Introduction
The composition of a parasite community is the result of multiple factors influencing parasitic organisms and their hosts (Poulin, Reference Poulin2007). Besides environmental factors (Galaktionov and Bustnes, Reference Galaktionov and Bustnes1999; Marcogliese, Reference Marcogliese2001; Maestri et al., Reference Maestri, Shenbrot and Krasnov2017; Clark et al., Reference Clark, Clegg, Sam, Goulding, Koane and Wells2018), the biology of a given host usually has a great impact on the species richness of parasite communities and the abundance of individual parasite species (or in combination with the environment, Berkhout et al., Reference Berkhout, Borregaard, Brandl, Brändle, Dehling, Hof, Poulin and Thieltges2020). In general, the species richness of parasites is intimately linked with the species diversity of their hosts (Krasnov et al., Reference Krasnov, Shenbrot, Khokhlova and Degen2004; Hechinger and Lafferty, Reference Hechinger and Lafferty2005; Thieltges et al., Reference Thieltges, Hof, Borregaard, Dehling, Brändle, Brandl and Poulin2011; Kamiya et al., Reference Kamiya, O'Dwyer, Nakagawa and Poulin2014); however, both the range and spatial pattern of distribution of a given host species may affect parasite assemblages, as the resource availability varies across the whole distribution range of the host (Clark et al., Reference Clark, Clegg, Sam, Goulding, Koane and Wells2018; Berkhout et al., Reference Berkhout, Borregaard, Brandl, Brändle, Dehling, Hof, Poulin and Thieltges2020). Studying parasite communities and the phylogenetic relationships between parasite species (especially parasite species exhibiting high host-specificity) can shed light on the evolutionary history and historical dispersion of their hosts (e.g. Nieberding et al., Reference Nieberding, Morand, Libois and Michaux2004; Nieberding and Olivieri, Reference Nieberding and Olivieri2007; Verneau et al., Reference Verneau, Du Preez and Badets2009). Moreover, an unusual recent distribution of host-specific parasites may serve as a good indicator of the human-induced introduction of invasive species into non-native regions (Benovics et al., Reference Benovics, Desdevises, Vukić, Šanda and Šimková2018; Šimková et al., Reference Šimková, Řehulkova, Jean Robertin, Jorissen, Scholz, Faltynková, Mašová and Vanhove2018; Wilson et al., Reference Wilson, Saunders and Hutson2019).
The Apennine Peninsula is one of the regions in Europe which has experienced numerous introductions of non-native species over the last few decades (Gherardi et al., Reference Gherardi, Bertolino, Bodon, Casellato, Cianfanelli, Ferraguti, Lori, Mura, Nocita, Riccardi, Rossetti, Rota, Scalera, Zerunian and Tricarico2008; Nocita et al., Reference Nocita, Tricarico and Bertolino2017; Volta et al., Reference Volta, Jeppesen, Sala, Galafassi, Foglini, Puzzi and Winfield2018). According to Gherardi et al. (Reference Gherardi, Bertolino, Bodon, Casellato, Cianfanelli, Ferraguti, Lori, Mura, Nocita, Riccardi, Rossetti, Rota, Scalera, Zerunian and Tricarico2008), approximately 46% of freshwater fish species are non-native and their presence in this peninsula was induced by humans. Moreover, anthropogenic modifications of habitats significantly altered the natural abundance and composition of native fish fauna (Bianco, Reference Bianco1995). Gherardi et al. (Reference Gherardi, Bertolino, Bodon, Casellato, Cianfanelli, Ferraguti, Lori, Mura, Nocita, Riccardi, Rossetti, Rota, Scalera, Zerunian and Tricarico2008) also hypothesized that with recent trends in introduction (either intentional to add commercially ‘more attractive’ fish into Italian rivers, or unintentional via ballast water exchange), the local fauna will become even more homogenized in the future. Currently, the northern Apennine Peninsula is characterized by two main ichthyogeographic districts: Padano-Venetian and Tuscano-Latium; each reflecting the recent distribution of particular endemic species (Bianco, Reference Bianco1990, Reference Bianco1995). The Padano-Venetian district covers the northern Adriatic basin and its faunal elements are shared with the north-western Balkans. The area of this district basically corresponds to the drainage of the Po River during the Last Glacial Maximum, when the sea level drastically regressed (Pielou, Reference Pielou1979; Bianco, Reference Bianco1990; Waelbroeck et al., Reference Waelbroeck, Labeyrie, Michel, Duplessy, McManus, Lambeck, Balbon and Labracherie2002). During that time, freshwater river streams facilitated the mixing of faunas between two currently isolated regions (i.e. the north-western part of the Balkan, and the north-eastern part of the Apennine peninsulas) (Waelbroeck et al., Reference Waelbroeck, Labeyrie, Michel, Duplessy, McManus, Lambeck, Balbon and Labracherie2002; Stefani et al., Reference Stefani, Galli, Crosa, Zaccara and Calamari2004). The Tuscano-Latium district spreads out along the Tyrrhenian slope of central Italy, between the River Arno in the north and the River Tiber in the south, and reflects the distribution of endemic Squalius lucumonis and encompasses almost exclusively endemic species (Bianco, Reference Bianco1990; Kottelat and Freyhof, Reference Kottelat and Freyhof2007).
The Cyprinoidei [recently elevated Cyprinidae, and divided into the Leuciscidae and Cyprinidae families, following Stout et al. (Reference Stout, Tan, Lemmon, Lemmon and Armbruster2016), Schönhuth et al. (Reference Schönhuth, Vukić, Šanda, Yang and Mayden2018) and Tan and Armbruster (Reference Tan and Armbruster2018)] is the most diversified freshwater fish group in the Apennine Peninsula represented by 15 species, of which 12 are endemic. The distributions of three out of five species native to the Padano-Venetian district [namely, Barbus plebejus, Rutilus aula (Bonaparte, 1841) and Squalius squalus] extend up to the north-western Balkans (for the distribution ranges of species, see Kottelat and Freyhof, Reference Kottelat and Freyhof2007). The other two species native to this district [i.e. Chondrostoma soetta and Protochondrostoma genei – the latter monotypic genus was only recently erected by Robalo et al. (Reference Robalo, Almada, Levy and Doadrio2007)] are present only in the Apennine Peninsula. In contrast, Telestes muticellus represents a species with a relatively wide distribution range covering both the ichthyogeographical Padano-Venetian and Tuscano-Latium districts. Nonetheless, T. muticellus actually appears to represent a species complex that contains taxa with large genetic diversity (Stefani et al., Reference Stefani, Galli, Crosa, Zaccara and Calamari2004), putatively linked with the fragmentation of populations into refuges during the last glaciation cycles (Marchetto et al., Reference Marchetto, Zaccara and Muenzel2010).
The distribution of the fish fauna, and especially cyprinoids, is well known in the Apennine Peninsula; however, knowledge about their parasite diversity is scarce. In light of the previous discussion, there are two major reasons to investigate the diversity of parasites in a given region: (1) parasites can represent a threat to native fauna (especially co-introduced parasite species), and (2) parasites can serve as a useful tool for investigating the evolutionary history of their hosts. Therefore, in the present study, we aimed to study the species diversity of parasitic helminths and investigate whether the diversity and distribution of the local parasite fauna of cyprinoid fish have been influenced by the introductions of non-native fish species into this region; i.e. that parasite assemblages also include alien parasite species. Moreover, we expect that native endemic cyprinoid fish will also harbour endemic parasite species (especially highly diversified and host-specific monogeneans), and by investigating the phylogenetic relationships of endemic parasites to congeners from different European regions, we will shed new light on the evolutionary history of their hosts.
