Introduction
Physiological changes that occur during ageing can contribute to a progressive decline in overall brain health including cognitive abilities. This impairment in brain health is observable in multiple age-related diseases, including mild cognitive impairment (MCI), Alzheimer’s disease (AD), Parkinson’s disease (PD) and multiple sclerosis (MS), and leads to a worsened quality of life for patients. There is an important difference between one’s chronologic age (time since birth) versus one’s biologic age, and differences in the rate of ageing, especially during midlife, can significantly impact future age-related decline (Elliott et al., Reference Elliott, Caspi, Houts, Ambler, Broadbent, Hancox, Harrington, Hogan, Keenan, Knodt, Leung, Melzer, Purdy, Ramrakha, Richmond-Rakerd, Righarts, Sugden, Thomson, Thorne, Williams, Wilson, Hariri, Poulton and Moffitt2021). Furthermore, biologic age in healthy elderly individuals can predict the development of dementia (Wu et al., Reference Wu, Yaqub, Ma, Koudstaal, Hofman, Ikram, Ghanbari and Goudsmit2021a). Recent studies suggest that peripheral changes in physiology may affect age-related cognitive decline and therefore represent an emerging therapeutic target to manage brain health in ageing. Moreover, gastrointestinal (GI) functions are disrupted during ageing, including weakened gut barrier function, altered intestinal neurotransmitter levels and altered intestinal immunity (Bosco and Noti, Reference Bosco and Noti2021; Saffrey, Reference Saffrey2014). Changes in GI physiology culminate in alterations to the gut microbiota, which may in turn influence brain ageing.
Trillions of microorganisms reside in the GI tract and constantly survey, adapt and respond to their environment. Collectively known as the gut microbiota, these microbes have coexisted and coevolved with their host and are increasingly recognised for their contributions to maintaining the health of their host throughout the lifespan, including brain health. The gut microbiota can communicate bidirectionally with the brain through several mechanisms, including endocrinal, nervous, immune and microbial metabolite-driven pathways (Cryan et al., Reference Cryan, O’Riordan, Cowan, Sandhu, Bastiaanssen, Boehme, Codagnone, Cussotto, Fulling, Golubeva, Guzzetta, Jaggar, Long-Smith, Lyte, Martin, Molinero-Perez, Moloney, Morelli, Morillas, O’Connor, Cruz-Pereira, Peterson, Rea, Ritz, Sherwin, Spichak, Teichman, van de Wouw, Ventura-Silva, Wallace-Fitzsimons, Hyland, Clarke and Dinan2019b). Investigations into these pathways, along with the use of germ-free (GF) rodents, which completely lack all microbes, and direct gut microbiota manipulation, such as antibiotic treatment, faecal microbiota transplantation and microbiota administration, have enabled a deeper understanding of how the gut microbiota influences biological functions in its host, including in the brain. Critically, the gut microbiota influences the blood–brain barrier (Braniste et al., Reference Braniste, Al-Asmakh, Kowal, Anuar, Abbaspour, Toth, Korecka, Bakocevic, Ng, Kundu, Gulyas, Halldin, Hultenby, Nilsson, Hebert, Volpe, Diamond and Pettersson2014), neurochemistry and cellular functions in the brain, such as immunity and neuroplasticity (Erny et al., Reference Erny, Hrabe de Angelis, Jaitin, Wieghofer, Staszewski, David, Keren-Shaul, Mahlakoiv, Jakobshagen, Buch, Schwierzeck, Utermohlen, Chun, Garrett, McCoy, Diefenbach, Staeheli, Stecher, Amit and Prinz2015; Guzzetta et al., Reference Guzzetta, Cryan and O’Leary2022; Mossad and Blank, Reference Mossad and Blank2021; Ogbonnaya et al., Reference Ogbonnaya, Clarke, Shanahan, Dinan, Cryan and O’Leary2015; Parker et al., Reference Parker, Romano, Ansorge, Aboelnour, Le Gall, Savva, Pontifex, Telatin, Baker, Jones, Vauzour, Rudder, Blackshaw, Jeffery and Carding2022; Rei et al., Reference Rei, Saha, Haddad, Haider Rubio, Perlaza, Berard, Ungeheuer, Sokol and Lledo2022; Scott et al., Reference Scott, Ida, Peterson, Prenderville, Moloney, Izumo, Murphy, Murphy, Ross, Stanton, Dinan and Cryan2017; Spichak and Guzzetta, Reference Spychala, Venna, Jandzinski, Doran, Durgan, Ganesh, Ajami, Putluri, Graf, Bryan and McCullough2018) and has been preclinically shown to modulate cognitive function during ageing (Boehme, Guzzetta and Bastiaanssen et al., Reference Boehme, Guzzetta, Bastiaanssen, van de Wouw, Moloney, Gual-Grau, Spichak, Olavarría-Ramírez, Fitzgerald, Morillas, Ritz, Jaggar, Cowan, Crispie, Donoso, Halitzki, Neto, Sichetti, Golubeva, Fitzgerald, Claesson, Cotter, O’Leary, Dinan and Cryan2021; Mossad et al., Reference Mossad, Nent, Woltemate, Folschweiller, Buescher, Schnepf, Erny, Staeheli, Bartos, Szalay, Stecher, Vital, Sauer, Lämmermann, Prinz and Blank2021), and neurodegeneration (Erny et al., Reference Erny, Dokalis, Mezo, Castoldi, Mossad, Staszewski, Frosch, Villa, Fuchs, Mayer, Neuber, Sosat, Tholen, Schilling, Vlachos, Blank, Gomez de Aguero, Macpherson, Pearce and Prinz2021). Taken together, the gut microbiota plays a crucial role in supporting healthy cognition and neurological functions of its host, including active participation in important aspects of brain ageing.
While the composition of the gut microbiota is generally understood to be relatively stable during early- and mid-adulthood, community stability appears to be uprooted in later stages of ageing, although the effects of ageing on gut microbiome diversity appear mixed, perhaps due to factors such as health and diet. For instance, elderly patients in care homes displayed an overall reduction in faecal microbial diversity, which also associated to worsened health status of the individual, while centenarians living amongst the general population had distinguishably increased alpha diversity, due to higher relative abundance of subdominant taxa accompanied with reduced relative abundances of core taxa (Biagi et al., Reference Biagi, Franceschi, Rampelli, Severgnini, Ostan, Turroni, Consolandi, Quercia, Scurti, Monti, Capri, Brigidi and Candela2016, Reference Biagi, Rampelli, Turroni, Quercia, Candela and Brigidi2017; Claesson et al., Reference Claesson, Jeffery, Conde, Power, O’Connor, Cusack, Harris, Coakley, Lakshminarayanan, O’Sullivan, Fitzgerald, Deane, O’Connor, Harnedy, O’Connor, O’Mahony, van Sinderen, Wallace, Brennan, Stanton, Marchesi, Fitzgerald, Shanahan, Hill, Ross and O’Toole2012; Fanli Kong et al., Reference Kong, Hua, Zeng, Ning, Li and Zhao2016; Wu et al., Reference Wu, Zeng, Zinellu, Rubino, Kelvin and Carru2019). These conflicting results suggest that ageing leads to divergences in the gut microbiome which are dependent on the community sampled, and which likely contribute to an increased microbiome uniqueness overserved in ageing (Wilmanski et al., Reference Wilmanski, Diener, Rappaport, Patwardhan, Wiedrick, Lapidus, Earls, Zimmer, Glusman, Robinson, Yurkovich, Kado, Cauley, Zmuda, Lane, Magis, Lovejoy, Hood, Gibbons, Orwoll and Price2021). Understanding the causal relationships between gut microbiome trajectory and healthy brain ageing may allow for the discovery of novel microbiota-driven therapies for maintaining brain health during ageing.
Biological ageing drives changes to the gut microbiome
The gut microbiome during ageing
Following birth, the composition of the gut microbiome is highly dynamic, and gradually increases in stability with the development of the adaptive immune system, weaning onto a solid food diet and standardisation of lifestyle factors in early adulthood (Koenig et al., Reference Koenig, Spor, Scalfone, Fricker, Stombaugh, Knight, Angenent and Ley2011). While the composition of the gut microbiome is relatively stable during early- to mid-adulthood if unperturbed, as an individual grows older, the gut microbiota enters a period of increased volatility and distinct shifts occur in the diversity of genera and functional capacity (Badal et al., Reference Badal, Vaccariello, Murray, Yu, Knight, Jeste and Nguyen2020; Claesson et al., Reference Claesson, Cusack, O’Sullivan, Greene-Diniz, de Weerd, Flannery, Marchesi, Falush, Dinan, Fitzgerald, Stanton, van Sinderen, O’Connor, Harnedy, O’Connor, Henry, O’Mahony, Fitzgerald, Shanahan, Twomey, Hill, Ross and O’Toole2011). Ageing-associated alterations to microbial diversity and composition of the gut microbiome have been observed in multiple species, including flies (eg., Drosophila), worms (eg., Caenorhabditis elegans), rodents, dogs, monkeys (Ambrosini et al., Reference Ambrosini, Borcherding, Kanthasamy, Kim, Willette, Jergens, Allenspach and Mochel2019; Dinić et al., Reference Dinić, Herholz, Kačarević, Radojević, Novović, Đokić, Trifunović and Golić2021; Kubinyi et al., Reference Kubinyi, Bel Rhali, Sandor, Szabo and Felfoldi2020; Lee et al., Reference Lee, Lee, Lee, Lee and Min2019; Pallikkuth et al., Reference Pallikkuth, Mendez, Russell, Sirupangi, Kvistad, Pahwa, Villinger, Banerjee and Pahwa2021; Scott et al., Reference Scott, Ida, Peterson, Prenderville, Moloney, Izumo, Murphy, Murphy, Ross, Stanton, Dinan and Cryan2017; van der Lugt et al., Reference van der Lugt, Rusli, Lute, Lamprakis, Salazar, Boekschoten, Hooiveld, Muller, Vervoort, Kersten, Belzer, Kok and Steegenga2018; Wei et al., Reference Wei, Rao, Tang, Zhao, Li, Wu, Liu, Li, Xiao, Liu and Chen2021) and in humans (Biagi et al., Reference Biagi, Nylund, Candela, Ostan, Bucci, Pini, Nikkila, Monti, Satokari, Franceschi, Brigidi and De Vos2010; Claesson et al., Reference Claesson, Jeffery, Conde, Power, O’Connor, Cusack, Harris, Coakley, Lakshminarayanan, O’Sullivan, Fitzgerald, Deane, O’Connor, Harnedy, O’Connor, O’Mahony, van Sinderen, Wallace, Brennan, Stanton, Marchesi, Fitzgerald, Shanahan, Hill, Ross and O’Toole2012; Jeffery et al., Reference Jeffery, Lynch and O’Toole2016; Leite et al., Reference Leite, Pimentel, Barlow, Chang, Hosseini, Wang, Parodi, Sedighi, Rezaie and Mathur2021). This may be due to a multitude of biological and environmental factors, including immunosenescence (Bosco and Noti, Reference Bosco and Noti2021), altered physiology of the gastrointestinal tract (Soenen et al., Reference Soenen, Rayner, Jones and Horowitz2016), development of age-related diseases, and in humans, increased exposure to medication and altered diet associated with long-term care facilities (Claesson et al., Reference Claesson, Jeffery, Conde, Power, O’Connor, Cusack, Harris, Coakley, Lakshminarayanan, O’Sullivan, Fitzgerald, Deane, O’Connor, Harnedy, O’Connor, O’Mahony, van Sinderen, Wallace, Brennan, Stanton, Marchesi, Fitzgerald, Shanahan, Hill, Ross and O’Toole2012).
In humans, characteristics of ageing within the gut microbiome begin to appear in mid-to-late adulthood (Wilmanski et al., Reference Wilmanski, Diener, Rappaport, Patwardhan, Wiedrick, Lapidus, Earls, Zimmer, Glusman, Robinson, Yurkovich, Kado, Cauley, Zmuda, Lane, Magis, Lovejoy, Hood, Gibbons, Orwoll and Price2021), though the exact timepoint for this shift may vary based on genetic, environmental and lifestyle factors which are unique to an individual. In general, ageing is associated with a decline in core microbial genera, increased bacterial community uniqueness, altered microbial diversity and altered functional ability of the gut microbiota (Claesson et al., Reference Claesson, Cusack, O’Sullivan, Greene-Diniz, de Weerd, Flannery, Marchesi, Falush, Dinan, Fitzgerald, Stanton, van Sinderen, O’Connor, Harnedy, O’Connor, Henry, O’Mahony, Fitzgerald, Shanahan, Twomey, Hill, Ross and O’Toole2011, Reference Claesson, Jeffery, Conde, Power, O’Connor, Cusack, Harris, Coakley, Lakshminarayanan, O’Sullivan, Fitzgerald, Deane, O’Connor, Harnedy, O’Connor, O’Mahony, van Sinderen, Wallace, Brennan, Stanton, Marchesi, Fitzgerald, Shanahan, Hill, Ross and O’Toole2012; Ghosh et al., Reference Ghosh, Shanahan and O’Toole2022a,Reference Ghosh, Shanahan and O’Tooleb; Wilmanski et al., Reference Wilmanski, Diener, Rappaport, Patwardhan, Wiedrick, Lapidus, Earls, Zimmer, Glusman, Robinson, Yurkovich, Kado, Cauley, Zmuda, Lane, Magis, Lovejoy, Hood, Gibbons, Orwoll and Price2021). Critically, there is noticeable variation between studies examining the gut microbiome within different older populations, which may be due to dietary, cultural, environmental or geographical differences that can influence the gut microbiome, or due to differences in microbiome analysis methodology (Biagi et al., Reference Biagi, Nylund, Candela, Ostan, Bucci, Pini, Nikkila, Monti, Satokari, Franceschi, Brigidi and De Vos2010, Reference Biagi, Rampelli, Turroni, Quercia, Candela and Brigidi2017; Claesson et al., Reference Claesson, Jeffery, Conde, Power, O’Connor, Cusack, Harris, Coakley, Lakshminarayanan, O’Sullivan, Fitzgerald, Deane, O’Connor, Harnedy, O’Connor, O’Mahony, van Sinderen, Wallace, Brennan, Stanton, Marchesi, Fitzgerald, Shanahan, Hill, Ross and O’Toole2012; Odamaki et al., Reference Odamaki, Kato, Sugahara, Hashikura, Takahashi, Xiao, Abe and Osawa2016; Salazar et al., Reference Salazar, Valdes-Varela, Gonzalez, Gueimonde and de Los Reyes-Gavilan2017; Wilmanski et al., Reference Wilmanski, Diener, Rappaport, Patwardhan, Wiedrick, Lapidus, Earls, Zimmer, Glusman, Robinson, Yurkovich, Kado, Cauley, Zmuda, Lane, Magis, Lovejoy, Hood, Gibbons, Orwoll and Price2021). For instance, the genus Bacteroides and other genera within the family Bacteroidales including Alistipes and Parabacteroides have been observed at higher levels in people aged 65+ compared to younger, healthy adults (Claesson et al., Reference Claesson, Cusack, O’Sullivan, Greene-Diniz, de Weerd, Flannery, Marchesi, Falush, Dinan, Fitzgerald, Stanton, van Sinderen, O’Connor, Harnedy, O’Connor, Henry, O’Mahony, Fitzgerald, Shanahan, Twomey, Hill, Ross and O’Toole2011). However, other studies indicate that ageing leads to a decrease in Bacteroidaceae, the family that contains Bacteroides, along with a reduction in Faecalibacterium and Lachnospiraceae, and an increased abundance of Akkermansia, as recently reviewed by Badal et al. (Reference Badal, Vaccariello, Murray, Yu, Knight, Jeste and Nguyen2020).
