Introduction
Management of cognitive impairments in psychosis is an urgent issue [Reference Insel1]. Cognitive impairments in psychosis are strongly associated with disability and poor daily functioning, impede treatment adherence and response to psychosocial rehabilitation treatments, and are more enduring than key psychotic symptoms [Reference Green2]. However, present antipsychotics do not adequately treat cognitive deficits and potential pharmacological cognitive enhancers show modest favorable effects [Reference Sinkeviciute, Begemann, Prikken, Oranje, Johnsen and Lei3]. While cognitive remediation therapies have reported some positive outcome, the treatment effects are still low-to-moderate [Reference Wykes, Huddy, Cellard, McGurk and Czobor4], in part because individual treatment responses can be variable and is influenced by differing cognitive profiles, psychopathology, and illness course.
Given the impetus to look for better cognitive-enhancing interventions in psychosis [Reference Eack, Hogarty, Cho, Prasad, Greenwald and Hogarty5,Reference Ramsay, Fryer, Boos, Roach, Fisher and Loewy6], there is a need for better understanding about the neural substrates underlying variability in degree and trajectory of cognitive impairments. Individuals with psychosis can be stratified into three cognitive subtypes, based on the extent of cognitive decline from the expected level of cognitive functioning and the timing at which it occurs [Reference Weickert, Goldberg, Gold, Bigelow, Egan and Weinberger7–Reference Reichenberg, Harvey, Bowie, Mojtabai, Rabinowitz and Heaton9]. The “deteriorated” cognitive subtype presents with impaired current but normal premorbid cognitive performance, suggestive of progressive decline in cognition over the course of illness. The second “compromised” cognitive subtype exhibits both impaired current and premorbid cognitive performance, suggestive of compromised cognitive function early in life. A third “preserved” cognitive subtype presents relatively intact premorbid and current cognitive abilities compared with healthy individuals.
Although many studies have linked structural brain abnormalities to impaired cognitive performance in psychotic spectrum disorders [Reference Hartberg, Sundet, Rimol, Haukvik, Lange and Nesvag10–Reference Andreasen, Nopoulos, Magnotta, Pierson, Ziebell and Ho13], the underlying neurobiology of the three cognitive subtypes is still not entirely clear. To date, earlier studies which had compared brain structural measures among cognitive subtypes in psychotic conditions were mainly cross sectional in design and reported mixed findings [Reference Woodward and Heckers14–Reference Van Rheenen, Cropley, Zalesky, Bousman, Wells and Bruggemann16] in terms of the extent of underlying brain anomalies. To the best of our knowledge, none have specifically examined the use of identified putative brain measures to classify cognitive subtypes in an independent subject group.
Based on extant literature, the present study thus has a few aims and hypotheses. First, we aimed to compare cross sectional brain subcortical volumes and cortical thickness across the three cognitive subtypes and hypothesized that “deteriorated” cognitive subtype would have greater subcortical and cortical abnormalities compared with “preserved” cognitive subtype. Second, we aimed to study abnormal brain laterality measures as indices of neurodevelopmental aberrations and hypothesized that differences in laterality indices of specific brain structures are associated with “compromised” compared with “preserved” cognitive subtype. Third, we examined neuroprogressive brain changes over time and hypothesized that they are associated with “deteriorated” compared with “preserved” cognitive subtype. Finally, we tested the reliability of the classification of cognitive subtypes using the identified brain structures on an independent smaller group of subjects.
Materials and Methods
Participants
Two hundred twenty-five clinically stable outpatients with psychotic conditions (schizophrenia or schizoaffective disorder: n = 164; bipolar disorder with psychotic features: n = 46; and brief psychotic disorder: n = 15) were recruited from the Institute of Mental Health from 2010 to 2017. Their diagnoses were based on clinical interviews, medical records and verified by the Structured Clinical Interview for DSM-IV (patient version) by board certified psychiatrists in the hospital. One hundred thirty-nine healthy controls were recruited from the community, affiliated academic centers and through word-of-mouth concurrently and were screened using Structured Clinical Interview for DSM-IV (nonpatient version) to rule out any formal Axis I disorders. Controls with first-degree relatives with Axis 1 disorders were excluded.