Material and methods
Parasite sampling, fixation and identification
During the summers of 2015 and 2018, a total of 92 fish specimens belonging to ten species were collected from five localities in northern Italy (Fig. 1) and examined for metazoan parasites. In addition, ten specimens of B. plebejus and six specimens of S. squalus were collected by a local fisherman from the uncertain site on Po River (Table 1). Fish species were determined using Kottelat and Freyhof (Reference Kottelat and Freyhof2007). Prior to parasitological dissection, live fish were kept in aerated holding barrels with river water from the collection site. Fishes were paralysed and then sacrificed by severing the spinal cord. All applicable institutional, national and international guidelines for the care and use of animals were followed. The study was approved by the Animal Care and Use Committee of the Faculty of Science, Masaryk University in Brno (Czech Republic).
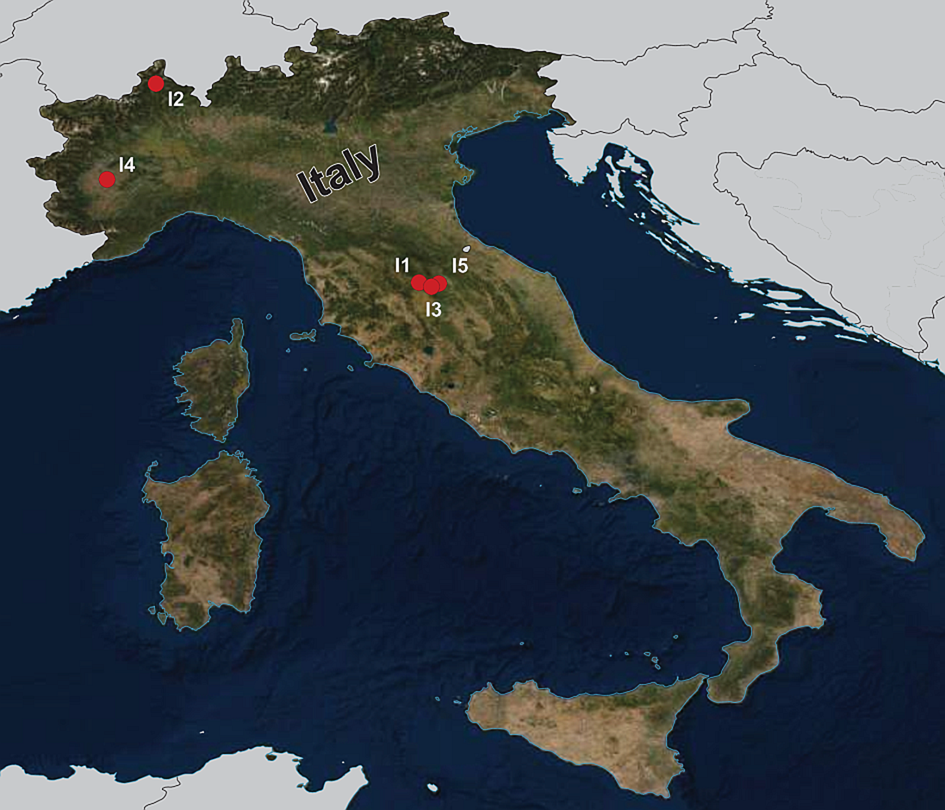
Fig. 1. Map of the collection sites in northern Italy.
Table 1. List of investigated cyprinoid species with their respective collection sites, and list of parasite species collected from each host species divided into major taxonomical groups

LocID, codes of collection sites corresponding to the codes in Fig. 1; N, number of processed fish specimens; NSP, number of parasite species; dashes (–) indicate that no parasite species of the given taxa was found. Potentially alien species are marked by asterisks (following Galli et al., Reference Galli, Strona, Benzoni, Crosa and Stefani2007).
Fish were dissected using the standard method described in Ergens and Lom (Reference Ergens and Lom1970) and individual organs were observed under a stereomicroscope. Monogenean parasites were collected from fish skin, fins, gills and head cavities. Eyes, brain and muscles were checked for the presence of metacercariae of digeneans. Intestine and abdominal internal organs (i.e. gallbladder, gas bladder, liver and spleen) were checked for the presence of acanthocephalans, cestodes, digeneans and nematodes.
Monogenean parasites (Dactylogyrus spp., Gyrodactylus spp. and Paradiplozoon spp.) were mounted on slides (completely flattened under coverslip pressure) and fixed in a mixture of glycerine and ammonium picrate (Malmberg, Reference Malmberg1957). For Dactylogyrus, at least five specimens of each species were cut in half, and one part (usually the half with the attachment organ) was preserved in 96% ethanol for further molecular analyses. The remaining body part was mounted on a slide for species determination by means of morphology. The body half used for the morphological study was later deposited (one per species) as a hologenophore [see Pleijel et al. (Reference Pleijel, Jondelius, Norlinder, Nygren, Oxelman, Schander, Sunberg and Thollesson2008) for terminology] in the helminthological collection of the Institute of Parasitology of the Czech Academy of Sciences in České Budějovice (IPCAS). Species determination was performed according to the size and shape of the sclerotized elements of the attachment organ and copulatory organs following Pugachev et al. (Reference Pugachev, Gerasev, Gussev, Ergens and Khotenowsky2009). Endoparasitic helminths were removed from hosts and preserved in 70% ethanol. Prior to fixation in Canada balsam, cestodes and digeneans were stained in iron acetocarmine, following the protocol of Georgiev et al. (Reference Georgiev, Biserkov and Genov1986). Species determination followed Kuchta et al. (Reference Kuchta, Scholz and Bray2008) and Gibson et al. (Reference Gibson, Jones and Bray2002), respectively. Nematodes were mounted on slides and covered in a mixture of glycerine and water (in the ratio 3:7), and cleared by gradually increasing the volume of glycerol (according to Moravec, Reference Moravec2013). The species determination of nematode parasites followed Moravec (Reference Moravec2013). Basic epidemiological data, i.e. prevalence, mean abundance, and minimum and maximum intensities of infection, were calculated for each parasite species (Table 2) according to Bush et al. (Reference Bush, Lafferty, Lotzs and Shostakll1997). Prevalence, as the percentage of fish infected by a given parasite species, and mean abundance, as the mean number of parasite specimens per individual host taking into account both infected and uninfected hosts, were calculated. Following the suggestion of Rózsa et al. (Reference Rózsa, Reiczigel and Majoros2000) for interpreting epidemiological data, a confidence interval at the level of 95% was calculated for mean abundance.
Table 2. Calculations of epidemiological data for parasite species

P, prevalence; A, mean abundance (confidence interval for level 95%); I, range of intensity of infection (min–max). Potentially alien species are marked by asterisks (following Galli et al., Reference Galli, Strona, Benzoni, Crosa and Stefani2007).