These differences in study outcomes suggest that it is currently impossible to pinpoint exactly when a person’s gut microbiome shifts to that of an ‘elderly’ state. Nonetheless, an ageing-associated drift in the gut microbiome was not as prevalent in less healthy individuals, suggesting that the shift in the microbiome occurring during ageing may be beneficial for and predictive of host health (Wilmanski et al., Reference Wilmanski, Diener, Rappaport, Patwardhan, Wiedrick, Lapidus, Earls, Zimmer, Glusman, Robinson, Yurkovich, Kado, Cauley, Zmuda, Lane, Magis, Lovejoy, Hood, Gibbons, Orwoll and Price2021). Furthermore, retention of a higher prevalence of Bacteroides or a low microbial uniqueness was associated with a higher risk of mortality in a 4-year follow up (Wilmanski et al., Reference Wilmanski, Diener, Rappaport, Patwardhan, Wiedrick, Lapidus, Earls, Zimmer, Glusman, Robinson, Yurkovich, Kado, Cauley, Zmuda, Lane, Magis, Lovejoy, Hood, Gibbons, Orwoll and Price2021). This research highlights that the microbiota may act as a novel marker for lifespan in elderly humans with specific bacterial genera potentially playing important roles.
Extreme ageing shows unique microbial signatures
It is important to consider the difference between biologic ageing that leads to physiologic decline versus healthy ageing that supports longevity. While the gut microbiome signatures associated with increased mortality uncovered by Wilmanski et al. (Reference Wilmanski, Diener, Rappaport, Patwardhan, Wiedrick, Lapidus, Earls, Zimmer, Glusman, Robinson, Yurkovich, Kado, Cauley, Zmuda, Lane, Magis, Lovejoy, Hood, Gibbons, Orwoll and Price2021) may be detrimental, there may be beneficial microbes unique or more abundant in healthy, long-living individuals that could support the health of their host (Wilmanski et al., Reference Wilmanski, Diener, Rappaport, Patwardhan, Wiedrick, Lapidus, Earls, Zimmer, Glusman, Robinson, Yurkovich, Kado, Cauley, Zmuda, Lane, Magis, Lovejoy, Hood, Gibbons, Orwoll and Price2021). Indeed, unique microbial signatures appear as an individual ages and are highly apparent in centenarians (individuals who have lived for 100 years or more), though differences in bacterial genera appear to vary depending on the culture and region in which the assessment occurred (Biagi et al., Reference Biagi, Franceschi, Rampelli, Severgnini, Ostan, Turroni, Consolandi, Quercia, Scurti, Monti, Capri, Brigidi and Candela2016; Kim et al., Reference Kim, Choi, Shin, Jin, Bae, Han, Seo, Chun and Chung2019a; Kong et al., Reference Kong, Hua, Zeng, Ning, Li and Zhao2016; Odamaki et al., Reference Odamaki, Kato, Sugahara, Hashikura, Takahashi, Xiao, Abe and Osawa2016; Sato et al., Reference Sato, Atarashi, Plichta, Arai, Sasajima, Kearney, Suda, Takeshita, Sasaki, Okamoto, Skelly, Okamura, Vlamakis, Li, Tanoue, Takei, Nittono, Narushima, Irie, Itoh, Moriya, Sugiura, Suematsu, Moritoki, Shibata, Littman, Fischbach, Uwamino, Inoue, Honda, Hattori, Murai, Xavier, Hirose and Honda2021; Tuikhar et al., Reference Tuikhar, Keisam, Labala, Imrat, Arunkumar, Ahmed, Biagi and Jeyaram2019; Wilmanski et al., Reference Wilmanski, Diener, Rappaport, Patwardhan, Wiedrick, Lapidus, Earls, Zimmer, Glusman, Robinson, Yurkovich, Kado, Cauley, Zmuda, Lane, Magis, Lovejoy, Hood, Gibbons, Orwoll and Price2021). In a well-characterised Italian cohort, the gut microbiome of centenarians had higher abundance of several taxa such as Akkermansia and Christensenellaceae, some of which have previously been associated with health, suggesting they may contribute to the maintenance of health during ageing (Biagi et al., Reference Biagi, Franceschi, Rampelli, Severgnini, Ostan, Turroni, Consolandi, Quercia, Scurti, Monti, Capri, Brigidi and Candela2016). Meanwhile, other research found that Ruminococcaceae, Lachnospiraceae and Bacteroidaceae families decreased with advancing age (Biagi et al., Reference Biagi, Franceschi, Rampelli, Severgnini, Ostan, Turroni, Consolandi, Quercia, Scurti, Monti, Capri, Brigidi and Candela2016; Pu et al., Reference Pu, Lee, Isho, Naouar and Gommerman2021). In another investigation in South Korea, Akkermansia, Collinsella, Clostridium and Christensenellaceae were increased in the faecal microbiome of centenarians, while there was a decreased abundance of Faecalibacterium and Prevotella (Kim et al., Reference Kim, Kwon, Kam, Panicker, Karuppagounder, Lee, Lee, Kim, Kook, Foss, Shen, Lee, Kulkarni, Pasricha, Lee, Pomper, Dawson, Dawson and Ko2019b). This was associated with a greater predicted ability of the gut microbiome to contribute to glycosphingolipid biosynthesis, various N-glycan biosynthesis and the phosphatidylinositol signalling pathway (Kim et al., Reference Kim, Choi, Shin, Jin, Bae, Han, Seo, Chun and Chung2019a). While several studies have reported different findings regarding the composition of the gut microbiome and ageing (Badal et al., Reference Badal, Vaccariello, Murray, Yu, Knight, Jeste and Nguyen2020; Ghosh et al., Reference Ghosh, Shanahan and O’Toole2022a), synergies between clinical studies may highlight potential health-promoting or health-degrading properties of specific bacteria, which could inspire investigations into whether these bacteria may also support cognitive health during extreme ageing (for further reading on this topic, see Ghosh et al., Reference Ghosh, Shanahan and O’Toole2022b).
It is tempting to speculate that longevity-associated bacterial taxa might be involved in the establishment of a longevity-promoting environment within the ageing host, and thus contribute to supporting the extreme limits of ageing within the human life, perhaps by buffering an individual from environmental challenges. Indeed, initial research involving the African turquoise killifish (Nothobranchius furzeri) demonstrated a causal role of the gut microbiota in longevity; transferring microbiota from young fish into middle-aged fish improved their lifespan and motor behaviour and was accompanied by distinct transcriptional changes in intestinal immunity (Smith et al., Reference Smith, Willemsen, Popkes, Metge, Gandiwa, Reichard and Valenzano2017). Furthermore, in a mouse model of progeria, which is a condition of accelerated ageing wherein gut microbiome is also altered, progeroid mice that received the faecal microbiota from wild-type mice showed a noticeable increase in lifespan and health span (Barcena et al., Reference Barcena, Valdes-Mas, Mayoral, Garabaya, Durand, Rodriguez, Fernandez-Garcia, Salazar, Nogacka, Garatachea, Bossut, Aprahamian, Lucia, Kroemer, Freije, Quiros and Lopez-Otin2019). While whole faecal microbiota transplantation (FMT) has shown success in improving lifespan in these models, there is currently no evidence demonstrating whether FMT has a beneficial impact on human lifespan. Moreover, FMT is an intensive clinical procedure. Therefore, the use of individual probiotic bacteria, which can confer health benefits in ageing, may prove to be a better strategy for intervening on the ageing microbiome. Indeed, single strains of bacteria may hold the key for lifespan extension; administration of the bacterium Akkermansia muciniphila alone was sufficient to exert similar effects on extending lifespan in progeroid mice (Barcena et al., Reference Barcena, Valdes-Mas, Mayoral, Garabaya, Durand, Rodriguez, Fernandez-Garcia, Salazar, Nogacka, Garatachea, Bossut, Aprahamian, Lucia, Kroemer, Freije, Quiros and Lopez-Otin2019). Whether these microbiota-targeted interventions could impact brain health during advanced ageing was not assessed in these studies, but other research has demonstrated a protective effect of Akkermansia in neurologic diseases including models of multiple sclerosis, amyotrophic lateral sclerosis, epilepsy and Alzheimer’s disease (Blacher et al., Reference Blacher, Bashiardes, Shapiro, Rothschild, Mor, Dori-Bachash, Kleimeyer, Moresi, Harnik, Zur, Zabari, Brik, Kviatcovsky, Zmora, Cohen, Bar, Levi, Amar, Mehlman, Brandis, Biton, Kuperman, Tsoory, Alfahel, Harmelin, Schwartz, Israelson, Arike, Johansson, Hansson, Gotkine, Segal and Elinav2019; Cox et al., Reference Cox, Maghzi, Liu, Tankou, Dhang, Willocq, Song, Wasen, Tauhid, Chu, Anderson, De Jager, Polgar-Turcsanyi, Healy, Glanz, Bakshi, Chitnis and Weiner2021; Liu et al., Reference Liu, Rezende, Moreira, Tankou, Cox, Wu, Song, Dhang, Wei, Costamagna and Weiner2019; Olson et al., Reference Olson, Vuong, Yano, Liang, Nusbaum and Hsiao2018; Ou et al., Reference Ou, Deng, Lu, Wu, Liu, Huang and Peng2020), suggesting there could be a benefit of elevated Akkermansia in ageing. Alternatively, certain bacteria may be harmful rather than helpful. Research involving germ-free mice has shown that they live roughly 17 per cent longer than their specific pathogen free peers, suggesting that there may be consequential interactions between bacteria and their host that accelerate ageing (Tazume et al., Reference Tazume, Umehara, Matsuzawa, Aikawa, Hashimoto and Sasaki1991). Therefore, the elimination of these ageing-related potentially pathogenic microbes, perhaps through select antibiotic usage or CRISPR knockout of individual genes or pathogens, presents another potential intervention strategy. However, these strategies are currently limited by a lack of scientific understanding of what makes the bacteria behave pathogenically.
The gut microbiota actively contributes to brain health during ageing
The microbiota and the ageing brain: establishing connections
The ageing brain is characterised by changes on multiple levels ranging from cellular and morphological to functional changes (Peters, Reference Peters2006). Systemic low-grade inflammation occurs and can lead to increased permeability of the blood–brain barrier which can trigger microglia activation, cause neuroinflammation and can increase the production of reactive oxygen species which can lead to increased oxidative stress and mitochondrial dysfunction (Peters, Reference Peters2006). Ageing can also induce a decline in neuronal volume and alterations in neurotransmitter levels such as dopamine, serotonin and gamma-aminobutyric acid (GABA). This can trigger impaired synaptic plasticity and impaired neurogenesis and can cause functional deficiencies such as cognitive impairments (Figure 1). Following adulthood, the volume of the brain gradually shrinks, along with diminished grey and white matter in distinct brain areas in a sex-dependent manner such as cortical and subcortical areas, which are implicated in cognitive processes (Murphy et al., Reference Murphy, DeCarli, McIntosh, Daly, Mentis, Pietrini, Szczepanik, Schapiro, Grady, Horwitz and Rapoport1996; Peters, Reference Peters2006). Further changes in vasculature and diffusion can occur, which can underly neurodegenerative diseases and stroke (Lendahl et al., Reference Lendahl, Nilsson and Betsholtz2019).
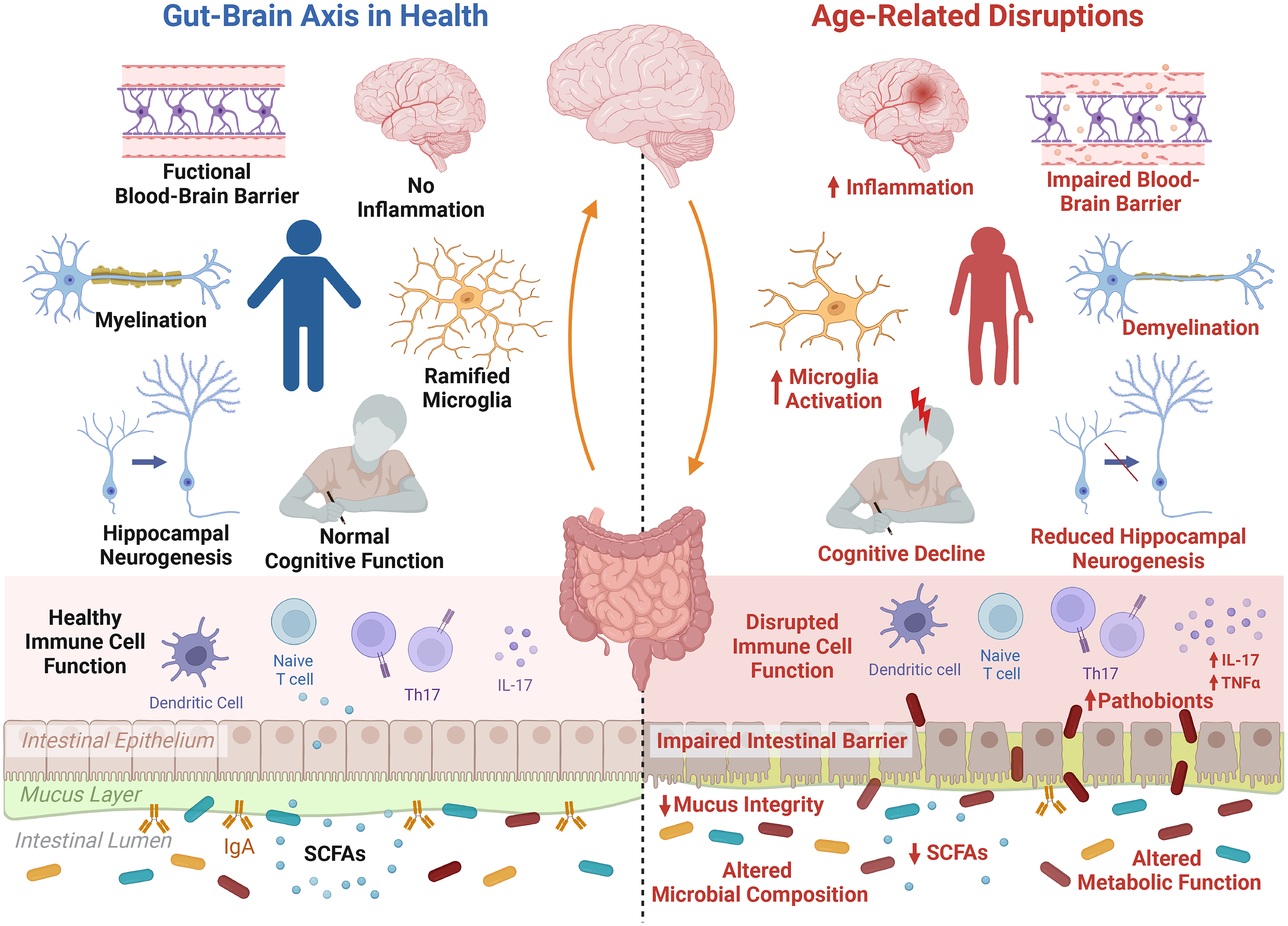
Figure 1 The microbiota–gut–brain axis during ageing. The process of ageing initiates distinct alterations in the gut, brain and signalling pathways. These manifest in alterations in the gut microbiota composition and metabolic function, including a reduction in short-chain fatty acids (SCFAs), depleted mucus integrity and impaired intestinal barrier function, allowing for increased invasion of pathobionts from the intestinal lumen. Furthermore, low-grade inflammation and immunosenescence occurs during ageing, disrupting the function of immune cell populations and leading to systemic inflammation triggered by increased pro-inflammatory cytokines, such as interleukin (IL)-17. This inflammatory state is also noticeable within the ageing brain, and is related to increased activation of microglia, the brain’s primary immune cell. Furthermore, ageing is associated with a decline in blood–brain barrier integrity, demyelination, a reduction in hippocampal neurogenesis and increased inflammation resulting in more microglia activation, which all in all may contribute to ageing-related cognitive decline. Created with BioRender.com.
