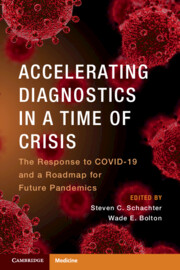
Book contents
- Accelerating Diagnostics in a Time of Crisis
- Accelerating Diagnostics in a Time of Crisis
- Copyright page
- Dedication
- Contents
- Contributors
- Foreword
- Preface
- Acknowledgments
- Introduction
- Chapter 1 Early Detection, Response, and Surveillance of the COVID-19 Pandemic Crisis
- Chapter 2 Immunology of COVID-19 and Ineffective Immunity
- Chapter 3 Clinical Management: A Roadmap Based on One New York City Hospital’s Response to the COVID-19 Pandemic
- Chapter 4 Contribution of RADx® Tech to the Rapid Development of COVID-19 Diagnostic Tests
- Chapter 5 Coordination of Resources for the Manufacturing and Deployment of COVID-19 Diagnostic Assays
- Chapter 6 Quality and Risk Management Processes for Diagnostic Assays during an Emergency Pandemic Response
- Chapter 7 Development of Assays to Diagnose COVID-19
- Chapter 8 Laboratory Verification and Clinical Validation of COVID-19 Diagnostic Assays
- Chapter 9 Importance of Timely Sequencing, Tracking, and Surveillance of Emergent Variants
- Chapter 10 The RADx® Regulatory Core and Its Role in COVID-19 Emergency Use Authorizations
- Chapter 11 Commercialization and Market Assessment of COVID-19 Assays
- Chapter 12 Testing Strategies to Mitigate COVID-19 Disease Spread
- Chapter 13 A Pandemic Not Just of Infection but of Inequality: The Social Impact of COVID-19
- Chapter 14 Summary and Path Forward for Future Pandemics
- Index
- References
Chapter 9 - Importance of Timely Sequencing, Tracking, and Surveillance of Emergent Variants
Published online by Cambridge University Press: 06 January 2024
- Accelerating Diagnostics in a Time of Crisis
- Accelerating Diagnostics in a Time of Crisis
- Copyright page
- Dedication
- Contents
- Contributors
- Foreword
- Preface
- Acknowledgments
- Introduction
- Chapter 1 Early Detection, Response, and Surveillance of the COVID-19 Pandemic Crisis
- Chapter 2 Immunology of COVID-19 and Ineffective Immunity
- Chapter 3 Clinical Management: A Roadmap Based on One New York City Hospital’s Response to the COVID-19 Pandemic
- Chapter 4 Contribution of RADx® Tech to the Rapid Development of COVID-19 Diagnostic Tests
- Chapter 5 Coordination of Resources for the Manufacturing and Deployment of COVID-19 Diagnostic Assays
- Chapter 6 Quality and Risk Management Processes for Diagnostic Assays during an Emergency Pandemic Response
- Chapter 7 Development of Assays to Diagnose COVID-19
- Chapter 8 Laboratory Verification and Clinical Validation of COVID-19 Diagnostic Assays
- Chapter 9 Importance of Timely Sequencing, Tracking, and Surveillance of Emergent Variants
- Chapter 10 The RADx® Regulatory Core and Its Role in COVID-19 Emergency Use Authorizations
- Chapter 11 Commercialization and Market Assessment of COVID-19 Assays
- Chapter 12 Testing Strategies to Mitigate COVID-19 Disease Spread
- Chapter 13 A Pandemic Not Just of Infection but of Inequality: The Social Impact of COVID-19
- Chapter 14 Summary and Path Forward for Future Pandemics
- Index
- References
Summary
Severe acute respiratory syndrome coronavirus 2 (SARS-CoV-2) evolution has given rise to new variants: viruses with different sets of mutations, some of which have increased transmissibility and immune escape. Detecting and monitoring new variants was made possible by intensive, global genomic surveillance efforts, including sequencing over 1.2 million SARS-CoV-2 genomes in the first pandemic year. Crucial to these efforts was the Rapid Acceleration of Diagnostics (RADx®) Variant Task Force, which was established in 2021 to monitor variants and assess the efficacy of RADx technologies against new variants. Major accomplishments of the RADx Variant Task Force include the establishment of the ROSALIND Diagnostic Monitoring system to identify mutations, the creation of an extensive biobank of sequenced samples, laboratory testing of RADx technologies against new variants as they emerged, and epitope mapping to identify mutations likely to affect an assay’s performance. Key lessons learned include (1) the need for very early establishment of pathogen sequencing, analysis, and data sharing; (2) the importance of both in silico and wet laboratory assessment of diagnostic assays; and (3) the benefits of interdisciplinary collaboration of government, academia, and industry.
Keywords
- Type
- Chapter
- Information
- Accelerating Diagnostics in a Time of CrisisThe Response to COVID-19 and a Roadmap for Future Pandemics, pp. 166 - 193Publisher: Cambridge University PressPrint publication year: 2024