DNA extraction, amplification and sequencing
The extraction of genomic DNA from Dactylogyrus parasites was performed using a commercially produced extraction kit (DNeasy Blood & Tissue Kit, Qiagen, Hilden, Germany) following the manufacturer's protocol. Two genomic DNA regions were amplified, the first one covering the partial gene coding for 18S rRNA, the complete ITS1 region and the partial gene coding for 5.8S rRNA (hereinafter abbreviated as 18S, ITS1 and 5.8S), and the second one covering the partial gene coding for 28S rRNA (hereinafter abbreviated as 28S). The first region was amplified using the primers S1 (forward, 5′-ATTCCGATAACGAACGAGACT-3′) and IR8 (reverse, 5′-GCTAGCTGCGTTCTTCATCGA-3′), which anneal to the segments of DNA coding for 18S and 5.8S rRNA, respectively (Šimková et al., Reference Šimková, Plaisance, Matějusová, Morand and Verneau2003). The amplification reaction followed the protocol optimized by Benovics et al. (Reference Benovics, Desdevises, Vukić, Šanda and Šimková2018). The latter region was amplified using the primers C1 (forward, 5′-ACCCGCTGAATTTAAGCA-3′) and D2 (reverse, 5′-TGGTCCGTGTTTCAAGAC-3′) (Hassouna et al., Reference Hassouna, Michot and Bachellerie1984), following the protocol of Benovics et al. (Reference Benovics, Desdevises, Šanda, Vukić and Šimková2020). The PCR products (~1000 for 18S, ITS1 and 5.8S, and ~800 bp for partial 28S) were checked on 1% agarose gel and purified using the ExoSAP-IT kit (Ecoli, Bratislava, Slovakia) following the standard protocol. The purified products were directly sequenced using the same primers as for PCR and BigDye Terminator Cycle Sequencing kit (Applied Biosystems, Prague, Czech Republic). Sequencing was performed on an ABI 3130 Genetic Analyzer (Applied Biosystems).
Morphometric data and species description
The mounted monogeneans (or their parts) were studied using an Olympus BX 61 microscope equipped with phase-contrast optics. Drawings were made with the aid of a drawing attachment and edited with a graphic tablet compatible with Adobe Illustrator and Adobe Photoshop. Measurements were taken using digital image analysis (Stream Motion, version 1.9.2) and are given in micrometres [mean followed by the range and number of specimens measured (n) in parentheses]. The measurement scheme for the sclerotized structures of Dactylogyrus is shown in Fig. 2. The numbering of hook pairs (in Roman numerals) follows that recommended by Mizelle (Reference Mizelle1936). The male copulatory organ is abbreviated as MCO.

Fig. 2. Scheme of measurements for sclerotized structures of Dactylogyrus spp. A, anchor (a – total length, b – inner root length, c – outer root length, d – point length); B, dorsal bar (e – width); C, ventral bar (f – width); D, hook (g – length); E, male copulatory organ (h – total straight length, i – tube curved length); F, vagina (j – curved length).
The parasitological material was deposited in the Helminthological collection of the Institute of Parasitology, Academy of Sciences of the Czech Republic, České Budějovice, Czech Republic (IPCAS). The material was remounted in Canada balsam (according to Ergens, Reference Ergens1969) prior to depositing. To comply with the regulations set out in article 8.5 of the amended 2012 version of the International Code of Zoological Nomenclature (ICZN, 2012), details of the new species have been submitted to ZooBank. Note that the authorities of the new taxa described below are Francová & Benovics (according to the ICZN, 2012).
Phylogenetic analyses
To infer the phylogenetic positions of new Dactylogyrus species, three genetic markers were selected for phylogenetic analyses; partial genes coding for 18S rRNA and 28S rRNA, and the ITS1 region. In addition to the newly obtained sequences, sequences corresponding to already described Dactylogyrus species were retrieved from GenBank (see accession numbers in the Supplementary data, Table S1). The Dactylogyrus species for phylogenetic analyses were selected in order to investigate the phylogenetic relationships between species from two southern European peninsulas with a historical connection (i.e. the Apennine and Balkan peninsulas), and their relationships to Dactylogyrus species parasitizing cyprinoids with a wide distribution range in Europe, especially in the geographically proximal central Europe. For Dactylogyrus species found on cyprinoids of the Apennine Peninsula and previously reported on cyprinoid species in other European regions (the Balkans or Central Europe, Šimková et al., Reference Šimková, Morand, Jobet, Gelnar and Verneau2004; Benovics et al., Reference Benovics, Kičinjaová and Šimková2017, Reference Benovics, Desdevises, Vukić, Šanda and Šimková2018; Řehulková et al., Reference Řehulková, Benovics and Šimková2020), already published DNA sequences were retrieved from GenBank. DNA sequences were concatenated and aligned using the fast Fourier transform algorithm implemented in MAFFT (Katoh et al., Reference Katoh, Misawa, Kuma and Miyata2002) and subsequently manually trimmed to unify the lengths of all used sequences. The final alignment was built from 42 Dactylogyrus species (38 previously described, and 4 new) of various cyprinoid taxa from the Apennine and Balkan peninsulas, and central Europe. Following the phylogenetic reconstruction of Benovics et al. (Reference Benovics, Desdevises, Vukić, Šanda and Šimková2018), three common Dactylogyrus species of Carassius gibelio (Bloch, 1782) (namely D. anchoratus, D. formosus and D. vastator) were used as the outgroup for rooting the phylogenetic trees. The aligned dataset was treated as partitioned, each partition associated with an individual genetic marker, i.e. 18S, 28S, ITS1. General time-reversible model was applied to each partition. Phylogenetic analyses were computed by means of maximum likelihood (ML) and Bayesian inference (BI) methods, using RaxML v 8.2.11 (Stamatakis, Reference Stamatakis2006, Reference Stamatakis2014) and MrBayes v 3.2.6 (Ronquist et al., Reference Ronquist, Teslenko, van der Mark, Ayres, Darling, Höhna, Larget, Liu, Suchard and Huelsenbeck2012), respectively. Internal node support for the ML tree was assessed by running 1000 bootstrap pseudoreplicates. Two parallel runs, each with four Markov chains, were executed for the BI analysis and run for 107 generations. Trees were sampled every 102 generations and the first 30% of the resulting trees were discarded as initial burn-in after checking convergence of runs in Tracer v 1.7.1 (Rambaut et al., Reference Rambaut, Drummon, Xie, Baele and Suchard2018). Posterior probabilities were calculated as the frequencies of samples recovering particular clades.
Results
Diversity of helminth parasites
A total of 41 helminth species were collected and identified (Table 2), with D. vistulae as the most prevalent parasite species, and G. lomi as the most abundant species. Parasites communities with the greatest species richness were harboured by S. lucumonis from the Torrente Cerfone locality (11 helminth species). In contrast, only three helminth species were collected from C. soetta (Carmagnola). The basic epidemiological parameters, i.e. prevalence, mean abundance with a confidence interval at the level of 0.95, and minimum and maximum intensity of infection, are presented for each parasite species in Table 2. In general, monogeneans exhibited the highest species diversity. Thirty-one known monogenean species and four newly-described species were found on the gills and in the nasal cavity of fish. Concerning Dactylogyrus, out of 20 species, D. vistulae was reported on the widest range of host species (collected from six host species) exhibiting the highest prevalence and highest mean abundance on S. squalus. The second most abundant monogenean genus, Gyrodactylus, was represented by 14 species, of which G. gobii and G. laevis were collected from the highest number of host species (four species). Only one diplozoid species (Paradiplozoon megan) was found, parasitizing all four species collected from Torrente Cerfone, with the highest prevalence on S. lucumonis. However, P. megan achieved the highest mean abundance and intensity of infection on P. genei. Only one species of eye fluke (metacercariae of Diplostomum spathaceum) was found on two specimens of T. muticellus from Torrente Cerfone with moderate abundance. No other digeneans were detected during this survey. Intestinal helminths were represented by three species of nematodes (Molnaria intestinalis, Pseudocapillaria tormentosa and Rhabdochona hellichi) and a single cestode species belonging to the monotypic genus Bathybothrium (Bathybothrium rectangulum) collected from two Barbus species (B. caninus and B. tyberinus). However, only a single specimen of B. rectangulum was collected from B. tyberinus.
Morphological and molecular characterization of the new species
The new Dactylogyrus species described in this study were classified into two morphological groups according to Pugachev et al. (Reference Pugachev, Gerasev, Gussev, Ergens and Khotenowsky2009) on the basis of the morphology of the sclerotized parts of the reproductive organs – the MCO and the vagina.