While the impact of ageing on the mammalian (Fransen et al., Reference Fransen, van Beek, Borghuis, Aidy, Hugenholtz, van der Gaast-de Jongh, Savelkoul, De Jonge, Boekschoten, Smidt, Faas and de Vos2017; Thevaranjan et al., Reference Thevaranjan, Puchta, Schulz, Naidoo, Szamosi, Verschoor, Loukov, Schenck, Jury, Foley, Schertzer, Larche, Davidson, Verdu, Surette and Bowdish2017; van der Lugt et al., Reference van der Lugt, Rusli, Lute, Lamprakis, Salazar, Boekschoten, Hooiveld, Muller, Vervoort, Kersten, Belzer, Kok and Steegenga2018) and human (Biagi et al., Reference Biagi, Franceschi, Rampelli, Severgnini, Ostan, Turroni, Consolandi, Quercia, Scurti, Monti, Capri, Brigidi and Candela2016; Claesson et al., Reference Claesson, Jeffery, Conde, Power, O’Connor, Cusack, Harris, Coakley, Lakshminarayanan, O’Sullivan, Fitzgerald, Deane, O’Connor, Harnedy, O’Connor, O’Mahony, van Sinderen, Wallace, Brennan, Stanton, Marchesi, Fitzgerald, Shanahan, Hill, Ross and O’Toole2012; Jeffery et al., Reference Jeffery, Lynch and O’Toole2016; Leite et al., Reference Leite, Pimentel, Barlow and Mathur2022; Wilmanski et al., Reference Wilmanski, Diener, Rappaport, Patwardhan, Wiedrick, Lapidus, Earls, Zimmer, Glusman, Robinson, Yurkovich, Kado, Cauley, Zmuda, Lane, Magis, Lovejoy, Hood, Gibbons, Orwoll and Price2021) gut microbiome is well established, the impact of the gut microbiota on brain ageing has only recently been investigated, and relies dominantly on preclinical evidence and association analysis derived from small clinical trials.
Studies utilising FMT have demonstrated that the gut microbiota from aged individuals has the capacity to hinder cognitive performance and neurobiological phenotypes when transferred to younger individuals. For instance, transplanting microbes from aged and diseased models to young mice has been shown to impair learning, memory and neuroplasticity in recipient young mice (D’Amato et al., Reference D’Amato, Di Cesare Mannelli, Lucarini, Man, Le Gall, Branca, Ghelardini, Amedei, Bertelli, Regoli, Pacini, Luciani, Gallina, Altera, Narbad, Gulisano, Hoyles, Vauzour and Nicoletti2020; Kim et al., Reference Kim, Ho Jeon, Gyoung Ju, Sung Gee, Do, Sook Oh and Kil Lee2021; Li et al., Reference Li, Ning, Yin, Wang, Zhang, Hao, Wang, Zhao, Yang, Yin, Wu, Guo and Zhang2020). This coincided with alterations in microbial metabolites such as short-chain fatty acids (SCFAs; D’Amato et al., Reference D’Amato, Di Cesare Mannelli, Lucarini, Man, Le Gall, Branca, Ghelardini, Amedei, Bertelli, Regoli, Pacini, Luciani, Gallina, Altera, Narbad, Gulisano, Hoyles, Vauzour and Nicoletti2020) which have previously been shown to regulate host microglial maturation and function (Erny et al., Reference Erny, Dokalis, Mezo, Castoldi, Mossad, Staszewski, Frosch, Villa, Fuchs, Mayer, Neuber, Sosat, Tholen, Schilling, Vlachos, Blank, Gomez de Aguero, Macpherson, Pearce and Prinz2021). Moreover, using humanised models by transplanting FMT from older subjects with Alzheimer’s disease into microbiota-depleted naïve adult rats showed impairment in hippocampal neurogenesis accompanied with memory deficits, which correlated with clinical cognitive scores (Grabrucker et al., Reference Grabrucker, Marizzoni, Silajdžić, Lopizzo, Mombelli, Nicolas, Dohm-Hansen, Scassellati, Moretti, Rosa, Hoffmann, English, Lavelle, O’Neill, Thuret, Cattaneo and Nolan2022). Furthermore, young mice who received gut microbiota from aged donors suffered increased rates of mortality following ischemic stroke, along with increased levels of pro-inflammatory plasma cytokines and impaired motor strength (Spychala et al., Reference Spychala, Venna, Jandzinski, Doran, Durgan, Ganesh, Ajami, Putluri, Graf, Bryan and McCullough2018). Conversely, aged mice who were colonised with the microbiota from young donor mice had increased survival and improved recovery post-stroke (Spychala et al., Reference Spychala, Venna, Jandzinski, Doran, Durgan, Ganesh, Ajami, Putluri, Graf, Bryan and McCullough2018), demonstrating the functional differences between microbiota derived from young or aged individuals in influencing brain recovery following trauma.
The age of the FMT recipient also appears to have a strong influence on how an individual responds to an FMT. Counterintuitively, young germ-free (GF) mice who received microbiota from old mice demonstrated increased hippocampal neurogenesis and signs of pro-longevity compared to those who received FMT from young mice, while the same effects did not occur in aged GF mice (Kundu et al., Reference Kundu, Lee, Garcia-Perez, Tay, Kim, Faylon, Martin, Purbojati, Drautz-Moses, Ghosh, Nicholson, Schuster, Holmes and Pettersson2019). Hippocampal neurogenesis is influenced by the gut microbiota (Guzzetta et al., Reference Guzzetta, Cryan and O’Leary2022; Ogbonnaya et al., Reference Ogbonnaya, Clarke, Shanahan, Dinan, Cryan and O’Leary2015), and is well characterised to decline with ageing (Kozareva et al., Reference Kozareva, Cryan and Nolan2019), suggesting there are underlying age-driven factors that may supersede how an individual responds to a microbial community (Wilmanski et al., Reference Wilmanski, Gibbons and Price2022). Overall, these studies highlight that there are functional differences in the gut microbiota of aged individuals which may be contributing to the decline in cognition and altered neurobiology that occurs during ageing.
Fascinatingly, the gut microbiota from young mice appears to harness properties that enable it to rejuvenate aspects of brain ageing when transferred into aged mice. Two studies recently confirmed similar findings, wherein FMT from young mice to aged mice improved ageing-related deficits in memory and learning ability (Boehme, Guzzetta, Bastiaanssen et al., Reference Boehme, Guzzetta, Bastiaanssen, van de Wouw, Moloney, Gual-Grau, Spichak, Olavarría-Ramírez, Fitzgerald, Morillas, Ritz, Jaggar, Cowan, Crispie, Donoso, Halitzki, Neto, Sichetti, Golubeva, Fitzgerald, Claesson, Cotter, O’Leary, Dinan and Cryan2021; Mossad et al. Reference Mossad, Nent, Woltemate, Folschweiller, Buescher, Schnepf, Erny, Staeheli, Bartos, Szalay, Stecher, Vital, Sauer, Lämmermann, Prinz and Blank2021). Microbiota from young mice restored age-related changes in peripheral and hippocampal immune responses and reversed age-related alterations in hippocampal transcriptional profiles and metabolites (Boehme, Guzzetta, Bastiaanssen et al., Reference Boehme, Guzzetta, Bastiaanssen, van de Wouw, Moloney, Gual-Grau, Spichak, Olavarría-Ramírez, Fitzgerald, Morillas, Ritz, Jaggar, Cowan, Crispie, Donoso, Halitzki, Neto, Sichetti, Golubeva, Fitzgerald, Claesson, Cotter, O’Leary, Dinan and Cryan2021), suggesting potential mechanisms by which the gut microbiota from young mice improve cognitive performance by modulating immune and metabolic pathways. In this regard, the microbially-derived metabolite δ-valerobetaine, which is increased in aged mice and humans, was shown to directly impair learning and memory abilities, and was reduced in aged mice following FMT from young donor mice (Mossad et al., Reference Mossad, Nent, Woltemate, Folschweiller, Buescher, Schnepf, Erny, Staeheli, Bartos, Szalay, Stecher, Vital, Sauer, Lämmermann, Prinz and Blank2021). Relatedly, other gut microbiota-derived metabolites which are linked to age-related shifts in the gut microbiota and are increased in aged humans indicate that specific microbially-derived metabolites can impair cognitive abilities during ageing. For example, isoamylamine supplementation induced cognitive dysfunction by triggering microglia cell death in young mice (Teng et al., Reference Teng, Mu, Xu, Zhang, Sriwastva, Liu, Li, Lei, Sundaram, Hu, Zhang, Park, Hwang, Rouchka, Zhang, Yan, Merchant and Zhang2022). Meanwhile, N6-carboxymethyllysine administration lead to oxidative stress and mitochondrial damage in microglia in young and aged mice, although a starker effect was evident in young mice when N6-carboxymethyllysine was administered intraperitonially rather than by oral gavage whereas the delivery method was insignificant in aged mice, suggesting a protective role of the intestinal barrier in minimising damage caused by harmful ageing-related microbially-derived metabolites (Mossad et al., Reference Mossad, Batut, Yilmaz, Dokalis, Mezo, Nent, Nabavi, Mayer, Maron, Buescher, de Aguero, Szalay, Lammermann, Macpherson, Ganal-Vonarburg, Backofen, Erny, Prinz and Blank2022).
On the other hand, it is currently unclear whether ageing-driven changes in the gut are protective or harmful to cognitive health in ageing. For instance, research by Wilmanski et al. (Reference Wilmanski, Diener, Rappaport, Patwardhan, Wiedrick, Lapidus, Earls, Zimmer, Glusman, Robinson, Yurkovich, Kado, Cauley, Zmuda, Lane, Magis, Lovejoy, Hood, Gibbons, Orwoll and Price2021) found that a higher gut microbiome uniqueness score correlated to several health metrics in elderly individuals, whereas a lower gut microbiome uniqueness score was associated with earlier mortality. This could suggest that ageing-related changes in the gut foster increased uniqueness in the microbiome which may in turn prevent or slow the progression of ageing. Therefore, there may exist key bacteria that are increased in healthy ageing, and the inability of these probiotic bacteria to sustain and function in the unhealthy ageing gut may underlie consequences of ageing. With this theory in mind, we should not assume that FMT from young to aged individuals provides only beneficial effects, as this procedure could also influence the existing, sensitive microorganisms supporting healthy ageing. In order to fully understand the beneficial and/or harmful impacts of FMT on the ageing brain, more research needs to be conducted to thoroughly investigate how FMT may impact broad aspects of health in ageing.
The gut microbiome during brain ageing and in age-related neurodegenerative diseases
Accumulating evidence is revealing associations between the gut microbiota and neurodegenerative diseases associated with ageing, including mild cognitive impairment (MCI), Alzheimer’s disease (AD), Parkinson’s disease (PD) and multiple sclerosis (MS) (Alkasir et al., Reference Alkasir, Li, Li, Jin and Zhu2017; Cox et al., Reference Cox, Maghzi, Liu, Tankou, Dhang, Willocq, Song, Wasen, Tauhid, Chu, Anderson, De Jager, Polgar-Turcsanyi, Healy, Glanz, Bakshi, Chitnis and Weiner2021; Guo, Reference Guo, Huang, Xiao, Huang and Zuo2021; Romano et al., Reference Romano, Savva, Bedarf, Charles, Hildebrand and Narbad2021; Saji et al., Reference Saji, Niida, Murotani, Hisada, Tsuduki, Sugimoto, Kimura, Toba and Sakurai2019; Vogt et al., Reference Vogt, Kerby, Dill-McFarland, Harding, Merluzzi, Johnson, Carlsson, Asthana, Zetterberg, Blennow, Bendlin and Rey2017). In addition to alterations in the microbiota in human populations, studies involving preclinical disease models highlight the potential causal roles which the gut microbiota plays in host neurodegenerative diseases (Berer et al., Reference Berer, Gerdes, Cekanaviciute, Jia, Xiao, Xia, Liu, Klotz, Stauffer, Baranzini, Kumpfel, Hohlfeld, Krishnamoorthy and Wekerle2017; Dodiya et al., Reference Dodiya, Lutz, Weigle, Patel, Michalkiewicz, Roman-Santiago, Zhang, Liang, Srinath, Zhang, Xia, Olszewski, Zhang, Schipma, Chang, Tanzi, Gilbert and Sisodia2021; Harach et al., Reference Harach, Marungruang, Duthilleul, Cheatham, Mc Coy, Frisoni, Neher, Fåk, Jucker, Lasser and Bolmont2017; Perez-Pardo et al., Reference Perez-Pardo, Kliest, Dodiya, Broersen, Garssen, Keshavarzian and Kraneveld2017; Sampson et al., Reference Sampson, Debelius, Thron, Janssen, Shastri, Ilhan, Challis, Schretter, Rocha, Gradinaru, Chesselet, Keshavarzian, Shannon, Krajmalnik-Brown, Wittung-Stafshede, Knight and Mazmanian2016). The interaction of the microbiota in AD, PD and MS has been extensively studied (Kincaid et al., Reference Kincaid, Nagpal and Yadav2021; Mirza et al., Reference Mirza, Forbes, Zhu, Bernstein, Van Domselaar, Graham, Waubant and Tremlett2020; Tansey et al., Reference Tansey, Wallings, Houser, Herrick, Keating and Joers2022). Thus, we will focus our review on highlighting key findings related to the contribution of the ageing gut microbiota to these diseases and healthy brain ageing, and the current evidence for the use of gut microbiota-targeted therapeutics.
