Evidence accumulated over the last 40 years has suggested that Si, in the form of soluble dietary silica (orthosilicic acid or Si(OH)4), is important for the health of bone and other connective tissues(Reference Jugdaohsingh1, Reference Nielsen2). Dietary Si deficiency studies conducted in the early 1970s have reported stunted growth and marked (detrimental) effects on bone and other connective tissues(Reference Carlisle3, Reference Schwarz and Milne4), suggesting that Si is essential for the normal growth and development of bone and connective tissues. However, recent studies have not been able to reproduce these dramatic effects, although moderately beneficial effects on skeletal health have been observed(Reference Jugdaohsingh1, Reference Jugdaohsingh, Calomme and Robinson5). Recent epidemiological and Si supplementation studies have also suggested that dietary Si may play an important role in bone and connective tissue health(Reference Jugdaohsingh, Tucker and Qiao6–Reference Spector, Calomme and Anderson11).
Despite the accumulating evidence, the exact role and metabolism of Si are not known(Reference Charnot and Pérès12). In addition, there is no evidence for a Si deficiency state for Si-responsive conditions in human subjects(Reference Solomons, Solomons and Rosenberg13). Thus, whether Si indeed has a biological role is questionable. However, a prior study may have provided the first evidence of Si regulation in human subjects. Van Dyck et al. (Reference Van Dyck, Robberecht and Van Cauwenbergh14) reported marked differences in circulating serum Si concentrations between pregnant women (38 (sd 10·3) μg/l; n 17) and age-matched non-pregnant female controls (126 (sd 73) μg/l; n 34) and between infants aged less than 1 year (516 (sd 258) μg/l; n 11) and adults (131 (sd 64) μg/l; n 128). These data imply a diversion of Si from the mother to the growing fetus, possibly for skeletal and connective tissue development, and thus could provide the first evidence of the essentiality of Si in human subjects. However, there are caveats that need to be considered while comparing circulating Si concentrations between such groups.
First, following a meal, Si levels can be raised in the serum and urine for up to 6 h(Reference Jugdaohsingh, Anderson and Tucker15). Hence, sera should be collected following an overnight fast and, preferably, at the same time of the day. Second, as with a number of trace elements, sample preparation and analysis are issues for Si, although the problems are not straightforward. Typically, in the Western world, adult fasting serum Si concentrations are about 100 μg/l, which is greater than that of a number of trace elements, and thus contamination is normally not a problem if appropriate trace-metal-free syringes and containers are used, even without pre-washing(Reference Jugdaohsingh, Anderson and Tucker15). Moreover, differences in serum Si concentrations following intervention (e.g. dietary intervention) are often relatively large and the baseline sample acts as an ideal control for the batch analysis of change in serum Si concentrations of each subject. So, this is also not generally an issue. However, when comparing baseline Si levels between groups, a different approach is required. Samples need to be prepared and analysed in (single if possible) randomised batch(es) with a pooled sample designated as the reference material that is run regularly between samples. This minimises temporal effects, which may give rise to small differences in absolute levels within (and between) batches. The sample diluent may also pose a problem as a potential source of Si contamination. Since the diluent is present at more than 50 % of the volume of the diluted sera, it can contribute a significant amount of Si in the diluted sample. Thus, it is important to check the Si concentration of the diluent before use. Finally, although time consuming, individual sample-based standards (ISBS) allow for the highest degree of accuracy(Reference Burden, Powell and Taylor16).
Taking the aforementioned aspects into consideration, in the present study, we analysed and compared fasting serum Si concentrations in healthy women (1) in the second trimester of pregnancy and (2) at the time of delivery with those of non-pregnant, non-lactating women (controls). We also compared serum Si concentrations in healthy newborn babies with those of their mothers, facilitated by analysing serum obtained from the umbilical cord vein and artery at delivery.
