Introduction
An outbreak of novel coronavirus pneumonia (coronavirus disease 2019 (COVID-19)) occurred in Wuhan, China in December 2019, which presented an outbreak situation and has now caused a global epidemic. The disease is caused by a novel coronavirus (severe acute respiratory syndrome-coronavirus 2 (SARS-CoV2)), which belongs to the novel beta genus coronavirus and is mainly characterised by strong transmission and high pathogenicity [Reference Lancet1]. As of 27 March 2021, the World Health Organization (WHO) has received a total of more than 125 million confirmed cases, including over 2.7 million deaths. As indicated from the epidemic report, the epidemic is spreading and accelerating continuously, and all mankind faces great challenges [2].
For a long time, smoking has been generally accepted to display a close relationship to the poor prognosis of lung diseases. There is substantial evidence that smoking negatively affects lung health [Reference Vardavas3]. In addition, ex vivo and in vivo studies suggested that smoking is capable of elevating the risk of respiratory tract infection by causing chronic lung inflammation and destructive immune response [Reference Han4]. Moreover, it elevates the risk of heart disease, cancer and other diseases in smokers and surrounding people. However, the association between smoking and COVID-19 disease remains controversial. Lippi et al. [Reference Lippi5] did not identify any significant association between COVID-19 disease severity and disease development and smoking by conducting a meta-analysis on five studies; while the results of study conducted by Karanasos et al. [Reference Karanasos6] suggested that smoking may increase disease severity and mortality in hospitalised COVID-19 cases.
In addition, considerable clinical studies have shown that severe COVID-19 cases are often associated with a variety of underlying diseases, especially chronic underlying diseases represented by cardiovascular diseases (CVDs) (e.g. coronary heart disease). Recent studies have shown that COVID-19 cases subject to underlying CVDs have a worse prognosis than ordinary cases, and some cases experience myocardial injury associated with viral infection [Reference Zhu7]. The results of Wang et al. [Reference Wang8] showed that more than 7% of COVID-19 cases had myocardial injury, accounting for 22% of critically ill cases. Data suggest that CVD accounts for one-third of smoking-related deaths; even if only one cigarette is smoked daily, the incidence of coronary atherosclerotic heart disease (CAD) and stroke is significantly higher [Reference Hackshaw9]. Although studies regarding the association between smoking or CVD and COVID-19 have been published, the association between smoking and CVD and the role of the two in the development of the disease in COVID-19 cases require a systematic summary.
Therefore, the present study systematically collected the relevant literature and used meta-analysis to comprehensively analyse the association between smoking and CVD and disease progression in COVID-19 cases to scientifically underpin the screening, prevention and treatment of high-risk COVID-19 cases.
Materials and methods
Literature search strategy
By complying with the PICOS principle of Cochrane Handbook of Systematic Reviews, search strategy with the goal of recall is developed, and the study results are reported and discussed in accordance with (PRISMA-P) and MOOSE specifications. Two investigators independently searched PubMed and Cochrane Library databases, supplemented by manual search, to collect and report the past smoking history and CVD-related literatures of severe, severe and dead COVID-19 cases. For the published literatures with the search date from 1 Jan to 6 October 2020, the search method combining free words and subject headings was adopted, which was regulated by considering the characteristics of different databases. Only articles in English and online publications were included in the study. Details of literature search strategy are available in Supplementary Material.
Inclusion and exclusion criteria
Inclusion criteria: (1) Published relevant studies on the clinical manifestations of hospitalised COVID-19 cases comprising or containing smoking history or CVD data, and the study types are case−control study, cohort study, as well as cross-sectional study; (2) the study subjects are hospitalised COVID-19 cases diagnosed over the age of 18 years; (3) the study contents involve the smoking status, prevalence of CVD, corresponding severity of illness and disease outcome of COVID-19 cases. In this study, we defined severe COVID-19, a composite outcome, as severe COVID-19 cases (including critical cases) along with either the requirement of ICU admission, invasive ventilation, high-intensity medical care or resulting in death. The deterioration of the patient's condition to severe COVID-19 was defined as disease progression. CVD was defined as having a history or comorbidity of cardiovascular or cardiac disease in our study. Hypertension/heart failure/stroke in specific terms was excluded because these diseases often overlap, which may lead to overestimation.