Alzheimer’s disease and mild cognitive impairment
The most common age-related dementia, AD, is estimated to affect about 57 million individuals worldwide with projections to 152 million in 2050 (Nichols et al., Reference Nichols, Steinmetz, Vollset, Fukutaki, Chalek, Abd-Allah, Abdoli, Abualhasan, Abu-Gharbieh, Akram, Al Hamad, Alahdab, Alanezi, Alipour, Almustanyir, Amu, Ansari, Arabloo, Ashraf, Astell-Burt, Ayano, Ayuso-Mateos, Baig, Barnett, Barrow, Baune, Béjot, Bezabhe, Bezabih, Bhagavathula, Bhaskar, Bhattacharyya, Bijani, Biswas, Bolla, Boloor, Brayne, Brenner, Burkart, Burns, Cámera, Cao, Carvalho, Castro-de-Araujo, Catalá-López, Cerin, Chavan, Cherbuin, Chu, Costa, Couto, Dadras, Dai, Dandona, Dandona, De la Cruz-Góngora, Dhamnetiya, Dias da Silva, Diaz, Douiri, Edvardsson, Ekholuenetale, El Sayed, El-Jaafary, Eskandari, Eskandarieh, Esmaeilnejad, Fares, Faro, Farooque, Feigin, Feng, Fereshtehnejad, Fernandes, Ferrara, Filip, Fillit, Fischer, Gaidhane, Galluzzo, Ghashghaee, Ghith, Gialluisi, Gilani, Glavan, Gnedovskaya, Golechha, Gupta, Gupta, Gupta, Haider, Hall, Hamidi, Hanif, Hankey, Haque, Hartono, Hasaballah, Hasan, Hassan, Hay, Hayat, Hegazy, Heidari, Heidari-Soureshjani, Herteliu, Househ, Hussain, Hwang, Iacoviello, Iavicoli, Ilesanmi, Ilic, Ilic, Irvani, Iso, Iwagami, Jabbarinejad, Jacob, Jain, Jayapal, Jayawardena, Jha, Jonas, Joseph, Kalani, Kandel, Kandel, Karch, Kasa, Kassie, Keshavarz, Khan, Khatib, Khoja, Khubchandani, Kim, Kim, Kisa, Kisa, Kivimäki, Koroshetz, Koyanagi, Kumar, Kumar, Lak, Leonardi, Li, Lim, Liu, Liu, Logroscino, Lorkowski, Lucchetti, Lutzky Saute, Magnani, Malik, Massano, Mehndiratta, Menezes, Meretoja, Mohajer, Mohamed Ibrahim, Mohammad, Mohammed, Mokdad, Mondello, Moni, Moniruzzaman, Mossie, Nagel, Naveed, Nayak, Neupane Kandel, Nguyen, Oancea, Otstavnov, Otstavnov, Owolabi, Panda-Jonas, Pashazadeh Kan, Pasovic, Patel, Pathak, Peres, Perianayagam, Peterson, Phillips, Pinheiro, Piradov, Pond, Potashman, Pottoo, Prada, Radfar, Raggi, Rahim, Rahman, Ram, Ranasinghe, Rawaf, Rawaf, Rezaei, Rezapour, Robinson, Romoli, Roshandel, Sahathevan, Sahebkar, Sahraian, Sathian, Sattin, Sawhney, Saylan, Schiavolin, Seylani, Sha, Shaikh, Shaji, Shannawaz, Shetty, Shigematsu, Shin, Shiri, Silva, Silva, Silva, Singh, Skryabin, Skryabina, Smith, Soshnikov, Spurlock, Stein, Sun, Tabarés-Seisdedos, Thakur, Timalsina, Tovani-Palone, Tran, Tsegaye, Valadan Tahbaz, Valdez, Venketasubramanian, Vlassov, Vu, Vu, Wang, Wimo, Winkler, Yadav, Yahyazadeh Jabbari, Yamagishi, Yang, Yano, Yonemoto, Yu, Yunusa, Zadey, Zastrozhin, Zastrozhina, Zhang, Murray and Vos2022) while pre-dementia states such MCI has a prevalence of about 16 per cent amongst elderly subjects in the USA, with amnestic MCI, which has a high likelihood to progress to AD, as the most common type (Petersen et al., Reference Petersen, Roberts, Knopman, Geda, Cha, Pankratz, Boeve, Tangalos, Ivnik and Rocca2010). Notably, the gut microbiota of AD patients living in the USA has been shown to contain reduced relative abundance of Firmicutes and Bifidobacterium, and higher levels of Bacteroidetes, including Bacteroides (Vogt et al., Reference Vogt, Kerby, Dill-McFarland, Harding, Merluzzi, Johnson, Carlsson, Asthana, Zetterberg, Blennow, Bendlin and Rey2017). In a Chinese cohort AD patients had lower levels of Lachnospira, Bacteroides and Ruminiclostridium_9, as well as an increased abundance of Prevotella compared with healthy, age-matched peers (Guo, Reference Guo, Huang, Xiao, Huang and Zuo2021). Similarly, a Japanese cohort examining elderly patients with mild cognitive impairment but no dementia, versus healthy elderly found higher prevalence of Bacteroides in patients with cognitive decline (Saji et al., Reference Saji, Niida, Murotani, Hisada, Tsuduki, Sugimoto, Kimura, Toba and Sakurai2019), though others have observed only Lachnospira was significantly lower in patients with mild cognitive impairments (Guo, Reference Guo, Huang, Xiao, Huang and Zuo2021). Additional research into gut microbiota of individuals ranging from healthy ageing over MCI to AD has revealed that bacterial genera that were differentially abundant in AD were also different in MCI, suggesting that changes in the gut microbiome might precede AD onset (Li et al., Reference Li, He, Ma, Huang, Du, Cao, Wang, Xiao, Tang and Chen2019).
While clinical mechanistic evidence is largely lacking, two case studies reveal a glimpse of hope for the direct potential of the gut microbiota to alleviate symptoms of dementia in AD. Fascinatingly, an 82-year-old man with AD who was administered FMT to treat Clostridioides difficile infection showed improved cognitive score in the Mini-Mental State Examination (MMSE) from MCI levels to healthy cognition at his 2-month follow-up visit (Hazan, Reference Hazan2020). Similarly, a 90-year-old woman suffering from AD treated with FMT for C. difficile displayed a marked improvement in several cognitive function tests within 3 months (Park et al., Reference Park, Lee, Shin, Kim, Cha, Lee, Kwon, Shin and Choi2021). This clinical FMT literature, albeit limited by sample size, supports the translatability of previous pre-clinical findings wherein FMT from young C57BL/6 wild-type male mice rejuvenated aspects of cognition when transplanted into aged male C57BL/6 mice (Boehme, Guzzetta, Bastiaanssen et al., Reference Boehme, Guzzetta, Bastiaanssen, van de Wouw, Moloney, Gual-Grau, Spichak, Olavarría-Ramírez, Fitzgerald, Morillas, Ritz, Jaggar, Cowan, Crispie, Donoso, Halitzki, Neto, Sichetti, Golubeva, Fitzgerald, Claesson, Cotter, O’Leary, Dinan and Cryan2021). Furthermore, FMT from wild-type mice into male APP/PS1 transgenic mice, a genetic mouse model of AD, led to reductions in memory impairment, Aβ accumulation, synaptic dysfunction and neuroinflammation (Sun et al., Reference Sun, Xu, Ling, Wang, Gong, Yang, Ye, Ye, Wei, Song, Chen and Liu2019), while FMT from an additional genetic AD mouse model known as 5xFAD mice into naïve C57BL/6 mice lead to decreased hippocampal neurogenesis and cognitive impairment (Kim et al., Reference Kim, Ho Jeon, Gyoung Ju, Sung Gee, Do, Sook Oh and Kil Lee2021). In addition to the transfer of the full microbiota between animals, attempts to modulate AD pathology by administrating specific AD-associated bacteria have also been made. Increasing levels of Bacteroides were reported in ageing Tg2576 transgenic mice and correlated to the amyloid plaque levels in their brains, and weekly administration of Bacteroides fragilis between 2 and 5 months of age increased amyloid plaque burden in APP/PS1 mice (Cox et al., Reference Cox, Schafer, Sohn, Vincentini, Weiner, Ginsberg and Blaser2019).
Furthermore, there are hypotheses that the gut microbiota contributes to the development of AD, which is supported by preclinical evidence involving antibiotic administration to rodent models of AD. For instance, cocktail of antibiotics given to three different amyloid mouse models of AD (APP/PS1, 5xFAD and AppNL-G-F) from early age reduced the amyloid plaque load observed later in life in male mice (Guilherme et al., Reference Guilherme, Nguyen, Reinhardt and Endres2021; Kaur et al., Reference Kaur, Nookala, Singh, Mukundan, Nagamoto-Combs and Combs2021; Mezö et al., Reference Mezö, Dokalis, Mossad, Staszewski, Neuber, Yilmaz, Schnepf, de Agüero, Ganal-Vonarburg, Macpherson, Meyer-Luehmann, Staeheli, Blank, Prinz and Erny2020; Minter et al., Reference Minter, Zhang, Leone, Ringus, Zhang, Oyler-Castrillo, Musch, Liao, Ward, Holtzman, Chang, Tanzi and Sisodia2016), while no difference has been observed in females. It was further demonstrated that it was sufficient to administer the antibiotic cocktail early in life (pre-weaning) to observe lasting alterations in the gut microbiome and reduced amyloid pathology later in life (Minter et al., Reference Minter, Hinterleitner, Meisel, Zhang, Leone, Zhang, Oyler-Castrillo, Zhang, Musch, Shen, Jabri, Chang, Tanzi and Sisodia2017). The administration of individual antibiotics, however, did not reduce the accumulation of amyloid plaques (Dodiya et al., Reference Dodiya, Frith, Sidebottom, Cao, Koval, Chang and Sisodia2020). The antibiotic cocktail administered early in life reduced alpha-diversity in the gut but led to increased relative abundance of Akkermansia in the APP/PS1 model (Minter et al., Reference Minter, Hinterleitner, Meisel, Zhang, Leone, Zhang, Oyler-Castrillo, Zhang, Musch, Shen, Jabri, Chang, Tanzi and Sisodia2017). Interestingly, mice given antibiotics in early life had lasting alterations to peripheral and central immunity, with increased proportions of regulatory T cells. Moreover, microglia appears critical for inducing this response; mice with depleted microglia did not have reduced plaque levels after treatment with the antibiotic cocktail, although microglia depletion also slightly reduced plaque burden (Dodiya et al., Reference Dodiya, Lutz, Weigle, Patel, Michalkiewicz, Roman-Santiago, Zhang, Liang, Srinath, Zhang, Xia, Olszewski, Zhang, Schipma, Chang, Tanzi, Gilbert and Sisodia2021). Antibiotic treatment in the 5xFAD model of AD improved spatial and recognition memory performance, which was also demonstrated in GF mice (Mezö et al., Reference Mezö, Dokalis, Mossad, Staszewski, Neuber, Yilmaz, Schnepf, de Agüero, Ganal-Vonarburg, Macpherson, Meyer-Luehmann, Staeheli, Blank, Prinz and Erny2020). Treatment with antibiotics from the timepoint of weaning also reduced anxiety in wild-type mice that were subjected to an intracerebral injection of amyloid peptides to simulate the onset of AD at 80 days of age (Mosaferi et al., Reference Mosaferi, Jand and Salari2021). Overall, this growing body of evidence strongly implicates the gut microbiota in the pathology of AD, as well as a potential target for novel therapeutics.
Parkinson’s disease
Parkinson’s disease (PD) is the second most common age-related neurodegenerative disease, and may have unique influences from the gut microbiota initiating disease pathogenesis in the gut (Yang et al., Reference Yang, Zhao, Ali Shah, Wu, Lai, Zhang, Li, Guan, Zhao, Li, Gao, Zhou and Yang2019). Mouse studies suggest that the underlying mechanism causing the death of dopaminergic neurons in the striatum, a brain region that controls motor behaviour, is caused by the propagation of misfolded alpha-synuclein along the vagus nerve (Kim et al., Reference Kim, Choi, Shin, Jin, Bae, Han, Seo, Chun and Chung2019a). Indeed, PD patients show gastrointestinal symptoms long before the onset of PD (Sung et al., Reference Sung, Park and Kim2014). A Danish study investigated the risk for PD in subjects who underwent vagotomy back in the 1970s and 1980s when it was used as a therapy to treat peptic ulcer disease and found that truncal vagotomy, which cuts the entire nerve, was associated with a decreased risk to develop PD (Svensson et al., Reference Svensson, Horvath-Puho, Thomsen, Djurhuus, Pedersen, Borghammer and Sorensen2015). This observation was confirmed two years later in a Swedish cohort (Liu et al., Reference Liu, Fang, Pedersen, Tillander, Ludvigsson, Ekbom, Svenningsson, Chen and Wirdefeldt2017). These epidemiological findings support the view that PD initially commences in the gut and not the brain, and strongly implicates a role of the vagus nerve in the pathogenesis of PD. A recent preprint now suggests that inflammatory bowel disease (IBD) is linked to PD suggesting that anti-inflammatory therapies targeting the gut may be preventive for PD later in life (Espinosa-Oliva et al., Reference Espinosa-Oliva, Laza, Soto, Serrano, Perez, Ceballos, Revilla, Pavon, Serres, Economopoulus, Vazquez, Carretero, Miranda, Klementieva, Martin, Deierborg, Infante, Sibson, Garcia, de la Quintana, Rubio, Carmona, Recio and de Pablos2022). In animal models of PD, transfer of microbiota from new-onset treatment-naïve PD patients worsened motor function in alpha-synuclein overexpressing mice (Sampson et al., Reference Sampson, Debelius, Thron, Janssen, Shastri, Ilhan, Challis, Schretter, Rocha, Gradinaru, Chesselet, Keshavarzian, Shannon, Krajmalnik-Brown, Wittung-Stafshede, Knight and Mazmanian2016), and depleting the microbiota with antibiotics or by germ-free status improved motor symptoms, which was linked to changes in microglia function. This study supports the potential role of the gut microbiota in contributing to PD. In a study of Pink1–/– mice, early-life infection with Citrobacter rodentium increased motor dysfunction and disease pathogenesis, which was linked to increased mitochondrial specific cytotoxic CD8 T cells in the brain (Matheoud et al., Reference Matheoud, Cannon, Voisin, Penttinen, Ramet, Fahmy, Ducrot, Laplante, Bourque, Zhu, Cayrol, Le Campion, McBride, Gruenheid, Trudeau and Desjardins2019). While PD is considered an age-related disease, this study suggests that immunologic programming in infancy may affect PD pathogenesis. Thus, gut-microbiota interactions throughout lifespan may be important determinants of age-related neurologic disease.