Materials and methods
Materials
Ultra-high purity (UHP) water (18 mΩ/cm) was obtained from an Elga water purifier (Elga). High-purity nitric acid, 65 % (w/v) HNO3 (puriss p.a. plus), and Si inductively coupled plasma (ICP)/direct coupled plasma (DCP) standard solution (9800 μg Si/ml) were obtained from Fluka Chemicals and Aldrich Chemical Company, respectively. Silicone-free S-Monovette® syringes and needles were procured from Sarstedt Limited. Polypropylene tubes were obtained from Medfor Products. These were pre-cleaned before use by rinsing in UHP water and dried under clean-air conditions. Transfer pipettes (polypropylene) were obtained from Greiner Bio-One Limited. A class J clean-air room and class C laminar clean-air workstation were utilised in the present study to avoid potential contamination of the samples with Si. The ICP optical emission spectrometer (Jobin-Yvon JY24; Instruments SA), class C laminar workstation and UHP water supply were stored in a clean-air room.
Subjects
Subjects were recruited from the Department of Obstetrics, King's College Hospital (London, UK). Pregnant women (n 14, median age 34 years; range 25–40 years) in the second trimester of pregnancy undergoing routine obstetric ultrasound examination at 15–24 weeks of gestation and women (n 14) who had uncomplicated deliveries at term were invited to participate in the study. Non-pregnant, non-lactating, departmental female staff (n 17, median age 32 years; range 20–41 years) provided control fasting sera, and although these women were not age matched to the pregnant women, they were of child-bearing age and at a similar stage in life. Serum was also collected from the umbilical cord artery (n 5) and vein (n 14) at the time of delivery. The subjects were fasted for at least 6 h before venepuncture, and samples were collected from the control and pregnant women at the same time of the day. The study was conducted according to the guidelines laid down in the Declaration of Helsinki, and ethical approval was obtained from the Local Research Ethics Committee of King's College Hospital. All participants provided written informed consent before the commencement of the study.
Blood collection and preparation
Blood (10 ml) was collected from a forearm vein from pregnant women in their second trimester and at the time of delivery and from non-pregnant controls. For samples of newborn babies, blood was collected from the umbilical cord vein and, if possible, from the umbilical artery immediately after birth. Silicone-free, rubber-free S-Monovette® syringes and needles were used for blood collection to avoid potential contamination with Si. Blood samples were transferred into pre-cleaned polypropylene tubes and allowed to clot at room temperature. The clotted samples were then centrifuged at 2000 rpm for 10 min at 4°C, and the separated serum was removed with a polypropylene transfer pipette and transferred into a pre-cleaned polypropylene tube. The samples were stored at − 70°C until analysis. Upon analysis, the samples were thawed to room temperature, briefly vortexed to mix and 1 ml was transferred into a clean pre-weighed polypropylene tube. The samples were then diluted with 3–4 ml of 0·25 or 0·7 % (w/v) HNO3 (see Table 1) prepared fresh from high-purity nitric acid and UHP water. For the preparation of pooled sample-based standards (PSBS), 1 ml of each of the diluted serum sample was pooled into a cleaned polypropylene container.
Table 1 Summary of the samples, dilution and analysis
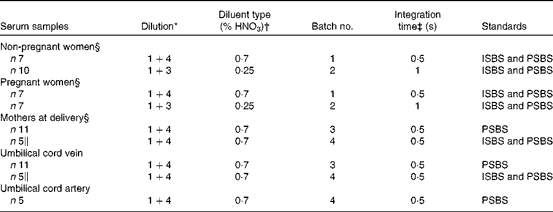
ISBS, individual sample-based standards; PSBS, pooled sample-based standards.
* Sample+diluent (i.e. 1 ml sample+3 or 4 ml diluent).
† Diluent was prepared by diluting high-purity nitric acid in ultra-high purity water (%, v/v).
‡ Integration times at each of the fifty-four increments per profile as measured by the inductively coupled plasma optical emission spectrometer.
§ Venous samples were obtained from a forearm vein.
∥ Two samples were repeated (reanalysed) from batch no. 3.
Total silicon analysis
The diluted serum samples and the sample diluents were analysed for total Si at 251·611 nm using the ICP optical emission spectrometer (Jobin-Yvon JY24; Instruments SA), fitted with a v-groove nebuliser and a conventional Scott-type double-pass spray chamber. A sample flow rate of 1 ml/min was used, as described previously(Reference Jugdaohsingh, Anderson and Tucker15). For greater accuracy and sensitivity(Reference Burden, Powell and Taylor16), peak profiles rather than the conventional on-peak measurements were used, with a window size of 0·1 nm (0·05 nm either side of the peak) and fifty-four increments per profile. The integration time was either 0·5 or 1 s per increment (Table 1).
