Introduction
Ice cores record information on climate system components at a high resolution and in a stratigraphic sequence, even providing direct access to entrapped atmospheric paleo air. Accordingly, ice cores are among the most suitable archives to access paleoclimate information. Significant attention focuses on the recovery of ice-core climate records that extend back in time beyond the currently oldest stratigraphic ice-core record of 800 ka (EPICA community members, 2004) to over 1 Ma (Dahl-Jensen, Reference Dahl-Jensen2018). Sites with potentially undisturbed ice records older than 1.0 Ma in Antarctica should have little ice-flow, be sufficiently thick (but not excessively thick), and exhibit super cold temperatures (Van Liefferinge and others, Reference Van Liefferinge2018). Previous studies indicate that drill sites near ice divides with smooth bedrock topography are favourable, which suggests that dome areas are ideal locations (Dahl-Jensen, Reference Dahl-Jensen2018). However, Bell and others (Reference Bell2011) proposed two diametrically opposed views: (1) the basal ice uplift caused by accretion moves older ice to a higher position in the ice sheet, increasing the potential for the preservation of very old ice in the ice sheet; and (2) the extensive melting necessary to support the freezing process may destroy ice containing paleoclimate records. Recently, Zhao and others (Reference Zhao, Moore, Sun, Tang and Guo2018) constrained the range of basal ages at the Kunlun drilling site to approximately 649−831 ka, with dated radar isochrones to a 2/3 ice depth, the surface vertical velocity, and also the spatial variability in radar isochrones dated to 153.3 ka BP. Regardless, drilling to obtain and date ice cores is necessary to reveal its exact age.
The 10 m firn temperature estimates the annual mean air temperature (AMAT) (Loewe, Reference Loewe1970) within 1%. During the 2010/2011 season, a temperature of −58.6°C was recorded at Dome A (see Fig. 1). This is the lowest recorded temperature among the available AMAT records across the Antarctic continent. In addition, the annual snow accumulation rate is approximately 23 mm water equivalent a–1, which is the lowest reported for inland Antarctic areas (see Fig. 1) (Hou and others, Reference Hou, Li, Xiao and Ren2007; Xiao and others, Reference Xiao2008). The surface velocity in the area of approximately 40 km around Dome A ranges from 31 ± 26 to 294 ± 12 mm a–1, with a mean of 111 ± 24 mm a–1 (Yang and others, Reference Yang2014), which is among the lowest observed values in Antarctica.