Inclusionary criteria for all participants included an age range of 21–65 years and fluency in the English language (which is the main working language within the country and the primary medium of instruction in school). Exclusionary criteria included history of neurological or neurodevelopmental disorders including intellectual disability, substance, or alcohol dependence/abuse 6 months before the study or magnetic resonance imaging (MRI) contraindications. Of the 364 participants, 52 formed the independent subject group. Participants in the independent subject group (n = 52) were recruited using the same inclusion/exclusion criteria. All the study procedures were approved by the National Healthcare Group Institutional Review Board. Written informed consent was obtained from all subjects.
Cognitive subtyping and clinical measures
For cognitive subtyping, we adopted the approach in which the estimated premorbid intellect as well as the discrepancy between present level of cognitive functioning and the expected level of cognitive functioning as predicted by premorbid cognitive estimates were considered [Reference Woodward and Heckers14,Reference Czepielewski, Wang, Gama and Barch15]. We aligned the cut-off thresholds with previous studies in that subjects were considered “preserved” if (a) their estimated premorbid cognition were above the 10th percentile of healthy controls and (b) the difference between their present cognitive and premorbid cognitive abilities were less than 0.8 standard deviation (SD) [Reference Woodward and Heckers14,Reference Czepielewski, Wang, Gama and Barch15]. The remaining patients (i.e., those with an estimated premorbid cognition less than 10th percentile or the discrepancy between present cognitive and premorbid cognitive abilities were more than 0.80 SDs below their predicted level) were considered cognitively impaired. Cognitively impaired patients were stratified into “deteriorated” and “compromised” groups if their estimated premorbid cognitive abilities were above or below the 10th percentile of the healthy subjects’ distribution, respectively [Reference Woodward and Heckers14,Reference Czepielewski, Wang, Gama and Barch15].
The composite score from all the six subtests of the Brief Assessment of Cognition (BAC), standardized to normative data of 595 subjects from our local community [Reference Eng, Lam, Bong, Subramaniam, Bautista and Rapisarda17] was used to evaluate the present level of cognitive functioning. BAC evaluates multiple domains of verbal memory and learning, working memory, speed of processing, verbal fluency, and executive function [Reference Keefe, Goldberg, Harvey, Gold, Poe and Coughenour18]. For longitudinal assessments, an alternate form of BAC was administered to minimize practice effects.
The Wide Range Achievement Test—Reading Subtest III (WRAT-3) was used to appraise premorbid cognitive abilities [Reference Keefe, Eesley and Poe19]. We had tested several models with various predictors to obtain the best fit of expected level of cognitive functioning in healthy reference subjects. WRAT-3 together with age and gender were the strongest predictors of expected level of cognitive abilities. Symptom severity and psychosocial functioning were evaluated using the Positive and Negative Syndrome Scale (PANSS) and the Global Assessment of Functioning (GAF) respectively.
Image acquisition, processing, and quality control
Data from MRI structural scans and follow-up scans used to conduct the primary analyses were acquired on the same 3-T scanner (Philips Achieva (Eindhoven, The Netherlands)) at the National Neurological Institute, with no major hardware or software updates in between the follow-up duration. T1 weighted MPRAGE scans for a cohort of 315 individuals were collected using Philips Achieva scanner (180 axial slices of 0.9 mm thickness with no gap, (FOV)Field of View = 230 × 230 mm2; (TR)Repetition Time = 7.2 s, (TI)Inversion Time = 856 ms, (TE)Echo Time = 3.8 ms, (FA)Flip Angle = 8°, voxel size = 0.9 × 0.9 × 0.9 mm3). A three-step image quality control process was applied for images acquired from the initial 367 subjects (228 patients and 139 healthy controls). First, images were inspected for motion artifact at the time of acquisition and scanning was repeated if necessary. Second, independent researchers visually inspected the raw DICOM structural images of individuals scanned before preprocessing. Of the original data collected, two were excluded after failing visual inspection for motion artifacts (ringing and ghosting); another one subject was excluded due to abnormally enlarged and anomalous shaped ventricles. The three excluded were patients with schizophrenia. Third, after preprocessing, the images were subjected to another round of quality check using guidelines by the Enhancing NeuroImaging Genetics through Meta-Analysis (ENIGMA) Consortium (http://enigma.ini.usc.edu).
