Book contents
- The Cambridge Handbook of the Learning Sciences
- The Cambridge Handbook of the Learning Sciences
- Copyright page
- Contents
- Figures
- Tables
- Contributors
- Preface
- 1 An Introduction to the Learning Sciences
- Part I Foundations
- Part II Methodologies
- Part III Grounding Technology in the Learning Sciences
- Part IV Learning Together
- Part V Learning Disciplinary Knowledge
- Part VI Moving Learning Sciences Research into the Classroom
- Index
- References
Part I - Foundations
Published online by Cambridge University Press: 14 March 2022
- The Cambridge Handbook of the Learning Sciences
- The Cambridge Handbook of the Learning Sciences
- Copyright page
- Contents
- Figures
- Tables
- Contributors
- Preface
- 1 An Introduction to the Learning Sciences
- Part I Foundations
- Part II Methodologies
- Part III Grounding Technology in the Learning Sciences
- Part IV Learning Together
- Part V Learning Disciplinary Knowledge
- Part VI Moving Learning Sciences Research into the Classroom
- Index
- References
Summary
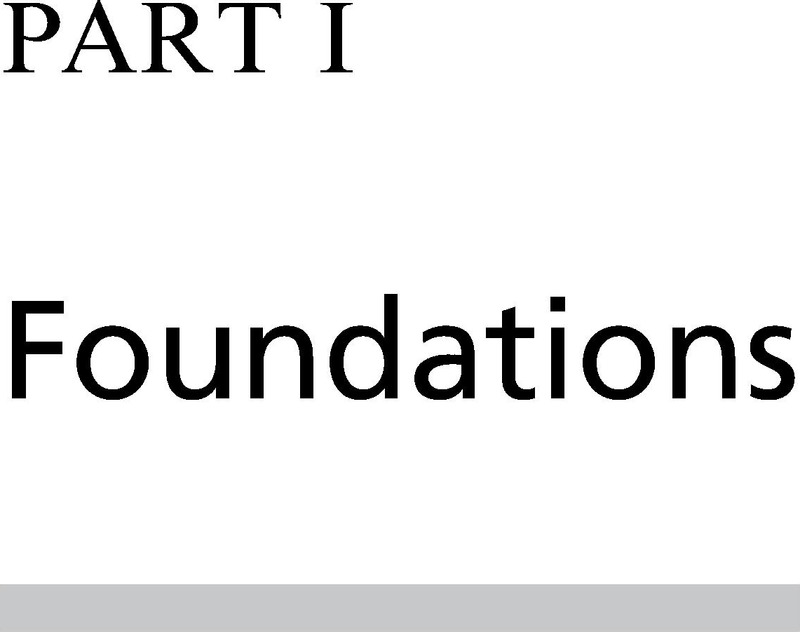
- Type
- Chapter
- Information
- The Cambridge Handbook of the Learning Sciences , pp. 25 - 174Publisher: Cambridge University PressPrint publication year: 2022