Introduction
Introduced mammalian predators are responsible for the decline and extinction of a disproportionately large quantity of native fauna relative to other introduced guilds and taxa (Doherty et al. Reference Doherty, Dickman, Nimmo and Ritchie2015). Great effort has gone into monitoring and reducing introduced predators via fencing, culling and poisoning, at an estimated global annual cost of billions of dollars (Courchamp et al. Reference Courchamp, Chapuis and Pascal2003, Doherty et al. Reference Doherty, Dickman, Nimmo and Ritchie2015). Recent decades have seen an increase in the successful control and eradication of invasive mammals (Courchamp et al. Reference Courchamp, Chapuis and Pascal2003, Jones et al. Reference Jones, Holmes, Butchart, Tershy, Kappes and Corkery2016) and in the documentation of post-eradication benefits for native biodiversity and trophic cascades (Doherty et al. Reference Doherty, Glen, Nimmo, Ritchie and Dickman2016).
Rats (genus Rattus, particularly R. rattus, R. norvegicus and R. exulans) are among the most widespread and damaging invasive species, particularly on islands, of which only an estimated 10% remain rat-free (Towns et al. Reference Towns, Atkinson and Daugherty2006). Numerous case studies from island systems have demonstrated the negative impacts of rats, as well as the recovery of prey species following their eradication (VanderWerf Reference VanderWerf2001, Fukami et al. Reference Fukami, Wardle, Bellingham, Mulder, Towns and Yeates2006, Heath et al. Reference Heath, Kershner, Cooper, Lynn, Turner and Warnock2008, Mulder et al. Reference Mulder, Grant-Hoffman, Towns, Bellingham, Wardle and Durrett2009, Auld et al. Reference Auld, Hutton, Ooi and Denham2010, Pender et al. Reference Pender, Shiels, Bialic-Murphy and Mosher2013, Shiels et al. Reference Shiels, Pitt, Sugihara and Witmer2014, Russell & Holmes Reference Russell and Holmes2015, Tabak et al. Reference Tabak, Poncet, Passfield, Goheen and del Rio2015). Black rats (alternatively, ship or roof rats; R. rattus L.) are particularly damaging as they have been the primary driver of presumed extinctions of at least 60 species worldwide (Towns et al. Reference Towns, Atkinson and Daugherty2006). The black rat poses significant threats in forested ecosystems because it is an adept tree climber and generalist consumer of fruits, seeds, arthropods, bird eggs and nestlings and carrion (Norman Reference Norman1970, Cole et al. Reference Cole, Loope, Medeiros, Howe and Anderson2000, Fukami et al. Reference Fukami, Wardle, Bellingham, Mulder, Towns and Yeates2006, Matsui et al. Reference Matsui, Hisaka and Takagi2010, VanderWerf et al. Reference VanderWerf, Mosher, Burt, Taylor, Sailer, Veitch, Clout and Towns2011, Shiels et al. Reference Shiels, Flores, Khamsing, Krushelnycky, Mosher and Drake2013, Shiels et al. Reference Shiels, Pitt, Sugihara and Witmer2014). Black rats consume bird nest contents directly and also shift bird demography, foraging behaviour and spatial habitat use (Knowlton et al. Reference Knowlton, Flaspohler, Paxton, Fukami, Giardina, Gruner and Wilson Rankin2017, Wilson Rankin et al. Reference Wilson Rankin, Knowlton, Gruner, Flaspohler, Giardina and Leopold2018). Members of the Rattus genus can also exacerbate the negative impacts of other invasive species by dispersing non-native seeds or propagules (Shiels Reference Shiels2011), as vectoring pathogens (Meerburg et al. Reference Meerburg, Singleton and Kijlstra2009, Smith & Banks Reference Smith and Banks2014) and by serving as alternative prey for higher-level predators (Shiels et al. Reference Shiels, Pitt, Sugihara and Witmer2014).
Widespread eradication efforts targeting rats and other invasive mammalian predators on islands are represented by hundreds of successful cases of recovery of native flora and fauna (Jones et al. Reference Jones, Holmes, Butchart, Tershy, Kappes and Corkery2016, Newton et al. Reference Newton, McKown, Wolf, Gellerman, Coonan and Richards2016, Duron et al. Reference Duron, Shiels and Vidal2017, Wolf et al. Reference Wolf, Young, Zilliacus, Wegmann, McKown and Holmes2018), including knock-on secondary extinctions of other invasive species (Lafferty et al. Reference Lafferty, McLaughlin, Gruner, Bogar, Bui and Childress2018). Key to many successful eradications is the use of aerially broadcast poison baits, formulated with anticoagulants such as diphacinone or brodifacoum, to cover large, spatially isolated areas in short time intervals (Keitt et al. Reference Keitt, Griffiths, Boudjelas, Broome, Cranwell and Millett2015). However, among those successes are problematic failures with non-target effects (e.g., Buckelew et al. Reference Buckelew, Byrd, Howald, MacLean, Sheppard, Veitch, Clout and Towns2011) or the spread of toxicants through food webs (Pitt et al. Reference Pitt, Berentsen, Shiels, Volker, Eisemann, Wegmann and Howald2015). Snap-trapping avoids some of the negatives associated with poison baiting, namely resistance to anticoagulent rodenticides, which has been documented in R. rattus (Tanaka et al. Reference Tanaka, Kawai, Ikenaka, Harunari, Tanikawa and Ando2012), R. norvegicus (Cowan et al. Reference Cowan, Gleeson, Howitt, Ramón-Laca, Esther and Pelz2017) and Mus musculus (Siddiqi Reference Siddiqi1982, Marquez et al. Reference Marquez, Khalil, Fourel, Ovarbury, Pinot and Rosine2019). For these reasons, toxicant bait broadcast techniques may be logistically and politically unfeasible in some areas (Duron et al. Reference Duron, Shiels and Vidal2017), necessitating strategic development and testing of alternative techniques, such as high-density snap trap grids.