Three of the new Dactylogyrus species (i.e. D. globulatus n. sp., D. conchatus n. sp. and D. sagittarius n. sp.) belonged to the ‘ergensi’ (or sometimes termed ‘chondrostomi’) morphological group, i.e. a group of Dactylogyrus species having an MCO with a complex accessory piece, comprising ‘a broad tongue-shaped lobe directed backwards along the circle of the curved tube’, and a vagina in the form of an elongate tube. Of the ‘ergensi’ group species, the three new species most resemble those possessing a three-armed or cross-shaped ventral bar (with a simple, not bifurcated, anterior arm): Dactylogyrus elegantis Gussev, 1966; D. dirigerus Gussev, 1966; D. ergensi Molnár, Reference Molnár1964; D. nybelini Markewitsch, 1933; D. naviculoides Ergens, 1956; D. soufii Lambert, 1977; D. caucasicus Mikailov et Shaova, 1973; and D. dimitrovae Kakacheva-Avramova, 1972.
Dactylogyrus ergensi, collected from Chondrostoma nasus in the Danube River in Hungary, was described by Molnár (Reference Molnár1964). Since then, specimens from several distant localities displaying the same basic morphology but also high morphometrical variability in their sclerotized structures have been assigned to this species (Pugachev et al., Reference Pugachev, Gerasev, Gussev, Ergens and Khotenowsky2009); we consider these specimens likely representing the members of more than one species. The morphology of the sclerotized structures of the present new species was compared with that of D. ergensi using an original description of the latter species (Molnár, Reference Molnár1964).
The last of the four Dactylogyrus species described in this study (i.e. D. opertus n. sp.) was assigned to the ‘nanus’ morphological group, specifically to the subgroup of species displaying the following features: an MCO comprising a simple copulatory tube, smoothly curved all along its length from the initial part (or more curved distally), and terminally enclosed with a markedly enlarged distal part (a sheath) of the accessory piece; a vagina present as a short or medium-size bent cylindrical tube, closed with a cap (i.e. the subgroup including Dactylogyrus nanus Dogiel et Bychowsky, 1934; D. nanoides Gussev, 1966; and D. suecicus Nybelin, 1937).
Descriptions of all new Dactylogyrus species are given below.
Dactylogyridea Bychowsky, 1937
Dactylogyridae Bychowsky, 1933
Dactylogyrus Diesing, 1850
Dactylogyrus globulatus n. sp. (Fig. 3)

Fig. 3. Sclerotized structures of Dactylogyrus globulatus n. sp. ex Chondrostoma soetta. A, anchor; DB, dorsal bar; VB, ventral bar; N, needle; I–VII, hooks; VG, vagina; MCO, male copulatory organ.
urn:lsid:zoobank.org:act:CAECC9DF-17D4-4699-9A52-2BF8C4373B30
Type host and locality: Chondrostoma soetta Bonaparte, 1840; Carmagnola, Cave Germaire (44°51′42.96″N, 07°40′26.33″E).
Site on host: Gill lamellae.
Type and voucher material: IPCAS M-753 (holotype; five paratypes; one hologenophore voucher).
Representative DNA sequences: A nucleotide sequence of partial gene for 28S rRNA (789 bp long; MW443032), and nucleotide sequences representing a fragment (961 bp long; MW443035) including partial gene for 18S rRNA (487 bp), and the ITS1 region (474 bp). No intraspecific variability was found (seven specimens were analysed).
Etymology: The specific name is derived from Latin (globus = ball, sphere, globe; treated as an adjective) and refers to the shape of the distal part of the MCO accessory piece which seems to be rolled into a ball.
Description (based on eight specimens): Body length 469 (437–531; n = 4); greatest width 70 (59–86; n = 4). Haptor with one pair of anchors (dorsal). Anchors with moderately developed roots (inner root longer, more or less flattened terminally); roots forming an angle of/>90°; shaft evenly curved to point; point with slightly recurved tip; total length 32 (30–34; n = 8); inner root length 12 (11–13; n = 8); outer root length 4 (3–4; n = 8); point length 9 (8–10; n = 8). Anchor filaments well developed. Dorsal bar 23 (20–25; n = 8) wide, saddle-shaped. Ventral bar 21 (18–23; n = 8) wide, cross-shaped, with posterior arm markedly reduced to fringe; medial aperture open at posterior edge. One pair of needles located near the hooks of pair V. Hooks seven pairs; each with delicate point, depressed truncate thumb, shank comprised of two subunits; proximal subunit expanded, with small terminal nipple (except for hooks of pair I); hook lengths: pair I 20 (19–21; n = 8); pairs II–VII 25 (22–29; n = 8). MCO composed of basally articulated copulatory tube and accessory piece; total length 49 (48–51; n = 7). Copulatory tube a loose coil of one terminal ring, 93 (91–95; n = 6) long; base an elongate oval; shaft following S-shaped path proximally, narrowing to delicate termination. Accessory piece proximally forming membranous skirt around the margin of copulatory tube base; medial portion a folded membrane, cone-shaped, serving as a guide for distal portion; distal portion a complex tongue-shaped structure curved (backwards) along the terminal coil of the copulatory tube. Vagina-like arrow with small arrowhead and long wavy arrow shaft, 71 (67–74; n = 8) long.
Remarks: Dactylogyrus globulatus n. sp. is similar to Dactylogyrus species belonging to the ‘ergensi’ morphological group (Pugachev et al., Reference Pugachev, Gerasev, Gussev, Ergens and Khotenowsky2009) (see above), including D. conchatus n. sp. and D. sagittarius n. sp. The new species most closely resembles D. ergensi and D. conchatus n. sp. – the species possessing a basically cross-shaped ventral bar. While the posterior arm ventral bar is reduced in the new species and D. conchatus n. sp., it is apparently more developed in D. ergensi [according to the drawing of Molnár (Reference Molnár1964)], separating the new species, and also D. conchatus n. sp., from all other species of the ‘ergensi’ group.
On the basis of the comparison with D. ergensi, originally described (and depicted) by Molnár (Reference Molnár1964), the present new species can be distinguished from the latter by the following characters: (i) dorsal anchor with shaft evenly curved to point, and slightly recurved point tip (vs well-differentiated and relatively long point in D. ergensi), (ii) dorsal and ventral haptoral bars of similar width (vs dorsal bar noticeably wider than ventral bar in D. ergensi), (iii) cross-shaped ventral bar with markedly reduced posterior arm and medial aperture open on posterior edge (vs cross-shaped ventral bar with elongate aperture pervading both anterior arm and relatively well-developed posterior arm in D. ergensi), (iv) markedly longer sclerotized part of the vagina (67–74 vs 24–28 μ m in D. ergensi).
The differentiation of D. globulatus n. sp. from D. conchatus n. sp. and D. sagittarius n. sp. is provided in the differential diagnosis for the latter two new species.
Dactylogyrus conchatus n. sp. (Fig. 4)

Fig. 4. Sclerotized structures of Dactylogyrus conchatus n. sp. ex Telestes muticellus. A, anchor; DB, dorsal bar; VB, ventral bar; N, needle; I–VII, hooks; VG, vagina; MCO, male copulatory organ.
urn:lsid:zoobank.org:act:271E1FD9-A859-427C-AC34-771B063FE05F
Type host and locality: Telestes muticellus (Bonaparte, 1837); Melezzo River, Masera (46°08′00.45″N, 08°19′20.51″E).
Other host and locality: Protochondrostoma genei (Bonaparte, 1839); Torrente Cerfone, Le Ville (43°28′42.00″N, 12°04′25.03″E).
Site on host: Gill lamellae.