Image pre-processing was performed using FreeSurfer version 5.3 (http://surfer.nmr.mgh.harvard.edu). Additional specialized longitudinal processing to reduce within-subject morphological variability resulting from cross-sectional image processing biases/scanner-related measurements [Reference Reuter, Schmansky, Rosas and Fischl20] as detailed in our earlier paper [Reference Ho, Iglesias, Sum, Kuswanto, Sitoh and De Souza21] was performed prior to longitudinal analyses. Outcome measures extracted for subsequent analyses include global brain measures of intracranial volume (ICV), total brain volume (TBV), total gray matter, subcortical gray matter volume, total cortical white matter, and regional brain measures of subcortical region volumes and cortical thickness.
Statistical analysis
Analyses were performed using open-source R software version 3.3.0. Before hypotheses testing, quantile–quantile plots and Shapiro–Wilks tests of each outcome measure were performed to determine normal distribution. Plots of residuals versus fitted values were also performed after regression analysis to ensure homoscedasticity.
Analyses of demographics, cognitive, clinical and baseline global brain variables
Chi-squared or analysis-of-variance (ANOVA) tests were used to analyze demographics, cognitive and clinical variables, followed by post-hoc Tukey’s tests upon main effect of subtype. For global brain measures, an analysis-of-covariance (ANCOVA) was performed, adjusting for age and gender, followed by post-hoc Tukey’s tests. Another ANCOVA was performed with ICV as an additional covariate [Reference Woodward and Heckers14].
Cross-sectional analyses of regional outcome measures (subcortical volume, cortical thickness, and their laterality indices)
To examine the relationship of cognitive subtypes on the subcortical volume and cortical thickness in the 312 individuals, an ANCOVA was performed with cognitive subtype, age, gender, ICV, and years of education as independent variables, followed by Bonferroni correction for the multiple brain regions tested. Post-hoc tests were conducted using simultaneous inference in general parametric models, with Tukey’s test to correct for the 6 pairwise group comparisons. Cohen’s ƒ2 values (local effect size, i.e., effect size of an individual variable within a multivariate model) were also calculated for various predictors including cognitive subtype. To determine subtype differences in lateralization, similar ANCOVA analyses were performed, with laterality indices of subcortical volume and cortical thickness (Measureleft − Measureright)/(Measureleft + Measureright) as the dependent variables; cognitive subtype, age, gender, years of education, and handedness as independent variables.
Secondary sensitivity analysis
Because the effect of cognitive subtypes could confounded by variability in age and diagnosis, we sought to substantiate the findings by performing identical analyses on a more homogeneous subset of participants, that is limiting the age group of all subjects to 21–45 years of age and confining the patients to those with a primary diagnosis of schizophrenia. This particular cohort is comprised of 105 healthy controls and 109 patients (see Supplementary Table S3).
Longitudinal analyses
To examine whether the brain trajectories differed among subset of 101 individuals with follow-up data (see participant characteristics in Supplementary Table S1), a linear mixed-effects model described in our previous study [Reference Ho, Iglesias, Sum, Kuswanto, Sitoh and De Souza21] was fitted, fixed effects included the interaction between cognitive subtype and time (number of years between baseline and follow-up scan), cognitive subtype, time, age at baseline, gender, ICV, and years of education, random effects included individual intercept and slope. Significant testing was performed using ANOVA, followed by Bonferroni correction for the multiple brain regions tested. Post-hoc pair-wise group comparisons were performed using Wald tests to compute asymptotic Chi-squared statistics, with p-values adjusted for between-group multiple comparisons using the Holm–Bonferroni method.
A posteriori longitudinal brain-clinical/cognitive correlations
Consequent to the findings of significant volume trajectory abnormalities in a cognitive subtype, regression analysis described in our previous study [Reference Ho, Holt, Cheung, Iglesias, Goh and Wang22] was conducted to determine whether standardized rates of volume change (adjusted for ICV, baseline age, gender, antipsychotic dosage, and years of education) were associated with standardized rates of change in measures (adjusted for age, gender, antipsychotic dosage, and years of education) of cognition (BAC composite), symptoms (PANSS and GAF symptoms) and social/occupational functioning (GAF disability).
Classification of cognitive subtypes on an independent dataset using putative imaging markers
To explore whether putative discriminative variables, that is outcome measures that showed significant between-group differences in the main cross-sectional dataset could predict group classification in an independent sample cohort (see participant characteristics in Supplementary Table S2), linear discriminant analysis was performed, which separates the classes (cognitive subtypes) by maximizing the centers of the combined predictor data and minimizing the variation within each class of data [Reference Venables and Ripley23].