Although rat removal has received a lot of attention in a conservation context, major gaps in our knowledge exist with regards to where rats forage in invaded habitats and whether snap-trapping efficacy scales across a gradient of habitat sizes. Here, we demonstrate the effectiveness of snap traps, continuously maintained over four years, in localized Rattus removal from forest fragments on the Island of Hawai‘i and assess the non-target impacts of trapping. By combining snap traps and inked tracking cards placed at three different heights from ground to mid-canopy, we describe how rats utilize forest fragments with regards to distance from edge, overall forest fragment size and vertically into the canopy.
Methods
Study area
Trapping and survey sites were located in 34 kīpuka (forest fragments) on the windward north-east slope of Mauna Loa Volcano within the Upper Waiakea Forest Reserve on the Island of Hawai‘i (˜19.6°N, –155.3°E). Focal kīpuka, isolated by a matrix of lava resulting from historical basaltic lava flows (1852–1881), ranged in size from 0.1 to 12 ha. Distributed from 1500 to 1800 m in elevation, the study area receives moderate levels of orographic precipitation (˜2500 mm per year; Giambelluca et al. Reference Giambelluca, Chen, Frazier, Price, Chen and Chu2013), with mean annual temperatures at the centre of this study system of 12.5°C (Giambelluca et al. Reference Giambelluca, Shuai, Barnes, Alliss, Longman and Miura2014). Similar to contiguous Hawaiian mesic forests, the kīpuka canopies are compositionally simple and dominated by mature stands of Hawaiian endemics including Metrosideros polymorpha Gaudichaud-Beaupré (‘ōhi‘a lehua) and Acacia koa Gray, although forest height increases and gap density decreases with increasing fragment size (Vaughn et al. Reference Vaughn, Asner and Giardina2014, Reference Vaughn, Asner and Giardina2015). The kīpuka are separated by a relatively homogeneous lava matrix, which may serve as a partial barrier to rat movement among kīpuka. Early successional matrix habitat, on a mixture of ‘a‘ā and pāhoehoe lava substrates (Macdonald et al. Reference Macdonald, Abbott and Peterson1983), features scattered, short-statured ‘ōhi‘a along with shrubs, ferns and sedges (Raich et al. Reference Raich, Russell and Vitousek1997). Both native and non-native animals inhabit these forests and use the matrix habitat (Gruner Reference Gruner2004), including birds endemic to Hawai‘i that have been eliminated from lower elevations (<1450 m) due to mosquito-vectored avian pox and malaria (Vanriper et al. Reference Vanriper, Vanriper, Goff and Laird1986, Flaspohler et al. Reference Flaspohler, Giardina, Asner, Hart, Price, Lyons and Castaneda2010, Samuel et al. Reference Samuel, Woodworth, Atkinson, Hart and LaPointe2015). Hawai‘i has only one native terrestrial mammal, the Hawaiian hoary bat (Aeorestes semotus (H. Allen)); thus, the mammalian predators present in the study system are all non-native, including the Polynesian rat (Rattus exulans (Peale)), house mouse (Mus musculus L.), Javan mongoose (Herpestes javanicus (Saint-Hilaire)) and the pervasive black rat (Rattus rattus).
Rodent trapping
We selected 16 of 34 kīpuka for rat removal, with each removal kīpuka paired with a similarly sized control kīpuka and spatially positioned to ensure that they were at least 500 m away from any untreated kīpuka (Supplementary Fig. S1, available online; Wilson Rankin et al. Reference Wilson Rankin, Knowlton, Gruner, Flaspohler, Giardina and Leopold2018, their fig. 1(b)). In each removal kīpuka, Victor M326 Pro Rat snap traps were deployed in corrugated plastic boxes with a rat-sized opening on one side (Stanford IACUC, no. 1776; Fig. S2(a) & S2(b)). Covered traps force rats to approach the traps directly, which promotes a quick kill, while the small opening reduces non-target captures. These trap boxes were distributed in a 25 m × 25 m grid system with additional traps every 12.5 m around the forest fragment perimeters (Pender et al. Reference Pender, Shiels, Bialic-Murphy and Mosher2013). Prior to the first setting of traps, each trap was pre-baited with locally sourced fresh coconut 6 and 3 days prior to setting the traps. During initial trapping in June 2011, traps were set and checked daily. After the first week of trapping, take from traps decreased substantially. Episodic control efforts in continuous forest tracts on Hawai’i Island, even in combination with diphacinone bait stations, may sustain high recolonization rates between trapping periods (Nelson et al. Reference Nelson, Woodworth, Fancy, Lindsey and Tweed2002). Thus, after the first week, traps were subsequently checked and maintained every 2 weeks from July 2011 to May 2015. Trap baits were cycled between three consecutive trapping periods with commercially available peanut butter and then one trapping period with locally sourced fresh coconut.