Multiple sclerosis
Multiple sclerosis (MS) is a demyelinating neurodegenerative disease, with a typical age of onset in the 20s to 40s, and ageing can play an important role in disease progression (Dobson and Giovannoni, Reference Dobson and Giovannoni2019). The majority of MS patients develop a relapsing remitting form of the disease (RRMS), while a smaller proportion of patients will initially present with a progressive form of the disease (primary progressive MS, PPMS (Lassmann, Reference Lassmann2018). Throughout the disease course, many RRMS patients will transition to a secondary progressive disease course (SPMS), and ageing is one of the largest risk factors for developing SPMS (Lassmann, Reference Lassmann2018). Because progressive MS is more refractory to treatment and is associated with higher disability and quality of life, it is critical to understand whether the host-microbiome interactions in ageing affect this transition. Patients diagnosed with progressive MS show increased Akkermansia, Clostridium bolteae and Ruthenibacterium lactatiformans along with reduced levels of Blautia wexlerae, Dorea formicigenerans and Erysipelotrichaceae CCMM and altered microbial β-diversity (Cox et al., Reference Cox, Maghzi, Liu, Tankou, Dhang, Willocq, Song, Wasen, Tauhid, Chu, Anderson, De Jager, Polgar-Turcsanyi, Healy, Glanz, Bakshi, Chitnis and Weiner2021). The abundance of Clostridium species was associated with worsened disability status on the Expanded Disability Status Scale, suggesting a potentially detrimental role. Interestingly, although Akkermansia was elevated in progressive MS, higher abundance of Akkermansia correlated with lower disability, and was demonstrated to ameliorate aspects of disease pathology in a mouse model of multiple sclerosis, perhaps through its ability to reduce RORγt+ and IL-17–producing γδ T cells (Cox et al., Reference Cox, Maghzi, Liu, Tankou, Dhang, Willocq, Song, Wasen, Tauhid, Chu, Anderson, De Jager, Polgar-Turcsanyi, Healy, Glanz, Bakshi, Chitnis and Weiner2021). Therefore, it might be that some microbes act protectively against the progression of neurodegenerative disease, and that the absence of these species could be detrimental, although further research must be conducted in order to conclude this. In an animal model of MS known as the experimental autoimmune encephalomyelitis (EAE) model, treatment with antibiotics ameliorates disease and GF mice resist EAE, suggesting that the microbiota can contribute to diseases pathogenesis. Interestingly, the colonization of mice with the putative probiotic species Lactobacillus reuteri exacerbated EAE susceptibility, likely through L. reuteri’s ability to metabolize dietary tryptophan into active metabolites which can induce IL-17 production by binding to the aryl hydrocarbon receptor on T cells (Montgomery et al., Reference Montgomery, Eckstrom, Lile, Caldwell, Heney, Lahue, D’Alessandro, Wargo and Krementsov2022). Furthermore, transferring microbiota from MS patients into GF mice worsened EAE (Berer et al., Reference Berer, Gerdes, Cekanaviciute, Jia, Xiao, Xia, Liu, Klotz, Stauffer, Baranzini, Kumpfel, Hohlfeld, Krishnamoorthy and Wekerle2017; Cekanaviciute et al., Reference Cekanaviciute, Yoo, Runia, Debelius, Singh, Nelson, Kanner, Bencosme, Lee, Hauser, Crabtree-Hartman, Sand, Gacias, Zhu, Casaccia, Cree, Knight, Mazmanian and Baranzini2017). In terms of ageing, recent studies have shown that mice show a more progressive form of MS when they are older (Cahill et al., Reference Cahill, Zhang, Ramaglia, Whetstone, Sabbagh, Yi, Woo, Przybycien, Moshkova, Zhao, Rojas, Gomes, Kuerten, Gommerman, Sled and Dunn2019), raising the question if age-related changes in the gut microbiota may drive MS disease progression.
Potential mechanisms for microbiota–gut–brain signalling in ageing
The mechanism(s) of action underlying microbiota–gut–brain communication in ageing-related cognitive decline remain elusive. Given the increased inflammation and immunosenescence which occurs during ageing, it is probable that alterations to microbiota-immune signalling contribute to differences in host brain ageing. Indeed, recently literature has demonstrated that some ageing-induced alterations to gut-associated immunity were reversed following FMT from young to aged mice (Boehme, Guzzetta, Bastiaanssen et al., Reference Boehme, Guzzetta, Bastiaanssen, van de Wouw, Moloney, Gual-Grau, Spichak, Olavarría-Ramírez, Fitzgerald, Morillas, Ritz, Jaggar, Cowan, Crispie, Donoso, Halitzki, Neto, Sichetti, Golubeva, Fitzgerald, Claesson, Cotter, O’Leary, Dinan and Cryan2021). This FMT also resulted in decreased microglia soma area and reversed the age-associated increase in proinflammatory-associated microglial genes, which indicates decreased immune activation in the brain following FMT from young mice into aged mice (Boehme, Guzzetta, Bastiaanssen et al., Reference Boehme, Guzzetta, Bastiaanssen, van de Wouw, Moloney, Gual-Grau, Spichak, Olavarría-Ramírez, Fitzgerald, Morillas, Ritz, Jaggar, Cowan, Crispie, Donoso, Halitzki, Neto, Sichetti, Golubeva, Fitzgerald, Claesson, Cotter, O’Leary, Dinan and Cryan2021). Contrarily, this same study found that old mice who received microbiota from aged-matched mice had elevated plasma levels of the anti-inflammatory cytokine IL-10, which was not the case when old mice received FMT from young mice. This difference in plasma IL-10 could suggest that either transferring microbiota from young to aged mice may not only confer health benefits, or alternatively indicates that the inflammatory phenotype observed in old animals who received age-matched FMT was no longer responsive to suppression by heightened IL-10.
Ageing-associated gut microbiota was previously demonstrated to play a key role in host inflammageing, suggesting the potential for microbiota-driven changes in gut–brain axis communication during ageing. Aged GF mice have lower levels of systemic inflammatory cytokines, including IL-6 and TNFα and increased macrophage ability to phagocytose bacteria compared to conventional aged mice, suggesting that the microbiome plays an important role in both inflammageing and immunosenescence (Thevaranjan et al., Reference Thevaranjan, Puchta, Schulz, Naidoo, Szamosi, Verschoor, Loukov, Schenck, Jury, Foley, Schertzer, Larche, Davidson, Verdu, Surette and Bowdish2017). Furthermore, administering FMT from aged mice into young mice transferred the ageing-related phenotypes of inflammation (increased systemic IL-6 and TNFα), immune dysfunction and impaired gut barrier integrity, and was linked to a reduction of specific bacterial taxa such as Akkermansia (Fransen et al., Reference Fransen, van Beek, Borghuis, Aidy, Hugenholtz, van der Gaast-de Jongh, Savelkoul, De Jonge, Boekschoten, Smidt, Faas and de Vos2017; Thevaranjan et al., Reference Thevaranjan, Puchta, Schulz, Naidoo, Szamosi, Verschoor, Loukov, Schenck, Jury, Foley, Schertzer, Larche, Davidson, Verdu, Surette and Bowdish2017), directly implicating the gut microbiota as a causative contributor to inflammageing. Furthermore, when microbes were transferred from young to aged mice via heterochronic FMT, the ageing-induced deficiency in the Peyer’s patch germinal cell reaction, a key compartment of intestinal immunity, was rescued (Stebegg et al., Reference Stebegg, Silva-Cayetano, Innocentin, Jenkins, Cantacessi, Gilbert and Linterman2019). This work demonstrated that the age-associated deficiency in intestinal stem cell activity was reversible by the gut microbiome by possibly providing them with the appropriate stimuli (Stebegg et al., Reference Stebegg, Silva-Cayetano, Innocentin, Jenkins, Cantacessi, Gilbert and Linterman2019). While accumulating preclinical evidence strongly implicates the gut microbiota in regulating host longevity, health span and immunity during ageing, whether the gut microbiota regulates host brain ageing warrants further study.
Monocytes and microglia express stimulatory receptors that are activated by microbe-associated molecular patterns (MAMPs) and other small molecule secreted metabolites. These molecules are constantly produced by gut bacteria and shape host immunity (Chu and Mazmanian, Reference Chu and Mazmanian2013). Some studies suggest that bacterial components originating from the gut can be found in the human brain under pathological conditions. For example, a small study in six subjects with AD detected lipopolysaccharide (LPS) in the human hippocampus and neocortex (Zhao et al., Reference Zhao, Jaber and Lukiw2017). Meanwhile, the bacterially-derived metabolite trimethylamine-N-oxide has been measured in human cerebrospinal fluid (Rio et al., Reference Rio, Zimetti, Caffarra, Tassotti, Bernini, Brighenti, Zini and Zanotti2017). Furthermore, a role of the oral microbiome in triggering neurodegenerative diseases such as Alzheimer’s disease is increasingly discussed (Shoemark and Allen Reference Shoemark and Allen2015). For instance, toxins called gingipains from the oral bacteria Porphyromonas gingivalis have been identified in the brains of AD patients (Dominy et al., Reference Dominy, Lynch, Ermini, Benedyk, Marczyk, Konradi, Nguyen, Haditsch, Raha, Griffin, Holsinger, Arastu-Kapur, Kaba, Lee, Ryder, Potempa, Mydel, Hellvard, Adamowicz, Hasturk, Walker, Reynolds, Faull, Curtis, Dragunow and Potempa2019). GF wild-type mice, or mice treated with high-dose antibiotics to nearly eliminate the microbiota had impaired maturation of microglia, which could be partially restored by orally administered bacteria-derived SCFAs (Erny et al., Reference Erny, Hrabe de Angelis, Jaitin, Wieghofer, Staszewski, David, Keren-Shaul, Mahlakoiv, Jakobshagen, Buch, Schwierzeck, Utermohlen, Chun, Garrett, McCoy, Diefenbach, Staeheli, Stecher, Amit and Prinz2015). GF APP/PS1 mice, a model of AD, had lower amyloid beta (Aβ) protein levels, demonstrating that bacterial-derived metabolites can affect amyloid load (Harach et al., Reference Harach, Marungruang, Duthilleul, Cheatham, Mc Coy, Frisoni, Neher, Fåk, Jucker, Lasser and Bolmont2017; Minter et al., Reference Minter, Zhang, Leone, Ringus, Zhang, Oyler-Castrillo, Musch, Liao, Ward, Holtzman, Chang, Tanzi and Sisodia2016). Colonising GF APP/PS1 mice with the microbiome of a conventionally raised APP/PS1 mouse increased Aβ levels compared to those colonised with WT microbiota (Harach et al., Reference Harach, Marungruang, Duthilleul, Cheatham, Mc Coy, Frisoni, Neher, Fåk, Jucker, Lasser and Bolmont2017).
Recent literature demonstrated that bacterial-derived metabolite acetate regulates the function of microglia in the brain. In the 5xFAD mouse model of AD, oral administration of acetate to GF mice promoted a pro-inflammatory phenotype in cortical microglia, with reduced phagocytosis of amyloid plaques leading to accelerated pathology (Erny et al., Reference Erny, Dokalis, Mezo, Castoldi, Mossad, Staszewski, Frosch, Villa, Fuchs, Mayer, Neuber, Sosat, Tholen, Schilling, Vlachos, Blank, Gomez de Aguero, Macpherson, Pearce and Prinz2021). In contrast, work from a group in Japan showed that acetate partially counteracted cognitive impairment in another mouse model of AD in which Aβ was injected into the hippocampus of SPF mice (Kobayashi et al., Reference Kobayashi, Sugahara, Shimada, Mitsuyama, Kuhara, Yasuoka, Kondo, Abe and Xiao2017). In addition to acetate, it has been shown that supplementation with the second major short-chain fatty acid, butyrate, decreased neuroinflammation in aged mice (Matt et al., Reference Matt, Allen, Lawson, Mailing, Woods and Johnson2018). Another preclinical study found that when administered even at a late stage of Alzheimer’s disease-related pathology, butyrate supplementation improved associative memory in APP/PS1-21 mice (Govindarajan et al., Reference Govindarajan, Agis-Balboa, Walter, Sananbenesi and Fischer2011). The exact mechanism by which the gut microbiota exerts effects in AD through SCFAs and altered inflammation remains unknown, but potentially involves altered age-related pro-inflammatory monocyte trafficking to the brain (van de Wouw et al., Reference van de Wouw, Boehme, Dinan and Cryan2019), as microglia are not known to express receptors for SCFAs (Erny et al., Reference Erny, Hrabe de Angelis, Jaitin, Wieghofer, Staszewski, David, Keren-Shaul, Mahlakoiv, Jakobshagen, Buch, Schwierzeck, Utermohlen, Chun, Garrett, McCoy, Diefenbach, Staeheli, Stecher, Amit and Prinz2015). Furthermore, butyrate has been shown to affect the release of serotonin and gut hormone release in the enteric nervous system which can stimulate the vagus nerve and can trigger endocrine signalling, which can impact brain function (Stilling et al., Reference Stilling, van de Wouw, Clarke, Stanton, Dinan and Cryan2016).
In addition to SCFAs, other microbially-derived metabolites have also been implicated in brain health during ageing. As discussed previously, the microbially-produced metabolite δ-valerobetaine is more abundant in older animals and was found to impair learning and memory abilities (Mossad et al., Reference Mossad, Nent, Woltemate, Folschweiller, Buescher, Schnepf, Erny, Staeheli, Bartos, Szalay, Stecher, Vital, Sauer, Lämmermann, Prinz and Blank2021). Meanwhile, FMT from young mice into aged recipient mice lowered levels of δ-valerobetaine systemically and in the brain, and improved learning and memory performance (Mossad et al., Reference Mossad, Nent, Woltemate, Folschweiller, Buescher, Schnepf, Erny, Staeheli, Bartos, Szalay, Stecher, Vital, Sauer, Lämmermann, Prinz and Blank2021). Using data from the UK Biobank, the authors showed that increasing human age is associated with an increase in systemic δ-valerobetaine levels. Interestingly, supplementation of δ-valerobetaine to GF and conventional mice enlarged their visceral fat mass, and systemic levels of this metabolite correlate with visceral adipose tissue mass in humans (Liu et al., Reference Liu, Owens, Saeedi, Cohen, Bellissimo, Naudin, Darby, Druzak, Maner-Smith, Orr, Hu, Fernandes, Camacho, Hunter-Chang, VanInsberghe, Ma, Ganesh, Yeligar, Uppal, Go, Alvarez, Vos, Ziegler, Woodworth, Kraft, Jones, Ortlund, Neish and Jones2021), a critical factor for dementia later in life (Cereda et al., Reference Cereda, Sansone, Meola and Malavazos2007), suggesting δ-valerobetaine as a potential target for future therapies.