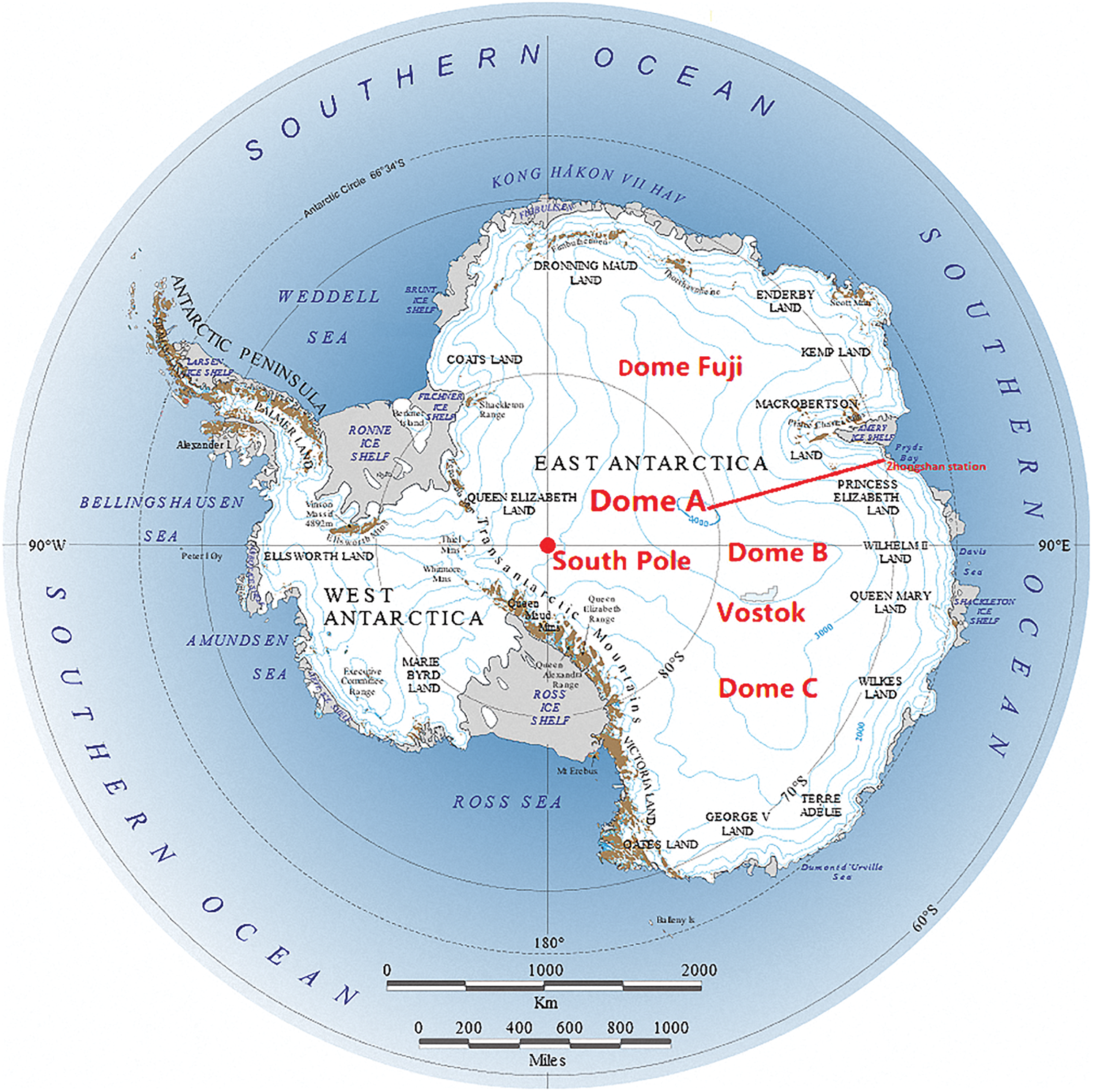
Fig. 1. Geographic location of Dome A, located 1260 km inland, edited from the Landsat Image Mosaic of Antarctica Project (https://lima.usgs.gov/download.php). The annual average temperature of Dome B, Dome C, Dome F, Vostok, and the South Pole are −57.4, −53.5, −54.3, −55.5, and −49.0°C, respectively. The snow accumulation rates are 38, 34, 32, 23, and > 60 mm water equivalent a–1, respectively (Jouzel and others, Reference Jouzel1995; Masson and others, Reference Masson2000; Ekaykin and others, Reference Ekaykin, Lipenkov, Barkov, Petit and Masson-Delmotte2002; Watanabe and others, Reference Watanabe2003; Ekaykin and others, Reference Ekaykin2004). The annual average temperature and snow accumulation rates at Dome A are −58.6°C and 23 mm water equivalent a–1, respectively. The original image can be found at https://lima.usgs.gov/ and details from it can be found here or from https://commons.wikimedia.org/wiki/File:Antarctica.svg.
Searching for the oldest ice core, with a 1.5 Ma record of climate and greenhouse gases, is one of the priority projects within the International Partnerships in Ice Core Sciences (Fischer and others, Reference Fischer2013) and was proposed during two initial workshops in 2004 and 2005. To address this challenge, China established the Kunlun station at Dome A in January 2009; the deep ice-core drilling site was selected with limited initial knowledge. The primary scientific aim of the project was to extend the available climate records from deep ice cores and investigate the key mechanisms of climate change and subglacial processes. According to advanced information collected since the start of drilling, the estimated age of the oldest ice at the current Dome A drilling site reduced to approximately 800 ka (Sun and others, Reference Sun2014; Zhao and others, Reference Zhao, Moore, Sun, Tang and Guo2018).
Herein, the collected parameters are presented, in addition to information on drilling activities completed as of 2017. During campaigns between 2009 and 2017, the drill site was constructed, pilot hole was drilled, and casing was installed. The deep ice coring drill was then assembled and deep ice coring commenced. During 2016/2017, a team from the Polar Research Institute of China, Jilin University, Nanjing University, and Northwest Institute of Ecology and Environmental Resources, Chinese Academy of Sciences, collected a deep ice core at Dome A from a depth of 800.2 m (Fig. 2).