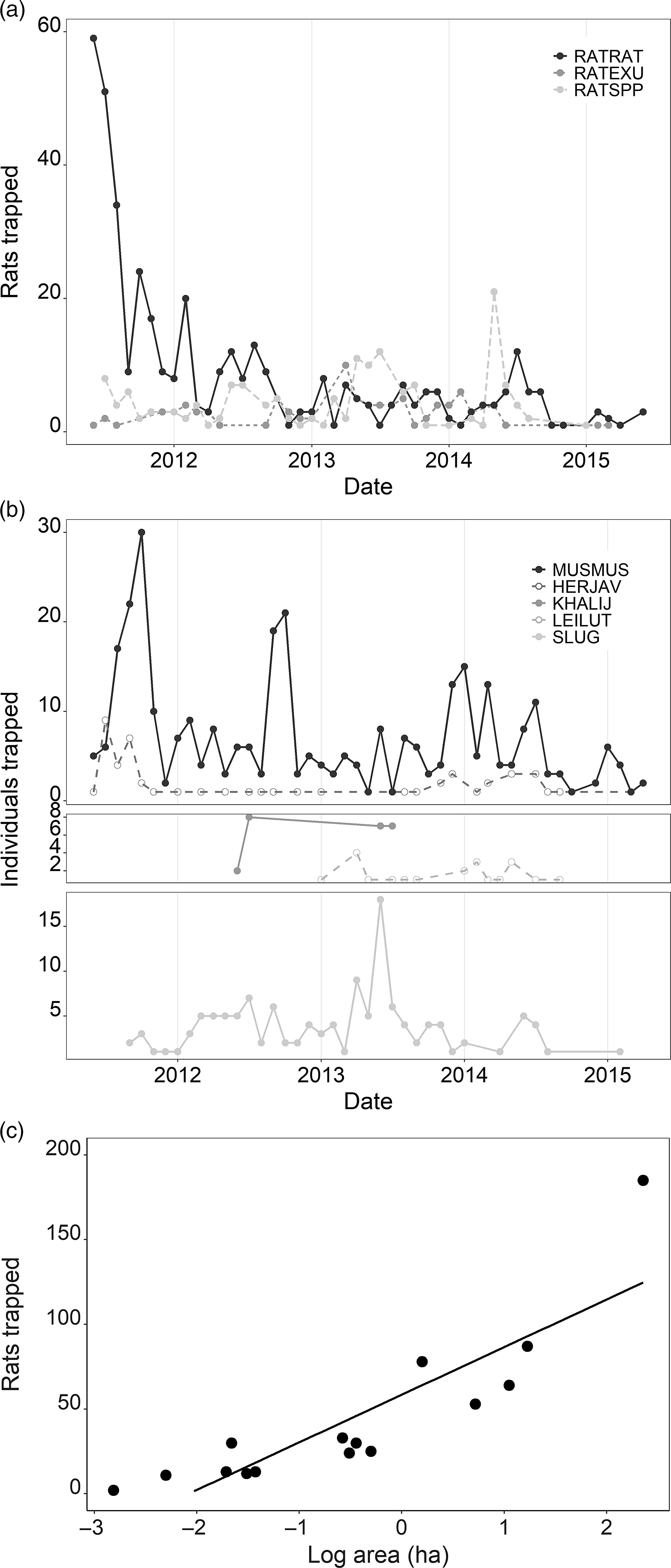
Fig. 1. Take from snap traps from July 2011 through May 2015 from 16 kīpuka. (a) Trapped Rattus individuals summed by species and month over time. (b) Non-target taxa trapped summed by species and month over time. (c) Relationship between total trapped Rattus individuals over entire trapping period in each kīpuka and log-transformed kīpuka area. Grey line indicates line of best fit. HERJAV = Herpestes javanicus; KHALIJ = Lophura leucomelanos; LEILUT = Leiothrix lutea; MUSMUS = Mus musculus; RATEXU = Rattus exulans (Polynesian rat); RATRAT = Rattus rattus (black rat); RATSPP = Rattus species; SLUG = undetermined slug species.
During initial daily trapping (June 2011), catch species in each trap was recorded on site and traps were immediately rebaited and set. Baits were always changed when traps were checked, regardless of whether there was a capture. Trapped Rattus spp. were taken back to the US Department of Agriculture (USDA) Forest Service’s Institute for Pacific Island Forestry (Hilo, HI) for detailed analysis. We identified each collected specimen to the species level where possible, assessed sex and measured body mass, body length and tail length. After processing, carcasses were disposed of as hazardous waste.