Results
Baseline sociodemographic, cognitive, and clinical characteristics
These baseline data including the cross-sectional comparisons are summarized in Table 1. Generally, the three cognitive subtypes did not significantly differ among and from the healthy controls in gender and handedness. “Deteriorated” and “compromised” cognitive subtypes received less years of education than healthy controls. While patient groups also did not differ in in antipsychotic dosage, severity of symptoms, or duration of illness, the “compromised” subjects were older and showed later onset of illness compared with other cognitive subtypes.
Table 1. Characteristics of cohort of 312 individuals with psychosis and healthy comparison individuals.

Abbreviations: A, ambidextrous; BD, bipolar disorder with history of psychosis; BAC, brief assessment of cognition; BPD, brief psychotic disorder; C, Chinese; CPZ, chlorpromazine equivalent daily doses; f, females; GM, gray matter; H, healthy control; I, Indian; ICV, intracranial volume; L, left; M, malay; m, Males; O, other ethnicities; R, right; SS, schizophrenia spectrum (i.e., schizophrenia, schizoaffective disorder and schizophreniform); PANSS, Positive and Negative Syndrome Scale; TBV, total brain volume; WM, white matter; WRAT3, Wide Range Achievement Test 3 (Reading subtest).
Measures of continuous variables are indicated by mean ± standard deviation. Post-hoc tests indicate
a C older than the H, P and D (p < 0.001).
b H received more years of education compared with all the patient subtypes (p < 0.001), and P received more years of education compared with D (p < 0.005) and C (p < 0.001).
c C performed worse than H, P, and D on WRAT3 (p < 0.001).
d H and P performed better than D and C subjects on all BAC subtests (p < 0.001). No significant group differences were found between D and C. H performed better than P in verbal memory, token motor task, verbal fluency, symbol coding, and composite scores (p < 0.01).
e C had a higher age onset of illness than P and D (p < 0.001). No significant group differences were found between P and D.
After adjusting for effects of age and gender, post-hoc Tukey’s tests indicate
f D showed lower TBV than H (p < 0.05).
g D and P showed lower total cortical GM than H (p < 0.05).
When ICV was adjusted for besides age and gender, post-hoc Tukey’s tests indicate
h P and D showed lower TBV than H (p < 0.05).
i P and D showed lower total cortical GM than H (p < 0.001).
j D showed lower total subcortical GM than H (p < 0.05).
k D showed lower total cortical WM than H (p < 0.01).
Global brain measures
There was significant cognitive subtype main effect on absolute TBV but not ICV. “Deteriorated” subjects had smaller absolute TBV than healthy controls. Subtype effects were also significant for all global measures after adjusting for ICV; relative to healthy controls, “deteriorated” and “preserved” cognitive subtypes showed lower TBV and total cortical gray matter volume, and “deteriorated” cognitive subtype showed lower total subcortical gray matter and total cortical white matter volume.
Regional brain measures
Table 2A shows significant subtype effects on limbic structures and lateral ventricles. Smaller bilateral hippocampal volume was found in “deteriorated” compared with healthy and “preserved” cognitive subtype, and “compromised” compared with healthy controls. Smaller left amygdala volume was seen in “compromised” compared with healthy and “preserved” cognitive subtype. Smaller thalamic volume and enlarged ventricles were found in “deteriorated” and “preserved” subtypes compared with healthy controls.
Table 2. Effects of cognitive subtypes on (a) volumes of subcortical regions and (b) thickness of cortical regions in the cohort of 312 subjects of patients with psychosis and healthy comparison controls.