Exclusion criteria: (1) Literature published repeatedly in the identical study; (2) non-English literatures; (3) review, short case report, conference abstract and letter; (4) literature with incomplete or missing data and data unavailable to contact authors.
Literature screening and data extraction
Two investigators independently screened the literatures, extracted the data and cross-checked by complying with the inclusion and exclusion criteria. In case of any disagreement, it was resolved by discussion or consultation with the third investigator. The main content of data extraction: (1) basic information of the study included (e.g., the first author of the study, publication time, region and study type); (2) baseline characteristics of the study subjects (e.g. smoking status, number of smokers, population smoking rate, sample size of the case group and the control group, as well as outcome indicators); (3) vital elements of risk of bias assessment.
Assessment of risk of bias of studies included
The Newcastle-Ottawa Literature Quality Assessment Scale (NOS) was adopted to assess the risk of bias of the studies included, and any disagreements were resolved by discussion with a third researcher. The studies included were scored from three aspects: the selection of study subjects, comparability between groups and exposure factors (overall 8 items), with a full score of 9, 0−4 as low-quality studies, as well as 5−9 as high-quality studies.
Statistical analysis
Meta-analysis on the data was conducted with the RevMan 5.2 software package offered by the Cochrane Collaboration. Where available, adjusted effect estimates were combined and in the absence of adjustment for confounders, raw effect estimates were combined in the study. Enumeration data were expressed as effect size using odds ratio (OR), which had the expression of 95% confidence interval (CI). Under the heterogeneity test results of the studies included as I 2 ⩽ 50%, the fixed-effect model was adopted for meta-analysis; under the heterogeneity test results of I 2 > 50%, the random-effect model was employed for meta-analysis. Significant clinical heterogeneity was dealt with by subgroup analysis or sensitivity analysis. Publication bias was assessed by plotting funnel plots.
Results
Literature search results and quality assessment
On the whole, 1651 studies were obtained from the search database, six duplicate studies were excluded, 1478 studies were excluded after reading the titles and abstracts, 146 studies were excluded after carefully reading the full texts and screening by complying with the inclusion and exclusion criteria, and finally 21 studies [Reference Cen10–Reference Zhou30] were included, all of which were retrospective cohort studies (Fig. 1). Seventeen of the studies were from China, and the remaining four were from the United States, United Kingdom, Japan and Italy. The exposure factors in this study were smoking history (including current or ex-smoking currently having quit smoking). Five studies assessed whether cases were currently smoking, six studies stratified cases by whether they were current or former smokers and the remaining 10 recorded whether cases had a history of smoking.

Fig. 1. Study flow diagram.
The result observed here was the presence of disease progression. To be specific, nine studies adopted disease severity as the outcome measure, six studies used death, two studies used disease progression, two studies used cases admitted to the ICU and the other two studies applied cases requiring upgrading of treatment regimen and requiring invasive ventilation as the outcome measures, respectively. Nineteen of the mentioned studies reported results in cases with CVD.
Basic information of included literatures
Among the 21 retrospective cohort studies included, comprising a total of 7041 COVID-19 cases, 2010 cases (28.6%) had disease progression, 984 (14.0%) had a history of smoking and 675 (9.6%) had concomitant CVD. The NOS quality scores of the studies included were 5 and above, all of which were high-quality studies, and the basic characteristics and quality assessment are listed in Tables 1 and 2.