During biweekly trapping (July 2011–May 2015), the sex and body length of the rat carcasses were recorded and tissue samples were collected for genetic work, but no additional dissections were conducted. In instances where posthumous predation or advanced decay occurred, specimens were identified to the narrowest taxonomic distinction possible. We recorded any non-target taxa caught in snap traps, including M. musculus, H. javanicus, kalij pheasant (Lophura leucomelanos (Latham)), red-billed leiothrix (Leiothrix lutea (Scopoli)) and slugs (Veronicellidae). During biweekly trapping, all carcasses were buried on site. Snapped traps with no sign of capture, indicating an unintended triggering, an escaped animal or the remains being removed by a scavenger, were noted.
Vertebrate survey: tracking tunnels
To assess the efficacy of our rodent trapping methods, we monitored each kīpuka for the presence or absence of rats and other small vertebrates using tracking tunnels. This technique attracts animals to a baited tunnel where they step on an inked tracking card and then leave ink tracks on adjacent white ends of the card as they exit. In 2009, for kīpuka that were larger than 0.64 ha in area, two representative trees in each kīpuka were selected, with one located near the kīpuka centre and another on the perimeter. At each tree, standard Black Trakka™ tunnels (10 cm × 10 cm × 50 cm; Gotcha Traps Ltd, New Zealand) were deployed on the forest floor, at 6 m and at 12 m. This approach allowed us to track rodent presence at the forest floor and into the canopy. For smaller kīpuka (<0.64 ha), we used only one survey tree located in the kīpuka centre. Aboveground tunnels were attached to wooden platforms (‘artificial branches’) and secured to the tree with an L-bracket and aluminium nails (Fig. S2(c)). A loop of 7-strand nylon parachute cord passing through the tunnel and extending to the ground was attached to a plastic base plate that fit inside the tunnel (Fig. S2(d)). The aboveground tunnels were baited by attaching a fresh ink card to the base plate, with coconut bait wired to the centre, which could be raised and lowered from the ground by reeling in the parachute cord loop. Tunnels were coconut baited once prior to the initial snap-trapping and thereafter quarterly for 4 years concurrent with the trapping efforts. Cards were left during each baiting session for 1 week before retrieval and track identification (Fig. S2(e) & S2(f)). The tracks left on each card were identified and used to confirm the presence or absence of rats in each kīpuka, although we were unable to distinguish the tracks of R. rattus from those of R. exulans. In addition, we could identify non-target taxa such as M. musculus, H. javanicus and introduced skinks, Lampropholis delicata (De Vis), from these tracking cards. To assess the efficacy of our limited spatial sampling regime, we baited and deployed an additional, one-time 50-m × 50-m grid of ground-level tracking tunnels for 72 h in all kīpuka in summer 2012.
Statistical analysis
To assess changes in trapping rates, we calculated the total number of rats (Rattus spp.) trapped each month as the response in linear mixed models, where month–year (e.g., June 2011), rat species and log-transformed kīpuka area (hectares) were treated as fixed effects and kīpuka identity was treated as a random effect. To assess whether there was any change in the size of rats trapped, body size (body length or tail length) was the response, with sex added to the model framework above as a fixed effect. For by-catch taxa from snap-trapping, we calculated the total number of individuals trapped each month as the response, with the presence or absence of rats, month, by-catch species and log-transformed kīpuka area modelled as fixed effects and kīpuka identity treated as a random effect. We then analysed the tracking tunnel data in two separate analyses: (1) the quarterly tunnel assessments at ground level, 6 m and 12 m in the canopy (2011–2015); and (2) the grid tracking effort of 2012 (all at ground level). For each, we calculated the number of tracking tunnels with identifiable rat tracks divided by the total number of tracking tunnels in that kīpuka. Because the response variable represents the proportion of tracking tunnel cards with a positive result, we used a binomial error structure in generalized linear mixed models. For the quarterly tunnel assessments, rat removal treatment, kīpuka area, tunnel height and the interaction between treatment and tunnel height were fixed effects, kīpuka identity was a random effect and the model was weighted by the number of tracking tunnels available in each kīpuka. In the one-time grid tracking effort in 2012, we excluded tunnel height (as all grid tunnels were at ground level) and included bait type as an additional fixed effect because the attractiveness of two baits was tested (Spam Classic (Hormel Foods Corporation) versus fresh coconut). For by-catch species (e.g., mice, mongoose and skink), we conducted analyses on a combined dataset of regular sampling and the 2012 grid sampling, where proportion of tracking tunnel cards with a positive hit for a non-target species was the response variable using a binomial error distribution. We included non-target species identity, rat removal treatment, kīpuka area, tunnel height, bait, interaction between non-target species and treatment, interaction between non-target species and tunnel height and interaction between treatment and tunnel height as fixed effects. The dataset (grid only or quarterly trapping) was nested within kīpuka identity as a random effect, and the model was weighted by the number of tracking tunnels available in each kīpuka.