Fig. 2. Ice core from a depth of 800 m during the 2016/2017 drilling season.
Operation procedure for Chinese deep ice-core drilling
The drilling system, based on the JARE concept (Takahashi and others, Reference Takahashi2001; Fuji and others, Reference Fuji2002), is largely made to the length of the core barrels and chip chamber with adjustments compared with the JARE drill. It is mainly composed of the following eight parts: ice-core drill, winch, mast, control panel, data sending unit/data relay unit, reamer, chip recovering tool, and cable changing brake. The details of the drilling system have previously been described in Zhang and others (Reference Zhang2014).
Logistic support
The CHINARE inland expedition team typically sails to Antarctica aboard the ‘MV Xue Long,’ which is the first Chinese ice-breaking polar research vessel. The Xue Long provides annual supplies and transport for the expedition team to Zhongshan station in late November or early December. The inland expedition team typically stays at Zhongshan for approximately 15 days to sort supplies and complete vehicle maintenance. After preparation of the inland traverse cargo, the team travels to Dome A. The trip from Zhongshan to Kunlun station usually takes a fortnight. The team does not leave Kunlun Station until the temperature remains below −40°C for three consecutive days. The team commonly leaves Kunlun at the end of January for personnel and vehicle safety. Accordingly, there are only 20−30 days available at Kunlun station during each season due to the extremely harsh climate.
The most important task for the Chinese inland expedition team is the deep ice coring operation at Kunlun station. The drilling operation is characterised by phases of preparation, drilling, ice-core processing, and tail-in work.
Drilling preparation
The deep ice-core drilling operation workshop is located underground, 3 m below the snow surface. After one year of snow accumulation, the ramp of the drilling trench becomes buried (Fig. 3a). It is impossible to clean the passage within a few days using manual labour; therefore, the accumulated snow on the ramp is cleared with a snow-blower (Fig. 3b).
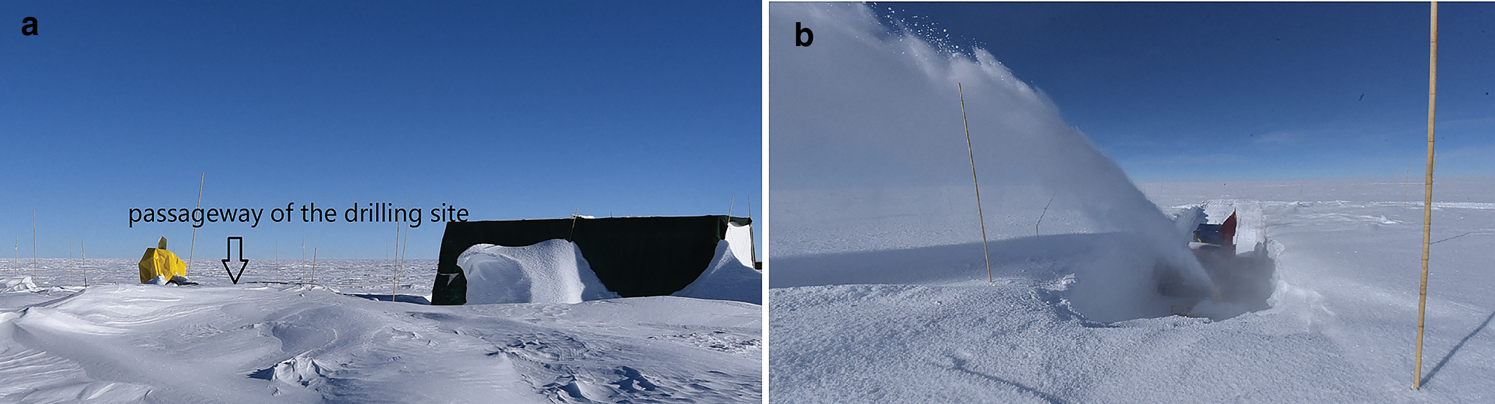
Fig. 3. Opening the ramp with a snow-blower.
Currently, Kunlun station only remains operational in summer, and the first task for the expedition team is to provide power for the drilling, illumination, and heating systems. The control cabinet and drilling trench have to be warmed gradually. The electronic drill control system is only switched on when the temperature in the control room has risen above ~ 0°C to protect the electronic devices.
Ice-core drilling
The deep ice drilling operating procedure can be summarised as the pre-operating check, lowering the drill, drilling, returning the drill to the surface, removing the core from the barrel, cleaning the drill, setting the drill, recycling the chips and other tasks, work in shifts, and work at the finish time. Although most ice-core drilling procedures are similar, some operational precautions at Dome A were established: (1) before rotation is started, the drill is lowered to the bottom of the hole and then raised approximately 10 cm off the bottom; (2) penetration continues until the rotation speed declines or the drill motor current abruptly drops; (3) ice cuttings are separated from the drilling fluid (n-butyl acetate) with a centrifuge before they are collected (Fig. 4a); and (4) ice cores are marked before they are carefully carried to the ice storage room (Fig. 4b).