Table 1. Characteristics of patients included in the smoking analysis cohort

Table 2. Characteristics of patients included in the CVDs analysis cohort

Smoking and COVID-19
Smoking history and COVID-19 disease progression
A total of 295 (30.0%) of the 984 COVID-19 cases with a smoking history (currently smoking or ex-smoking) experienced disease progression, compared with 28.4% (1715/6043) of the cases without a smoking history. As revealed from the heterogeneity test results, there was mild heterogeneity among the studies (I 2 = 47%, P = 0.010), and the fixed-effect model was adopted for meta-analysis. According to the results, (Fig. 2) cases with a smoking history achieved a higher rate of disease progression than cases without a smoking history (OR 1.53, 95% CI 1.29–1.81, P < 0.00001). Besides, no difference (P = 0.81) was observed between studies from China (17 studies; OR 1.52, 95% CI 1.26–1.83; I 2 = 54%; P < 0.00001) and studies outside China (4 studies; OR 1.60, 95% CI 1.08–2.36; I 2 = 2%; P = 0.02). Funnel plots were applied to detect publication bias, and the results showed that the funnels were symmetrical and publication bias was less likely.

Fig. 2. Association between smoking history and COVID-19 disease progression.
Smoking status and COVID-19 disease progression
Current smoking status was reported in 11 studies comprising 3601 COVID-19 cases, of which 340 (9.4%) were current smokers, resulting in a disease progression rate of 80/340 (23.5%) and 847/3261 (26.0%) were current non-smokers. The heterogeneity test results showed that there was mild heterogeneity among the studies (I 2 = 33%, P = 0.13), and the fixed-effect model was used for meta-analysis. As revealed from the results (Fig. 3), no significant difference was identified between current smoking and COVID-19 disease progression (OR 1.23, 95% CI 0.93–1.63, P = 0.15).

Fig. 3. Association between smoking status and COVID-19 disease progression.
Smoking history and COVID-19 mortality
In this study, a total of six studies, comprising 1822 COVID-19 cases, had an all-cause mortality rate of 37.5% (683/1822) using patient death as the outcome measure. According to the heterogeneity test results, there was no heterogeneity among the six studies on patient death (I 2 = 0%, P = 0.66), and the fixed-effect model was employed for meta-analysis. As demonstrated from the results (Fig. 4), cases with a smoking history had a higher mortality rate than cases without a smoking history (OR 1.91, 95% CI 1.35–2.69, P = 0.0002).

Fig. 4. Association between smoking history and COVID-19 mortality.
Cardiovascular disease and COVID-19
Cardiovascular disease and severe COVID-19
In this study, a total of 675 cases (9.7%) were diagnosed with COVID-19 and had CVD. A total of 18 studies reported disease results in COVID-19 cases with CVD, 13 of which reported severe disease as an outcome measure. A total of 49.3% (222/450) of COVID-19 cases with CVD developed severe disease in 13 studies, while only 23.1% (1048/4534) of cases without underlying cardiac disease experienced disease progression. According to the heterogeneity test results, there was no heterogeneity among the studies (I 2 = 1%, P = 0.44), and the fixed-effect model was employed for meta-analysis. As revealed from the results (Fig. 5), underlying CVD elevated the incidence of severe disease by 2.87 times in COVID-19 cases (OR 2.87, 95% CI 2.29–3.61, P < 0.00001). Funnel plots were adopted to detect publication bias, and the results demonstrated that the funnels were symmetrical and publication bias was less likely.

Fig. 5. Association between CVD and severe COVID-19.
Cardiovascular disease and COVID-19 mortality
A total of 60.0% (120/207) of COVID-19 cases with CVD died in the six studies, compared with only 34.9% (563/1615) of cases without underlying cardiac disease. According to the heterogeneity test results, significant heterogeneity was identified among the studies (I 2 = 75%, P = 0.001), and the random-effects model was employed for meta-analysis. As demonstrated from the results (Fig. 6a), underlying CVD made a large association with mortality in COVID-19 cases (OR 3.26, 95% CI 1.53–6.94, P = 0.002).

Fig. 6. Association between CVD and COVID-19 mortality.