For the quarterly tunnel assessments, we also estimated whether pairs of species shared or avoided using the same tracking tunnel following the method of Wells et al. (Reference Wells, Pfeiffer, Lakim and Linsenmair2004). Calculations were done for seven pairs of species where the calculated number exceeded the critical value of the χ2 distribution for p < 0.05 (χ2 crit = 3.84), with species occurring at a minimum of 25 different tunnels. All model results are reported in the Supplementary Materials (Table S1). All analyses were conducted in R v. 3.5.0 (R Core Team 2021) and all means are reported ± SE. Mixed models were conducted using the lmer or glmer functions in package lme4 (Bates et al. Reference Bates, Maechler and Bolker2013). Where appropriate, post-hoc tests were conducted using the emmeans package, with false-discovery rate adjustments for multiple comparisons (Lenth Reference Lenth2019).
Results
Rodent trapping
Overall, we captured 660 rats, including 503 rats that we were able to identify to the species level. Black rats (R. rattus) represented 81.5% of the rats that were identifiable to the species level and 62.1% of all rats captured (n = 410). The less commonly trapped Polynesian rat (R. exulans) comprised 14.1% of all rats captured (n = 93). Overall, monthly averages of rats trapped significantly decreased over time (Fig. 1(a); F1,367 = 14.97, p = 0.0001), resulting in a 95% decrease over the course of the project. Similarly, non-target by-catch decreased over time (Fig. 1(b); F1,311 = 10.71, p = 0.001) regardless of species, although mice dominated the by-catch (327/423). By-catch in snap traps had a marginally significant positive correlation with the presence of rats (F1,316 = 3.43, p = 0.065). The number of rats trapped increased with kīpuka area (Fig. 1(c); F1,367 = 14.79, p = 0.0001). Both rat body size and tail length decreased over time (Fig. 2(a) & 2(b); body length: F1,446 = 9.66, p = 0.002; tail length: F1,446 = 74.36, p < 0.0001). In 2011, trapped R. rattus had a mean body length of 14.7 ± 0.2 cm, but this decreased to 12.6 ± 1.3 cm by 2015. The average tail length of trapped R. rattus followed a similar trend. In 2011, tails were an average of 17.9 ± 0.2 cm, but the mean was 14.6 ± 1.1 cm in 2015. Trapped R. exulans body size decreased by a similar magnitude from 2011 to 2014 (11.8 cm body and 12.5 cm tail, 9.9 cm body and 10.6 cm tail, respectively), but increased back to 2011 levels in 2015.

Fig. 2. Monthly mean Rattus (a) body length and (b) tail length in centimetres by species over time with standard error bars. RATEXU = Rattus exulans (Polynesian rat); RATRAT = Rattus rattus (black rat).
Tracking tunnels
For the quarterly checks, untreated kīpuka had a significantly higher mean proportion of tunnels with tracks of 0.51 ± 0.02 per week (n = 1336), while rat removal kīpuka had a mean proportion of 0.09 ± 0.01 per week (n = 1004; F1,30 = 26.25, p < 0.0001). The proportion of tracking cards with tracks per week varied with location of the tracking tunnel (F2,42 = 184.02, p < 0.0001), with the highest proportion located on the ground and the lowest proportion at 12 m (Fig. 3; ground: 0.53 ± 0.02, 6 m: 0.23 ± 0.02, 12 m: 0.12 ± 0.02). Additionally, there was a significant interaction between treatment and tunnel height (F2,43 = 7.76, p = 0.001). Rat tracks were more common in untreated kīpuka as compared to removal kīpuka at all tunnel heights (ground: χ2 = 113.31, p < 0.0001; 6 m: χ2 = 26.37, p < 0.0001), except at 12 m, where there was very low detection of rats in both treatments (χ2 = 0.91, p = 0.34). There was only a marginal effect of kīpuka size on the proportion of tracking tunnels with rat tracks (F1,30 = 4.2, p = 0.05) and no effect of time (F1,42 = 0.004, p = 0.95).