Meanwhile, indoles, which include a range of microbially-produced metabolites of dietary tryptophan, have been implicated to play a critical role in ageing. Through binding to the aryl hydrocarbon receptor, indoles can act locally on gut inflammation but also in the brain, as have been shown to modulate neuroinflammation through microglia control of astrocytes (Rothhammer et al., Reference Rothhammer, Borucki, Tjon, Takenaka, Chao, Ardura-Fabregat, de Lima, Gutierrez-Vazquez, Hewson, Staszewski, Blain, Healy, Neziraj, Borio, Wheeler, Dragin, Laplaud, Antel, Alvarez, Prinz and Quintana2018). Furthermore, indoles in blood plasma have been positively associated with gut microbiome uniqueness (Wilmanski et al., Reference Wilmanski, Diener, Rappaport, Patwardhan, Wiedrick, Lapidus, Earls, Zimmer, Glusman, Robinson, Yurkovich, Kado, Cauley, Zmuda, Lane, Magis, Lovejoy, Hood, Gibbons, Orwoll and Price2021). Of note, Bacteroides species produce indoles, and high level of Bacteroides was linked with increased mortality in 85+ individuals (Wilmanski et al., Reference Wilmanski, Diener, Rappaport, Patwardhan, Wiedrick, Lapidus, Earls, Zimmer, Glusman, Robinson, Yurkovich, Kado, Cauley, Zmuda, Lane, Magis, Lovejoy, Hood, Gibbons, Orwoll and Price2021).
Accumulating clinical evidence suggests a link between bile acids and dementia (MahmoudianDehkordi et al., Reference MahmoudianDehkordi, Arnold, Nho, Ahmad, Jia, Xie, Louie, Kueider-Paisley, Moseley, Thompson, St John Williams, Tenenbaum, Blach, Baillie, Han, Bhattacharyya, Toledo, Schafferer, Klein, Koal, Risacher, Kling, Motsinger-Reif, Rotroff, Jack, Hankemeier, Bennett, De Jager, Trojanowski, Shaw, Weiner, Doraiswamy, van Duijn, Saykin, Kastenmuller and Kaddurah-Daouk2019; Varma et al., Reference Varma, Wang, An, Varma, Bilgel, Doshi, Legido-Quigley, Delgado, Oommen, Roberts, Wong, Davatzikos, Resnick, Troncoso, Pletnikova, O’Brien, Hak, Baak, Pfeiffer, Baloni, Mohmoudiandehkordi, Nho, Kaddurah-Daouk, Bennett, Gadalla and Thambisetty2021; Wang et al., Reference Wang, Wei, Xie, Arnold, Kueider-Paisley, Louie, Mahmoudian Dehkordi, Blach, Baillie, Han, De Jager, Bennett, Kaddurah-Daouk and Jia2020). By combining transcriptomics analyses with a metabolic network analysis approach, researchers of the Alzheimer’s disease metabolomics consortium found that bile acid synthesis differs in AD compared to cognitively healthy individuals (Baloni et al., Reference Baloni, Funk, Yan, Yurkovich, Kueider-Paisley, Nho, Heinken, Jia, Mahmoudiandehkordi, Louie, Saykin, Arnold, Kastenmuller, Griffiths, Thiele, Alzheimer’s Disease Metabolomics, Kaddurah-Daouk and Price2020). Fascinatingly, the presence of some of these bile acids measured in the brain cannot be explained by locally expressed enzymes, which suggests that they may be derived from the gut microbiome (Baloni et al., Reference Baloni, Funk, Yan, Yurkovich, Kueider-Paisley, Nho, Heinken, Jia, Mahmoudiandehkordi, Louie, Saykin, Arnold, Kastenmuller, Griffiths, Thiele, Alzheimer’s Disease Metabolomics, Kaddurah-Daouk and Price2020). Preclinically, metabolomic analyses point to a role of secondary bile acids as a potential mechanism in rebalancing disturbed gut microbiota associated with an improvement in health and lifespan in progeria mice following FMT (Barcena et al., Reference Barcena, Valdes-Mas, Mayoral, Garabaya, Durand, Rodriguez, Fernandez-Garcia, Salazar, Nogacka, Garatachea, Bossut, Aprahamian, Lucia, Kroemer, Freije, Quiros and Lopez-Otin2019). Interestingly, a recent study found that the secondary bile acid, isoallolithocholic acid, is increased in centenarians (Sato et al., Reference Sato, Atarashi, Plichta, Arai, Sasajima, Kearney, Suda, Takeshita, Sasaki, Okamoto, Skelly, Okamura, Vlamakis, Li, Tanoue, Takei, Nittono, Narushima, Irie, Itoh, Moriya, Sugiura, Suematsu, Moritoki, Shibata, Littman, Fischbach, Uwamino, Inoue, Honda, Hattori, Murai, Xavier, Hirose and Honda2021) and has been shown to influence host T-cell function by increasing the differentiation of Treg cells through increasing mitochondrial activity (Hang et al., Reference Hang, Paik, Yao, Kim, Trinath, Lu, Ha, Nelson, Kelly, Wu, Zheng, Longman, Rastinejad, Devlin, Krout, Fischbach, Littman and Huh2019) though whether secondary bile acids can directly exert effects on the brain is currently unknown. Modulation of secondary bile acid metabolism might be a novel strategy to modulate brain ageing and may impact cognition in ageing.
The vagus nerve plays a fundamental role in microbiota–gut–brain axis signalling (Fulling et al., Reference Fulling, Dinan and Cryan2019). Intriguingly, a recent study found that an increase in vagal activity was able to mitigate the adverse effects of FMT from aged mouse donors on hippocampal function in young mice (Rei et al., Reference Rei, Saha, Haddad, Haider Rubio, Perlaza, Berard, Ungeheuer, Sokol and Lledo2022). Initial clinical data suggests that stimulation of the vagus nerve may improve some aspects of quality of life under specific conditions in elderly subjects (Bretherton et al., Reference Bretherton, Atkinson, Murray, Clancy, Deuchars and Deuchars2019), and improved walking speed and motor function in subjects with PD which was associated with a decrease in inflammatory biomarker and an increase in the myokine BDNF (Mondal et al., Reference Mondal, Choudhury, Banerjee, Roy, Chatterjee, Basu, Singh, Halder, Shubham, Baker, Baker and Kumar2021).
Strategies for targeting the gut microbiome for better brain health during ageing
As the causal relationships between the gut microbiota and host brain ageing become increasingly clear, it is critical to continue to investigate whether microbiota-targeted therapeutics hold the potential to ameliorate the effects of ageing onto the brain. Several approaches to altering the gut microbiota, including medical interventions such as FMT and antibiotics, as well as lifestyle choices such as diet including probiotics, prebiotics, Mediterranean diet and intermittent fasting, and exercise, may hold the key to the fountain of brain youth (Figure 2). In the following section, we review the existing evidence with a focus on clinical evidence, accompanied with preclinical data as supporting evidence.

Figure 2 Potential strategies for intervening on the microbiota–gut–brain axis for improved brain health in ageing. Abbreviations: FMT, faecal microbiota transplant; LPS, lipopolysaccharides; SCFAs, short-chain fatty acids. Created with BioRender.com.
Faecal microbiota transplant
Faecal microbiota transplant is now a commonly performed medical procedure to treat patients suffering from antibiotic-resistant C. difficile, and is being explored for use in a variety of other diseases and disorders, including pathologies impacting brain health (Sorbara and Pamer, Reference Sorbara and Pamer2022). Fascinatingly, small-scale clinical trials have shown that FMT can alleviate symptoms in patients with Parkinson’s disease (Kuai et al., Reference Kuai, Yao, Xu, Zhou, Zhang, Liu, Pei and Zhou2021; Xue et al., Reference Xue, Yang, Tong, Shen, Ma, Wu, Zheng and Wang2020), although these studies are limited by small sample size. Furthermore, individual case studies have shown that FMT was sufficient to improve metrics of mobility in a patient with multiple sclerosis (Engen et al., Reference Engen, Zaferiou, Rasmussen, Naqib, Green, Fogg, Forsyth, Raeisi, Hamaker and Keshavarzian2020), and improve cognitive performance in two individuals diagnosed with Alzheimer’s disease who were administered FMT to treat their C. difficile infections (Hazan, Reference Hazan2020; Park et al., Reference Park, Lee, Shin, Kim, Cha, Lee, Kwon, Shin and Choi2021). This evidence provides promise that FMT may offer a potential treatment option for patients suffering ageing-related neurological diseases.
Still, clinical FMT presents safety, logistical and feasibility questions, especially when operating within a community that has a higher incidence of frailty and immunodeficiency (Marquez et al., Reference Marquez, Chung, Marches, Rossi, Nehar-Belaid, Eroglu, Mellert, Kuchel, Banchereau and Ucar2020). Therefore, although preclinical FMT studies have shown promise at improving brain health during ageing (Boehme, Guzzetta, Bastiaanssen et al., Reference Boehme, Guzzetta, Bastiaanssen, van de Wouw, Moloney, Gual-Grau, Spichak, Olavarría-Ramírez, Fitzgerald, Morillas, Ritz, Jaggar, Cowan, Crispie, Donoso, Halitzki, Neto, Sichetti, Golubeva, Fitzgerald, Claesson, Cotter, O’Leary, Dinan and Cryan2021; Mossad et al., Reference Mossad, Nent, Woltemate, Folschweiller, Buescher, Schnepf, Erny, Staeheli, Bartos, Szalay, Stecher, Vital, Sauer, Lämmermann, Prinz and Blank2021; Parker et al., Reference Parker, Romano, Ansorge, Aboelnour, Le Gall, Savva, Pontifex, Telatin, Baker, Jones, Vauzour, Rudder, Blackshaw, Jeffery and Carding2022), it would be highly beneficial to develop alternative, less invasive and hazardous strategies to remodel the microbiome–gut–brain axis, which would allow physicians to move beyond faecal microbiota transplantation.
Antibiotics
Antibiotics are commonly administered to treat infections and antibiotic usage by elderly populations is rising substantially, making it critical to understand whether there are off-target negative or beneficial consequences to antibiotic usage in ageing. For instance, antibiotics can have off-target implications by harming beneficial microbes or resulting in the emergence of antibiotic resistance (Barbosa and Levy, Reference Barbosa and Levy2000). Alternatively, antibiotics that eradicate a harmful gut microbe may be able to improve brain health, though this is largely unexplored. Furthermore, several orally administered antibiotics can leak from the gut, allowing them to act directly on other systems including the brain regardless of the microbiota–gut–brain axis. This is of higher concern in disease states where barrier function is known to be compromised (Nau et al., Reference Nau, Sörgel and Eiffert2010).
Compelling preclinical evidence demonstrates that antibiotics may be therapeutic for neurodegeneration when administered to rodent models of AD, as discussed earlier. While clinical evidence is largely lacking, one pilot, open-label trial involving 10 patients with mild to moderate probable AD dementia found that treatment with rifaximin (a minimally absorbed antibiotic) for 3 months significantly reduced neurofilament-light levels in the serum and modestly improved other serum markers for disease severity, although no improvements to cognition were observed in this timeframe (Suhocki et al., Reference Suhocki, Ronald, Diehl, Murdoch and Doraiswamy2022). Interestingly, another study administering rifaximin to patients with cirrhosis and cognitive impairment found that rifaximin treatment improved working memory and inhibitory control, along with enhanced fronto-parietal and subcortical activation and connectivity (Ahluwalia et al., Reference Ahluwalia, Wade, Heuman, Hammeke, Sanyal, Sterling, Stravitz, Luketic, Siddiqui, Puri, Fuchs, Lennon, Kraft, Gilles, White, Noble and Bajaj2014).
Nonetheless, antibiotics may not be the best treatment approach for multiple reasons, including their potential for triggering harmful neurotoxic side effects. For instance, broad-spectrum antibiotics may deplete beneficial symbiotic gut bacteria, which may be playing critical support roles to the brain during ageing. Several case reports have linked the consumption of antibiotics and high plasma levels of antibiotics with the onset of delirium in the elderly (Moore and O’Keeffe, Reference Moore and O’Keeffe1999; Schliamser et al., Reference Schliamser, Cars and Norrby1991). Furthermore, one recent observational study involving over 14,000 women self-reporting their antibiotic use found associations between chronic antibiotic use during midlife with slightly reduced cognitive scores after a follow-up period of roughly 7 years (Mehta et al., Reference Mehta, Lochhead, Wang, Ma, Nguyen, Kochar, Huttenhower, Grodstein and Chan2022), although the type of antibiotic and the reason for chronic antibiotic use were not known. These few clinical publications implicating antibiotic use and cognitive function are also confounded by the requirement of antibiotics to treat a specific health condition, rather than solely investigating their impact on brain health.
Since it is unclear whether the harms of antibiotic use outweigh the potential benefits in individuals that are not actively battling a bacterial infection, and because antibiotic use supports the development of antibiotic-resistant bacteria, the potential for antibiotics as a therapeutic for ageing-associated neurodegenerative diseases may be limited. Therefore, the development of novel antibiotics, such as engineered phages that could specifically eliminate individual identified pathogenic bacteria, could become valuable in treating conditions wherein specific pathogenic gut microbes are identified.