The diluted serum samples were analysed using PSBS, and in some cases, to examine the issue of PSBS v. ISBS, we analysed some of the samples using both PSBS and ISBS. PSBS were prepared by spiking aliquots of the pooled diluted serum sample (see above) with 0–100 μg Si/l from a Si ICP/DCP standard solution. ISBS were prepared by spiking aliquots of the individual diluted serum sample with 100 μg Si/l from the same Si ICP/DCP standard solution.
The sample diluents (0·25 and 0·7 % HNO3) were similarly analysed with appropriate standards, prepared by spiking the diluents with Si from the ICP/DCP standard solution. Mean Si concentrations of the sample diluents were 49·1 (sd 5·5) μg/l for 0·7 % HNO3 and 10·3 (sd 2·6) μg/l for 0·25 % HNO3, illustrating the (subtle, non-linear) variation in Si levels that can occur between batches of diluents. Thus, the limits of quantification for samples were restricted not by the limits of detection of the instrument, but by background (diluent) Si levels. Hence, the limits of quantification for a diluted sample, set as mean+3 sd of background, were 66 μg/l with the 0·7 % HNO3 diluent and 18 μg/l with the 0·25 % HNO3 diluent. To calculate Si levels in the samples, four-fifths, for 1+4 serum dilution, and three-fourths, for 1+3 serum dilution, of the concentration of Si in the diluents were subtracted from the diluted serum sample results. Table 1 summarises the different batches and approaches used for the analysis.
Statistical analysis
Results are expressed as means and standard deviations and where appropriate medians and ranges are also presented. Grubbs' test for outliers was carried out on all datasets collected (i.e. from pregnant women, non-pregnant controls, maternal vein samples, newborn umbilical vein samples and newborn umbilical artery samples) to identify values that were potential outliers in each dataset. Agreement between serum Si values obtained using PSBS and ISBS was assessed on the log scale using the Bland–Altman approach. Pearson's correlation was also performed to correlate serum Si levels obtained using PSBS and ISBS. For comparison of Si concentrations between pregnant and non-pregnant women and between maternal vein and fetal vein and artery samples, t tests were performed on the log Si values and the differences quantified and 95 % CI reported. All statistical analyses were performed in SPSS version 11.0 (SPSS, Inc.). Differences with a P value of less than 0·05 were considered significant, and to correct for multiplicity of testing, a Bonferroni correction was applied to the P values.
Results
Pooled v. individual sample-based standards
Si concentrations in fifty-four serum samples (including five maternal forearm vein and five umbilical cord vein samples) were analysed using PSBS and ISBS. There was a close agreement between the values (r 0·95, P< 0·0001; Fig. 1). However, on average, the values obtained using PSBS were 13 (95 % CI 9, 18) % greater than those obtained using ISBS.
Fig. 1 Correlation between fasting silicon concentrations in serum samples (n 54) calculated using pooled sample-based standards and individual sample-based standards. r 0·946; P< 0·0001; y= 1·072x+5·202. The dotted line indicates the y= x line.
Pregnant and non-pregnant women
Serum Si concentrations were 110 (sd 38) μg/l (median 98 μg/l; range 69–194 μg/l) in the pregnant women (n 14, median gestational age: 22 weeks and 6 d) v. 105 (sd 38) μg/l (median 97 μg/l; range 43–177 μg/l) in the non-pregnant, non-lactating women (n 17) using ISBS. Using PSBS, the values were, respectively, 117 (sd 30) μg/l (median 108 μg/l; range 84–177 μg/l) v. 115 (sd 38) μg/l (median 112 μg/l; range 50–190 μg/l). Thus, serum Si concentrations in the pregnant women did not differ (mean 6 (95 % CI − 22, 27) %) from those in the non-pregnant, non-lactating women. The correlation between serum Si concentrations and pregnancy stage (weeks) was not significant (r 0·19, P= 0·51; n 14).