Type and voucher material: IPCAS M-754 (holotype; one paratype; three vouchers; one hologenophore voucher).
Representative DNA sequences: A nucleotide sequence of partial gene for 28S rRNA (788 bp long; MW443033) and nucleotide sequences representing a fragment (986 bp long; MW443036) including partial gene for 18S rRNA (487 bp), the ITS1 region (493 bp) and 5.8S (6 bp). No intraspecific variability was found (nine specimens were analysed).
Etymology: The specific epithet is derived from Latin (concha = mollusc shell; treated as an adjective) and reflects the snail shell appearance of the distal potion of the MCO accessory piece.
Description (based on four specimens): Body length 542 (470–583; n = 3); greatest width 92 (78–106; n = 3). Haptor with one pair of anchors (dorsal). Anchors with moderately developed roots (inner root longer, terminally flattened); roots forming acute angle; medially slightly constricted bent shaft; point; total length 33 (32–33; n = 4); inner root length 12 (11–13; n = 4); outer root length 4 (4–5; n = 4); point length 9 (8–9; n = 4). Anchor filaments well developed. Dorsal bar 23 (21–24; n = 4) wide, saddle-shaped. Ventral bar 17 (16–17; n = 4) wide, cross-shaped, with anterior arm widened terminally; posterior arm markedly reduced; medial aperture open at posterior edge. One pair of needles located near hooks of pair V. Hooks seven pairs; each with delicate point, depressed truncate thumb, shank comprised of two subunits; proximal subunit expanded; terminal part of proximal subunit sometimes ball-like, with small nipple (except for hook of pair I); hook lengths: pair I 20 (19–20; n = 4); pairs II–VII 26 (22–31; n = 4). MCO comprising copulatory tube and basally articulated accessory piece; total length 47 (45–49; n = 5). Copulatory tube a loose coil of one terminal ring, 92 (90–93; n = 2) long; base an elongate oval; shaft following S-shaped path proximally, narrowing to delicate termination. Accessory piece proximally forming membranous skirt around the margin of copulatory tube base; medial portion a folded membrane, cone-shaped, serving as a guide for distal portion; distal portion a complex tongue-shaped structure curved (backwards) along the terminal coil of the copulatory tube. Vagina-like arrow with small arrowhead and long wavy arrow shaft, 63 (54–74; n = 5) long.
Remarks: Dactylogyrus conchatus n. sp. fits the ‘ergensi’ morphological group (Pugachev et al., Reference Pugachev, Gerasev, Gussev, Ergens and Khotenowsky2009) (see above), including D. globulatus n. sp. and D. sagittarius n. sp. The new species most closely resembles D. ergensi and D. globulatus n. sp. – the species possessing a basically cross-shaped ventral bar. While the posterior arm ventral bar is reduced in the new species and D. globulatus n. sp., it is apparently more developed in D. ergensi [according to the drawing of Molnár (Reference Molnár1964)], separating the new species, and also D. globulatus n. sp., from all other species of the ‘ergensi’ group.
According to the original description (and depiction) of D. ergensi by Molnár (Reference Molnár1964), D. conchatus n. sp. can be distinguished from the latter species by the following characters: (i) cross-shaped ventral bar with markedly reduced posterior arm and with medial aperture open on posterior edge (vs cross-shaped ventral bar with elongate aperture pervading both anterior arm and relatively well-developed posterior arm in D. ergensi), and (ii) markedly longer sclerotized part of the vagina (54–74 vs 24–28 μ m in D. ergensi).
Dactylogyrus conchatus n. sp. can be separated morphologically from D. globulatus n. sp. on the basis of the following characters: (i) dorsal anchor possesses well-differentiated point (vs less differentiated point, with a slightly recurved tip in D. globulatus n. sp.), (ii) dorsal bar is more slender, (iii) anterior arm of ventral bar is widened terminally. Moreover, the D. conchatus n. sp. is also divergent from other congeners at the molecular level.
Differences between D. conchatus n. sp. and D. sagittarius n. sp. are specified in the differential diagnosis for the latter species.
Dactylogyrus sagittarius n. sp. (Fig. 5)

Fig. 5. Sclerotized structures of Dactylogyrus sagittarius n. sp. ex Telestes muticellus. A, anchor; DB, dorsal bar; VB, ventral bar; N, needle; I–VII, hooks; VG, vagina; MCO, male copulatory organ.
urn:lsid:zoobank.org:act:3EB571AF-C1B5-4360-9E7E-CE268C7466B6
Type host and locality: Telestes muticellus (Bonaparte, 1837); Melezzo River, Masera (46°08′00.45″N, 08°19′20.51″E).
Site on host: Gill lamellae.
Type and voucher material: IPCAS M-755 (holotype; one paratype; one hologenophore voucher).
Representative DNA sequences: A nucleotide sequence of partial gene for 28S rRNA (789 bp long; MW443034) and nucleotide sequences representing a fragment (966 bp long; MW443037) including partial gene for 18S rRNA (487 bp), and the ITS1 region (479 bp). No intraspecific variability was found (six specimens were analysed).
Etymology: The specific name, a noun, is from Latin (Sagittarius = the archer armed with arrow), referring to the new species having an arrow-shaped vagina.
Description (based on five specimens): Body length 512 (485–539; n = 2); greatest width 59 (54–64; n = 2). Haptor with one pair of anchors (dorsal). Anchors with moderately developed roots (inner root slightly constricted subterminally); roots (sometimes) forming obtuse angle; elongate curved shaft; short point; total length 34 (33–35; n = 5); inner root length 9 (8–9; n = 5); outer root length 4 (3–4; n = 5); point length 3 (3–4; n = 5). Anchor filaments well developed. Dorsal bar 20 (19–22; n = 5) wide, saddle-shaped. Ventral bar 19 (18–20; n = 4) wide, three-armed, with small medial aperture open on posterior edge. One pair of needles located near hooks of pair V. Hooks seven pairs; each with delicate point, depressed truncate thumb, shank comprised of two subunits; proximal subunit expanded (except for hook of pair I); hook length (pairs I–VII) 21 (15–25; n = 5). MCO composed of basally articulated copulatory tube and accessory piece; total length 37 (35–41; n = 3). Copulatory tube a loose coil of one terminal ring, 71 (70–72; n = 2) long; base an elongate oval; shaft following S-shaped path proximally, narrowing to delicate termination. Accessory piece basically comprising two portions; proximal portion membranous, folded, serving as a guide for distal portion; distal portion a complex tongue-shaped structure curved (backwards) along the terminal coil of the copulatory tube. Vagina-like arrow with small arrowhead and long wavy arrow shaft; blossom-like initial part (opening), 49 (42–55; n = 5) long.
Remarks: Dactylogyrus sagittarius n. sp. resembles Dactylogyrus species belonging to the ‘ergensi’ morphological group (Pugachev et al., Reference Pugachev, Gerasev, Gussev, Ergens and Khotenowsky2009) (see above), including D. globulatus n. sp. and D. conchatus n. sp. The presence of a three-armed ventral bar, together with the absence of a membranous skirt around the margin of the copulatory tube base, allows this species to be distinguished from D. ergensi, D. globulatus n. sp. and D. conchatus n. sp. Dactylogyrus sagittarius n. sp. differs from all the species of the ‘ergensi’ group by the shape of the dorsal anchor (i.e. an anchor having an elongate evenly curved shaft and well-differentiated but very short point).