Fig. 4. (a) Centrifuging fluid from the cuttings and (b) ice cores in the ice storage room.
To preserve Antarctica's pristine environment, the centrifuged cuttings are placed in empty oil drums and are transported back to China as some drilling fluid remains in the cuttings.
A small number of ice cuttings remain in the borehole after each drilling roundtrip, which results in a reduced drilling efficiency. Consequently, filtering the ice cuttings is essential for efficient drilling (Fig. 5). For the uppermost 650 m, the cuttings needed to be filtered approximately twice for every ~100 m of penetration. The first run collects a majority of the ice cuttings, and the second collects the remaining ice cuttings while verifying that only a minor amount of ice cuttings remain in the hole. On average, each filter run collects approximately 6.5 kg of ice cuttings. Filtering occurs each day every 10 m between depths of 650 and 800 m, collecting approximately 3.5 kg of cuttings each time.

Fig. 5. (a) Filter for cuttings and (b) emptying cuttings from the filtering tool.
Ice-core processing
Immediately after the retrieval of an ice core from the core barrel, the core is cleaned and weighed, and the run number, bag number, and direction of the top is marked. The ice core is then moved, usually for 10 days, to the core buffer room to release stress. The ice core is then split into 1.0 ± 0.1 m lengths to weigh, gauge (i.e., length and diameter), and mark each section. The ice core is finally packed in a clear polyethylene bag, which is placed in a polypropylene insulating box. Each box holds six ice cores (Fig. 6).

Fig. 6. (a) Cutting the ice core to length; (b) weighing the ice core; (c) packing the ice core into the insulated transport box; and (d) stowing boxes on a sled for transportation.
Progress of deep ice-core drilling project at dome A
The deep ice-core drilling project at Dome A began in 2009/2010. A total of seven CHINARE campaigns have been conducted, including site construction (two seasons), pilot hole drilling (one season), installation of the deep ice-core drilling system (one season), and deep ice-core drilling (three seasons).
In total, approximately 100 days were spent at Kunlun station during the five drilling seasons (2011–2017; Fig. 7). However, there have only been 52 ice-core drilling days. During the first drilling season (2011/2012), the drilling and reaming (shallow ice-core drilling) of the pilot hole required eight days. The remaining 44 days throughout the other four seasons were used mainly for deep ice-core drilling. To date, there have been a total of 374 drilling runs, which include 337 runs with core retrieval, 15 runs without core retrieval, and 22 filtering runs.
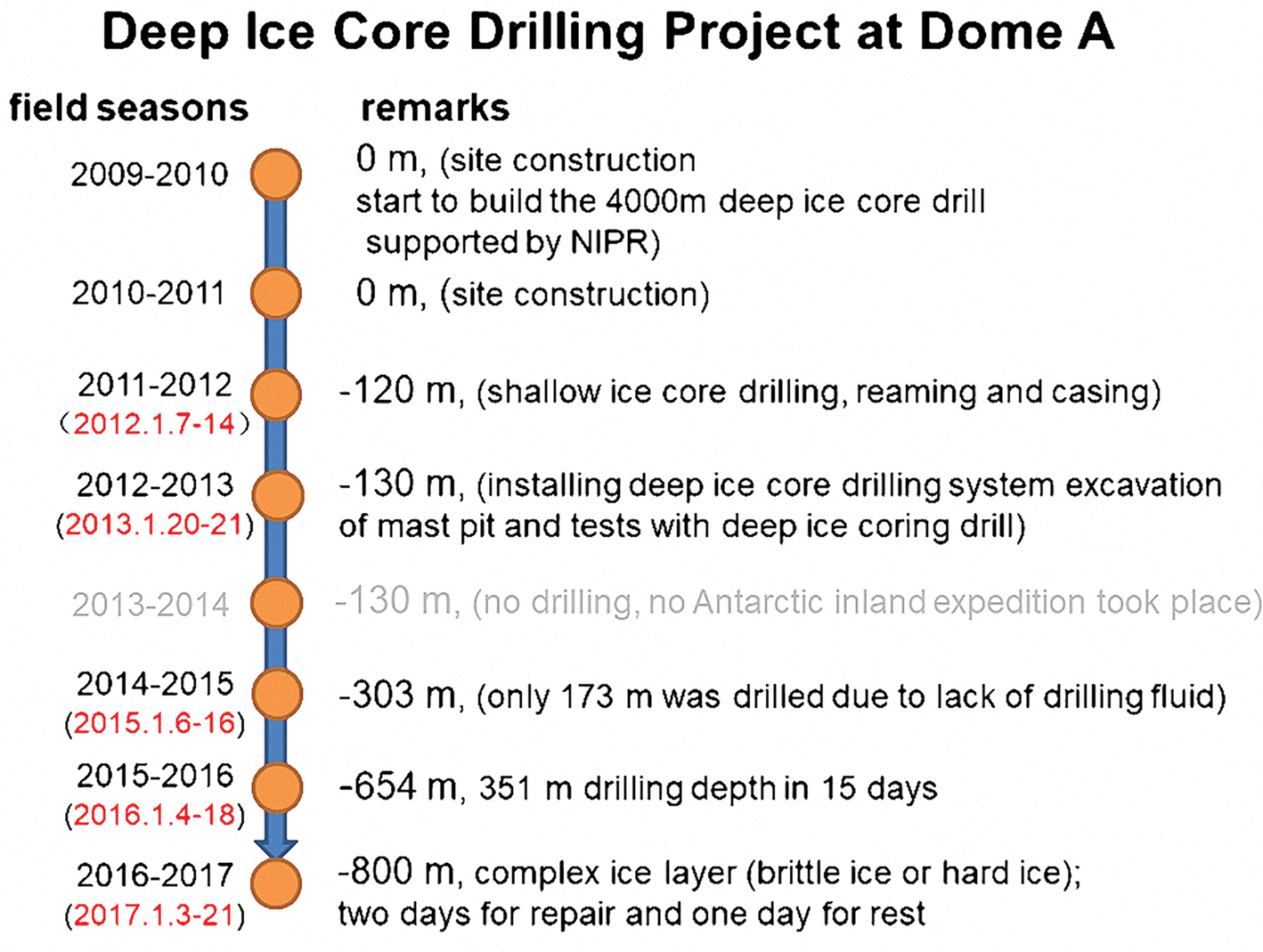
Fig. 7. Progress of the deep ice-core drilling project at Dome A. The dates in red represent actual drilling periods.
There have effectively been 118 shallow ice-core drilling runs, with minimum and maximum penetration depths of 0.1 and 1.45 m, respectively, where 63.6% of the retrieved core lengths were > 1 m (Fig. 8). The average penetration depth per run was 1.01 m for all shallow ice-core drilling runs. The average core length during shallow ice-core drilling was 1.09, 1.23, 1.12, 1.05, 0.99, 0.78, 0.83, and 0.66 m/day.