Mothers and newborn babies
Serum Si concentrations in the maternal forearm vein at delivery and in the corresponding umbilical cord vein and artery, calculated using PSBS, are shown in Fig. 2. Serum values were not different when analysed using ISBS in a subset of samples (n 10) compared with PSBS (data not shown). Serum Si concentrations in the umbilical cord vein samples (169 (sd 192) μg/l (n 14); median 110·2 μg/l, range 10·4–753 μg/l) were, on average, 52 % higher (95 % CI − 7, 148 %) than those in the maternal forearm vein samples (89 (sd 73) μg/l (n 14); median 70·8 μg/l, range 0·74–255 μg/l), but these were not significant. Fetal artery serum Si concentrations (218 (sd 143) μg/l (n 5); median 152 μg/l, range 66·6–399 μg/l) were 235 % higher (95 % CI − 37, 1673 %) than mean maternal vein levels and higher (mean 150 %, 95 % CI − 65, 1681 %) than mean fetal vein levels, but these were not significant, with the wide CI reflecting the small number of samples obtained from the fetal artery. Individually, however, eight of the fourteen umbilical cord vein samples and all (n 5) of the umbilical cord artery samples had higher Si concentrations than their corresponding maternal forearm vein samples (Fig. 3).
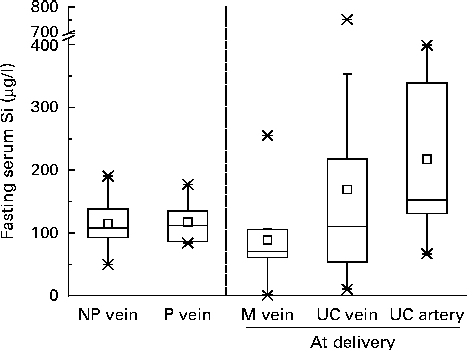
Fig. 2 Fasting serum Si concentrations in the forearm vein of non-pregnant women (NP vein, n 17), pregnant women (P vein, n 14) and full-term mothers at delivery (M vein, n 14) and in the umbilical cord (UC) vein (n 14) and UC artery (n 5) of their newborns. Data are shown as box plots where the horizontal lines indicate the 5th, 25th, 50th (or median), 75th and 95th percentiles; □ indicates the mean and × indicates the minimum and maximum concentrations. Serum Si concentrations were calculated using pooled sample-based standards.

Fig. 3 Paired analysis of serum Si concentrations in the forearm vein of full-term mothers (M vein, n 14) at the time of delivery and in the corresponding umbilical cord (UC) vein (n 14) and UC artery (n 5). Data points shown at < 56 μg/l are not absolute values but simply designate below the limits of quantification (LOQ).
Comparison was also made between serum Si concentrations in the maternal forearm vein samples and those in the pregnant and non-pregnant women. Mean serum Si concentrations in the maternal forearm vein at delivery appeared to be lower (approximately 50 %) than those in the pregnant and non-pregnant women (Fig. 2), but these were not significant.
Discussion
The present study primarily investigated the effect of pregnancy on serum Si concentrations, by comparing fasting serum Si levels between pregnant and non-pregnant women. It also compared serum Si concentrations in the umbilical cord blood of newborns with those of their mothers at delivery. However, in contrast to the previous findings of Van Dyck et al. (Reference Van Dyck, Robberecht and Van Cauwenbergh14), who reported a marked decrease in serum Si concentrations in pregnant women compared with non-pregnant controls, we found no significant effect of pregnancy on fasting serum Si concentrations. Additionally, within the pregnant group, we found little change in serum Si concentrations with advancing gestation (from 15 to 24 weeks). However, we did find that mean serum Si concentrations in the full-term mothers at delivery appeared, on average, to be approximately 50 % lower than those in both pregnant and non-pregnant/non-lactating women; however, these did not approach significance (P≥ 0·8), and there was a large variation in serum Si concentrations in the mothers (see below). Moreover, we also noted that this was a post hoc analysis and samples were analysed in a batch/run different from that of the pregnant and non-pregnant/non-lactating samples. Mean fasting serum Si concentrations in the newborns (umbilical vein and artery) were higher than those in the maternal forearm vein at delivery, but, again, these were not significant (see below).