Dactylogyrus opertus n. sp. (Fig. 6)

Fig. 6. Sclerotized structures of Dactylogyrus opertus n. sp. ex Telestes muticellus. A, anchor; DB, dorsal bar; VB, ventral bar; N, needle; I–VII, hooks; VG, vagina; MCO, male copulatory organ.
urn:lsid:zoobank.org:act:0136688B-7547-436F-8CC7-B66D8473ECE2
Type host and locality: Telestes muticellus (Bonaparte, 1837); Torrente Cerfone, Intoppo (43°26′12.03″N, 11°58′33.00″E).
Site on host: Gill lamellae.
Type and voucher material: IPCAS M-756 (holotype; one paratype; one hologenophore voucher).
Representative DNA sequences: A nucleotide sequence of partial gene for 28S rRNA (790 bp long; MK434964) and nucleotide sequences representing a fragment (984 bp long; MK434944) including partial gene for 18S rRNA (487 bp), the ITS1 region (491 bp) and 5.8S (6 bp). No intraspecific variability was found (four specimens were analysed).
Etymology: The specific name is derived from Latin (opertus = hidden, secret) and refers to the distal part of the copulatory tube enclosed by (covered with) an accessory piece.
Description (based on four specimens): Body length 503 (499–507; n = 2); greatest width 77 (65–89; n = 2). Haptor with one pair of anchors (dorsal). Anchors with moderately developed roots forming acute angle (inner root longer); medially slightly constricted bent shaft; point; total length 38 (37–38; n = 4); inner root length 12 (11–13; n = 4); outer root length 4 (4–5; n = 4); point length 10 (9–10; n = 4). Anchor filaments well developed. Dorsal bar 25 (24–25; n = 4) wide, saddle-shaped. Ventral bar 19 (18–21; n = 4) wide, cross-shaped, with longitudinal aperture; anterior arm widened terminally; anterior and posterior arm each disrupted by elongate longitudinal splits. One pair of needles located near hooks of pair V. Hooks seven pairs; each with delicate point, depressed truncate thumb, shank comprised of two subunits (proximal subunit expanded); hook lengths: pair I 20 (19–21; n = 4); pairs II–VII 25 (22–29; n = 4). MCO composed of copulatory tube and basally articulated accessory piece; total length 33 (33–34; n = 4). Copulatory tube simple, evenly curved. Accessory piece with proximal part forming large flange around copulatory tube base; medial part composed of sclerotized supporting portion (terminating with spherical enlargement) and weakly sclerotized membrane (associated with copulatory tube base); terminal part bluntly finished, enlarged to form sheath directed backwards and enclosing the distal part of the copulatory tube. Vagina-like arrow with bent arrow shaft (shaft margins irregular); 16 (12–20; n = 4) long.
Remarks: Dactylogyrus opertus n. sp. was initially included in the study by Benovics et al. (Reference Benovics, Desdevises, Šanda, Vukić and Šimková2020) as Dactylogyrus sp. 7. Dactylogyrus opertus n. sp. was classified into the ‘nanus’ morphological group, showing a close resemblance to D. nanus, D. nanoides and D. suecicus (Pugachev et al., Reference Pugachev, Gerasev, Gussev, Ergens and Khotenowsky2009) (see above). However, the new species differs from all species of the ‘nanus’ group mentioned above by possessing a cross-shaped ventral bar [the ventral bar is three-armed in D. nanus, D. nanoides and D. suecicus (the anterior arm divided into horn-like ends in D. suecicus)]. Further, the D. opertus n. sp. accessory piece of the MCO in D. opertus n. sp. is distinctive, having a bluntly finished terminal part, enlarged to form a sheath directed backwards and enclosing (covering) the distal part of the copulatory tube (in D. nanus, D. nanoides and D. suecicus, the distal part of the accessory piece is simply enlarged to form a sheath supporting the distal part of the copulatory tube in D. nanus, D. nanoides and D. suecicus).
The presence of a cross-shaped ventral bar makes D. opertus n. sp. similar to other species of the ‘nanus’ group, i.e. to D. rutili Glaeser, 1965 and D. distinguendus Nybelin, 1937. However, these two species, in contrast to the new species, belong to the species of the ‘nanus’ group having a proximally sharply curved copulatory tube and an elongate (S- or C-shaped) vagina (Pugachev et al., Reference Pugachev, Gerasev, Gussev, Ergens and Khotenowsky2009). While in D. opertus n. sp., the posterior arm of the ventral bar is of similar length to (or longer than) the transverse arm in D. opertus n. sp., the ventral bar in D. rutili and D. distinguendus has a short posterior arm in D. rutili and D. distinguendus (i.e. a posterior arm shorter than the transverse arm; Pugachev et al., Reference Pugachev, Gerasev, Gussev, Ergens and Khotenowsky2009). Dactylogyrus opertus n. sp. also resembles D. sandai Řehulková, Benovics et Šimková, Reference Řehulková, Benovics and Šimková2020, a species recently described from Balkan cyprinoids and displaying close similarity with respect to the basic morphology of both the haptoral and reproductive structures (Řehulková et al., Reference Řehulková, Benovics and Šimková2020). The anchors of the new species possess slightly more elongated points, and roots differ even more in their lengths. The anterior arm of the connective ventral bar in D. opertus n. sp. is also wider in comparison to D. sandai. Moreover, the terminal part of the MCO of D. opertus n. sp. forms a sheath pointing backwards and covering the distal part of the copulatory tube, while a similar structure in D. sandai does not enclose the copulatory tube but points forward.
Phylogenetic position of the newly described Dactylogyrus species
The final concatenated alignment of the DNA sequences of the 42 Dactylogyrus species studied here spanned 1787 unambiguously aligned nucleotide positions (446 bp for 18S, 704 bp for 28S and 637 bp for ITS1). Both phylogenetic analyses (ML and BI) yielded trees with identical topologies, differing only in their node support values (see tree from BI in Fig. 7).

Fig. 7. Phylogenetic tree of 41 Dactylogyrus species from European cyprinoids resulting from BI analysis. The tree is based on concatenated partial sequences of genes coding for 18S rRNA and 28S rRNA, and ITS1 region. Numbers along branches represent posterior probabilities (>0.80) and bootstrap support values (>50) for individual nodes, resulting from BI and ML analyses, respectively. Lower values are shown as dashes (–). Length of the branches represents the number of substitutions per site. Newly described Dactylogyrus species are shown in red colour.
The phylogenetic analyses revealed that the newly-described Dactylogyrus species are associated with two phylogenetic lineages. Dactylogyrus opertus n. sp. is phylogenetically close to the recently described D. sandai – an endemic species of the Balkan Peninsula – and nested within a clade containing the morphologically similar species D. nanus, D. rutili and D. suecicus. All other new species, i.e. D. conchatus n. sp., D. globulatus n. sp. and D. sagittarius n. sp., are nested within the phylogenetic lineage that includes species with the ‘ergensi’ type of copulatory organ (i.e. D. ergensi, D. dirigerus, D. caucasicus and D. tissensis).
Discussion
The species richness of a parasite community in fish usually reflects the effects of numerous abiotic factors related to the environment, the biology of the host and the diversity of the communities of syntopic organisms serving as intermediate hosts for many fish endoparasites (e.g. Gregory, Reference Gregory1990; Landsberg et al., Reference Landsberg, Blakesley, Reese, McRae and Forstchen1998; Poulin, Reference Poulin2007). One of the less obvious factors is the introduction of non-native fish into a new region, as introduced fish may also serve as vectors for the co-introduction of non-native parasites (especially monogeneans, e.g. Havlátová et al., Reference Havlátová, Ondračková and Přikrylová2015; Benovics et al., Reference Benovics, Kičinjaová and Šimková2017, Reference Benovics, Desdevises, Vukić, Šanda and Šimková2018, Reference Benovics, Desdevises, Šanda, Vukić and Šimková2020; Šimková et al., Reference Šimková, Řehulkova, Jean Robertin, Jorissen, Scholz, Faltynková, Mašová and Vanhove2018; Kmentová et al., Reference Kmentová, Van Steenberge, van den Audenaerde, Nhiwatiwa, Bukinga, N'sibula, Mulungula, Gelnar and Vanhove2019; Wilson et al., Reference Wilson, Saunders and Hutson2019), which may represent a substantial threat to native host populations (Lymbery et al., Reference Lymbery, Morine, Kanani, Beatty and Morgan2014). Since current Italian freshwater fauna includes numerous non-native species (Bianco, Reference Bianco1995), it is tempting to expect that non-native parasites, co-introduced with their non-native host species, will also be present on endemic cyprinoids.