Fig. 8. Total number of runs, drilling runs with core retrieval, and penetration depth per run in the seasons from 2011/2012 to 2016/2017. The red numbers on the x-axis indicate the end day of each drilling season. The total number of drilling runs is the sum of runs with core retrieval, runs without core retrieval, and filter runs.
During deep ice-core drilling, there have been 219 runs with core retrieval, and the minimum and maximum penetration depths per run were 0 and 3.94 m, respectively, with an average penetration depth per run of 3.1 m. The total penetration depth is currently 800.76 m (Fig. 9).

Fig. 9. Hole depth of five drilling seasons, represented with five different colours.
Deep ice-core drilling seasons
2011/2012
During the CHINARE 2011/12 season, four drill operators from two institutions were engaged in the project. Shallow drilling was conducted, and the hole was reamed before they installed the casing pipe. The pilot hole was completed within 120 drilling runs, including two runs without core retrieval.
At the end of the season, the hole depth was 120.79 m (Fig. 9), and an ice core 120.3 m in length was recovered. For the pilot hole drilling, the core recovery rate was 99.6%, and the average penetration rate was 6.9 m h–1. The maximum daily penetration depth in January 2012, was 23.51 m, achieved during 21 runs, with an average penetration depth of 1.12 m, and a penetration rate of 7.84 m h–1. The minimum daily penetration depth of 1.31 m occurred on 14 January 2012, when only two drilling runs could be executed.
2012/2013
During the 2012/2013 season, an additional drilling operator was deployed. The team finished the installation of the deep ice-core drilling system and carried out three runs of core drilling. The technical parameters of the electromechanical deep ice-core drilling are described in a previous study (Zhang and others, Reference Zhang2014). This season was mainly utilised for testing the deep ice-core drilling system, and approximately 11 m of the ice core was recovered over three runs. The first run, at a depth of ~124 m from the surface, contained a visible volcanic eruption layer (Fig. 10). Considering the average snow accumulation at Dome A (Jiang and others, Reference Jiang2012), this suggests a large volcanic eruption occurred between 4000 and 5000 B.P.

