Projectile-point sizes tended to decrease over time in North America. This phenomenon has been variously attributed to the transition from atlatl to bow-and-arrow technology (Bettinger and Eerkens Reference Bettinger and Eerkens1999; Whittaker et al. Reference Whittaker, Pettigrew and Grohsmeyer2017), decreasing prey size (Tomka Reference Tomka2013), the invention of fletching (Hughes Reference Hughes1998), and stochastic cultural transmission processes in which flintknappers tend to create smaller points than those of their mentors because of copying errors (Hamilton and Buchanan Reference Hamilton and Buchanan2009). On the Andean Altiplano, projectile-point size decreased dramatically after the apparent introduction of the bow and arrow around 3,000 years ago (de Souza Reference de Souza2004, Reference de Souza and Hubert2011; Klink and Aldenderfer Reference Klink, Aldenderfer, Stanish, Cohen and Aldenderfer2005). Although the trend of decreasing point size is common across many regions and time spans in the Americas (for a broader discussion of lithic miniaturization, see also Pargeter and Shea Reference Pargeter and Shea2019), we observe what appears to be an unusual period of projectile-point enlargement on the Andean Altiplano over about 6,000 years between the Early and Late Archaic periods, 11–5 cal ka (Klink and Aldenderfer Reference Klink, Aldenderfer, Stanish, Cohen and Aldenderfer2005).
Early Archaic points of the Altiplano are notably small, whereas Middle and Late Archaic points are perceptibly larger (Figure 1). This qualitative pattern bears out quantitatively among several assemblages. Restifo's (Reference Restifo2013) data from the high puna of northwest Argentina show that average projectile-point mass increased from approximately 3 g in the Early Holocene to approximately 20 g in the Middle Holocene. Complete surface-collected projectile points from the Ilave drainage west of Lake Titicaca similarly exhibit a statistically significant increase from approximately 3 g in the Early Archaic period to 6 g in the Middle and Late Archaic periods (Haas and Viviano Llave Reference Haas and Llave2015; Figure 2). Data from the Ramis region north of Lake Titicaca show that average stem widths—measured near the stem/blade juncture—of surface-collected projectile points significantly increased from 13 mm in the Early Archaic period (11–9 cal ka) to 19 mm in the Late Archaic period (7–5 cal ka; see Figure 2).

Figure 1. Examples of projectile-point forms of the Titicaca Basin. Top row, Early Archaic period (11–9 cal ka). Second row, Early/Middle Archaic period (10–8 cal ka). Third row, Middle Archaic period (9–7 cal ka). Bottom row, Late Archaic period (7–5 cal ka). Labels indicate types as defined by Klink and Aldenderfer (Reference Klink, Aldenderfer, Stanish, Cohen and Aldenderfer2005). Artifacts are from collections made by Klink (Reference Klink, Stanish, Cohen and Aldenderfer2005), Aldenderfer (Craig Reference Craig2011), and Haas and colleagues (Reference Haas, Klink, Maggard and Aldenderfer2015). (Color online)

Figure 2. Projectile-point size increase in the Titicaca Basin. Left: projectile-point mass among complete points from the Ilave drainage shows a statistically significant increase as a function of time period (3.1 ± 0.6 mm/period, t = 5.3, p < 0.01). Right: projectile-point stem width, measured at the stem/blade intersection, shows a statistically significant increase as a function of time period (1.0 ± 0.2 g/period, t = 4.8, p < 0.01). Numbers overlaying the boxes indicate sample sizes for each period. Slope estimates and standard errors are solved using generalized linear models, and associated t ratios and p-values assume Gaussian family dispersion (R Core Team 2020).
The pattern of projectile-point enlargement in the Titicaca Basin is also evident in projectile points reported from the few directly dated contexts in the Titicaca Basin. The average mass of six complete Early Archaic projectile points from a 9.0 ka burial at Wilamaya Patjxa is 3.1 g (Haas et al. Reference Haas, Watson, Buonasera, Southon, Chen, Noe and Smith2020). Those points contrast with a large lanceolate Middle Archaic point weighing 10.7 g associated with a 7.4 ka burial at Soro Mik'aya Patjxa (Haas and Viviano Llave Reference Haas and Llave2015; Haas et al. Reference Haas, Stefanescu, Garcia-Putnam, Aldenderfer, Clementz, Murphy, Llave and Watson2017).
Given that the projectile-point forms found in the Titicaca Basin are found throughout the Altiplano and that the pattern is observed in both the northern and southern Altiplano, it is likely that the trend can be interpolated throughout the Altiplano more generally. Although the pattern has not yet been systematically analyzed and reported, the qualitative and quantitative data summarized here strongly suggest a pattern of projectile-point enlargement throughout the Altiplano Archaic.
The observation of increasing projectile-point size in the Altiplano Archaic against a backdrop of decreasing point size across a larger time scale and elsewhere in the Americas raises the question of why projectile-point size should increase over time in those relatively rare cases. As a first approximation, we can readily imagine several models to explain the enlargement of projectile points across the Altiplano Archaic. An exogenous historical explanation might imagine that migrating populations entered the region bringing their cultural conventions for the production of larger projectile points that replaced smaller local forms, perhaps by chance or perhaps as part of intergroup competition that favored larger armaments.