Two studies of Xie et al. [Reference Xie26] and Zhou et al. [Reference Zhou30] were, respectively, identified as the main sources of heterogeneity by excluding line sensitivity analysis one by one for the six studies included. Analysis of the remaining four studies after excluding the mentioned two confirmed that all-cause mortality was 26.2% (235/898) in COVID-19 cases. The results of heterogeneity test showed that there was no heterogeneity among the four studies (I 2 = 12%, P = 0.33), and fixed effect model was used for meta-analysis. The results showed (Fig. 6b) that underlying CVD increased mortality by 3.05-fold in COVID-19 cases (OR 3.05, 95% CI 1.82–5.11, P < 0.0001). Funnel plots were applied to detect publication bias, and the results showed that the funnels were symmetrical and publication bias was less likely.
Discussion
A total of 21 studies were included in this meta-analysis, and the results showed that 14.0% of COVID-19 cases had smoking history, while 4.8% were still smoking. Smoking history displayed a significant association with COVID-19 disease progression (OR 1.53). Moreover, we found that the rate of CVD in COVID-19 cases was 9.7%, and the rate of severe disease (49.3%) and mortality (51.2%) were significantly increased in such cases, suggesting that underlying CVD is significantly associated with poor prognosis in COVID-19 cases.
As suggested by the World Health Organization's 2018 Global Adult Tobacco Report, the adult smoking rate in China continues to maintain a high value of 26.6%, and more than half (50.5%) of men are still smoking [31]. Wang et al. collected the data of national health survey in China in 2003, 2008 and 2013, respectively. As reported by the study, the proportion of smokers in China was 26.0%, 24.9% and 25.2%, respectively, demonstrating that the smoking status in China has not been improved over the past decade since the implementation of the Tobacco Control Regulations [Reference Wang32]. However, the smoking rate of COVID-19 cases obtained in this study was only 14.0%, which was much lower than the current international epidemiological survey data and study expectations. It is considered that besides the representativeness of the studies included, and the need for further adjustment of the relevant factors in methodology, factors (e.g. the considerable critically ill cases at the early stage of the epidemic, lack of medical history and records) should be considered. Furthermore, relevant information on smoking habits, number and patient complications in subsequent clinical work or even in response to the next epidemic should be rigorously collected to more effectively guide disease treatment.
Some studies have proposed relevant conclusions and opinions on the association between smoking and COVID-19 disease progression. Karanasos et al. [Reference Karanasos6] found that smoking increased the risk of severe disease in COVID-19 cases, particularly in younger cases without diabetes (OR 1.34, 95% CI 1.07–1.67). Patanavanich et al. [Reference Patanavanich33] proposed that smoking displayed a significant association with COVID-19 disease progression (OR 1.91, 95% CI 1.42–2.59). The study by Farsalinos et al. [Reference Farsalinos34] indicated that cases who were currently smoking had a worse prognosis than cases without a history of smoking (OR 1.53, 95% CI 1.06 −2.20). The study by Zhao et al. [Reference Zhao35] also proposed that active and persistent smoking can increase the incidence rate of severe COVID-19 by nearly 2-fold (OR 1.98, 95% CI 1.29–3.05). Lowe et al. [Reference Lowe36] recently found patients who smoked more than 30 pack-years had a higher odds of hospitalisation (OR 2.25, 95% CI 1.76–2.88), and were more likely to die following a COVID-19 diagnosis (OR 1.89, 95% CI 1.29–2.76) when compared with never smokers. The above studies and analyses support the conclusions drawn from our study, but some studies still draw opposite conclusions. The study by Lippi et al. [Reference Lippi5] reported that active smoking was not associated with COVID-19 severity (OR 1.69; 95% CI 0.41–6.92; P = 0.254). Similar conclusions were drawn by Lombardi et al. [Reference Lombardi37], who concluded that active smoking was not significantly associated with COVID-19 severity. However, Lippi et al. did not perform a risk of bias assessment of the studies included, Lombardi et al. only investigated in-hospital vs. non-in-hospital mortality using active smoking as an exposure factor, and the study was limited to the induction and description of clinical data rather than a systematic review and analysis. Therefore, the conclusions of the mentioned two studies should be objectively analysed and interpreted. Comparison between the six systematic reviews or meta-analyses and the present review among smoking is listed in Table 3.