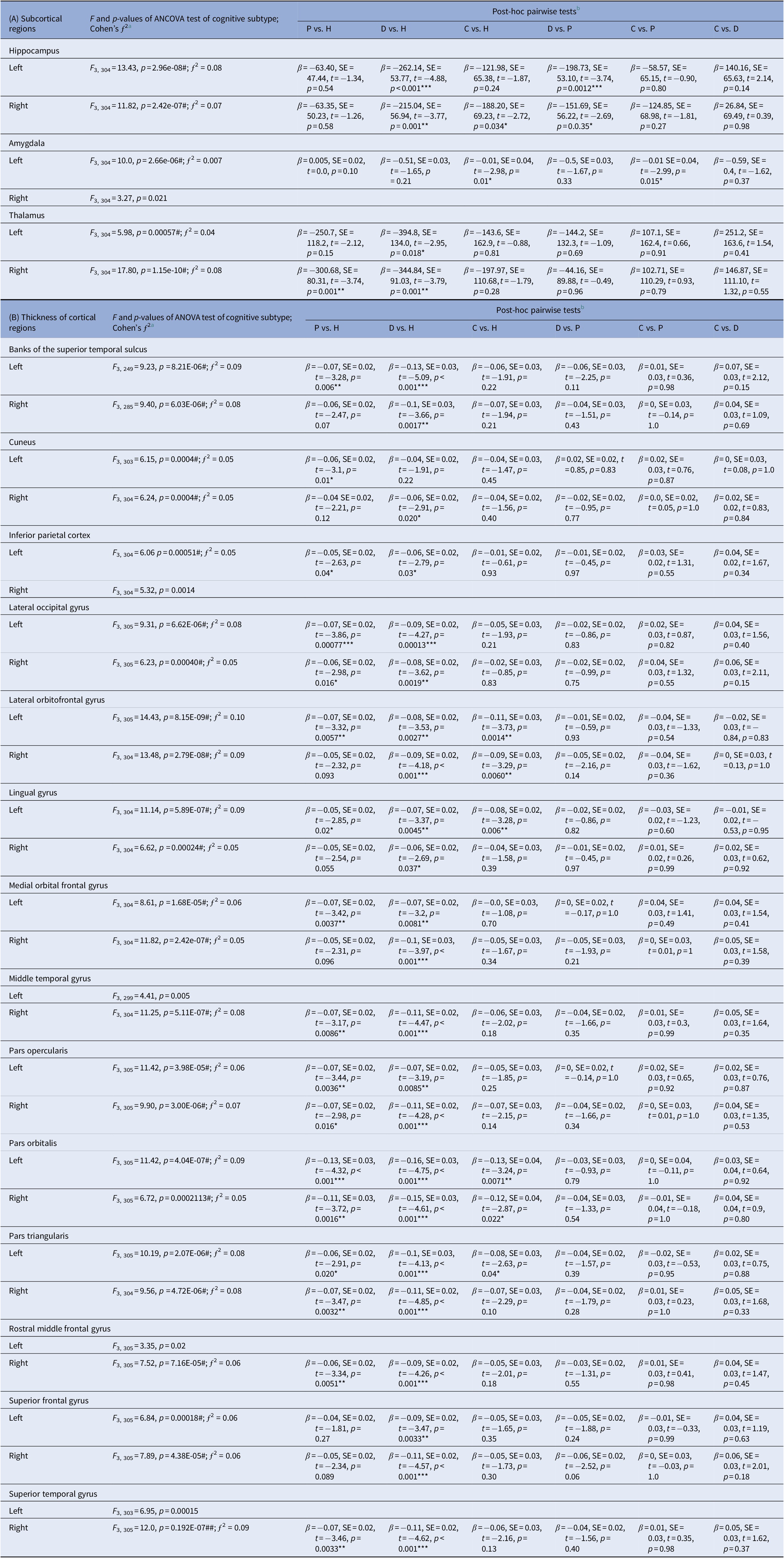
Abbreviations: β, beta coefficients; C, compromised; D, deteriorated; H, healthy; P, preserved; SE, standard error.
a ƒ 2 effect sizes of 0.02, 0.15, and 0.35 are considered small, medium, and large, respectively.
b Post-hoc tests, with multiple comparison adjusted p-values of * < 0.05, ** < 0.01, or *** < 0.001 shown.
Table 2B shows the effect of cognitive subtype on cortical thickness measures. Among the cognitive subtypes, “deteriorated” showed the most widespread cortical thinning across the four lobes relative to healthy controls. “Preserved” showed similar patterns of cortical thinning compared to “deteriorated” subtype within the prefrontal regions (pars orbitalis, pars opercularis, pars triangularis, rostral middle frontal gyrus, medial and lateral orbitofrontal gyri; temporal regions (middle and superior temporal gyri, banks of superior temporal sulcus), lateral occipital gyrus and inferior parietal cortex, but not in the lingual gyrus and superior frontal gyrus as seen in “deteriorated” cognitive subtype. “Compromised” subtype showed most limited cortical thining relative to healthy controls that was restricted to the lingual gyrus and lateral orbitofrontal gyrus.