An endogenous version of a competition model might suppose that competition and territoriality increased among local individuals or groups throughout the Archaic as populations grew. Individuals might have enlarged projectile points as threat displays. The use of threat displays has been observed in ethnographic contexts and among other species in competitive environments (Crivelli et al. Reference Crivelli, Russell, Jarillo and Fernández-Dols2016; Ladich Reference Ladich1990; Scott and Fredericson Reference Scott and Fredericson1951). In favor of these two competition hypotheses, evidence of increasing population has been consistently found on the Andean Altiplano (Craig Reference Craig2011; Gayo et al. Reference Gayo, Latorre and Santoro2015; Klink Reference Klink, Stanish, Cohen and Aldenderfer2005; Marsh Reference Marsh2014; Muscio and Lopez Reference Muscio and López2016; Vining et al. Reference Vining, Steinman, Abbott and Woods2018). Moreover, violence has been observed as perimortem traumas in the Middle–Late Archaic osteological remains at the site of Soro Mik'aya Patjxa, 8.0–6.5 cal ka, suggestive of a competitive environment at that time (Haas and Viviano Llave Reference Haas and Llave2015).
An alternative endogenous model, which considers techno-functional drivers, might suppose that the emergence of communal hunting strategies underwrote an increase in projectile- point size. Aschero, Martínez, and Restifo have suggested that communal hunting strategies may have appeared in the Archaic, and those strategies, which brought humans into close range with animals, may have favored the use of hand-held spears that, in turn, would have favored the use of large stone points (Martínez and Aschero Reference Martínez and Aschero2003; Martínez and Funes Reference Martínez, Funes Coronel, Martínez and Bozzuto2011; Restifo Reference Restifo2013; Restifo et al. Reference Restifo, Patané Aráoz and Piraino2019). In a review of ethnographic weapons systems, Ellis (Reference Ellis and Knecht1997) observed that stone-tipped spears indeed occur in special hunting circumstances. Although this is a plausible model for the Andean case, the extent to which stoned-tipped spears were used in the Andes remains unclear. To our knowledge there is currently no clear material evidence of stone-tipped spears in the Andes. In contrast, atlatls and associated artifacts are documented in the archaeological record (de Souza Reference de Souza and Hubert2011; Fernández Reference Fernández Distel1986; Núñez et al. Reference Núñez, Fasting, Carlos Carrasco, Patricio de Souza and Grosjean2006), and rock art scenes, including communal hunting scenes, commonly depict atlatl use (de Souza Reference de Souza and Hubert2011; Hostnig Reference Hostnig2018).
This article examines two alternative techno-functional hypotheses, which envision that increasing projectile-point size reflects technological adaptation to changing economic landscapes. One hypothesis considers that increasing projectile-point mass reflects an effort to increase dart penetration. The other hypothesis considers that the increasing mass reflects an effort to increase dart accuracy. At more than 3,800 m asl, few trees grow on the high plains or Altiplano. Only a single small tree taxon, queñual (Polylepis sp.), is endemic to the region (Winterhalder and Thomas Reference Winterhalder and Thomas1978). The lack of wood on the Altiplano would have challenged the production of atlatls—likely the major hunting technology throughout the Archaic (de Souza Reference de Souza and Hubert2011; Hostnig Reference Hostnig2018; Núñez et al. Reference Núñez, Fasting, Carlos Carrasco, Patricio de Souza and Grosjean2006; see also Ellis Reference Ellis and Knecht1997; Hughes Reference Hughes1998). Perhaps most problematic, atlatl systems require long, straight, small-diameter dart shafts of uniform thickness on the order of 2 m in length. The atlatl itself—typically comprising a straight or slightly arced stick—is on the order of 45 cm in length (Baugh Reference Baugh1998). High-quality atlatl-production materials would have been limited in the Altiplano's treeless landscape and increasingly so as those resources were exhausted by continued harvesting and human population growth. Further exacerbating the wood supply problem, the Early–Middle Archaic transition was characterized by aridification and a decline in queñual forests (Craig et al. Reference Craig, Aldenderfer, Baker, Rigsby and Dean2010; Rigsby et al. Reference Rigsby, Baker and Aldenderfer2003). Lake Titicaca levels dropped approximately 100 m beginning around 8.5 cal ka and did not recover until the Terminal Archaic period around 4.0 cal ka (Cross et al. Reference Cross, Baker, Seltzer, Fritz and Dunbar2001). Archaic hunter-gatherers would have needed to find ways to simultaneously make use of increasingly suboptimal wood materials while maintaining the effectiveness of their hunting implements.