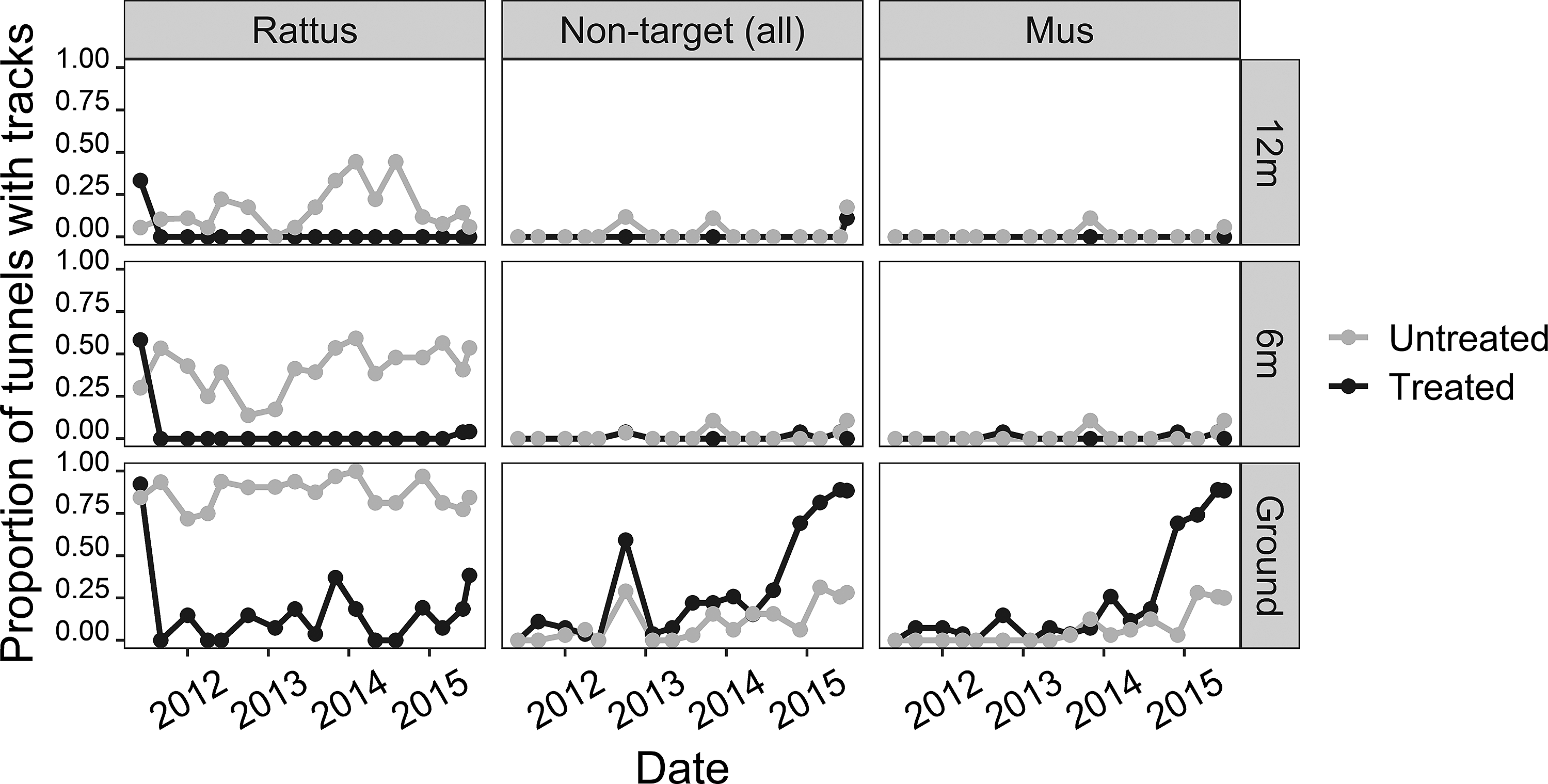
Fig. 3. Proportion of tracking tunnels with tracks over time in untreated and treated (rat removal) kīpuka (untreated: n = 1336, treated: n = 1004) for all Rattus, all non-target taxa and all Mus musculus.
When additional tracking tunnels were deployed weekly on ground-level grids in the summer of 2012, similar trends were observed. The proportion of tracking tunnels with rat tracks was significantly affected by treatment (untreated versus rat removal), tunnel location (grid versus perimeter) and bait type (coconut versus Spam). The mean proportion of tracking tunnels with rat tracks was higher in untreated kīpuka (0.46 ± 0.03) than rat removal kīpuka (0.081 ± 0.02; F1,31 = 35.27, p < 0.0001). The mean proportion of tracking tunnels with rat tracks was higher when Spam Classic was used as bait (0.51 ± 0.06) compared to fresh coconut (0.36 ± 0.05; F1,105 = 45.37, p < 0.0001). Overall, more grid tunnels were positive for rat tracks (0.49 ± 0.06) than perimeter tunnels (0.39 ± 0.05; F1,106 = 18.29, p < 0.0001). There was no significant effect of kīpuka size on proportion of ground-level tunnels visited by rats (F1,38 = 0.57, p = 0.46).
Effect of rats on other vertebrate taxa
In addition to Rattus spp., Javan mongoose, house mouse and skink were recorded on the tracking cards. To examine how these non-native taxa used the kīpuka, we reran analyses on a combined dataset of the monthly trapping and the summer 2012 grid. There was a significant treatment effect (Fig. 3; F1,24 = 30.90, p < 0.0001), with a higher proportion of non-rat hits to tracking tunnels in treated kīpuka as compared to kīpuka with rats. There were differences among the species as well (F2,715 = 68.03, p < 0.0001), where house mice were by far the most common visitors to the tracking tunnels, followed by both Javan mongooses and skinks. A similar pattern for the effect of tunnel height was detected (F2,671 = 32.96, p < 0.0001), with the ground tunnels having the highest proportion of hits (Fig. 3). Similarly to the rats, there was a preference for Spam Classic baits (F1,759 = 58.83, p < 0.0001); non-target taxa – mongoose and house mouse – were significantly more attracted to Spam Classic than fresh coconut baits (Table 1). There was a significant interaction between species and treatment (F2,715 = 4.94, p = 0.007), where there was no difference among the non-target taxa in the proportion of tunnels hit in untreated kīpuka, but mice hit a higher proportion of tunnels in treated kīpuka as compared to mongooses and skinks. We also detected an interaction between tunnel and species (F4,715 = 2.98, p = 0.019): at the ground level, mice were more common than mongooses and skinks. At all other heights, there were no species differences. Similarly, there was a treatment by tunnel height interaction (F2,669 = 6.60, p = 0.0015), where the most non-target taxa were recorded at ground level in treated kīpuka.