Fig. 10. Clearly identifiable volcanic eruption layer at a depth of ~124 m from the surface.
The casing tightness was also tested. To prevent hole-closure due to glacial-static pressure, the borehole was filled with drilling liquid. The borehole diameter (135 mm) requires 143 L of drilling fluid per a 10-m-depth-increment to geometrically fill the hole. In practical terms, approximately 185 L of drilling fluid are consumed per 10-m-depth. Drilling fluid loss occurs due to evaporation, ice cuttings residue, and residue on the ice core. The topmost 102 m of the casing, above the firn−ice transition, is not filled with liquid. The depth of the suddenly decreasing load is monitored to plumb the liquid level, which was maintained between 120 and 180 m below the casing top. The liquid level does not reduce to below 220 m, which helps retain a high ice-core quality (Sheldon and others, Reference Sheldon, Popp, Hansen and Steffensen2014).
2014/2015
Nine ice-core drillers from four institutes travelled to Dome A for the deep ice-core drilling project in 2014/2015. Due to a shortage of drilling fluid at the site, only 51 successful drilling runs were possible during this season. Averaged over the season, the penetration rate was approximately 5.65 m h–1 and the average penetration depth per run was ~3.4 m. Moreover, penetration was not possible for three out of the 54 runs in the season, which is only 5.56% of the total runs. The statistical results substantiate that the average penetration was the highest during this season, and the number of lost runs was the lowest.
The penetration depth in this season was 173.4 m (Fig. 9), and the total length of the recovered ice core was 169.2 m, with a core recovery of 97.6%. Approximately 3000 L of drilling fluid was injected into the borehole. The maximum daily penetration depth was 23.85 m (14 January 2015), which was drilled in seven runs, and the average penetration depth per run was approximately 3.41 m. The first day of this drilling season only permitted one drilling run and a penetration depth of 3.32 m.
2015/2016
Eight of the 109 total drilling runs failed (3.42%) during this season. Five runs were undertaken with a filter to remove ice cuttings from the hole. Approximately 6400 L of drilling fluid was injected into the borehole. Table 1 summarises the principal drilling situation during this season.
Table 1. Principal drilling situation during the 2015/2016 season

In total, 353.3 m of ice core was extracted (Fig. 9), and the borehole penetration advanced by a further 350.8 m, the largest advance out of the five drilling seasons. The core recovery during this drilling season was 99.3%. The average penetration rate during the 2015/2016 season was 5.31 m h–1 and the maximum penetration rate was 8.98 m h–1, which is also the maximum out of all five drilling seasons. The average penetration depth per day was approximately 23.42 m and the maximum daily penetration depth was 38.7 m.
2016/2017
The borehole deepened by 146.1 and 146.0 m of the ice core was extracted in 2016/2017 (Fig. 9). The core recovery during this drilling season was approximately 100%. Some problems with the drilling system occurred during this season: (1) more ice cores broke into pieces than in other seasons. In addition, the ice cuttings were very coarse, similar to large particles of crushed ice (Fig. 11a); (2) the armoured cable kinked at a payed out length of 678 m because of a minor operational error (Fig. 11b); (3) drilling fluid leaked into the pressure chamber, compromising the motor and CPU; (4) the harmonic reducer broke continuously until it was installed according to instructions provided by the National Institute of Polar Research, Japan (Figs 11c and d); (5) drilling ceased due to lost filter screws, which dropped into the borehole and damaged the cutters (Figs 11e and f); (6) once the drilling time exceeded approximately 2 h, it became extremely difficult to remove the inner core barrel from the outer tube and push the ice core out of the core barrel; and (7) during run 59 of the drilling season, on 14 January 2017, the angle of inclination (monitored by a control system) suddenly increased to over 10° without warning, and the cutter load gradually increased to a maximum. The angle of inclination returned to normal when the rotation speed of the drill bit decreased to less than 20 rpm. This occurred five more times in the following four days.