One solution to increasing wood scarcity might have been the use of large mammal bone for the atlatl (Figure 3A). The use of camelid radioulna for this purpose is documented in specimens from the Chilean Atacama Desert highlands (de Souza Reference de Souza and Hubert2011; Nuñez et al. Reference Núñez, Fasting, Carlos Carrasco, Patricio de Souza and Grosjean2006; Figure 3B). Camelid and deer bone would have been relatively accessible to Archaic period big-game hunters, solving part of the material-supply problem. However, this material alternative would have come at a cost. At approximately 25–35 cm long, camelid radioulna are short compared to optimal atlatl lengths. Because velocity increases with distance from a rotation point (v = r*ω, where v is velocity, r is radius, and ω is angular velocity), the use of bone would tend to decrease a thrown dart's velocity. Baugh (Reference Baugh1998) shows empirically that increasing atlatl length increases dart velocity until the atlatl exceeds its optimal length of 45 cm, beyond which dart velocity decreases.

Figure 3. Bone atlatls and experimental design. (A) Alpaca radioulna used in this study before shaping; (B) archaeological camelid-radioulna atlatls from the Atacama Desert highlands (adapted from de Souza [Reference de Souza and Hubert2011] and reproduced with permission of the author); (C) experimental alpaca radioulna atlatl; (D) ballistic trial with atlatl, dart, target, and ballistic gel; (E) atlatl-dart articulation (photographs by Randall Haas). (Color online)
In addition, decreasing dart length would decrease dart mass, which could be expected to reduce the dart's penetration power, given that penetration is a function of force and momentum, each of which vary as a function of mass (f = ma, where f is force, m is mass, and a is acceleration; p = mv, where p is momentum and v is velocity). A simple and tractable solution to compensate for these performance losses could have been to increase the mass of foreshaft assembly. This could be accomplished by using dense woods for the foreshaft or increasing foreshaft size by, for example, carving them in such a way that left large bulges, as observed in an archaeological specimen from the Atacama (de Souza Reference de Souza and Hubert2011; Núñez et al. Reference Núñez, Fasting, Carlos Carrasco, Patricio de Souza and Grosjean2006). Another practical solution, particularly when wood is scarce, would be to increase projectile-point mass. Such increases in foreshaft or projectile-point mass would have the effect of increasing dart momentum and thus, theoretically, affect force, penetration, and killing power (Hughes Reference Hughes1998; Whittaker et al. Reference Whittaker, Pettigrew and Grohsmeyer2017). Importantly, increasing dart thickness would not be a viable option because proper atlatl functioning requires flexible darts to ensure proper oscillation during release (Hughes Reference Hughes1998).
A related effect of diminishing dart length and corresponding mass loss would have been reduced stability of a dart in flight, which would have the effect of diminishing accuracy. Reduced mass renders the dart more susceptible to deflection by wind, branches, and stochastic drift in the trajectory as the oscillating dart is released from the atlatl. Increasing projectile-point mass could therefore be expected to counter such instability (Christenson Reference Christenson1986; Hughes Reference Hughes1998) potentially offsetting accuracy losses due to decreasing dart mass that might be expected with diminishing dart length.
The dart momentum and accuracy hypotheses lead us to expect that larger and heavier dart points should exhibit greater penetration and accuracy than lighter dart points. The primary goal of this study is to test these expectations experimentally. We focus on techno-functional hypotheses not to diminish the alternative hypotheses outlined earlier nor any hypotheses that we may have failed to consider. Rather, our focus reflects a small, tractable contribution toward a larger effort of evaluating competing models to explain increasing projectile-point size.
A related secondary goal of our analysis is to gain insight into the use and manufacturing costs of camelid bone atlatls. Although such implements are known from a few rare Atacama specimens (Figure 3B), how the unconventional forms work remains unknown because, to our knowledge, their use has never been observed ethnographically or experimentally. Curiously, the archaeologically observed bone atlatls are curved in the opposite direction from typical atlatls observed elsewhere in the Americas and used by atlatl enthusiasts today. Such atlatls are typically flat or arc upward such that the ends bow toward the dart when the two components are engaged. The Andean bone atlatls, however, bow in the opposite direction such that the atlatl ends arc away from the dart when engaged. What is particularly peculiar about this geometry is that it would seem to preclude one-hand operation. Qualitative examination of images of the archaeological specimens seem to suggest that it is impossible to simultaneously engage the atlatl hook with the butt end of the dart while supporting the dart shaft with the throwing hand fingers, as is the typical atlatl throwing style. The counter-arcing form of the bone atlatl would seem to prevent such an arrangement. We therefore hypothesize that the distinct geometry of camelid radioulna atlatls would have entailed a distinct throwing technique that required two-handed operation, with the nonthrowing hand used to support the dart shaft before launch.
In terms of production costs, we expect relatively long manufacturing times, because bone is denser than wood and bone grease would tend to clog the pores of sanding implements—a problem that is less likely to be experienced with wood. We suppose that such high production costs would have been absorbed out of necessity under conditions of wood scarcity.