Our findings, therefore, contradict the previous findings, which reported a marked significant difference (by 332 %) in circulating serum Si concentrations between pregnant women (38 (sd 10·3) μg/l; n 28) and matched non-pregnant women (126 (sd 73) μg/l; n 34)(Reference Van Dyck, Robberecht and Van Cauwenbergh14). Reasons for the differences between the two studies are unclear. In both studies, serum Si concentrations in the non-pregnant female controls were the same (114 (sd 38) μg/l in the present study v. 126 (sd 73) μg/l in the previous one). There were twice as many subjects in the previous study, but this is unlikely to have significantly affected the results; that is, the present results should not change dramatically if additional samples were collected to make up the difference in numbers between the two studies. The mean gestational age of the pregnant women in the present study was 22 weeks and 6 d, which is less than that of those in the previous study (mean gestational age 29 weeks and 6 d(Reference Van Dyck, Robberecht and Van Cauwenbergh14)). It is possible that this could explain the discrepancy; that is, lower serum Si concentrations are found in a more advanced pregnancy state. However, pre-delivery, we found no correlation between serum Si concentrations and gestational age or a trend towards change in serum Si concentrations with gestation. Additionally, even for the full-term mothers, the apparent mean decrease in serum Si concentrations in the present study was only approximately 50 % compared with that for the non-pregnant women and not the 332 % difference reported previously between pregnant and non-pregnant women(Reference Van Dyck, Robberecht and Van Cauwenbergh14).
Our findings appear to suggest that serum Si concentrations in blood from the umbilical cord vein and artery of newborns, on average, are higher (by 52 and 235 %, respectively) than those of their mothers, although the results were not significant, perhaps due to the large variation in serum Si concentrations in both sample sets. In particular, serum Si concentrations in three of the maternal samples and four of the newborn umbilical vein samples were much lower than the mean concentrations and below the limits of quantification, while serum Si concentration in one of the umbilical cord vein samples was markedly higher than the mean concentration. Grubbs' test for outliers only identified the latter, i.e. the higher Si value in the umbilical cord vein sample, to be a significant outlier (at P< 0·05 and P< 0·01). Removal of this sample and its corresponding maternal vein sample reduced the difference in mean Si concentrations between the umbilical vein and maternal samples to 36 % (or 124 (sd 97) and 91 (sd 76) μg/l, respectively).
We are unable to explain the large variation in Si concentrations in the maternal and umbilical cord samples. As noted above, we were careful while collecting, preparing and analysing the blood samples and thus believe that the values obtained are a true representation of in vivo variability in these sample types. Under these conditions, it seems most unlikely that the fasting serum Si levels could reflect body stores of Si, which is suggested to be the case outside of pregnancy(Reference Charnot and Pérès12, 17). However, it is well recognised that the turnover of connective tissue components differs greatly in pregnancy v. the non-pregnant state(Reference Stewart, Floor and Jain18–Reference Hellmeyer, Ziller and Anderer20).
Unlike in the previous study, blood was taken from the umbilical cord and not directly from the newborns. This was done for ethical reasons and to minimise contamination. We found that Si concentrations were lower than those reported previously: i.e. mean of 169 μg/l (range 10·36–753 μg/l; n 14) in our term infants v. 516 μg/l (range 343–689 μg/l; n 11) for infants aged less than 1 year in the previous study(Reference Van Dyck, Robberecht and Van Cauwenbergh14). Reasons for the differences between the two studies could be fourfold. First, since we used umbilical cord blood, a larger volume of blood was available in the present study and contamination during collection and preparation is likely to have been reduced. Second, all samples were taken at the time of delivery, compared with < 1 year after delivery in the previous study(Reference Van Dyck, Robberecht and Van Cauwenbergh14), which would negate the effect of dietary Si exposure. In the previous study, although subjects were fasted overnight, it is unlikely that newborn babies were. Milk is generally low in Si(Reference Pennington21), but infant formula contains Si additives to maintain a longer shelf life(Reference Jugdaohsingh, Tucker and Qiao6, Reference Pennington21, Reference Giammarioli, Mosca and Sanzini22). Third, in the present study, mothers and babies were matched, unlike in the previous study(Reference Van Dyck, Robberecht and Van Cauwenbergh14). Fourth, in the previous study, samples were collected from subjects, including infants, who were hospitalised between 1998 and 1999 in Antwerp (Belgium)(Reference Van Dyck, Robberecht and Van Cauwenbergh14). They were described as ‘apparently healthy’, but no reasons were given for hospitalisation.