Probiotics
It is increasingly recognised that microbiome-host modulation through probiotics is a promising avenue to improve cognitive function in human ageing (Eastwood et al., Reference Eastwood, Walton, Van Hemert, Williams and Lamport2021). Probiotics are defined as live microorganisms that, when administered in adequate amounts, confer a health benefit on the host (Hill et al., Reference Hill, Guarner, Reid, Gibson, Merenstein, Pot, Morelli, Canani, Flint, Salminen, Calder and Sanders2014). Recent work by researchers in Japan has shown evidence that probiotics improve cognition in elderly with mild cognitive impairment. In a randomised, double-blind, placebo-controlled trial, a group from Japan showed that a 4-month intervention with a Bifidobacterium breve strain could be an effective approach for improving memory functions of subjects with MCI showing improvements in various cognitive domains such as immediate, delayed and visuospatial memory (Xiao et al., Reference Xiao, Katsumata, Bernier, Ohno, Yamauchi, Odamaki, Yoshikawa, Ito and Kaneko2020). Previously the same group investigated the effects of this strain on cognitive function of older adults with a range of memory complaints, and found that, once the cohort was stratified, this probiotic induced beneficial effects only in a sub-population with more severe mild cognitive impairment (Kobayashi et al., Reference Kobayashi, Kuhara, Oki and Xiao2019). Intriguingly, new work from the group suggests that it may have an impact on age-related brain atrophy (Asaoka et al., Reference Asaoka, Xiao, Takeda, Yanagisawa, Yamazaki, Matsubara, Sugiyama, Endo, Higa, Kasanuki, Ichimiya, Koido, Ohno, Bernier, Katsumata, Nagahara, Arai, Ohkusa and Sato2022). In a Korean study, 12 weeks supplementation with Lactobacillus plantarum fermented soybean significantly improved a cognitive composite score which was predominantly driven by an improvement in attention in MCI subjects (Hwang et al., Reference Hwang, Park, Paik, Chae, Kim, Jeong, Ha, Kim, Hong, Park, Jung, Lee, Na, Kim and Chung2019). Compared to these, the first evidence in a Caucasian population showed efficacy in improving a cognitive composite score in elderly with MCI, with another strain in the Lactobacillus genus, Lactobacillus rhamnosus GG (Sanborn et al., Reference Sanborn, Azcarate-Peril, Updegraff, Manderino and Gunstad2020). Although these results are interesting, the design of the study demands caution. For example, the researchers were not blinded to the randomisation of the groups. The beneficial effects of L. rhamnosus GG could be mediated through immunomodulatory pathways (Hibberd et al., Reference Hibberd, Kleimola, Fiorino, Botelho, Haverkamp, Andreyeva, Poutsiaka, Fraser, Solano-Aguilar and Snydman2014; Schultz et al., Reference Schultz, Linde, Lehn, Zimmermann, Grossmann, Falk and Scholmerich2003), which have been linked to poor cognitive performance in elderly (Baune et al., Reference Baune, Ponath, Golledge, Varga, Arolt, Rothermundt and Berger2008; Trollor et al., Reference Trollor, Smith, Baune, Kochan, Campbell, Samaras, Crawford, Brodaty and Sachdev2010). In a small Japanese cohort, (Ueda et al. (Reference Ueda, Shinkai, Shiroma, Taniguchi, Tsuchida, Kariya, Kawahara, Kobayashi, Kohda, Ushida, Kitamura and Yamada2021) observed a reduced relative abundance of Faecalibacterium prausnitzii in elderly with MCI compared to healthy individuals. By isolating two F. prausnitzii strains from the healthy elderly group, the authors improved cognitive impairments in mice concomitant with changes in genes related to oxidative stress and mitochondrial function (Ueda et al., Reference Ueda, Shinkai, Shiroma, Taniguchi, Tsuchida, Kariya, Kawahara, Kobayashi, Kohda, Ushida, Kitamura and Yamada2021). Notably, one of the F. prausnitzii strains was given in a pasteurised form suggesting that beneficial effects are not only restricted to live bacteria, which directly exert effects on the host but could be also mediated through effects elicited by extracellular membrane components or could act immunomodulatory. Another next generation probiotic besides F. prausnitzii, A. muciniphila, showed a similar phenomenon in a small RCT in humans exerting its positive effects on some metabolic parameters predominantly in its pasteurised form (Depommier et al., Reference Depommier, Everard, Druart, Plovier, Van Hul, Vieira-Silva, Falony, Raes, Maiter, Delzenne, de Barsy, Loumaye, Hermans, Thissen, de Vos and Cani2019). While there is currently no direct clinical evidence that Akkermansia improves cognition in elderly people, the possibility that A. muciniphila influences cognition-linked metabolic factors, such as insulin sensitivity (Willmann et al., Reference Willmann, Brockmann, Wagner, Kullmann, Preissl, Schnauder, Maetzler, Gasser, Berg, Eschweiler, Metzger, Fallgatter, Häring, Fritsche and Heni2020), suggests that it may have the potential for improving cognitive health during ageing.
The efficacy of probiotics was also assessed in AD with mixed findings (Agahi et al., Reference Agahi, Hamidi, Daneshvar, Hamdieh, Soheili, Alinaghipour, Esmaeili Taba and Salami2018; Akbari et al., Reference Akbari, Asemi, Daneshvar Kakhaki, Bahmani, Kouchaki, Tamtaji, Hamidi and Salami2016). An Iranian study using a multispecies mixture containing different stains and species of the genera Lactobacillus and Bifidobacterium for 12 weeks, did not find a positive effect on cognition in patients with severe AD (Agahi et al., Reference Agahi, Hamidi, Daneshvar, Hamdieh, Soheili, Alinaghipour, Esmaeili Taba and Salami2018). It is plausible that probiotics may not exert positive effects on cognition when the damage is too advanced. Supplementation with a probiotic milk containing Lactobacillus acidophilus, Lactobacillus casei, Bifidobacterium bifidum and Lactobacillus fermentum another Iranian study showed improved insulin sensitivity, lower levels of the inflammatory marker CRP, and an improved cognitive score in the Mini-Mental State Examination (MMSE) after 12-weeks supplementation (Akbari et al., Reference Akbari, Asemi, Daneshvar Kakhaki, Bahmani, Kouchaki, Tamtaji, Hamidi and Salami2016). Furthermore, one study found that a probiotic mix containing L. acidophilus, B. bifidum and Bifidobacterium longum combined with selenium improved the MMSE score in patients with AD (Tamtaji et al., Reference Tamtaji, Heidari-Soureshjani, Mirhosseini, Kouchaki, Bahmani, Aghadavod, Tajabadi-Ebrahimi and Asemi2019), while another study in a Caucasian population did not find benefits on a cognitive score in AD with a multispecies probiotic consisting of L. casei W56, L. lactis W19, L. acidophilus W22, B. lactis W52, L. paracasei W20, L. plantarum W62, B. lactis W51, B. bifidum W23 and L. salivarius W24 (Leblhuber et al., Reference Leblhuber, Steiner, Schuetz, Fuchs and Gostner2018) possibly due to the shorter intervention time. The criteria for recruiting subjects across MCI and AD studies differ substantially. Similarly, the evaluation metrics for cognitive assessments also differ. Larger, well-controlled RCTs with harmonised inclusion and cognitive evaluation criteria, and longer interval follow-ups are needed to provide more reliable evidence (Xiang et al., Reference Xiang, Ji, Li, Cao, Xu, Tan and Tan2022).
Apart from interventions with classical lactic acid bacteria, studies utilising preclinical models showed that a combination of five Lactobacillus strains with five Enterococcus strains had beneficial effects on high-fat diet–induced disturbances of the microbiome, intestinal permeability, metabolism, immunity ultimately improving physical decline in older mice (Ahmadi et al., Reference Ahmadi, Wang, Nagpal, Wang, Jain, Razazan, Mishra, Zhu, Wang, Kavanagh and Yadav2020). Interestingly, a recent preclinical study suggests that Enterococcus faecalis plays a role in regulating social behaviour and social stress-induced stress response in mice (Wu et al., Reference Wu, Adame, Liou, Barlow, Lai, Sharon, Schretter, Needham, Wang, Tang, Ousey, Lin, Yao, Abdel-Haq, Beadle, Gradinaru, Ismagilov and Mazmanian2021b) though ageing was not considered in this paper.
Apart from studies examining older individuals with dementia or pre-dementia status, there is very limited evidence of the beneficial effects of probiotics on cognition in healthy older adults with memory complaints. Herein, probiotic-induced improvements have thus far been observed only under conditions where additional stressors were present (Eastwood et al., Reference Eastwood, Walton, Van Hemert, Williams and Lamport2021). For instance, one Korea-based study found that Lactobacillus helveticus IDCC3801 fermented milk improved cognitive functioning during cognitive fatigue tests (Chung et al., Reference Chung, Jin, Cui, Kim, Jung, Park, Jung, Choi and Chae2014). It seems that the cognitive status and accompanied underlying physiological changes could be predictive for the susceptibility of an individual to cognitive decline, although more studies are needed to understand the factors driving sensitivity to probiotic interventions.
Studies on the use of probiotics for PD are sparse. Pilot data in an open-label, single-arm, baseline-controlled trial suggests possible benefits on motor skills and quality of life with L. plantarum PS128 (Lu et al., Reference Lu, Chang, Weng, Chen, Kuo and Tsai2021). Further placebo-controlled studies are needed to support these initial results.
Certain clinical studies are looking into the prevention of neurodegenerative conditions through microbiome-targeted strategies utilising microbial metabolites such as SCFAs. Duscha et al. (Reference Duscha, Gisevius, Hirschberg, Yissachar, Stangl, Eilers, Bader, Haase, Kaisler, David, Schneider, Troisi, Zent, Hegelmaier, Dokalis, Gerstein, Del Mare-Roumani, Amidror, Staszewski, Poschmann, Stuhler, Hirche, Balogh, Kempa, Trager, Zaiss, Holm, Massa, Nielsen, Faissner, Lukas, Gatermann, Scholz, Przuntek, Prinz, Forslund, Winklhofer, Muller, Linker, Gold and Haghikia2020) have shown that two weeks of propionic acid intake leads to a sustained increase of immunoregulatory T (Treg) cells while three years of propionic acid intake positively influenced functional parameters through a reduced relapse rate associated with reduced brain atrophy. In addition, preclinical models have shown that modulation of neuroinflammation and cognitive decline can be achieved through oral supplementation with butyrate (Govindarajan et al., Reference Govindarajan, Agis-Balboa, Walter, Sananbenesi and Fischer2011; Matt et al., Reference Matt, Allen, Lawson, Mailing, Woods and Johnson2018), however, these findings have yet to be explored in humans. In contrast, a population-based cohort in France with over 9,000 community dwellers aged 65 years or more, found that increased propionic acid in serum was associated with increased odds of cognitive decline (Neuffer et al., Reference Neuffer, González-Domínguez, Lefèvre-Arbogast, Low, Driollet, Helmer, Du Preez, de Lucia, Ruigrok, Altendorfer, Aigner, Lucassen, Korosi, Thuret, Manach, Pallàs, Urpi-Sardà, Sánchez-Pla, Andres-Lacueva and Samieri2022). Mediation analyses suggested that this adverse association may be mediated through hypercholesterolemia and glycaemia with a strong correlation with a fasting blood glucose suggesting metabolic disruption as a possible pathway in relation of propionic acid to cognitive health (Neuffer et al., Reference Neuffer, González-Domínguez, Lefèvre-Arbogast, Low, Driollet, Helmer, Du Preez, de Lucia, Ruigrok, Altendorfer, Aigner, Lucassen, Korosi, Thuret, Manach, Pallàs, Urpi-Sardà, Sánchez-Pla, Andres-Lacueva and Samieri2022). Overall these data suggests that the effect of microbial metabolites are context-dependent where further research is needed to understand how underlying conditions can prevent or perturbate the effects of microbial metabolites.
Intriguingly, a recent study suggests that the bacteriophages group Caudovirales can improve executive function and memory in both preclinical models and in humans (Mayneris-Perxachs et al., Reference Mayneris-Perxachs, Castells-Nobau, Arnoriaga-Rodríguez, Garre-Olmo, Puig, Ramos, Martínez-Hernández, Burokas, Coll, Moreno-Navarrete, Zapata-Tona, Pedraza, Pérez-Brocal, Ramió-Torrentà, Ricart, Moya, Martínez-García, Maldonado and Fernández-Real2022). However, the impact of bacteriophages on cognitive health in the elderly remains underexplored. It is plausible that some of the apparent effects that bacteria have on the host may be in part mediated or influenced by bacteriophages (Teng et al., Reference Teng, Mu, Xu, Zhang, Sriwastva, Liu, Li, Lei, Sundaram, Hu, Zhang, Park, Hwang, Rouchka, Zhang, Yan, Merchant and Zhang2022).
Prebiotics
Clinical evidence for prebiotics, substrates that are selectively utilised by host microorganisms conferring a health benefit (Gibson et al., Reference Gibson, Hutkins, Sanders, Prescott, Reimer, Salminen, Scott, Stanton, Swanson, Cani, Verbeke and Reid2017), to improve brain health in ageing is limited. Despite this lack of research, there is some evidence that a prebiotic mix containing inulin and fructo-oligosaccharides can improve frailty, a risk factor for cognitive decline (Borges et al., Reference Borges, Canevelli, Cesari and Aprahamian2019), under certain conditions (Buigues et al., Reference Buigues, Fernandez-Garrido, Pruimboom, Hoogland, Navarro-Martinez, Martinez-Martinez, Verdejo, Mascaros, Peris and Cauli2016; Theou et al., Reference Theou, Jayanama, Fernandez-Garrido, Buigues, Pruimboom, Hoogland, Navarro-Martinez, Rockwood and Cauli2019) without showing a benefit on cognition (Buigues et al., Reference Buigues, Fernandez-Garrido, Pruimboom, Hoogland, Navarro-Martinez, Martinez-Martinez, Verdejo, Mascaros, Peris and Cauli2016). Preclinically, supplementation with inulin decreased neuroinflammation in brains of middle-aged mice (Boehme et al., Reference Boehme, van de Wouw, Bastiaanssen, Olavarría-Ramírez, Lyons, Fouhy, Golubeva, Moloney, Minuto, Sandhu, Scott, Clarke, Stanton, Dinan, Schellekens and Cryan2020), while inulin in combination with Enterococcus faecium (Romo-Araiza et al., Reference Romo-Araiza, Gutierrez-Salmean, Galvan, Hernandez-Frausto, Herrera-Lopez, Romo-Parra, Garcia-Contreras, Fernandez-Presas, Jasso-Chavez, Borlongan and Ibarra2018) showed similar effects in aged mice (Matt et al., Reference Matt, Allen, Lawson, Mailing, Woods and Johnson2018), and modulated brain ageing-related metabolites such as TMAO following chronic stress in the brains of aged mice (Cruz-Pereira et al., Reference Cruz-Pereira, Moloney, Bastiaanssen, Boscaini, Tofani, Borras-Bisa, van de Wouw, Fitzgerald, Dinan, Clarke and Cryan2022). Possible mechanisms may include microbial metabolite signalling-mediated modulation of peripheral and brain immune activation, modulation of brain metabolites, stimulation of gut hormones and neurotransmitter signalling (Boehme et al., Reference Boehme, van de Wouw, Bastiaanssen, Olavarría-Ramírez, Lyons, Fouhy, Golubeva, Moloney, Minuto, Sandhu, Scott, Clarke, Stanton, Dinan, Schellekens and Cryan2020; Matt et al., Reference Matt, Allen, Lawson, Mailing, Woods and Johnson2018; Stilling et al., Reference Stilling, van de Wouw, Clarke, Stanton, Dinan and Cryan2016; van de Wouw et al., Reference van de Wouw, Boehme, Dinan and Cryan2019). Moreover, the combination of the prebiotic fructo-oligosaccharide with multiple bacterial strains (L. paracasei, L. rhamnosus, L. acidophilus and B. lactis) mildly enhanced cognitive performance in healthy elderly (Louzada and Ribeiro, Reference Louzada and Ribeiro2020).