Materials and Methods
This study used replicated camelid bone atlatls, wood darts, and flaked-stone projectile points in ballistic trials to evaluate production costs and performance characteristics under conditions of variable projectile-point size. There have been at least two other experimental Andean atlatl studies, both of which examined different questions than we did. Martínez and Aschero (Reference Martínez and Aschero2003) examined projectile-point breakage patterns, whereas Martínez and Funes (Reference Martínez, Funes Coronel, Martínez and Bozzuto2011) examined the performance of beveled hafting systems and dart fletching. We are particularly interested in understanding how projectile size affects penetration depth and accuracy. We opted for a relatively naturalistic experimental approach that would allow us to gain broad insights into production and performance while evaluating the model expectations. We acknowledge the importance of more controlled experiments, but our approach offers a critical first step for broadly understanding an atlatl system that has, to our knowledge, not been used in several millennia. Such semistructured experimentation should intelligently guide more focused experiments conducted at a later time. To the extent possible, aboriginal tools were used to create the atlatl, darts, and projectile points.
Although vicuña radioulna would have been used in the Archaic period, we used alpaca radioulna for our experiment for two reasons. First, whereas vicuña are a protected species, alpaca are common farm animals, making alpaca bone readily accessible. Second, this substitution is likely to be of little consequence for the experimental design, given that the two taxa are essentially the same species: vicuña are the wild ancestor of the alpaca, and their bone structures are likely isomorphic (Bonavia Reference Bonavia2008; Pacheco Torres et al. Reference Pacheco Torres, Enciso and Porras1979). We used thick tabular pieces of sandstone from the German Rancho Formation in California to grind the superior surface of each alpaca bone. We ground the bone until the medullary cavity was exposed as observed in archaeological specimens (see Figure 3C). Then, we used a thin piece of sandstone with an acute edge for more detailed shaping of the spur.
We used heat-treated chert for projectile-point production (Figure 4). Chert was commonly used in the Lake Titicaca Basin because of its abundance and accessibility (Haas et al. Reference Haas, Stefanescu, Garcia-Putnam, Aldenderfer, Clementz, Murphy, Llave and Watson2017), and Archaic period Titicaca Basin groups heat-treated lithic material to facilitate flaking. Our points were manufactured from heat-treated Keokuk chert, which was purchased from a US material supplier. We modeled our replicas after Altiplano 3D-style points (Klink and Aldenderfer Reference Klink, Aldenderfer, Stanish, Cohen and Aldenderfer2005). We chose this form because of its relatively simple lanceolate shape and the fact that it was used over a long time span across the Middle and Late Archaic periods. Six projectile points of varying size were manufactured with the goal of uniformly spanning the range of sizes in archaeological samples.
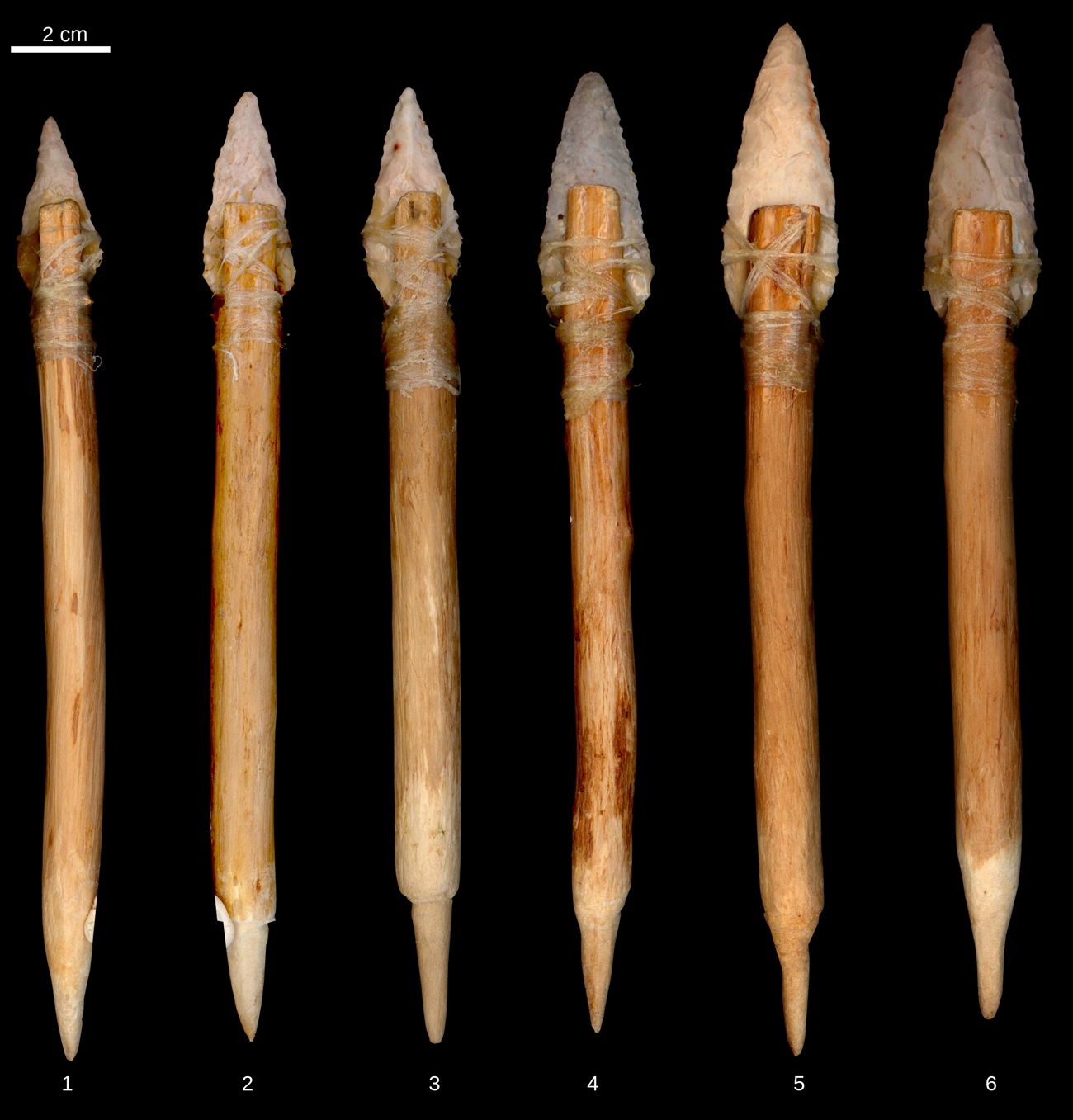
Figure 4. Experimental projectile points and foreshafts used in this study (photograph by Caleb Chen). (Color online)