In the present study, we investigated ten species of endemic Italian cyprinoids from six sites in the northern part of the Apennine peninsula for their helminth parasites. The highest parasite diversity was observed among monogeneans, which are a group of ectoparasitic platyhelminths usually parasitizing either on the gills (Gyrodactylus, Dactylogyrus and Paradiplozoon), or on the skin (Gyrodactylus) of fish. A total of 20 Dactylogyrus species were identified, exceeding the number of species reported in the checklist of Galli et al. (Reference Galli, Strona, Benzoni, Crosa and Stefani2007). Herein, we reported 15 Dactylogyrus species that were not previously documented in Italy. Historically, Italian cyprinoids have not been comprehensively investigated for the presence of monogenean parasites. Before 2002, only eight monogenean species had been reported (Molnár and Ghittino, Reference Molnár and Ghittino1977; Bona et al., Reference Bona, Buriola, Cerioni, Orecchia, Paggi, Minelli, Ruffo and La Posta1995). Some efforts to investigate the diversity of monogeneans came after the studies of Galli et al. (Reference Galli, Stefani, Zaccara and Crosa2002, Reference Galli, Stefani, Benzoni and Zullini2005, Reference Galli, Strona, Benzoni, Crosa and Stefani2007); however, endemic species were tackled only marginally, since studies were focused on investigating the parasite fauna of either invasive species [e.g. C. gibelio and Cyprinus carpio L.], or species with a wide distribution range across Europe [e.g. Abramis brama, Squalius cephalus (Linnaeus, 1758) or Scardinius erythrophthalmus (Linnaeus, 1758)]. The finding, in our study, of D. achmerowi, D. falciformis and D. vastator on endemic B. plebejus from the Po River is very unexpected [the presence of D. vastator on B. plebejus was also reported in the earlier work of Benovics et al. (Reference Benovics, Kičinjaová and Šimková2017)]. All three parasite species are host-specific to C. carpio and C. gibelio across their whole distribution range (e.g. Moravec, Reference Moravec2001; Galli et al., Reference Galli, Stefani, Zaccara and Crosa2002; Jalali and Barzegar, Reference Jalali and Barzegar2005; Šimková et al., Reference Šimková, Verneau, Gelnar and Morand2006, Reference Šimková, Dávidová, Papoušek and Vetešník2013; Ling et al., Reference Ling, Tu, Huang and Wang2016). Their presence on B. plebejus, an endemic species of the Padano-Venetian ichthyogeographical district (sensu Bianco, Reference Bianco1990), may be a result of the introduction of the abovementioned alien species into the Apennines and the subsequent host-switch to endemic and phylogenetically close Barbus (all host species belonging to Cyprinidae). The presence of non-native D. vastator on the endemic Balkan Aulopyge huegelii Heckel, 1843 was previously reported by Benovics et al. (Reference Benovics, Kičinjaová and Šimková2017) and also explained by the host switch from C. carpio or C. gibelio. However, the alien Dactylogyrus species were not observed on B. tyberinus; therefore, we can postulate that host-switching occurs only in water bodies where congeneric Barbus lives in sympatry with introduced cyprinids, such as the Po River, where C. carpio and C. gibelio are commonly distributed (Galli et al., Reference Galli, Strona, Benzoni, Crosa and Stefani2007; Kottelat and Freyhof, Reference Kottelat and Freyhof2007).
The most common Dactylogyrus species on endemic cyprinoids was D. vistulae (present on 60% of investigated species), which is considered as a true generalist species with the widest host range in Europe (Moravec, Reference Moravec2001; Šimková et al., Reference Šimková, Verneau, Gelnar and Morand2006; Benovics et al., Reference Benovics, Desdevises, Vukić, Šanda and Šimková2018, Reference Benovics, Desdevises, Šanda, Vukić and Šimková2020). Herein, we present B. plebejus as a new host of D. vistulae, increasing its host range to 34 cyprinoid species. In addition to already known Dactylogyrus species, we reported and described four new species from Apennine leuciscids. While D. globulatus n. sp., D. opertus n. sp. and D. sagittarius n. sp. appear to be host-specific to C. soetta (D. globulatus n. sp.) and T. muticellus (the latter two species), D. conchatus n. sp. was reported from two leuciscid species – T. muticellus and P. genei. The morphological similarity of D. globulatus n. sp., D. conchatus n. sp. and D. sagittarius n. sp. in the shape of their copulatory organs, and the fact that the only difference among them is in the shape of their haptoral elements suggest their common evolutionary origin (illustrations of the sclerotized structures directly taken from hologenophores are shown for comparison in Fig. 8). These endemic species most likely share a common ancestor with other species of the ‘ergensi’ morphological group, and evolved in the Apennines by subsequent intra-host duplication on T. muticellus (or its ancestor). The common origin of these three species and other species from the ‘ergensi’ morphological group (i.e. D. caucasicus, D. dirigerus, D. ergensi and D. tissensis) is also supported by our phylogenetic analyses. Endemic species most likely diverged and underwent speciation after the dispersion of their ancestor into the Apennine Peninsula. The differences in the haptoral elements might be adaptations for attaching to different substrates on different parts of the gill arch (i.e. microhabitats) of the same host species after intra-host speciation [morphological adaptations in the forms of monogenean haptors were suggested by Rohde (Reference Rohde1979) and Šimková et al. (Reference Šimková, Desdevises, Gelnar and Morand2000, Reference Šimková, Ondračková, Gelnar and Morand2002)]. A different evolutionary origin may be postulated for D. opertus n. sp., which more resembles, on the basis of morphology, species parasitizing on Squalius, e.g. D. nanus, D. nanoides and D. suecicus (see Pugachev et al., Reference Pugachev, Gerasev, Gussev, Ergens and Khotenowsky2009 for morphotypes). Dactylogyrus opertus n. sp. [initially included as Dactylogyrus sp. 7 in the phylogenetic study by Benovics et al. (Reference Benovics, Desdevises, Šanda, Vukić and Šimková2020)] was found to be phylogenetically close to recently described D. sandai [identical with Dactylogyrus sp. 6 included in the study of Benovics et al. (Reference Benovics, Desdevises, Šanda, Vukić and Šimková2020)], a species from Telestes in the Balkans. The study of Benovics et al. (Reference Benovics, Desdevises, Šanda, Vukić and Šimková2020) also included a third species from Telestes (Dactylogyrus sp. 8 from T. montenigrinus), which has not yet been described; according to their study, all three Dactylogyrus species have a common evolutionary origin. Moreover, the present study confirmed the close morphological similarity between D. sandai and D. opertus n. sp. We can assume that these two species (probably also Dactylogyrus sp. 8 – a species of clade 6 in Fig. 1, Benovics et al., Reference Benovics, Desdevises, Šanda, Vukić and Šimková2020) are genus-specific to Telestes (more specifically, they parasitize T. karsticus and T. muticellus, respectively). The two Telestes species are recently distributed over the Padano-Venetian ichthyogeographic district and, according to Buj et al. (Reference Buj, Marčić, Ćaleta, Šanda, Geiger, Freyhof, Machordom and Vukić2017), they share a common ancestor in the Balkans, which dispersed into the northern part of the peninsula and subsequently diverged into the Apennines. Therefore, we can assume that the evolutionary histories of host-specific D. sandai and D. opertus n. sp. are intimately linked with their Telestes hosts and that these Dactylogyrus species originated from cospeciation.