Table 1. Proportion of tracking tunnels with tracks for each recorded species in the summer 2012 grid based on bait type in post-hoc tests across species.

*p < 0.05, **p < 0.01, ***p < 0.001.
To gain additional insight into how these species overlapped, we then examined the co-occurrence of different species of vertebrates at a specific tracking card (Table 2). There were six species pairs with each species occurring at a minimum of 30 tunnels. There was significantly higher tunnel overlap between mongoose and house mouse than expected by chance alone (χ2 = 10.62, p = 0.0011). There was significantly lower tunnel overlap between R. rattus and mongoose than expected by chance (χ2 = 4.61, p = 0.032), as well as lower tunnel overlap between R. rattus and house mouse than expected (χ2 = 16.27, p < 0.0001).
Table 2. Number of tracking tunnels with tracks for each recorded species by treatment.

Discussion
Snap-trapping has a long history in the successful management of non-native, invasive predatory mammals (Shiels et al. Reference Shiels, Pitt, Sugihara and Witmer2014), with recent case studies showing sizable rat reductions (Armstrong et al. Reference Armstrong, Gorman, Pike, Kreigenhofer, McArthur and Govella2014, referenced in Simberloff et al. Reference Simberloff, Keitt, Will, Holmes, Pickett and Genovesi2019) or even short-term eradications of populations from forest fragments (King et al. Reference King, Innes, Gleeson, Fitzgerald, Winstanley and O’Brien2011). Here, we show that continuous control of rats over a 4-year period was effective at greatly reducing rat numbers in managed fragments. While colonists continued to be trapped over time, levels of colonization were low in comparison to other studies (e.g., King et al. Reference King, Innes, Gleeson, Fitzgerald, Winstanley and O’Brien2011, Pender et al. Reference Pender, Shiels, Bialic-Murphy and Mosher2013). The rocky, exposed habitat matrix of lava flows, which is less likely to sustain rat densities comparable to forests, may impose a partial barrier that lowers colonization rates in this study system. Comparisons across both removal and untreated kīpuka demonstrate that tracks of Rattus spp. were significantly more common on tracking cards within the grid compared to at the perimeter. This suggests that rats prefer the vegetated kīpuka to the lava matrix and are therefore probably using the associated edge habitats at reduced rates. Our findings are consistent with other studies of R. rattus that documented increased capture rates with increasing distance from the forest edge (Ruffell et al. Reference Ruffell, Didham, Barrett, Gorman, Pike and Hickey-Elliott2014) and showed that R. rattus spent most of its time under vegetation rather than in open habitats (Shiels Reference Shiels2010). In such environments where the habitats between fragments are inhospitable or less utilized, trapping efforts may be focused on fragment interiors.
The presence of rats in removal kīpuka, quantified by capture rate and tracking surveys, dropped to low levels after a single week of trapping. The continued trapping of intermittent individuals after this period was likely due to immigration by individuals from nearby patches. Innes et al. (Reference Innes, King, Bridgman, Fitzgerald, Arnold and Cox2010) found that rats recolonized similarly sized forest fragments (range 2–10 ha) within 33 days of eradication. Thus, we would expect low but steady arrival of immigrants to our removal kīpuka, which is consistent with our trapping data. This explanation is further supported by the greater incidence of rat tracks in the removal kīpuka on ground tracking tunnels than on tree tunnels. Moreover, body size metrics also decreased in removal kīpuka over the trapping period. Maturity and body length are often correlated in rats (Himsworth et al. Reference Himsworth, Jardine, Parsons, Feng and Patrick2014). One potential explanation for this trend is that resident adults were trapped first and younger remaining rats were trapped as they became more exploratory and moved into unoccupied territories in treated kīpuka. While this suggests that continuous trapping may be needed to prevent fragments from being recolonized, our adjacent, experimental untreated kīpuka were likely a source pool of these immigrants.
We used fresh coconut, commercial peanut butter and, for a portion of the study, Spam Classic as bait types, rotated to reduce the potential for trap shyness or acclimation. While Spam Classic was the most attractive bait for Rattus spp., it also attracted other predators such as H. javanicus and M. musculus. In Hawai‘i, these species are also introduced, and studies have demonstrated their negative impacts on the native flora and fauna (Hays & Conant Reference Hays and Conant2007, Angel et al. Reference Angel, Wanless and Cooper2009, Harris Reference Harris2009, St Clair Reference St Clair2011, Harper & Bunbury Reference Harper and Bunbury2015). In other locations where additional native mammals may be trapped, bait type should be considered as one way to limit non-target captures.