Because Andean dart forms and materials are currently unknown from the archaeological record, some speculation was required for the design of the experimental dart. We suspected that queñual (Polylepis sp.) would have been the major material for dart construction. Unfortunately, we were unable to identify suitable queñual shafts during limited, informal searches on the Altiplano. We therefore used material that is readily accessible to us in California. We constructed the dart main shaft from a branch of seep willow (Baccharis salicifolia), which is a species that produces long, straight branches. The shaft was steam-straightened after harvesting and subsequently bundled with other branches and dried in the shade for three weeks.
Because of resource scarcity in the Andean highlands, and given the documentation of atlatl foreshafts elsewhere in the Andes (de Souza Reference de Souza and Hubert2011; Martínez and Funes Reference Martínez, Funes Coronel, Martínez and Bozzuto2011; Núñez et al. Reference Núñez, Fasting, Carlos Carrasco, Patricio de Souza and Grosjean2006) and in the Americas (e.g., Frison Reference Frison1968; Harrington Reference Harrington1924; Lahren and Bonnichsen Reference Lahren and Bonnichsen1974; Lindsay Reference Lindsay1968), we speculated that Altiplano Archaic populations likely manufactured dart foreshafts. We therefore manufactured multiple foreshafts and a single mainshaft. The use of a single mainshaft with multiple foreshafts not only replicated archaeological dart design but also provided a degree of experimental control by holding the main shaft constant for all throws. Six foreshafts of identical mass were made of toyon (Heteromeles arbutifolia) wood. The foreshaft bark was removed when green, and the ends of the foreshaft preforms were coated with paraffin wax to prevent wood checking. We cut projectile-point haft slots into the foreshafts using a groove-and-snap technique (Cosgrove Reference Cosgrove1947:52–54; Whittaker Reference Whittaker1994) and shaped the proximal ends of the foreshafts by grinding them on a slab of sandstone. Thicker foreshafts were slightly shortened to equalize mass for all the foreshafts.
Hide glue and alpaca sinew were used to haft points onto the foreshafts and fletch the mainshaft. Our attempt at making alpaca hide glue failed. We therefore used Titebond liquid hide glue. Dried alpaca leg sinew was processed using a hammerstone with a hammer-anvil technique and subsequently hydrated by chewing prior to application to the foreshafts and main shaft. Turkey feather fletching and projectile points were bound to the shafts with the sinew and hide glue.
For the ballistic target, a ballistic gelatin mixture of 4,990 g gelatin and 43 L water was poured into a plywood mold, yielding a gel block with dimensions 40.5 × 30 × 21.5 cm. Paper bullseye targets with direction and distance markers were placed on the anterior surface of the gel block to measure accuracy.
The first author used one of the experimental alpaca bone atlatls to fire darts into the ballistic gel block at a distance of 5 m (Figure 3D). We randomized projectile-point firing order to control for potential effects of user improvement or material changes (e.g., implement wear or target degradation) over time. Penetration depths were recorded by marking the dart foreshafts in the gel block and measuring from the marked area to the point tip. Accuracy was recorded as distance from the center of the target, which was demarcated with a paper bullseye target overlaying the ballistic gel block. Because complete misses were frequent, we also recorded miss frequency for use in calculating hit:miss ratios as a secondary measure of accuracy.
We performed two sets of trials, each on separate days. Opposite sides of the gel block were used on days one and two to minimize chances of redundant hits. Each projectile point was fired until the point or foreshaft failed.
