revealed that the most recent common ancestor was shared between L. rufaxilla, L. megalura, and the Grenada Dove. The mito-nuclear incongruence may have arisen for a variety of reasons; a larger amount of mtDNA sequence data was used providing a stronger phylogenetic signal, the differing characteristics between these markers such as differential lineage sorting and linkage disequilibrium, all of which can lead to ambiguous patterns of variation and evolutionary inferences (Hurst and Jiggins Reference Hurst and Jiggins2005, Rubinoff and Holland Reference Rubinoff and Holland2005). Overall, we identify L. cassinii and L. plumbeiceps as the species sharing the most recent common ancestor with the Grenada Dove, however, we recommend caution due to the disparity between the mitochondrial or nuclear phylogeny. Nevertheless, our result was unexpected as L. cassinii is predominantly a Central American species and has a larger geographical distance from Grenada than L. rufaxilla, of which the Grenada Dove was previously thought to be a subspecies. We reveal a genetic difference (P = 0.04) and 126 nucleotide polymorphisms between the Grenada Dove and L. rufaxilla. Our findings support the work of Blockstein and Hardy (Reference Blockstein and Hardy1989) whose morphological analysis (showing differing morphological traits such as cinnamon underwing on primary feathers, a greater extent of white abdomen and a lesser extent of white on the tail tips) and sonographic data (no response was made by the Grenada Dove to L. rufaxilla playback presentations) led to the reclassification of the Grenada Dove as a distinct taxon. Anecdotal evidence suggested the Grenada Dove is closely related to L. jamaicensis (Anthony Jeremiah, Head of Forest and National Parks Department, Government of Grenada, pers. comm.), however our results show that the Grenada Dove shares common ancestors with four other Leptotila more recently than its shared ancestor with L. jamaicensis and with a greater genetic distance and number of nucleotide polymorphisms in comparison.
The evolutionary history of the Leptotila genus was inferred by Johnson and Weckstein (Reference Johnson and Weckstein2011) using a molecular phylogeny to investigate the role of the Central American land-bridge in avian dispersal-driven diversification events. Their results show that this genus diverged into two clades at a time that coincides with the closure of the Isthmus of Panama forming the Central American land-bridge. Their study suggests that the northern clade dispersed southwards from North America stepwise through Central America, crossing the land-bridge into South America, while the southern clade, containing L. rufaxilla and L. cassinii, dispersed in the opposite direction from South America across the Panama Isthmus (Johnson and Weckstein Reference Johnson and Weckstein2011). Our results indicate that the Grenada Dove is placed within the southern clade and not with the northern clade containing L. jamaicensis. We hypothesise that there was a shared evolutionary history with an upwards dispersal event from South America by the ancestor shared by L. rufaxilla, L. cassinii and the Grenada Dove with a range expansion to Central America and the southern islands of the Lesser Antilles. Dispersal over the barriers presented by the South American Andes and the Caribbean Sea implies reduced gene flow between the ancestral populations and eventual isolation. This is supported by the absence of the indel in the Grenada Dove nDNA, suggesting this mutation occurred after divergence from the shared common ancestor with L. cassinii and L. plumbeiceps. Our data support the hypothesis that allopatric speciation due to isolation of the Grenada Dove and exposure to differing selective pressures resulted in the evolution of Grenada Dove as a distinct species.
Our analyses were able to predict six life history values for the Grenada Dove. Values for clutch size, clutches per year and fledging age agreed with documented values from focal observations (Rusk, unpubl. data). With our model estimations of only two eggs per clutch and 1.4 clutches per year the Grenada Dove has a relatively slow breeding rate, which has been shown to be correlated with extinction risk (Robinson et al. Reference Robinson, Hau, Klasing, Wikelski, Brawn, Austin, Tarwater and Ricklefs2010, Hutchings et al. Reference Hutchings, Myers, García, Lucifora and Kuparinen2013). Given that IUCN generated a 4.2 year generation length and with a low predicted longevity (genus median = 8.6 years), these values suggest this species would be slow to recover after a major population decline (Bird et al. Reference Bird, Martin, Akçakaya, Gilroy, Burfield, Garnett, Symes, Taylor, Şekercioğlu and Butchart2020). As not all traits are phylogenetically conserved (Kamilar and Cooper Reference Kamilar and Cooper2013), we tested for phylogenetic signal and found that signal strength varied amongst traits. Clutch size and generation length both exhibited strong phylogenetic signals, as is expected owing to their correlation with body mass, which indicates phylogenetic conservatism of these life history traits (Kamilar and Cooper Reference Kamilar and Cooper2013, Calhoon et al. Reference Calhoon, Jimenez, Harper, Jurkowitz and Williams2014). All other traits exhibited low phylogenetic signal strength, which is not uncommon in traits such as these that often evolve as a response to differing environmental conditions (Kamilar and Cooper Reference Kamilar and Cooper2013, Martin et al. Reference Martin, Ton and Oteyza2018). Although our model validation has suggested that many of our predicted values are accurate, these results have to be used cautiously, and would benefit from validation from field data (Ando Reference Ando2019, Bolton et al. Reference Bolton, Van Oosterhout, Collar and Bell2016).
When comparing predicted values for life history traits for the Grenada Dove and L. cassinii (the species we reveal shares the most recent common ancestor and the smallest genetic distance) and L. rufaxilla (the species it was once considered a subspecies of) we find that a clutch size of two and more than one clutch per year were shared between all three species. The Grenada Dove and L. cassinii both having a hatching weight of over 3 g and an incubation time of around 14 days whereas L. rufaxilla has a slightly lower hatching weight of around 2 g and a slightly higher incubation time of 15 days. While there is no great difference in life history traits between studied members of the Leptotila genus, as expected, we find that the species with life history traits most similar to the Grenada Dove is L. cassinii which is the species we also reveal has the smallest genetic distance.
The more favourable conservation status of the other Leptotila species examined in this study may be attributed to their much larger ranges and availability of suitable habitat (BirdLife International 2021). We reinforce the need for increasing suitable habitat available to the Grenada Dove to reduce extinction risk, both through habitat restoration and protection, as has been outlined in the recovery and action plan for this species, as well as identification of additional habitat for establishing new populations and enabling population increase (Rusk et al. Reference Rusk, Temple, Wege, Wallace, Blockstein, Ledec and Sorenson2008).
Supplementary Materials
To view supplementary material for this article, please visit http://doi.org/10.1017/S0959270922000065.
Acknowledgements
We would like to thank the Forest and National Parks Department of the Government of Grenada for offering their support and expertise, in particular Chief Forestry Officer Anthony Jeremiah for providing us with the eggshell sample and Forest Ranger Doland Francis for providing us with the feather samples. We thank Dr Nigel Collar for his advice and support with this manuscript, Dr Ian McDowall for his support and guidance with the molecular methods, Dr Alejandro González-Voyer for his advice and support with multivariate phylogenetic comparative methods and the Chester Biological Sciences technical team for their laboratory assistance. This work was funded by the University of Chester. Ethical approval was awarded by the Faculty Research and Ethics Committee at the University of Chester. CITES import (546012/01, 567389/01) and export (Grenada IACUC 14002, 14004) permits were obtained for movement of samples.