Secondary analysis on the cohort more homogenous for age and diagnosis revealed similar pattern of findings as above, with strong local effect sizes for cognitive subtypes (Supplementary Table S4), suggesting that the cognitive subtypes contributed more to the variances in group-based volumes rather than diagnosis or age.
Lateralization measures
“Compromised” showed significantly increased rightward amygdalar lateralization when compared with healthy (p = 0.033) and “preserved” cognitive subtype (p = 0.021).
Longitudinal comparisons
The subtype-by-time interaction effect was significant only in the hippocampus, after adjusting for multiple comparisons. Figures 1A,B indicates that this effect was driven by progressive bilateral hippocampal volumetric decline in “deteriorated” compared with healthy (p = 0.00061 for left hippocampus; p = 0.00018 for right hippocampus) and “preserved” (p = 0.017 for left hippocampus; p = 0.036 for right hippocampus) cognitive subtype. No significant subtype-by-time effects on cortical thickness measures.

Figure 1. Hippocampal volume changes across the cognitive subtypes and healthy controls over time: a longitudinal snapshot. Spaghetti plots indicate progressive volume decline over time in the (A) left hippocampus and (B) right hippocampus in “deteriorated” cognitive subtype compared with “preserved” subtype and healthy individuals. (C) Within the “deteriorated” cognitive subtype, left hippocampal volume decline correlated with less improvements in cognitive composite scores (higher scales indicate better performance over time) and worse negative symptoms. Volume decline in both the left and right hippocampi also correlated with worse general psychopathology and psychosocial and occupational functioning. ***p < 0.001, *p < 0.05.
A posteriori longitudinal brain-behavioral correlations
In “deteriorated” individuals, progressive volume decline of hippocampus correlated with less improvement in the BACS composite score over time (left: p = 0.017; right not significant: p = 0.12), worse GAF disability scores i.e. psychosocial and occupational functioning (left: p = 0.035; right: p = 0.027) and greater severity of negative symptoms (left: p = 0.012; right not significant: p = 0.50), general psychopathology (left: p = 0.05; right: p = 0.036) but not positive symptoms (Figure 1C).
Cognitive subtype classification based on putative markers:
A composite index of the above putative neuroimaging measures associated with the cognitive subtypes (i.e., bilateral volumes of the hippocampus, lateral ventricles, thalamus, ICV to adjust for brain size) and amygdala laterality measure without demographic or clinical variables) classified an independent cohort of patients with schizophrenia with an accuracy of 90% for healthy controls, 83% for “preserved,” 73% for “deteriorated,” and 75% for “compromised” cognitive subtypes, with an overall accuracy of 71%.
Discussion
The current study found shared and distinct corticolimbic abnormalities among the cognitive subtypes. Cortical thinning, ventricular enlargements and limbic volume reductions were the most widespread in “deteriorated” cognitive subtype and were the most limited in “compromised” cognitive subtype compared with healthy controls. Lateralization of brain regions did not differ among the groups, apart from the amygdala, which showed more pronounced rightward lateralization in “compromised” compared with healthy controls and “preserved” cognitive subtype. Progressive shrinkage of the hippocampus was associated with “deteriorated” subtype compared with healthy controls and “preserved” subtype; the hippocampal shrinkage correlated with worsening cognitive and psychosocial functioning, and increasing severity of negative symptoms.