We used linear regression to evaluate the effect of point mass on penetration depth and accuracy. This provided a preliminary visualization and assessment of the data. To account for random effects that result from the repeated use of projectile points (i.e., each shot is not an entirely independent event), we also performed linear regression with dependent variables averaged by projectile point. Finally, we performed linear fixed-effects modeling with projectile-point mass means as the fixed effect and projectile-point number as the random effect. All computation was performed in R statistical computing language using linear modeling base routines (R Core Team 2020), the lme4 package for fixed-effects analysis (Bates et al. Reference Bates, Martin Mächler and Walker2015), and the lmerTest package (Kuznetsova et al. Reference Kuznetsova, Brockhoff and Christensen2017) for generating fixed-effects model p-values. Because our analytical procedure is largely naturalistic so as to gain broad insights into the technology, we take a rather liberal approach to assessing statistical significance, seeking tentative relationships that merit further consideration with more controlled experimentation. We therefore consider p-values of less than 0.1 to be suggestive of statistically significant relationships.
Results
We manufactured two radioulna atlatls in 5.5 and 3.3 hours (Table 1). The reduced time for the second atlatl reflects improvements in technique. In particular, we realized that clogging of the sandstone pores could be minimized by scraping grease from the bone with a flake. Counter to expectations, we experienced surprisingly short production times. We also learned that the medullary cavity of the radioulna bone created a convenient resting channel for the dart. The channel stabilized the dart, allowing its butt end to articulate with the atlatl hook as the thrower used the fingers of the throwing hand to support the shaft (see Figure 3D and 3E). In other words, contrary to our hypothesis of a two-hand throwing style, we learned that the radioulna bone atlatl can be operated with one hand.
Table 1. Atlatl Production Times.

Experimental projectile-point mass including foreshafts ranged from 17.9 to 30.9 g. Final masses for assembled projectile foreshafts 4 and 5 were the same after hafting. The dart main shaft weighed 75.6 g and was used for all trials. Given our relative inexperience in producing such atlatl systems, we use our minimal manufacturing times to estimate a manufacturing time of approximately 9.5 hours for Andean hunter-gatherers (Table 1). Our results make clear that, given all of the right materials, an effective camelid bone atlatl can be produced in a single day. Note that these estimates only include active production times and do not include material drying times.
The experiment resulted in 136 shots: 48 hits and 88 misses (Table 2). We used hit:miss data, aggregated by projectile point (n = 6), to assess the effects of mass on accuracy. Furthermore, the 48 hits were used to assess accuracy as measured by distance from the target center. Not all target hits contacted the gel block and thus not all provided penetration depth data. We recorded 34 depth measurements.
Table 2. Ballistic Test Data.
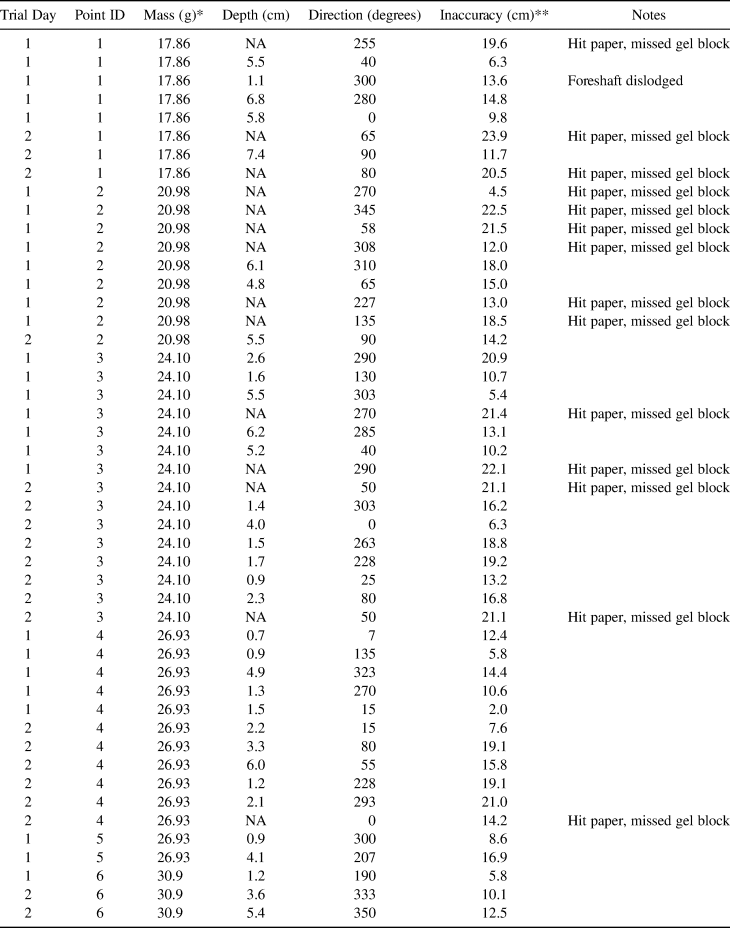
* point + foreshaft
** distance from center of target
The raw penetration depth data (n = 34) reveal a statistically significant negative relationship with point mass at an estimated rate of −0.25 ± 0.09 cm/g (p = 0.01)—a trend that is opposite to the predicted relationship (Figure 5A). When averaging depth values by projectile point (n = 6) to control for autocorrelation, a statistically significant negative relationship of −0.23 ± 0.09 cm/g is maintained, although it is slightly diminished (p = 0.07, Figure 5B). Fixed-effects analysis confirms a statistically significant negative relationship of −0.25 ± 0.09 cm/g (p = 0.06).