Fig. 11. Multiple problems occurred during the 2016/2017 drilling season: (a) coarse cuttings; (b) kinked armoured cable; (c) broken harmonic gear/drive; (d) mounting discrepancy between the harmonic gear/drive and transmission shaft; (e) loosened or dropped screws; and (f) a broken cutter, which occurred during the filtration of ice cuttings in the borehole.
Due to these problems, one-third of the available time at Kunlun station was lost, including four days to repair drilling tools and one day to search for dropped screws. Accordingly, only 55 runs were conducted. Nine runs were lost, and 24 runs were spent on retrieving screws and filtering to retrieve ice cuttings (Fig. 7). Table 2 summarises the principal drilling situation during this season.
Table 2. Principal drilling situation for the 2016/2017 season

The proportion of filtering, fishing, and lost runs in these five field seasons was approximately 11% of the total number of runs undertaken. This was worse in the 2016/2017 season, and the proportion reached 30%. The main reasons for failure were: (1) too many novices, most of whom had little practical experience; (2) the complex structural properties of the ice interval between 650 and 800 m, which could include brittle or hard ice; and (3) the drilling rig itself developed some mechanical ageing problems as it was transported to Kunlun Station.
Ice-core drilling parameters
Total run time
The ice-core drilling process for each run includes tower erection and tilting, initial ice-core processing, drill string hoisting and lowering, and drilling of the ice core. The total time for each drilling run can be predicted by the development of the hole and changes observed during the three previous drilling seasons. The time required for tower erection and tilting was ~12.5 min and the time required for initial ice-core processing (cleaning the drill from ice cuttings, removal of the core barrel from the drill, retrieval of the ice core from the core barrel, marking the ice core and moving it to the ice-core buffer, mounting of the core barrel, and processing of the ice cuttings) was ~10 min. The total time for each drilling run (T, min) can be calculated as follows:

where T1 is the time required to lower the drill (min); T2 is the time required to hoist the drill (min); T3 is the penetration time, which is affected by the ice properties of the drilled section (min); h is the hole depth (m); Δh is the penetration depth (m); and the mean penetration rate is ~4 m h–1.
Penetration depth
The average daily penetration depths during the 2011/2012, 2014/2015, 2015/2016, and 2016/2017 seasons were 15.1, 15.7, 23.4, and 9.1 m, respectively, with an overall average penetration depth of 15.4 m day–1. There were 18 days with a penetration depth of less than 10 m (Fig. 12). The low penetration rate predominantly occurred during the 2016/2017 drilling season. The lowest penetration depth was 0.73 m, which occurred on day 46. There are three probable reasons for the low production: (1) hard or brittle ice sections during the 2016/2017 season; (2) during the first days of each season, new drillers familiarise themselves with the drilling system and received operational training; and (3) the final days of operation of a field season are not exclusively devoted to drilling, but also to clean the drilling site for the next season. The maximum penetration depth (39 m) occurred on day 24.

Fig. 12. Daily penetration depth.
Actual bit on bottom times of each drilling run
Figure 13 shows the actual bit on bottom time (ABOBT) for each drilling run. The average ABOBT value was 0.76 h. During shallow ice-core drilling, the ABOBT was relatively stable and the average time was 0.16 h. In deep ice-core drilling, this fluctuates between 0.5 and 1.0 h, except for the ice section between 650 and 800 m. The average ABOBT from a depth of 120−650 m was 0.62 h. However, the average time was up to 1.16 h between the depths of 650 and 800 m.

Fig. 13. Actual bit on bottom time as a function of the hole depth.
Penetration rate
Figure 14 illustrates the generally larger penetration rate for the dry shallow drilling of the pilot hole compared with the penetration rate of the deep ice-core drill. The maximum penetration rate was ~12 m h–1, and the minimum penetration rate was close to 0 m h–1. Between a depth of 100 and 650 m, the penetration rate was relatively stable, i.e., between 4.8 and 6.4 m h–1. At greater depths, i.e., between 650 and 800 m, the penetration rate decreased below 4 m h–1.

Fig. 14. Penetration rate as a function of the hole depth.
The penetration rates of the deep ice-core drilling ranged between 4 and 8 m h–1 (with an average of 6 m h–1) whereas that of the shallow ice-core drilling were approximately 8 m h–1. The penetration rate was less than 4 m h–1 during the 2016/2017 season, mainly due to the complex material properties of the ice section and the lower proficiency for user operating of the drilling system. Theoretically, the penetration rate of deep ice-core drilling should remain stable and only slightly fluctuate with depth; this was the case, except for the 2016/2017 field season.
Core-length per run
For shallow ice-core drilling, one notable trend was that the core-length per run decreased with the depth of the hole. The shallow drilling core length changed, which is common and was expected due to an increasing density. The density increases with depth, thus filling a greater proportion of the drill with cuttings, which decreases the core length. However, most of the cores exceeded 1 m in length (Fig. 15). In the deep ice-core drilling, the density was relatively constant and the core-length per run was relatively stable (between 3 and 4 m). Most of the cores were approximately 3.5 m long, although some cores were shorter than 2.5 m.