Diet
Novel dietary interventions modulating the gut microbiota composition has been proposed to combat age-related decline and improve physiological well-being (Cryan et al., Reference Cryan, Boehme and Dinan2019a). From epidemiological studies, compelling evidence suggests that Mediterranean diet can be beneficial for the ageing human brain (van de Rest et al., Reference van de Rest, Berendsen, Haveman-Nies and de Groot2015). Components of a Mediterranean diet such as Omega-3 polyunsaturated fatty acids (O3-PUFAs) or vitamin A have been shown to elicit protective effects on the ageing brain in preclinical models (Labrousse et al., Reference Labrousse, Nadjar, Joffre, Costes, Aubert, Gregoire, Bretillon and Laye2012; Touyarot et al., Reference Touyarot, Bonhomme, Roux, Alfos, Lafenetre, Richard, Higueret and Pallet2013). Also, polyphenols have been shown to positively influence cognition in preclinical and clinical settings (de Vries et al, Reference de Vries, Medawar, Korosi and Witte2021). Conversely, these nutrients have been shown to modulate the gut microbiota and can rescue microbiome-associated perturbations on brain health in preclinical models (Donoso et al., Reference Donoso, Egerton, Bastiaanssen, Fitzgerald, Gite, Fouhy, Ross, Stanton, Dinan and Cryan2020; Provensi et al., Reference Provensi, Schmidt, Boehme, Bastiaanssen, Rani, Costa, Busca, Fouhy, Strain, Stanton, Blandina, Izquierdo, Cryan and Passani2019). Work from the NU-AGE consortium shed light onto the relationship between Mediterranean diet-induced changes in the gut microbiota and their link to improved health in elderly, showing reduced frailty and improved health status associated with an intermediate microbiome response (Ghosh et al., Reference Ghosh, Rampelli, Jeffery, Santoro, Neto, Capri, Giampieri, Jennings, Candela, Turroni, Zoetendal, Hermes, Elodie, Meunier, Brugere, Pujos-Guillot, Berendsen, De Groot, Feskins, Kaluza, Pietruszka, Bielak, Comte, Maijo-Ferre, Nicoletti, De Vos, Fairweather-Tait, Cassidy, Brigidi, Franceschi and O’Toole2020). Adherence to the Mediterranean diet was linked to increased abundance in taxa which were positively associated with improved health outcomes such as decreased frailty and improved cognition, and negatively associated with inflammatory markers such as C-reactive protein and interleukin-17 (IL-17) suggesting that Mediterranean diet may have the potential to promote healthier ageing (Ghosh et al., Reference Ghosh, Rampelli, Jeffery, Santoro, Neto, Capri, Giampieri, Jennings, Candela, Turroni, Zoetendal, Hermes, Elodie, Meunier, Brugere, Pujos-Guillot, Berendsen, De Groot, Feskins, Kaluza, Pietruszka, Bielak, Comte, Maijo-Ferre, Nicoletti, De Vos, Fairweather-Tait, Cassidy, Brigidi, Franceschi and O’Toole2020). Accumulating preclinical and clinical research highlights IL-17 as a major cytokine involved in neurodegenerative processes and cognitive decline (Brigas et al., Reference Brigas, Ribeiro, Coelho, Gomes, Gomez-Murcia, Carvalho, Faivre, Costa-Pereira, Darrigues, de Almeida, Buee, Dunot, Marie, Pousinha, Blum, Silva-Santos, Lopes and Ribot2021; Cipollini et al., Reference Cipollini, Anrather, Orzi and Iadecola2019; Regen et al., Reference Regen, Isaac, Amorim, Nunez, Hauptmann, Shanmugavadivu, Klein, Sankowski, Mufazalov, Yogev, Huppert, Wanke, Witting, Grill, Galvez, Nikolaev, Blanfeld, Prinz, Schmitt-Kopplin, Strowig, Reinhardt, Prinz, Bopp, Becher, Ubeda and Waisman2021), suggesting that dietary strategies which decrease IL-17 could promote healthy ageing (Dupraz et al., Reference Dupraz, Magniez, Rolhion, Richard, Da Costa, Touch, Mayeur, Planchais, Agus, Danne, Michaudel, Spatz, Trottein, Langella, Sokol and Michel2021). Notably, elderly individuals who maintained high adherence showed improved global cognition and episodic memory after one year compared to low adherence (Marseglia et al., Reference Marseglia, Xu, Fratiglioni, Fabbri, Berendsen, Bialecka-Debek, Jennings, Gillings, Meunier, Caumon, Fairweather-Tait, Pietruszka, De Groot, Santoro and Franceschi2018) which were associated with alterations in their gut microbiome, suggesting that broad dietary intervention could be considered as a less invasive strategy than FMT for altering the gut microbiota towards improved cognition in elderly people (Ghosh et al., Reference Ghosh, Rampelli, Jeffery, Santoro, Neto, Capri, Giampieri, Jennings, Candela, Turroni, Zoetendal, Hermes, Elodie, Meunier, Brugere, Pujos-Guillot, Berendsen, De Groot, Feskins, Kaluza, Pietruszka, Bielak, Comte, Maijo-Ferre, Nicoletti, De Vos, Fairweather-Tait, Cassidy, Brigidi, Franceschi and O’Toole2020). Analysis of inferred microbial functions showed an increase in short and branch chained fatty acid production and lower production of secondary bile acids (Ghosh et al., Reference Ghosh, Rampelli, Jeffery, Santoro, Neto, Capri, Giampieri, Jennings, Candela, Turroni, Zoetendal, Hermes, Elodie, Meunier, Brugere, Pujos-Guillot, Berendsen, De Groot, Feskins, Kaluza, Pietruszka, Bielak, Comte, Maijo-Ferre, Nicoletti, De Vos, Fairweather-Tait, Cassidy, Brigidi, Franceschi and O’Toole2020). Of note, metabolic network analysis in clinical studies of AD points to a possible link of secondary bile acids with deterioration of cognition (Baloni et al., Reference Baloni, Funk, Yan, Yurkovich, Kueider-Paisley, Nho, Heinken, Jia, Mahmoudiandehkordi, Louie, Saykin, Arnold, Kastenmuller, Griffiths, Thiele, Alzheimer’s Disease Metabolomics, Kaddurah-Daouk and Price2020). In a large multicentre study, the authors report here an association between altered bile acid profile, genetic variants implicated in Alzheimer’s disease, and cognitive changes (MahmoudianDehkordi et al., Reference MahmoudianDehkordi, Arnold, Nho, Ahmad, Jia, Xie, Louie, Kueider-Paisley, Moseley, Thompson, St John Williams, Tenenbaum, Blach, Baillie, Han, Bhattacharyya, Toledo, Schafferer, Klein, Koal, Risacher, Kling, Motsinger-Reif, Rotroff, Jack, Hankemeier, Bennett, De Jager, Trojanowski, Shaw, Weiner, Doraiswamy, van Duijn, Saykin, Kastenmuller and Kaddurah-Daouk2019). Adherence to the Mediterranean diet has further shown to slow down the progression of AD (Berti et al., Reference Berti, Walters, Sterling, Quinn, Logue, Andrews, Matthews, Osorio, Pupi, Vallabhajosula, Isaacson, de Leon and Mosconi2018). The impact on dementia-type cognitive impairment has been also assessed by introducing a broader change in diet, which holds promise towards a more profound adjustment of the gut microbiome as opposed to the use of supplements (Del Bo et al., Reference Del Bo, Bernardi, Cherubini, Porrini, Gargari, Hidalgo-Liberona, Gonzalez-Dominguez, Zamora-Ros, Peron, Marino, Gigliotti, Winterbone, Kirkup, Kroon, Andres-Lacueva, Guglielmetti and Riso2021; Ghosh et al., Reference Ghosh, Shanahan and O’Toole2022a,Reference Ghosh, Shanahan and O’Tooleb; Nagpal et al., Reference Nagpal, Neth, Wang, Craft and Yadav2019). In a pilot study using a modified Mediterranean-ketogenic diet, the authors found changes in several taxa with distinct patterns between cognitively impaired versus cognitively normal subjects (Nagpal et al., Reference Nagpal, Neth, Wang, Craft and Yadav2019).
Vice versa, western-style diets, rich in saturated fat and high in sugar, may trigger cognitive dysfunction in ageing (Beilharz et al., Reference Beilharz, Maniam and Morris2015; Gardener et al., Reference Gardener, Rainey-Smith, Barnes, Sohrabi, Weinborn, Lim, Harrington, Taddei, Gu, Rembach, Szoeke, Ellis, Masters, Macaulay, Rowe, Ames, Keogh, Scarmeas and Martins2015; Wieckowska-Gacek et al., Reference Wieckowska-Gacek, Mietelska-Porowska, Wydrych and Wojda2021). A recent study shed more light into the role of the microbiota in this process showing a microbiota-dependent accumulation of the advanced glycation end product N6-carboxymethyllysine in microglia of aged mice linked to oxidative stress and mitochondrial dysfunction (Mossad et al., Reference Mossad, Batut, Yilmaz, Dokalis, Mezo, Nent, Nabavi, Mayer, Maron, Buescher, de Aguero, Szalay, Lammermann, Macpherson, Ganal-Vonarburg, Backofen, Erny, Prinz and Blank2022), risk factors for cognitive impairment. Furthermore, calorie restriction resulted in a lower level of amyloid plaque in a mouse model of AD (Cox et al., Reference Cox, Schafer, Sohn, Vincentini, Weiner, Ginsberg and Blaser2019). Intermittent fasting and caloric restriction emerged as a viable strategy to improve cognition not only in mouse models of ageing and Alzheimer’s disease and primates, but also in humans (Chakraborty et al., Reference Chakraborty, Banerjee, Mukherjee and Poddar2020; Dal-Pan et al., Reference Dal-Pan, Pifferi, Marchal, Picq, Aujard and Consortium2011; Ooi et al., Reference Ooi, Meramat, Rajab, Shahar, Ismail, Azam and Sharif2020; Pan et al., Reference Pan, Zhang, Wang, Wang, Li, Liao, Liao, Zhang, Liu, Song, Yu and Yuan2022; Witte et al., Reference Witte, Fobker, Gellner, Knecht and Floel2009). While the level and feasibility of caloric restriction in humans remains to be elucidated, intermittent fasting represents a possible strategy to improve cognitive health during ageing.
Conclusions and future perspectives
Research published over the last few years has demonstrated that the gut microbiota is a crucial contributor to (and potential target to improve) host brain ageing, including in neurodegenerative diseases, making this an exciting time for research within this scope. Indeed, microbiota-targeted interventions have shown promise for improving cognitive performance and overall brain health during ageing. While this field of research is very young and mechanistic evidence is entirely preclinical, clinical studies involving probiotics and dietary interventions that alter the gut microbiota have shown success at improving aspects of cognition in older people. Furthermore, clinical trials and case studies involving FMT demonstrate promise for improving symptoms of neurodegenerative diseases, including PD, AD and MS, giving hope to the future of medicine for the thousands of individuals living with these diseases who currently have limited treatment options. Nonetheless, this is a rapidly emerging field, and large-scale, well-controlled clinical studies are required to better characterise the potential for the gut microbiota as a treatment target within these diseases.
Ageing is a complex biological process, and individual’s age at different speeds and trajectories, which complicates defining healthy ageing, including how the gut microbiota should be modulated to support healthy ageing. Moreover, the impact of biological sex on ageing remains understudied, including how sex shapes age-related physiological changes in the microbiota-host dialogue, regardless of the increasing evidence that suggests biological sex contributes to the susceptibility for several age-related diseases. While the gut microbiota may become a diagnostic tool to better understand an individual’s biological age(ing) (Ratiner et al., Reference Ratiner, Abdeen, Goldenberg and Elinav2022), it may be necessary to tailor a microbiota-targeted intervention to an individual’s ageing process, considering individualised factors such as lifestyle, dietary habits and its existing resident gut microbiome. Therefore, there may not be a one-size-fits-all solution to improving brain ageing via the gut microbiota, and instead personalised solutions may be required. Increasing our understanding on ecological aspects in diet-microbiome interrelationship and its impact on the host are key going forward in designing efficacious nutritional interventions. This also includes an understanding on how the resident gut microbiome may impact the efficacy of nutritional solutions on host outcomes (Maldonado-Gomez et al., Reference Maldonado-Gomez, Martinez, Bottacini, O’Callaghan, Ventura, van Sinderen, Hillmann, Vangay, Knights, Hutkins and Walter2016; Shepherd et al., Reference Shepherd, DeLoache, Pruss, Whitaker and Sonnenburg2018), taking into account factors such as geographical, genetic and lifestyle differences across populations (Deschasaux et al., Reference Deschasaux, Bouter, Prodan, Levin, Groen, Herrema, Tremaroli, Bakker, Attaye, Pinto-Sietsma, van Raalte, Snijder, Nicolaou, Peters, Zwinderman, Bäckhed and Nieuwdorp2018).
A dominant, lingering question is the definition of a healthy gut microbiome, and whether a universal microbiome indicative of health during ageing indeed exists (Hill, Reference Hill2020; Shanahan et al., Reference Shanahan, Ghosh and O’Toole2021). Technological advances including high-resolution next-generation sequencing, metabolomics, transcriptomics, proteomics and machine learning and our knowledge of microbial ecology will drive forward our understanding of the microbiota–gut–brain axis in healthy brain ageing. Further well-designed randomised-controlled clinical studies, mechanistic investigations and large-scale population studies must be conducted to better understand the role of microbiota-targeted therapeutics for brain health during ageing. Elucidating the factors which drive individualised responses and outcomes are key to designing personalised microbiome-targeted interventions to improve physiology and brain function in ageing.
Disclosure statement
The contributor Marcus Boehme is an employee of Société des Produits Nestlé S.A. Katherine E. Guzzetta, Caroline Wasén and Laura M. Cox declare that they have no competing financial interests. The publication did not receive any funding from Société des Produits Nestlé S.A.
Research transparency and reproducibility
Links to the references that are highlighted in this review are available from the corresponding author.
Author contributions
M.B. and K.E.G. contributed equally to this manuscript and thus share co-first authorship. Therefore, the position of M.B. and K.E.G. in the list of authors is interchangeable. For the purpose of this manuscript, the written authorship order was determined by a single game of Viking Chess. Conceptualization: M.B., K.E.G.; Investigation: M.B., K.E.G., C.W., L.M.C.; Project administration: M.B.; Visualization: K.E.G., M.B.; Writing—original draft: M.B., K.E.G., C.W., L.M.C.; Writing—review and editing: M.B., K.E.G., C.W., L.M.C.
Funding
This work was supported in part by the BrightFocus Foundation, the CureALZ Foundation, and the National Institutes of Aging (NIA)/National Institutes of Health (NIH) grants R01-AG076008 and RF1-AG065270, as well as the Swedish Research Council, grant number 2020-00592.