Figure 5. Regression test results. (A) Raw data (n = 34) showing statistically significant negative effect of mass on penetration depth, counter to expectation; (B) data averaged by projectile point (n = 6) showing statistically significant negative effect of mass on penetration depth; (C) raw data (n = 45) showing statistically nonsignificant negative effect of mass on inaccuracy or distance from center of target; (D) data averaged by projectile point (n = 6) showing statistically significant negative effect of mass on inaccuracy.
For accuracy assessment, raw inaccuracy measurements (n = 45) fail to support the predicted negative relationship with point mass (Figure 5C), though the observed relationship is negative and marginally nonsignificant (p = 0.14). In contrast, averaging the inaccuracy values by projectile point (n = 6) to control for autocorrelation reveals a statistically significant negative trend of −0.43 ± 0.12 cm/g (p = 0.03; Figure 5D). However, fixed-effects analysis reveals the model fit to be singular due to the small sample size, suggesting no statistically significant relationship. The hit percentage analysis is consistent with this finding, showing no statistically significant relationship between mass and hit percentage for the six points (Table 3; p = 0.58).
Table 3. Hit:Miss Data Summary.

* includes point
Discussion
The finding of a negative relationship between projectile-point mass and penetration depth is consistent with the recent analysis by Mika and colleagues (Reference Mika, Flood, Norris, Wilson, Key, Buchanan and Redmond2020), which observed this trend in a more controlled experiment using bow-and-arrow technology. They found that smaller points outperformed larger points in penetration tests when draw weight was held constant. In similar controlled experiments testing projectile-point penetration, Eren and colleagues (Reference Eren, Story, Perrone, Bebber, Hamilton, Walker and Buchanan2020) and Sitton and colleagues (Reference Sitton, Story, Buchanan and Eren2020) also observed a negative relationship between projectile-point size and penetration. It would appear that lost velocity or increased drag, which results from size increase, outweighs any potential gains in momentum, thus diminishing penetration depth with increasing point size. Alternatively, increased dart mass may reduce dart velocity, thus reducing net kinetic energy, net dart momentum, and penetration of larger projectiles.
The results of our accuracy analysis were less clear. The linear model on the raw data, fixed-effects model, and hit:miss analyses showed no statistically significant relationship between accuracy and point mass. In contrast, the linear model on projectile-point-averaged accuracy data revealed a statistically significant negative relationship. This observation may hint at a latent effect masked by other variables in our relatively uncontrolled experiment. More trials would have been useful for resolving the analytical ambiguity, but unfortunately our experimental equipment was exhausted, preventing additional data collection. Here we see the merit of the naturalistic experimental design in identifying an area in need of more focused investigation while also highlighting its limits in generating conclusive results for specific relationships.
Given that projectile-point mass affects accuracy and penetration, how would small differences in mass affect these variables? Would they appreciably affect hunting success rates? To begin to answer these questions, we consider the magnitude of effects observed in our analyses. In our point-aggregate analysis, we observed that accuracy increased at a rate of 4.3 cm for each gram of increase in projectile point mass. Recognizing that those results are statistically insignificant, we nonetheless wish to consider the extent to which the tentative trends matter and additional research is justified. First, consider that the size of the kill zone of an ungulate is approximately 30 cm in diameter (O'Connor Reference O'Connor1970; Tomka Reference Tomka2013), which gives a radius of 15 cm. Second, consider that our experiment observed an average inaccuracy of 16 cm with an 18 g dart (with foreshaft) at a distance of 5 m (see Figure 5D). This means that roughly half of all shots would land inside the kill zone. When the dart mass was increased to 30 g, average inaccuracy dropped 60% to 10 cm. Thus, roughly three-fourths of all shots would land inside the kill zone with the larger dart point. Of course, we should not make too much of these particular numbers, given that we are rather inexperienced atlatlists. Collecting additional accuracy data in future trials with experienced atlatlists (sensu Grund Reference Grund2017) could potentially lead to more precise accuracy estimates. Nonetheless, our numbers are instructive in showing that small differences in projectile-point mass may have accuracy effects of sufficient magnitude to appreciably affect hunting success. If so, under certain economic conditions, we should expect strong selection for larger points, given the ostensible performance gains of increasing point size.
Our observations on penetration depths point in the opposite direction. We observed that large points with a mass of 30 g could be expected to penetrate to a depth of 2 cm. By decreasing point size to 18 g, expected penetration depth more than doubles to approximately 5 cm. If we assume that an ungulate kill zone is spherical in shape with a radius of 15 cm, we should expect that, near the margins of the kill zone, the depth to projectile-point contact would be 8.5 cm greater than at the center. Four cm of increased penetration depth, which is roughly half that distance, could therefore be expected to make a substantial difference between a projectile point entering the kill zone or not in a high proportion of on-target shots. We should therefore expect selection for small points in the interest of increasing penetration.