Fig. 15. Drilling depth as a function of the core length in each drilling run.
Drilling data parameters
Approximately 30 parameters were monitored, including the cutter load, penetration rate, current and voltage, rotation speed, temperature, drill inclination, and run length, among others. All sensor data were transferred through a relay computer to a personal computer and were ultimately saved onto a hard disk. Some data loss occurred due to sensor failure (Figs 16–18), which is critical to drill operation.

Fig. 16. Cutter load and rotation speed of the drill head.
As illustrated in Figure 16, the cutter load at the bottom ranged from 35 to 55 kg. In drilling runs 140–175, the cutter loads remained stable (at ~45–50 kg). In drilling runs 175–275, the cutter load fluctuated within a load interval (between 35 and 55 kg). The rotation speed of the drill head was limited to between 30 and 80 RPM. The rotation speed remained relatively stable (between 42 and 64 RPM) during drilling runs 140−175. The rotation speed fluctuated widely during drilling runs 175−275, although it mostly remained between 34 and 66 RPM.
The output voltage varied between 40 and 140 V (Fig. 17). The output current appeared to increase with the drilling depth. The output current was relatively stable during shallow ice-core drilling, and the current ranged between 0.7 and 1.2 A. During deep ice-core drilling, the output current was greater than 1.5 A and lower than 3.3 A. For drilling runs 140−180, the current ranged between 1.5 and 1.8 A, which is very stable. During drilling runs 180−280, the current fluctuated between 1.8 and 3.3 A.

Fig. 17. Output voltage and current to the drilling motor.
As shown in Figure 18, the drilling fluid temperature in the borehole varied with depth. Between 100 and 300 m, the temperatures fluctuated between −55 and −52°C while the temperatures were even lower between 300 and 480 m (between −56 and −53°C). Between 480 and 800 m, the temperatures gradually rose (from −56 to −46°C). The temperature gradient is controlled by the vertical upward heat flux via conduction at the bottom provided by the difference in the geothermal heat flux. Thus, the increase with depth is expected for an inland drilling site (see Fischer and others, Reference Fischer2013).

Fig. 18. Variations in the liquid temperature and borehole inclination as a function of the hole depth.
The borehole inclination changed in the range of 0.8–1.9° along the depth interval between 140 and 680 m. When the hole depth reached 680 m, the inclination sensor was replaced, and there was a sudden change in inclination. The inclination of the borehole decreased to between 0° and 0.5°, with relatively little fluctuation. We note that both the new and old inclination sensors had the same specifications. Based on the available data and experience, ascertaining the causes of the sudden changes in the borehole inclination is difficult. However, some possible reasons include the following: (1) the two inclination sensors may have had a different scale (resistor), or a different coding for digital sensors; and (2) the leaked drill liquid may have compromised the electronics.
Conclusions and prospects
Some of the practices, experiences, and lessons were discussed and introduced herein to share with peers and as a reference. Although the drilling depth exceeds 800 m, the total time exceeds 5 years. We hope to increase the actual drilling time by changing the operational mode of CHINARE. If each drilling season can be extended from approximately 40−50 days at Dome A, we expect to reach the estimated bottom of the ice sheet within another 3–4 drilling seasons.
Supplementary material
The supplementary material for this article can be found at https://doi.org/10.1017/aog.2021.2
Acknowledgements
First, we would like to extend our sincere gratitude to the 32nd and 33rd CHINARE for logistic support. We are deeply indebted to all Kunlun team members for their direct and indirect help. We especially acknowledge the deep ice-core drilling team members who, through their efforts, overcame hypobaric, hypoxia, and extreme cold severe environmental conditions at the highest altitude of the inland Antarctic to drill to 800 m. We express our sincere thanks to H. Motoyama at the National Institute of Polar Research, Japan, and Japanese drilling experts Y. Tanaka, M. Miyahara, and A. Takahashi for drilling technology support. Finally, we acknowledge Frank Wilhelms, Kenji Kawamura, and one anonymous reviewer for their detailed and constructive comments, which significantly improved this manuscript. This study was supported under the National Natural Science Foundation of China (41922046), the National Key Research and Development Program of China (2016YFA0302204), the Chinese Polar Environment Comprehensive Investigation & Assessment Programmes (CHINARE 201X–02–02), the National Natural Science Foundation of China in 2019 special project polar Basic Science Frontier project (No. 41941004, No. 41941006), the General Project of the National Natural Science Foundation of China (No. 41876225), the Chinese National special environment, and the special function observation research station sharing service platform, Zhongshan Station.