Book contents
- Liquid Cell Electron Microscopy
- Advances in Microscopy and Microanalysis
- Liquid Cell Electron Microscopy
- Copyright page
- Contents
- Contributors
- Preface and Acknowledgements
- Part I Technique
- Part II Applications
- 9 Nanostructure Growth, Interactions, and Assembly in the Liquid Phase
- 10 Quantifying Electrochemical Processes Using Liquid Cell TEM
- 11 Application of Electrochemical Liquid Cells for Electrical Energy Storage and Conversion Studies
- 12 Applications of Liquid Cell TEM in Corrosion Science
- 13 Nanoscale Water Imaged by In Situ TEM
- 14 Nanoscale Deposition and Etching of Materials Using Focused Electron Beams and Liquid Reactants
- 15 Liquid Cell TEM for Studying Environmental and Biological Mineral Systems
- 16 Liquid STEM for Studying Biological Function in Whole Cells
- 17 Visualizing Macromolecules in Liquid at the Nanoscale
- 18 Application of Liquid Cell Microscopy to Study Function of Muscle Proteins
- Part III Prospects
- Index
- References
9 - Nanostructure Growth, Interactions, and Assembly in the Liquid Phase
from Part II - Applications
Published online by Cambridge University Press: 22 December 2016
- Liquid Cell Electron Microscopy
- Advances in Microscopy and Microanalysis
- Liquid Cell Electron Microscopy
- Copyright page
- Contents
- Contributors
- Preface and Acknowledgements
- Part I Technique
- Part II Applications
- 9 Nanostructure Growth, Interactions, and Assembly in the Liquid Phase
- 10 Quantifying Electrochemical Processes Using Liquid Cell TEM
- 11 Application of Electrochemical Liquid Cells for Electrical Energy Storage and Conversion Studies
- 12 Applications of Liquid Cell TEM in Corrosion Science
- 13 Nanoscale Water Imaged by In Situ TEM
- 14 Nanoscale Deposition and Etching of Materials Using Focused Electron Beams and Liquid Reactants
- 15 Liquid Cell TEM for Studying Environmental and Biological Mineral Systems
- 16 Liquid STEM for Studying Biological Function in Whole Cells
- 17 Visualizing Macromolecules in Liquid at the Nanoscale
- 18 Application of Liquid Cell Microscopy to Study Function of Muscle Proteins
- Part III Prospects
- Index
- References
Summary
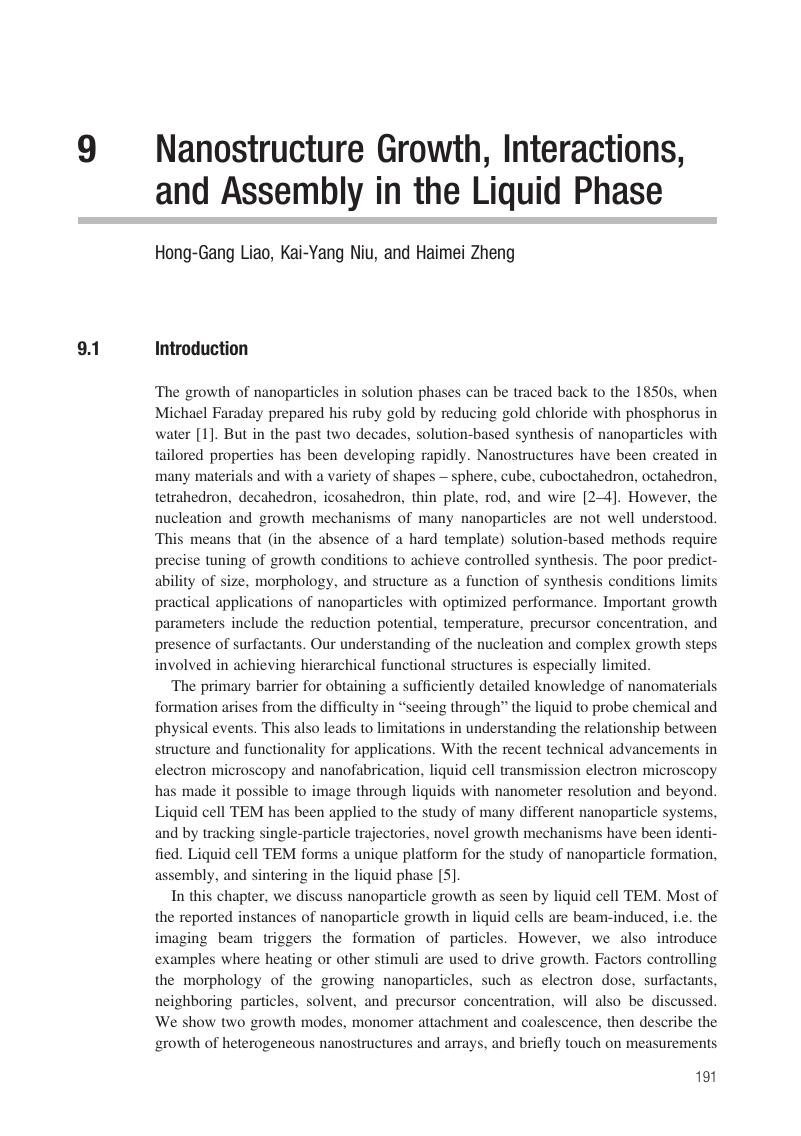
- Type
- Chapter
- Information
- Liquid Cell Electron Microscopy , pp. 191 - 209Publisher: Cambridge University PressPrint publication year: 2016
References
- 1
- Cited by