The nature and extent of brain structure abnormalities in our cross-sectional findings were consistent with some but not all prior findings. First, no group differences in ICV were found which was similar to findings of an earlier study [Reference Van Rheenen, Cropley, Zalesky, Bousman, Wells and Bruggemann16]. Second, we found that “deteriorated” cognitive subtype exhibited the largest extent of gray matter reductions in measures of total cortical gray matter and total subcortical gray matter when compared with healthy controls, in contrast with an earlier finding that “compromised” subtype exhibited the largest extent of gray matter reductions [Reference Czepielewski, Wang, Gama and Barch15,Reference Van Rheenen, Cropley, Zalesky, Bousman, Wells and Bruggemann16]. Third, relatively extensive brain tissue loss was observed in “preserved” cognitive subtype, which was in line with previous literature [Reference Woodward and Heckers14,Reference Van Rheenen, Cropley, Zalesky, Bousman, Wells and Bruggemann16,Reference Ortiz-Gil, Pomarol-Clotet, Salvador, Canales-Rodriguez, Sarro and Gomar24,Reference Wexler, Zhu, Bell, Nicholls, Fulbright and Gore25]. One possible explanation to account for the absence of direct relationship between brain pathology and clinical manifestation could be cognitive reserve [Reference Stern26]. Cognitive reserve is posited as an active process in psychosis, in which efficiency in neural networks and/or recruitment of alternative networks can compensate for the loss of brain tissue [Reference Barnett, Salmond, Jones and Sahakian27]. Education is a known neuroprotective factor contributing to cognitive reserve [Reference Barnett, Salmond, Jones and Sahakian27] and in this present study “preserved” received more years of education than “compromised” and “deteriorated.” Another possible explanation could be the effects of the type, duration of use and dosages of antipsychotics, which have been linked to brain tissue loss [Reference Andreasen, Nopoulos, Magnotta, Pierson, Ziebell and Ho13,Reference van Haren, Schnack, Cahn, van den Heuvel, Lepage and Collins28,Reference Sugihara, Oishi, Son, Kubota, Takahashi and Murai29]. Although not markedly significant, we note that “preserved” received relatively higher antipsychotic dose than the other groups. Conversely, the duration of untreated psychosis—found to differentially affect the general cognitive abilities of patients with low premorbid IQ and high premorbid IQ [Reference Wang, Ho, Sum, Collinson and Sim30]—may also exert differential effects on frontal and temporal regions of the brain [Reference Lappin, Morgan, Morgan, Hutchison, Chitnis and Suckling31,Reference Malla, Bodnar, Joober and Lepage32].
We noted generally an absence of group lateralization differences in regional subcortical and cortical thickness measures except the amygdala. “Compromised” showed increased rightward amygdala lateralization, which was driven by a differentially smaller left amygdala, compared with “preserved” and healthy controls. Longitudinally, this pattern of abnormal amygdala lateralization did not change. The amygdala continues to grow non-linearly throughout infancy into adolescence, reaching maximal volume at 9–11 years of age [Reference Uematsu, Matsui, Tanaka, Takahashi, Noguchi and Suzuki33], and has recently been shown to be an area of ongoing postnatal neurogenesis [Reference Jhaveri, Tedoldi, Hunt, Sullivan, Watts and Power34]. Different cognitive roles have been posited for the left and right amygdala, with the left being involved in deliberate evaluation and the right involved in subliminal and more rapid affective responses relevant in psychosis [Reference Costafreda, Brammer, David and Fu35,Reference Sergerie, Chochol and Armony36].
Besides laterality differences, “compromised” showed the most limited cortical thinning and ventricular enlargement among the subtypes. Brain atrophy was also relatively spared in “compromised.” This begets the question of why brain changes are more circumscribed in “compromised” than “preserved.” One explanation could be that the progression of brain changes in “compromised” are later in onset. Another explanation could be that “compromised” had taken the “first hit” in cognitive impairments earlier in life, hence subsequent onslaught of disease-related effects had affected this subtype to a lesser degree than the other two.
In contrast, the hippocampal shrinkage, and to a certain extent thalamic shrinkage, in “deteriorated” is suggestive of more dynamic and aggresive brain changes occurring in this subtype. The progressive loss of hippocampal volume is on top of the reduction in hippocampal volume in “deteriorated” when compared to healthy and “preserved” at baseline, the latter finding by itself consistent with a previous study, which though did not include comparisons with the “compromised” cognitive subtype [Reference Weinberg, Lenroot, Jacomb, Allen, Bruggemann and Wells37]. The observed relationship between the brain changes and cognitive performances are consistent with a large-scale study of schizophrenia spectrum conditions spanning over 18 years showing that only a subset of the patients showed progressive brain changes, and which correlated most closely with cognitive impairments [Reference Andreasen, Nopoulos, Magnotta, Pierson, Ziebell and Ho13]. Previously, we had also reported progressive hippocampal loss accompanying decline across all symptom domains in a schizophrenia cohort [Reference Ho, Iglesias, Sum, Kuswanto, Sitoh and De Souza21]. This additional subtyping of cognitive profile now revealed that the hippocampal shrinkage correlated with worsening negative symptoms and global psychopathology but not positive symptoms in “deteriorated” cognitive subtype. This could be attributed to the clinical overlap between cognitive dysfunction and phenomenology such as poverty of speech, deficits with abstract thinking and poor attention in negative syndrome of schizophrenia [Reference Harvey, Koren, Reichenberg and Bowie38]. Cognitive difficulties also affect daily functioning [Reference Green2], and may explain the observed association between hippocampal reduction in “deteriorated” subtype and declining psychosocial and occupational functioning.