Table 3. Comparison of the six systematic reviews or meta-analyses with the present review among smoking
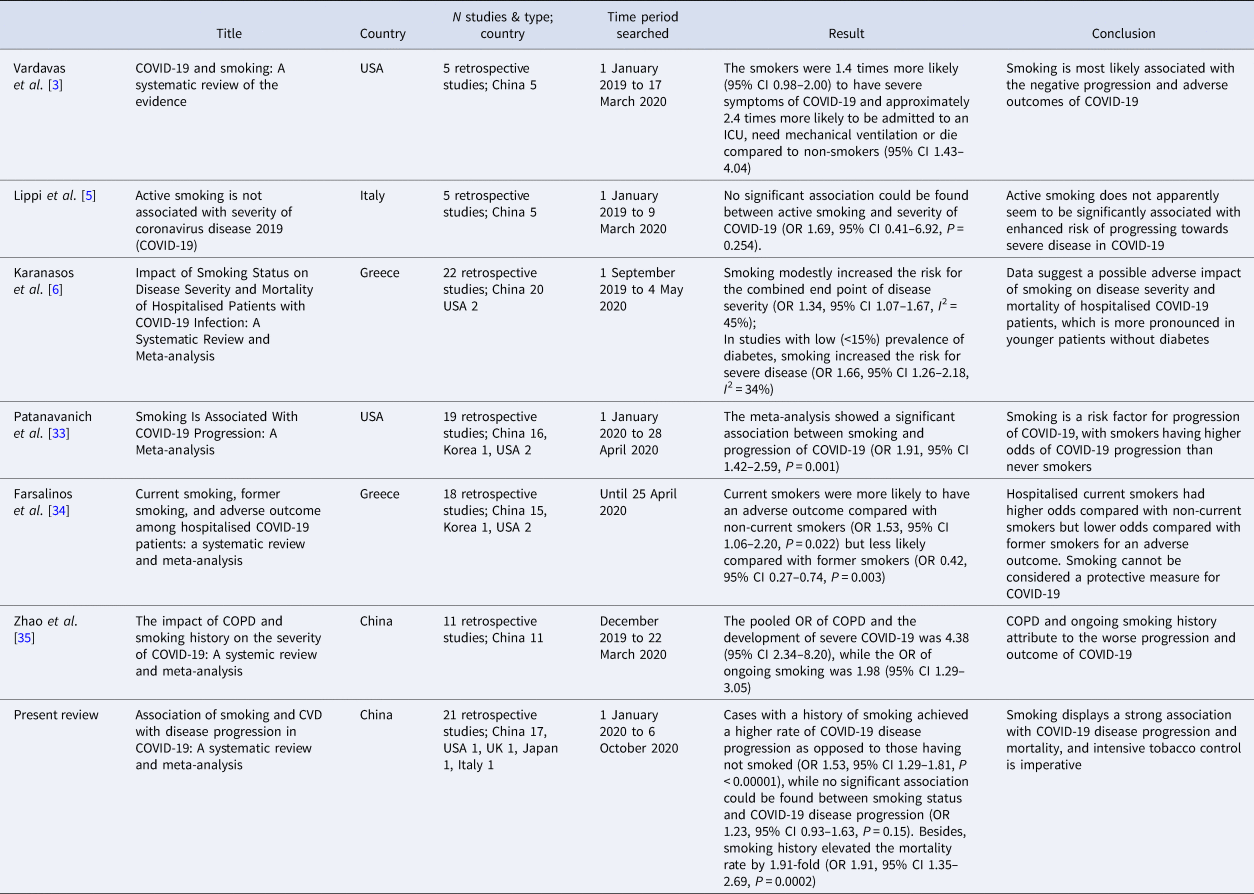
Recent studies suggest that active smokers are underrepresented among patients with COVID-19 [Reference Tsigaris38]. ‘Smoker's paradox’ has been claimed recently and a false impression that smoking can give protection against COVID-19 was perceived by the general population. Changeux et al. [Reference Changeux39] have proposed the nicotinic hypothesis. Nicotinic acetylcholine receptor (nAChR) was found to play a role in the COVID-19 inflammatory syndrome. Due to this finding, the hypothesis suggests that nicotine could reduce SARS-CoV-2 infection and alleviate COVID-19 progression by competing with SARS-CoV-2 in binding to the nAChR. Besides, the anti-inflammatory effect of nicotine and the inhibiting effect of nitrogen monoxide in SARS-CoV-2 replication might also support the hypothesis [Reference Usman40]. But the hypothesis could only be explained by exposure to nicotine, rather than the cigarette smoke with thousands of harmful chemicals. Since only questionable data were reported, and evidence is inadequate, the protective effects of nicotine should not be inferred determinedly. However, nicotinic hypothesis offers the public an alternative vision and a potential treatment of COVID-19. Placebo-controlled trials should be performed to assess the viability of nicotine as a therapeutic option.