Omnivorous rats are often deeply embedded within invaded systems, and with their removal other invasive competitors or prey species may emerge upon ecological release from rats (Roemer et al. Reference Roemer, Donlan and Courchamp2002, Caut et al. Reference Caut, Casanovas, Virgos, Lozano, Witmer and Courchamp2007, Angel et al. Reference Angel, Wanless and Cooper2009, Mulder et al. Reference Mulder, Grant-Hoffman, Towns, Bellingham, Wardle and Durrett2009, Ruscoe et al. Reference Ruscoe, Ramsey, Pech, Sweetapple, Yockney and Barron2011, Shiels et al. Reference Shiels, Medeiros and von Allmen2017). Interestingly, we found that the proportion of tracking cards with non-target taxa was affected by rat removal treatments when we combined data for all species. Our data suggest that the presence of rats led to shifts in the foraging behaviours of mongooses and mice, which were both less likely to co-occur at the same tracking tunnel where rats would forage. Co-occurrence of mongooses and mice was significantly higher than expected by chance, which suggests that these two non-native species may fill the void left by black rats. While snap-trapping did result in the trapping of some non-target taxa, our data suggest that snap-trapping had limited effects on their populations (Fig. 1(b)). Additionally, our study sites may be particularly suitable for removal of Rattus spp. because the lava matrix is a poor reservoir habitat for mice and rats. Therefore, rats and mice may be more likely to compete for habitat in the kīpuka themselves (Caut et al. Reference Caut, Casanovas, Virgos, Lozano, Witmer and Courchamp2007). By contrast, in a recent study on the neighbouring island of Maui, Shiels et al. (Reference Shiels, Medeiros and von Allmen2017) observed that black rats dominated a restored native dry forest, while house mice prevailed over rats in the adjacent grassland. Thus, management strategies aimed at removing all invasive rodents may need to incorporate multiple methods to control both rat and mice populations.
Our study demonstrates that rats can be effectively controlled from partially isolated forested areas using snap traps. This trapping work was sustained by two full-time field technician positions visiting each of the 873 traps on a biweekly schedule. Though labour-intensive, snap-trapping can be a reasonable alternative in areas where long-term use of rodenticide is logistically impractical or unwanted (Shiels et al. Reference Shiels, Bogardus, Rohrer and Kawelo2019, Duron et al. Reference Duron, Cornulier, Vidal, Bourguet and Ruffino2020). Furthermore, on islands without native rodents the risk of by-catch in snap traps is low, whereas anticoagulants have the potential to contaminate food webs and impact other vertebrate species, scavenging species in particular (Shiels et al. Reference Shiels, Pitt, Sugihara and Witmer2014, Pitt et al. Reference Pitt, Berentsen, Shiels, Volker, Eisemann, Wegmann and Howald2015). In areas such as our study system, where full eradication may not be possible, rat control efforts must be implemented instead to protect vulnerable native species. Thus, we recommend snap-trapping as an option in such cases, either alone or in tandem with integrated programmes where toxicants can be delivered and carefully monitored as part of an integrated management strategy (Shiels et al. Reference Shiels, Pitt, Sugihara and Witmer2014, Keitt et al. Reference Keitt, Griffiths, Boudjelas, Broome, Cranwell and Millett2015).
Supplementary material
To view supplementary material for this article, please visit https://doi.org/10.1017/S0376892921000205.
Data availability
The dataset for this study has been uploaded to a public repository and will be available at https://doi.org/10.6086/D1PD72.
Acknowledgements
We thank the Hawai‘i Division of Forestry and Wildlife for permitting access to the research sites. The US Department of Agriculture (USDA) Animal and Plant Health Inspection Service and R. Sugihara provided essential support for the implementation and maintenance of rat removal treatments. We gratefully acknowledge the work of field technicians B Hwang, M Pina, C Morrison, L Nakagawa, J Pang-Ching, R De Mattos and K Tsuchiyama. We also thank Daniel Halford, Tess Morgridge, Liz Parissenti, Jenny Rempel, Jake Riley, Nessarose Schear, David Zimmerman and Amy Zuckerwise, supported by the Office of the Vice President for Undergraduate Education at Stanford University, for fieldwork and lab work assistance. We thank the USDA Forest Service Institute of Pacific Islands Forestry, which provided critical logistical and vehicle support and office and lab space.
Financial support
Funding was provided by the National Science Foundation (DEB-1020412 to T Fukami and CP Giardina; DEB-1020007 to DS Gruner; and DEB-1019928 to DJ Flaspohler) and the Terman Fellowship of Stanford University to T Fukami. The USDA Forest Service Institute of Pacific Islands Forestry provided funding support for the implementation and maintenance of the rat traps. Any use of trade, firm or product names is for descriptive purposes only and does not imply endorsement by the US Government.
Conflict of interests
None.
Ethical standards
This research was approved by the State of Hawai‘i Department of Land and Natural Resources Division of Forestry and Wildlife (Protected Wildlife permit no. WL13-02), the Michigan Technological University Institutional Animal Care and Use Committee (IACUC, no. 332879-4) and Stanford University IACUC (IACUC, no. 1776).