Fig. 8. Sclerotized structures of three new Dactylogyrus species. A, anchor; DB, dorsal bar; VB, ventral bar; MCO, male copulatory organ.
A total of 14 Gyrodactylus species were reported in this study, of which the most prevalent were G. katharineri and G. laevis. Both species exhibit a rather wide host range without obvious specificity to some hosts (each of them was reported from four Apennine cyprinoid species). They can be harboured by cyprinids, leuciscids and also gobiids (e.g. Gutiérrez-Galindo and Lacasa-Millán, Reference Gutiérrez-Galindo and Lacasa-Millán2001; Moravec, Reference Moravec2001; Harris et al., Reference Harris, Shinn, Cable and Bakke2004; Djikanovic et al., Reference Djikanovic, Paunovic, Nikolic, Simonovic and Cakic2012). Gyrodactylus cf. markewitschi was isolated from Barbus caninus from the Malezzo River. This species resembles G. markewitschi, which appears to be specific to the western Palearctic Barbus (Kakacheva-Avramova, Reference Kakacheva-Avramova1973; Moravec, Reference Moravec2001; Harris et al., Reference Harris, Shinn, Cable and Bakke2004), with some accidental infections reported from percids (Angelescu, Reference Angelescu1974), or carp (Hussain et al., Reference Hussain, Mhaisen and Al-Rubaie2007). Therefore, G. cf. markewitschi might represent a species derived from G. markewitschi, endemic for the Apennine Peninsula. Unfortunately, to validate our hypothesis of the evolutionary proximity of these two species, molecular data for G. markewitschi would be required; however, these are still missing. The only locality where no Gyrodactylus parasites were recorded was Carmagnola, comprising gravel pits of relatively recent origin, filled with water from the Po River. Potential changes in abiotic factors from the river stream to the gravel pits (e.g. water current or temperature) may putatively have influenced the populations of gyrodactylid parasites and facilitated their reduction. For example, water temperature has a significant influence on parasite communities; therefore, the different water temperatures at the different sites in this study most probably influenced gyrodactylid communities to different degrees. Temperature directly affects the reproduction and survival time of Gyrodactylus (Jansen and Bakke, Reference Jansen and Bakke1991; Bakke et al., Reference Bakke, Cable and Harris2007); during summer months, the intensity of gyrodactylids on hosts tends to decline (e.g. Koskivaara et al., Reference Koskivaara, Valtonen and Prost1991; Blažek et al., Reference Blažek, Jarkovský, Koubková and Gelnar2008). The water in gravel pits is captured from the Po River but, due to its stationariness, reaches a higher temperature than the running water of the River, which (considering studies above) might impact the parasite communities.
This study provides evidence that phylogenetically-close cyprinoid species from the same locality share generalist monogenean species. For example, all leuciscid species from Torrente Cerfone (i.e. P. genei, R. rubilio, S. lucumonis, S. squalus and T. muticellus) were parasitized by D. vistulae. Moreover, all abovementioned leuciscids, except T. muticellus, were also hosts of P. megan, suggesting that this diplozoid species does not exhibit host specificity in the investigated region, but rather a geographical specificity, which is in concordance with the previous reports of P. megan in cyprinoids (Benovics et al., Reference Benovics, Koubková, Civáňová, Rahmouni, Čermáková and Šimková2021).
The fauna of intestinal helminths in the herein investigated cyprinoid species was species poorer in comparison to ectoparasitic monogeneans, and included only one species of eye fluke (metacercariae of D. spathaceum), three species of nematodes and one species of cestode. Among them, the most remarkable record is B. rectangulum, a tapeworm species from both investigated Barbus spp. from two geographically distant localities. While this representative of the monotypic genus Bathybothrium is relatively common in Barbinae distributed in the Palearctic region (Lühe, Reference Lühe1910; Joyeux and Baer, Reference Joyeux and Baer1936; Protasova, Reference Protasova and Ryzhikov1977; Moravec and Amin, Reference Moravec and Amin1978; Van Maren, Reference van Maren1979; Dubinina, Reference Dubinina and Bauer1987; Scholz, Reference Scholz1989; Moravec, Reference Moravec2001), prior to this date there was no evidence of this cestode species in the Apennine Peninsula. The common host of B. rectangulum is B. barbus (e.g. Scholz and Moravec, Reference Scholz and Moravec1996; Moravec, Reference Moravec2001), the Barbus species with the widest distribution range in Europe (Kottelat and Freyhof, Reference Kottelat and Freyhof2007). Bathybothrium rectangulum reached relatively high prevalence and abundance in B. barbus (e.g. Laimgruber et al., Reference Laimgruber, Schludermann, Konecny and Chovanec2005; Chunchukova and Kirin, Reference Chunchukova and Kirin2018), and this widely distributed host species may have served as a potential vector for the distribution of B. rectangulum into different European regions. A more thorough ichthyoparasitological investigation in other southern European Peninsulas may potentially reveal other new hosts for this remarkable parasite species.
Conclusions
Even though the Apennine Peninsula represents a region of high interest regarding ichthyological research, from the parasitological point of view, it remains underexplored, especially with respect to the parasites of endemic fish species. After more than a decade, we present the first study focused on the parasite fauna of endemic cyprinoids in northern Italy, and described four new monogenean species. We also found that local parasite communities appear to be influenced by the continual introduction of non-native fish into the region, potentially threatening native endemic species that are already undergoing reductions in their population sizes.
Supplementary material
The supplementary material for this article can be found at https://doi.org/10.1017/S0031182021000615.
Data
The data supporting the conclusions of this study are included in this article. The type-material of the new species described in this study was deposited in the Helminthological Collection of the Institute of Parasitology, Czech Academy of Sciences, České Budĕjovice, Czech Republic under the accession number IPCAS M-753–M-756. The newly generated sequences were submitted to the GenBank database under accession numbers MW443031–MW443037.
Acknowledgements
We are grateful to Kateřina Čermáková, Jaroslav Červenka, Maria Lujza Červenka Kičinja and Petra Zahradníčková for their help with fish dissection and parasite collection, and to Šárka Mašová and Tomáš Scholz with the identification of intestinal helminths. We are also grateful to Jasna Vukić, Radek Šanda, Stefano Porcelloti and Paolo Sala for arranging permits, and for fish collection in the field. We kindly thank Matthew Nicholls for the English revision of the final draft.
Author contributions
A.Š. designed and supervised the study. P.V. collected and identified fish in the field. A.Š. and M.B. processed fish and collected parasites during the field trip. M.B., K.F. and V.D. performed microscopical observations, and determination of parasite species. M.B. performed all laboratory procedures, and phylogenetic and statistical analyses. K.F. prepared descriptions of the new species. M.B. wrote the draft of the manuscript. A.Š., K.F. and P.V. revised the manuscript. All authors read and approved the final version of the manuscript.
Financial support
The field study was financially supported by the Czech Science Foundation (project number GA15-19382S). From 2020, the study was funded by the Czech Science Foundation (project number GA20-13539S).
Conflict of interest
None.
Ethical standards
All applicable institutional, national and international guidelines for the care and use of animals were followed. This study was approved by the Animal Care and Use Committee of the Faculty of Science, Masaryk University in Brno (Czech Republic).