Perhaps most interestingly, the fact that accuracy and penetration respond to projectile-point mass in opposite directions—at least potentially so—offers a hypothetical explanation for why archaeologically observed projectile points tend to exhibit modal sizes. It may be that those modes reflect optimal trade-offs between accuracy and penetration as a function of point mass, which Christenson (Reference Christenson1986) and Hughes (Reference Hughes1998) have previously suggested. We might further expect different optima for atlatl and bow-and-arrow technology, given the differences in firing mechanisms. Indeed, modal size differences between atlatl dart points and arrow points are well documented (Christenson Reference Christenson1986; Hildebrandt and King Reference Hildebrandt and King2012; Shott Reference Shott1997; Thomas Reference Thomas1978).
This potential trade-off leads us to consider an updated technological hypothesis for increasing projectile-point size on the Andean Altiplano. As Andean populations grew, increasing raw material competition would have diminished access to high-quality dart shafts of appropriate length, which would have reduced dart mass and compromised dart accuracy. Atlatl producers would have offset such accuracy losses by increasing projectile-point mass, at least up to the point that resultant losses in dart penetration overwhelmed the gains in accuracy. Additional theoretical and experimental work aimed at validating the accuracy–mass relationship and identifying the statistical structure of theoretical point-size optima for different projectile technologies would be invaluable.
Summary and Conclusion
This study began with the observation of an unusual case of projectile-point enlargement from the Early Archaic through the Late Archaic periods on the Andean Altiplano. We presented a series of candidate explanations, including dart-penetration and dart-accuracy hypotheses. We tested expectations derived from these two hypotheses, performing atlatl test trials with variably sized projectile points. We opted for a relatively naturalistic experimental design to gain broad insights into the production and use dynamics of bone atlatls, which are known from Andean archaeology. Following this naturalistic approach rather than a more controlled experimental design has come at some cost to analytical certainty. Nonetheless, several clear qualitative observations were made, and at least one quantitative signal rose above the noise.
First, we found that a camelid radioulna atlatl system can be produced relatively quickly—in less than a single day given access to suitable materials. Production costs are thus surprisingly low, counter to our initial expectations. We further observed that the medullary cavity of the radioulna is a key morphological feature that (1) overcomes the atlatl's unusual counter-arcing geometry and (2) creates a convenient resting channel for stabilizing the dart before launch. Second, we confidently rejected our prediction that heavier points penetrate deeper than lighter ones. In fact, the results indicated that increasing projectile-point mass reduced dart penetration. Third, we tentatively concluded that mass does not significantly affect accuracy, but we were unable to rule out a potential relationship given that one of our analyses produced a statistically significant result, suggesting a potentially latent effect.
These observations lead us to update our technological model for increasing projectile-point size on the Altiplano. We consider that projectile-point size reflects a trade-off between penetration and accuracy. Increasing projectile-point size in the Altiplano Archaic may reflect attempts to compensate for diminishing accuracy as the raw materials for dart production became increasingly scarce in a resource-limited landscape. We underscore that our working model for projectile-point size increase is provisional—contingent on one sound premise and another admittedly ambiguous one.
Whether or not the posited relationship between mass and accuracy proves valid, alternative models also require consideration. We further recognize that the models are not mutually exclusive. Recall that one hypothesis considered earlier envisions that population migration drove changes in projectile-point size. Another posits that point enlargement on the Altiplano occurred as a result of increasing territoriality and violence. Readers will certainly envision other potential explanations. Tests derived from the various hypotheses and drawing on various lines of archaeological evidence are needed. For example, the migration model predicts changes in the genetic structure of Altiplano Archaic populations. The threat-display model predicts increasing interpersonal violence rates over time and increasingly localized isotopic signatures in human skeletal remains. We are confident that such multifaceted analytical approaches will ultimately resolve the question of why projectile-point size increased on the Andean Altiplano and perhaps other times and places. Such basic insights into allometric phenomena will contribute to our understanding of culture change in human societies.
Acknowledgments
Virginia Incacoña, Juan Incacoña, and Mateo Incacoña (Community of Totorani, Puno, Peru) helped obtain the camelid bone for this experiment. Kevin Smith (UC Davis) provided consultation on atlatl production. Damien Caillaud (UC Davis) provided statistical consultation. Trenton Yackzan, Caleb Weeks, Thomas Stull, and Adam Cooperman (Sudwerk Brewery) and Gregory Wada (UC Davis) assisted with refrigeration of the ballistic gel target. Ondrej Uma assisted with constructing the plywood ballistic gel mold for this study. Freeman George (UC Davis) assisted with the experiments. Christyann Darwent (UC Davis) provided lab space. Nicolas Zwyns (UC Davis) provided flintknapping materials and advice. Sara Watson (UC Davis) assisted with efforts to heat-treat California cherts, although those materials were not ultimately used in this study. UC Davis Department of Anthropology Undergraduate Research Program provided financial support. Members of the UC Davis Forager Complexity Lab group and four anonymous reviewers provided valuable feedback on this study. Author contributions: RH and CC designed the research; CC replicated the tools and performed the experiments; RH and CC performed the statistical analysis; LF and RH analyzed the archaeological projectile points; and CC and RH wrote the article. The authors declare no competing interests.
Data Availability Statement
All data to support our analysis and make it reproducible are provided in the article.