Although the major symptoms of COVID-19 do not arise from the cardiovascular system, data analysis shows that more COVID-19 cases have CVD, probably related to the older age of COVID-19 cases and their male predominance [Reference Huang16]. The present study indicated that the rate of coexisting CVD in COVID-19 cases was 9.7%, supporting this view as well. Cases subject to underlying CVDs were found to be at higher risk for acute cardiovascular events, thromboembolism, infection and disease progression after SARS-COV-2 infection [Reference Zheng41]. In the study, the proportion of severe disease and death in such cases was found to be significantly elevated as well. Likewise, considerable existing studies on respiratory infectious diseases (e.g., SARS and MERS) have also reported related cardiovascular complications, the basic pathophysiological mechanism of which is that viral infection increases systemic inflammatory response, thereby causing an imbalance in cardiac metabolic supply and demand [Reference Alhogbani42]. For this reason, the special situation of cardiovascular system diseases combined with COVID-19 refers to a major focus and difficulty in this outbreak treatment.
A study of 138 SARS-COV-2 infected cases found that 7.2% had myocardial injury, while the proportion of myocardial injury in SARS-COV-2 infected cases admitted to ICU was 22.2%, which was significantly higher than that in SARS-COV-2 infected cases admitted to general ward (2.0%) [Reference Wang8]. Acute myocardial injury (77%) and heart failure (49%) were common complications and associated with higher mortality in COVID-19 cases regardless of whether the cases had a relevant previous medical history [Reference Chen12]. As highlighted by another study, myocardial injury displayed a significant association with mortality in COVID-19, while elevated TnT levels had some cautionary role [Reference Guo43].
The specific mechanism by which SARS-COV-2 causes cardiopulmonary injury is unknown. Studies have shown that SARS-COV-2 enters cells largely through spike protein on the viral surface and ACE2 in host respiratory epithelial cells after entering the human body, causing down-regulation of ACE2 and increased angiotensin II (Ang II) levels in the body; as a result, multiple organ dysfunction is induced (e.g. cardiovascular lesions and lung injury) [Reference Liu44]. It has been confirmed in the existing literature that Ang II levels are significantly elevated in cases infected with SARS-COV-2, and the degree of its increase is associated with the severity of the disease, suggesting that SARS-COV-2 may activate the renin−angiotensin system (RAS) to induce an inflammatory storm and cause poor prognosis of cases [Reference Liu44].
It is necessary to pay attention to the treatment of underlying CVDs in cases with COVID-19, as well as to guard against the side effects of drugs and avoid aggravating the condition. Existing studies suggest that the use of ACEI and ARB drugs may cause potential harm, and their application has sparked numerous controversies since they may cause ACE2 to up-regulated and accelerate SARS-COV-2 infection and lung injury [Reference Callera45]. However, large-sample clinical studies on the effects of ACEIs or ARBs on lung injury in infected cases have been rarely conducted [Reference Vaduganathan46]. At present, there is no clinical or scientific evidence to support the change or discontinuation of ACEI/ARB drugs in cases with COVID-19. In addition, antiviral drugs (e.g., lopinavir/ritonavir) although used as first-line anti-SARS-COV-2 drugs, have common cardiovascular adverse effects including hypertension, prolonged P-R interval and torsades de pointes [Reference Reyskens47]. Chloroquine phosphate is capable of inducing serious cardiovascular adverse effects (e.g. arrhythmias, shock and Asperger's syndrome) [Reference Pareek48]. The adverse reactions of antiviral drugs and drug−drug interactions still need to be further observed in clinical practice.