We then used our main findings (namely limbic structures, lateral ventricular volumes, and amygdala laterality index) to predict the cognitive subtypes within a small independent cohort of subjects. The overall accuracy was 71% which suggest that further elucidation of specific brain substrates underlying cognitive subtypes in psychosis is warranted, which could be monitored for changes with clinical management (pharmacological or non-pharmacological) over time. A previous trial of a 2-year cognitive enhancement therapy reported left amygdala volume increase and greater left hippocampal volume preservation [Reference Eack, Hogarty, Cho, Prasad, Greenwald and Hogarty5]. Another trial of auditory cognitive training reported a correlation between thalamic volume change and cognitive improvements in the intervention group [Reference Ramsay, Fryer, Boos, Roach, Fisher and Loewy6] which highlights the need to account for brain measures associated with cognitive subtype in psychosis at baseline and longitudinally.
Our findings should be interpreted within the confines of several limitations. First, the cognitive profiling heavily relied on a cross-sectional tool of reading ability for assessing premorbid cognitive abilities. Second, the findings between cognitive subtypes and brain pathology are associational; the study design cannot whether the brain reductions already present in the different subtypes are because of stunted neurodevelopment, accelerated neurodegeneration, diminished neuroplasticity, or due to confounding effects of poor lifestyle choices or cumulative antipsychotic exposure. Also, our longitudinal analyses were based on a modest sample size. Future prospective studies may want to extend the age range starting from adolescence which could proffer further insights into brain structural changes underlying cognitive subtypes. Additional imaging modalities of structural or resting-state functional connectivity could potentially provide richer information on multimodal cerebral networks associated with cognitive subtypes.
In conclusion, our present findings pointed toward distinct extent and trajectory of corticolimbic structure abnormalities associated with cognitive subtypes in psychosis, which may allow better biological understanding of the course of cognitive functioning with treatment over time.
Supplementary Materials
To view supplementary material for this article, please visit http://dx.doi.org/10.1192/j.eurpsy.2020.36.
Acknowledgments.
This study is supported by the Singapore Ministry of Health’s National Medical Research Council under the Center Grant Program (Institute of Mental Health, Singapore) (NMRC/CG/004/2013), Open Fund Young Investigator Research Grant (NMRC/OFYIRG/0020/2016) (NFH) and National Healthcare Group SIG/15014 (NFH); SIG/05004, SIG/05028 (KS), the Singapore Bioimaging Consortium (RP C-009/2006) (KS); the Biomedical Research Council, Singapore (BMRC 04/1/36/372), the Agency for Science, Technology, and Research (A*STAR) and Duke-NUS Graduate Medical School Signature Research Program funded by Ministry of Health, Singapore (JZ).
Conflict of Interest
Dr. Keefe has received support from AbbVie, Acadia, Akebia, Akili, Alkermes, Astellas, Asubio, Avanir, AviNeuro/ ChemRar, Axovant, Biogen, BiolineRx, Biomarin, Boehringer-Ingelheim, Cerecor, CoMentis, FORUM, Global Medical Education, GW Pharmaceuticals, Intracellular Therapeutics, Janssen, Lundbeck, MedScape, Merck, Minerva Neurosciences Inc, Mitsubishi, Moscow Research Institute of Psychiatry, Neuralstem, Neuronix, Novartis, the New York State Office of Mental Health, Otsuka, Pfizer, Reviva, Roche, Sanofi, Shire, Sunovion, Takeda, Targacept, the University of Moscow, the University of Texas Southwest Medical Center, and WebMD. Dr. Keefe also receives royalties from the BACS testing battery. He is also a shareholder in NeuroCog Trials and Sengenix. All the other authors report no competing interests.
Comments
No Comments have been published for this article.