Although we collected only a few samples from the umbilical cord artery, Si concentrations appeared to be higher than maternal vein and umbilical cord vein concentrations, albeit not significantly so. The umbilical cord artery carries deoxygenated blood from the fetus to the placenta and thus may be a true representation of the circulating Si levels in the fetus/newborn. It could be argued that if Si were essential for growth and development in the newborn, levels in the umbilical cord artery should be lower than those in the umbilical cord vein or maternal vein (i.e. Si having been utilised by the fetus). The present results, however, indicate the opposite. However, it is important to note that the umbilical arteries surround the urinary bladder and then carry all the deoxygenated blood from the fetus through the umbilical cord, and therefore it is possible that the high serum Si concentrations are due to the excretion of Si from the urinary bladder into the umbilical arteries. Thus, there is still the possibility that Si is acquired by the fetus from the mother but without incorporation/utilisation when fetal Si status is sufficient.
Finally, analysis of some samples in the present study was initially hampered by the high concentration of Si in the diluent (0·7 % nitric acid). Since the diluent makes up to four-fifths of the volume of the diluted sera, it can be responsible for a significant concentration of Si in the diluted sample. Thus, it is important to check the Si concentration of the diluent before use. The high concentration of Si in the diluent in our initial analysis was probably due to the leaching of low levels of Si from the UHP water system. Si and B are the first elements to be released (i.e. not retained) in the UHP system and may even precede a drop in electrical resistivity (water purity measurement). For this reason, the Si concentration of UHP water is measured regularly in our laboratory, but, despite this, levels can rise between analyses. This emphasises that an understanding of the potential analytical pitfalls is needed for the accurate analysis of Si.
In conclusion, at best, the results of the present study are only in partial agreement with the previous findings(Reference Van Dyck, Robberecht and Van Cauwenbergh14); that is, newborns/infants may have higher serum Si concentrations than adults, but further work would be required to prove this. It is unclear why levels in pregnant women differed between the two studies, but it is likely that it is due to methodological differences(Reference Van Dyck, Robberecht and Van Cauwenbergh14). Overall, we provide some evidence, in terms of higher average serum Si concentrations in the umbilical cord artery and vein of newborns compared with serum Si concentrations in the maternal forearm vein, to support the prior study(Reference Van Dyck, Robberecht and Van Cauwenbergh14) and that could imply a higher requirement of Si in newborns for bone and connective tissue development. However, in the present study, these findings were not significant, so further work would be needed to prove this, with larger sample numbers and careful sampling and analytical protocols. We would also suggest preparation of a single, large container of ultra-clean diluent for sample dilution in any future study involving measurement of baseline serum levels. Furthermore, contamination, sample size, temporal analytical and physiological effects, and analytical batch effects should all be keenly considered in future work.
Acknowledgements
Some of the data reported here was presented in conference proceedings of the 10th International Symposium on Metal Ions in Biology and Medicine, Bastia, France, 19–22 May 2008(Reference Ratcliffe, Jugdaohsingh and Anderson23) and as a poster at ‘Silicon by the Sea’, 3rd Workshop on the Aqueous Chemistry and Biochemistry of Silicon, San Diego, CA, USA, 9–11 December 2009.
S. S. was sponsored by a studentship from the Thai government. We thank Mark Chatfield (MRC HNR) for his statistical advice. The Charitable Foundation of the Institute of Brewing and Distilling provided running costs for some of the Si research programme (R. J. and J. J. P.). We thank Professor Sir Richard P. H. Thompson for his advice and consultation. The present study was supported by the Medical Research Council (grant no. MC_US_a090_0008/unit programme no. u1059). The authors' contributions are as follows: S. H. C. A., R. J., L. L. and J. J. P. were responsible for the concept and design of the study; S. H. C. A., R. J., L. L., S. S. and J. J. P. were responsible for the experimental and analytical aspects of the manuscript; S. H. C. A. was the study coordinator; S. H. C. A., R. J., S. R., L. L. and J. J. P. were responsible for the data interpretation, presentation and writing of the first draft of the manuscript. All authors contributed to the writing of the final manuscript. The authors have no conflict of interest.