Smoking can be harmful to all the organs in the human body. It is a common risk factor for CVD, cancer, diabetes and chronic respiratory disease. Cardiovascular morbidity and mortality attributed to smoking remains a global health problem, especially in low-income countries that lack smoking restriction policies. As revealed from the existing studies, CVD risk in light smokers decreases to baseline levels within 5 years after smoking cessation, while CVD risk in heavy smokers does not decrease to baseline levels in never smokers until 15 years after smoking cessation [Reference Duncan49]. The mechanism of smoking leading to the occurrence and development of coronary heart disease may consist of the following processes: damaging endothelial function and inducing vasospasm, aggravating inflammatory response, disrupting the balance of coagulation and fibrinolysis system in the body, as well as promoting abnormal lipid metabolism. With the flooding of tobacco and long-term, extensive and massive smoking by the people, the age of onset of CVD is gradually younger, the prevalence tends to rise, and the cardiovascular basis and cardiac functional reserve are decreasing. It was also demonstrated that ACE2 expression was up-regulated in airway epithelial cells of smokers, causing smokers to be more susceptible to COVID-19 [Reference Leung50]. After the outbreak of COVID-19, severe infections and inflammatory factor storms attributed to SARS-COV-2 caused a second blow to the already fragile heart of cases with CVD and progressed. In addition, the relative tension of medical resources at the early stage of the epidemic, the closure of considerable pharmacies and the closed management of residential areas make some cases with stable CVD experience drug interruption and cannot complete the self-management of the disease. Cases subject to CVD can be suggested to have a higher risk of conversion to severe and critical illness and even life-threatening during the COVID-19 epidemic, while smoking impacts the development of CVD and COVID-19.
In brief, COVID-19 has plunged both China and the world into a difficult period in history. The long-term impact of this outbreak may profoundly change the world. With the society gradually recovering from the epidemic, the anti-smoking campaign should continue to persist and facilitate the people's health and sustainable economic and social development.
Several limitations are revealed here. First, the studies included in this paper were retrospective cohort studies, and most of the studies were from China. We cannot exclude the possibility of ethnic differences in smoking and susceptibility to severe COVID-19. Yet, the use of aggregate data may preclude adjustments for certain confounders such as age, gender and comorbidities reported to be predictive of disease severity. Further prospective cohort studies dedicated to analysing this matter should take into consideration of such adjustments. Second, some studies did not clearly distinguish smoking history from current smoking status. Third, the association between duration or cumulative smoking exposure and COVID-19 severity could not be assessed in our study since no relevant data were reported in the included studies. Despite all the pressure in the pandemic, Smoking Index should be promoted in clinical settings, and detailed data about smoking history needs to be collected by healthcare professionals/researchers adequately. Finally, most studies separate CVD population from hypertension population, resulting in overlapping and missing data.
Despite the mentioned limitations, this meta-analysis assessed the association between smoking, CVD and disease progression in COVID-19. There were many included literatures with large sample size and no significant publication bias. Thus, the reliability of meta-analysis results remains strong.
Conclusion
It is currently evidenced that smoking displays a strong association with COVID-19 disease progression and mortality. It is imperative to curb tobacco flooding. Moreover, cases with CVD have a significantly elevated risk of poor disease progression and death when subject to COVID-19. The association between COVID-19 and CVD, and the potential effect exerted by smoking in the development of the two, require in-depth verifications by larger and higher quality studies.
Supplementary material
The supplementary material for this article can be found at https://doi.org/10.1017/S0950268821001138
Financial support
This study was funded by grants from the Hebei Province Science and Technology Support Program (20277706D). The research was designed, conducted, analysed and interpreted by the authors entirely independently of the funding sources.
Conflict of interest
The authors declare that they have no competing interests.
Data availability statement
The data that support the findings of this study are available from the corresponding author upon reasonable request.